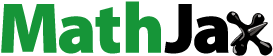
Abstract
Diseases of the posterior segment of the eye are common causes of blindness and can be difficult to treat due to their location. Recently, there has been increased interest in the use of the suprachoroidal space to deliver therapeutics to the posterior segment. This space is accessible through a trans-scleral approach and blunt dissection of the adjacent scleral and choroidal tissues. However, despite recent commercial interest, there are few tools designed specifically to provide targeted delivery of therapeutics to a localised region within the suprachoroidal space. Therefore, we designed and prototyped a novel navigational catheter system for the targeted delivery of payloads within the suprachoroidal space. The system consists of a customised catheter tip designed to minimise blunt dissection stresses on neighbouring tissues, a mechanism for controlled catheter navigation, and a method for targeted delivery of large payloads. A customised in vitro model of the eye was also designed to visually demonstrate the capability of the catheter system to controllably navigate within the suprachoroidal space and deliver a targeted payload. This system can enable the delivery of large therapeutic payloads to the eye for the treatment of posterior eye diseases, thereby impacting the development and availability of vision-saving treatments.
Introduction
Diseases of the retina, such as age-related macular degeneration [Citation1] and diabetic retinopathy [Citation2], are common causes of blindness. While the pathophysiology of these diseases varies, their location in the posterior segment has historically made drug delivery challenging. These diseases, or stages thereof, are commonly treated by repeated intravitreal injections [Citation3]. However, this method precludes the targeted delivery of novel therapies like retinal implants [Citation4], extracellular scaffolds [Citation5,Citation6], adeno-associated viral vectors [Citation7,Citation8], cellular transplants [Citation9,Citation10], and other genetic therapies [Citation11] due to restrictions on size or location of the injected payload. Another potential delivery medium to the posterior segment is through the suprachoroidal space (SCS) [Citation12]. The SCS spans the perimeter of the ocular globe and can be accessed via a trans-scleral approach with blunt dissection of the neighbouring chorioretinal and scleral tissues. To date, the SCS has been utilised for many purposes, including drug delivery [Citation13–15], retention drainage of the anterior chamber [Citation16], drainage of choroidal effusions [Citation17], and repair of retinal detachment [Citation18]. Moreover, drug delivery within the SCS can increase the efficacy and bioavailability of the administered therapeutic [Citation19,Citation20], potentially reducing adverse side effects caused by delivery to other ocular tissues.
Recently, there has been some commercial interest in developing tools or systems for the delivery of therapeutics to the SCS. For example, Clearside Biomedical has developed a microneedle for direct injection of agents into the SCS. While this methodology has demonstrated enhanced pharmacokinetics compared to traditional intravitreal injection [Citation21], it cannot target localised regions of the SCS for delivery. Another system, the iTrack Microcatheter by iScience, was developed to navigate the SCS and deliver therapeutics, as demonstrated in minipigs [Citation22]. However, this system does not provide steerability of the catheter, making navigation through the SCS laborious, recursive, and unpredictable.
The difficulty associated with navigating instruments through the SCS precludes clinical adoption and the availability of novel therapies for individuals with vision-threatening disease. Therefore, we designed a novel catheter system for the targeted delivery of large payloads, consistent with novel therapeutics, within the SCS. The catheter tip is designed to minimise stress to the tissues defining the SCS and, in combination with novel design features for controlled steerability, has the potential to deliver large payloads consistent with advanced therapies to targeted locations in the SCS.
Materials and methods
Principles of the catheter design
Current use of catheters in the SCS is limited. However, previous studies used existing blunt- or olive-tipped catheters in the SCS [Citation12]. To the best of our knowledge, there are no navigational catheter systems for the delivery of large payloads designed specifically for use in the SCS. The following criteria are met with the design of our system: 1) the catheter tip minimises blunt dissection stresses to SCS-defining tissues, 2) the catheter tip is controllably articulated, and 3) the catheter facilitates the targeted delivery of large payloads within the SCS.
Catheter tip
The catheter tip should facilitate the blunt dissection of the choroid from the sclera. The choroid is a highly elastic network of dense vasculature whereas the sclera is a thicker and less elastic tissue. Access to and use of the SCS is not likely to cause damage to the sclera, but damage to the choroid can cause haemorrhaging, choroidal perforations, and retinal detachments, which may result in blindness. Therefore, the catheter tip should minimise pressure and potential damage to the choroid through the use of design features.
Three fundamental catheter tip designs are proposed. All three designs were derived from a common 1:1 cross-sectional ratio (height:width). To facilitate blunt dissection at the catheter tip, all tips featured a bevelled design. Catheter tip “A” featured a single bevel, whereas tips “B” and “C” had an asymmetric double-bevel design, with tip “C” adding an additional taper to “B”. Computer-aided design models of these three tip designs, as well as two existing tips (blunt- and olive-tipped), were rendered in Solidworks (Dassault Systèmes, Vélizy-Villacoublay, France; Version: 2019) and are shown in . The asymmetric double bevel of tips “B” and “C” was designed to provide greater contact area with the choroid as opposed to the sclera.
Figure 1. Computer-aided design models of the catheter tips. Isometric (left) and side (right) profiles are provided for each catheter tip model generated. Novel catheter tip designs “A” (subfig A), “B” (subfig B), and “C” (subfig C) are shown. Existing blunt- and olive-tipped catheter tip designs (subfigs D and E, respectively) are also shown.
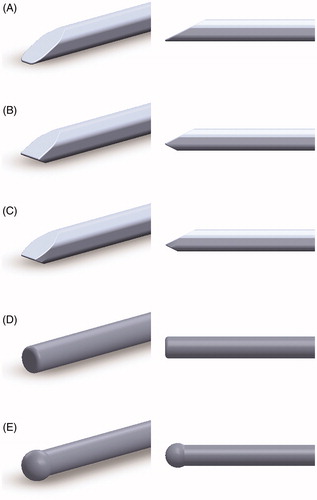
Catheter tip articulation
The natural curve of a catheter (resulting from manufacturing, storage, and handling) does not normally match the curvature of the human eye. Therefore, inserting the catheter into the SCS without control over the tip trajectory results in friction, stalling, and damage to either the choroid or sclera. Therefore careful and controlled navigation of the catheter is needed. We defined catheter navigation as the motion resulting from the combined actions of tip articulation and drive of the catheter itself. Here, catheter tip articulation was accomplished by longitudinal bifurcation of the catheter shaft to produce two opposing members connected at the tip. These opposing members (referred to as “sliding layers”) are shifted relative to each other to produce uniform flex of the entire catheter. shows a simple Solidworks simulation of this concept using a standard blunt-tipped catheter.
Figure 2. Solidworks finite-element simulation of the design for catheter articulation. A blunt-tipped catheter was bifurcated and uniform force was applied to the top member while the bottom was fixed, demonstrating uniform flex of the catheter. The outline represents the original catheter location prior to applied force.

This design produces uniform flex of the entire catheter, but it is more desirable to articulate the catheter tip alone. Therefore, a semi-rigid sheath is introduced to span the length of the catheter and minimise uniform catheter flex in favour of isolated tip articulation. Notably, in this design, the sheath also enters the SCS and must be flexible enough to bend with variable eye curvature. To facilitate precise and accurate control of tip articulation, an electric motor is used to translate the sliding layers relative to each other. Through combined use of the sheath and motor, catheter flex would be refined to precise, accurate, and controllable tip articulation. Drive of the catheter itself is accomplished by the operator moving the entire system by hand.
Targeted delivery of payloads
The catheter system should facilitate the delivery of large payloads to targeted regions of the SCS. Combined use of the catheter and sheath permits this coordination. Upon insertion, the sheathed catheter is navigated to a targeted location in the SCS. The catheter is then withdrawn, leaving behind the sheath which serves as a direct port to the targeted region of the SCS. Using the sheath, a payload is introduced and delivered to the eye.
Catheter tip modeling
Computer-based modelling was used to evaluate the stresses applied to the SCS-defining tissues by each catheter tip design. This modelling only provided stress calculations for the purpose of identifying a preferential catheter tip design and was not meant to provide a comprehensive simulation of the catheter as it navigated the SCS. A model of the eye with an SCS was generated in Solidworks using two concentric spherical shell features representing the scleral (outer layer) and the chorioretinal (inner layer) tissues. The overall diameter of the eye was 62.5 mm, with a scleral and chorioretinal thickness of 1.25 mm and 0.5 mm respectively (roughly 2x that of the human eye [Citation23,Citation24]). This scale was chosen to be consistent with accessible fabrication techniques and equipment, which facilitated our rapid iteration and prototyping. The two tissue layers were separated by 0.15 mm for meshing purposes, and the model was reduced to a single quadrant to help reduce simulation run-time and demand on computer resources. Catheter models were scaled appropriately for the eye and placed at a consistent position just prior to insertion into the SCS ().
Figure 3. Explicit Dynamics simulation setup for comparative assessment of catheter tip designs. Shown here is the spatial orientation between the blunt-tipped catheter, chorioretinal (inner layer of semi-sphere), and the scleral (outer layer of semi-sphere) tissues immediately prior to insertion.
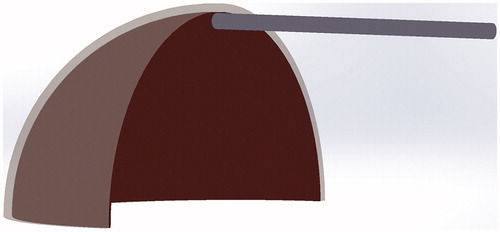
All bodies (eye and catheter models) were imported into ANSYS Explicit Dynamics (Ansys Inc, Canonsburg, PA, USA; Version: 2018 Student Edition) for finite element analysis. The Explicit Dynamics simulation type was chosen to model nonlinear multibody dynamic behaviour over short periods of time. First, representative material properties were applied to the three bodies. The scleral and chorioretinal tissues were given custom material settings consistent with those found in literature [Citation25,Citation26], with elastic moduli of 2.0 MPa and 0.6 MPa respectively, densities of 1.077 g/cm3 and 1.063 g/cm3 respectively, and Poisson’s ratios of 0.47. The catheter material was ethylene vinyl acetate (EVA), a tough, flexible plastic with an elastic modulus of 24.5 MPa, a density of 9.50 g/cm3, and Poisson’s ratio of 0.48. EVA is a commonly used polymeric biomaterial in intravitreal devices and catheters [Citation27].
Second, analysis settings were applied. Meshing of the bodies was performed using the automeshing feature. A resolution value of 3 was chosen, which provided a relatively fine mesh that remained under the ANSYS Student Licence limit of 32,000 nodes/elements. For all considered models, the number of nodes and elements was approximately 5000 and 20,000, respectively. Total simulation time was set to 0.7 s and time steps were program-controlled. Automatic mass scaling, a feature that adds mass to the smallest elements to reduce solution time, was implemented with a maximum element scaling value of 100. Contacts between all bodies were defined as frictionless.
Third, transient forces, pressures, and displacements representative of those experienced by the SCS during cannulation were applied within ANSYS Mechanical. The eye was assumed to be fixed at the flat planar surfaces along the rim of the scleral outer layer. A pressure of 2 kPa (equivalent to 15 mmHg) was applied to the inner chorioretinal surface, comparable to normal intraocular pressure in the human eye. An initial force was used to pull a section of the chorioretinal tissue near the catheter away from the sclera so that the catheter head could be advanced into the space unimpeded. This force was immediately removed after catheter insertion. To drive the catheter, a remote displacement condition at 5 cm/s was applied to the planar surface at the rear of the body. All other translational and rotational motions at the rear surface were fixed. This effectively directed the catheter through the SCS as a surgeon would, holding a section of the catheter body rigid and driving it forward.
Nodal measurements of equivalent stress, equivalent strain, and deformation were calculated and recorded for all three bodies and at each time step. Data used for comparative analysis of catheter tip designs were taken from the last time point, after full insertion of the catheter into the SCS. Data was only evaluated from nodes where model deformation was >1 mm, corresponding to regions of the model that were affected by catheter insertion.
Catheter stress-payload ratio (SPR)
When delivering payloads to the posterior eye through the SCS, the ideal solution would maximise payload delivery potential while minimising induced stress. To quantify these competing ideals, we defined the catheter stress-payload ratio (SPR), in units of Pascal/mm2, as:
(1)
(1)
where
is the mean stress induced on the SCS along the catheter length, and A is the cross-sectional area of the catheter shaft. Thus, lower values of SPR indicate a decreased induced stress on opposing tissues and/or an increased cross-sectional area, corresponding to a greater payload delivery potential.
Catheter system fabrication
Catheters were fabricated by casting a two-part urethane compound at 50 D Shore hardness (Smooth-On Inc, Macungie, PA, USA; Model: Task 14) into a customised three-piece mould. The mould consisted of two mating blocks milled (Haas Automation Inc, Oxnard, CA, USA; Model: 40-Taper Mill) from aluminium block with a third brass shim insert to bifurcate the catheter shaft, and produced catheters at 75 mm in length, 2 mm in height, and 4 mm in width (2x scale). The bifurcation was 0.1 mm in thickness and extended through the catheter length, ending 4 mm from the catheter tip. Catheters were fabricated at a 2x scale (compared to the ideal size of a catheter for use in humans) for ease of machining. The material hardness values were chosen to permit flexibility while maintaining necessary rigidity to translate force along the sliding layers at the 2x scale.
The catheter sheath was fabricated by casting the same two-part urethane compound at 50 D Shore hardness into a customised mould. The mould consisted of two concentric extruded ovals and was 3 D printed from an FDM printer (Prusa Research, Prague, Czech Republic; Model: MK3S) using water-soluble polyvinyl alcohol filament. After curing, the mould was placed in a perturbed water bath to release the sheath. Sheaths were fabricated with a major axis of 4.5 mm, a minor axis of 2.5 mm, and were approximately 0.7 mm in thickness. The sheath extended the length of the catheter shaft, leaving 8–10 mm of the tip exposed.
An electromechanical mount was designed to provide controlled tip articulation by attaching the sliding layers to an electric motor. The electromechanical mount was 3 D printed using a stereolithography 3 D printer (Formlabs Inc, Somerville, MA, USA; Model: Form 2 with Clear RS-F2-GPCL-04 resin) and consisted of a translational and fixed element, which attached to the layers of the bifurcated catheter. The translational element featured a linear rack and was connected to a servomotor (Tower Pro Pte Ltd, China; Model: SG90) with an attached pinion gear. The servomotor was programmed using an Arduino microprocessor (Arduino, Ivrea, Italy; Model: Arduino Uno) to provide linear motion of the translational element, and ultimately, to articulate the catheter tip. The servomotor position was controlled by a series of foot pedals (URBEST; Model: TFS-01) to incline or decline the catheter tip in adjustable angle increments.
A customised enclosure was designed and 3 D printed to anchor the electromechanical mount, servomotor, catheter, and sheath into a single, space-efficient profile. The sheath was anchored to the case using a 3 D printed customised press-fit collar. An example assembled navigational catheter system with labelled components is shown in .
Figure 4. (Left) The assembled case (E) with catheter (A), sheath (B), collar (C), electromechanical mount (D), and servomotor (F). The lid of the enclosure was removed for the picture. (Right) The assembled case (A) alongside the control box (B) with Arduino and breadboard leading to the three foot pedals (C).
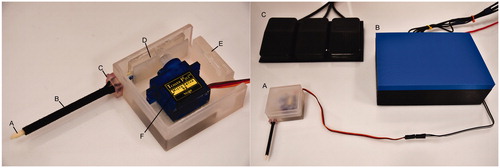
In vitro model
A customised in vitro model was created to visually demonstrate the ability of the navigational catheter system to reach and deliver a payload to a targeted location. The in vitro eye model was fabricated at a 2x scale (60 mm diameter), consistent with the scale of the catheter. This model represented the whole eye and consisted of an oblate spheroid rubber membrane filled with water which was coated with ballistics gel (Clear Ballistics LLC, Fort Smith, AR, USA; Model: 10% gelatin). Ballistics gel was chosen to provide a transparent view of the catheter as it interacted with the model, and layers were between 2 and 3 mm thick. This model also provided qualitative material characteristics of the eye as the vitreous humour was represented as a liquid (the water), the chorioretinal complex was represented as a thin and easily perforated elastic (the rubber membrane), and the sclera was represented by a denser and thicker elastic (the ballistics gel) which provided shape to the model. In this model, the SCS was represented as the interface between the membrane and the ballistics gel. Successful access to the SCS analog was available following incision through the scleral analog, and without puncturing the chorioretinal analog. Internal pressure within the model was controlled using a cannula attached to a water-filled reservoir on an adjustable-height post. The model was also produced in a variant that included a spatial restriction on the fluid-filled membrane. This restriction provided non-uniform curvature to the model, analogous to curvature changes that can occur in human eyes, and represented a navigational challenge for evaluation of the catheter system.
Data analysis
ANSYS mesh convergence was determined by unpaired t-test of SCS stress from repeated simulations using the blunt-tipped catheter with small and large mesh sizing for all bodies. The mean and standard deviation of SCS stress data were calculated for each catheter tip design. The SPR was calculated using mean SCS data and known catheter cross-sectional areas from the Solidworks models. ANOVA with Tukey post hoc was used to determine statistical significance between catheter tip designs. Statistical analysis was performed using SPSS (IBM Corporation, Armonk, NY, USA; Version 26) and statistical significance was accepted at p ≤ 0.05.
Results
Catheter tip evaluation
There was no statistically significant difference in SCS stress data from repeated blunt-tipped catheter simulations with varied mesh sizes (p = 0.88), indicating mesh convergence in the ANSYS model.
provides SCS stress data from catheter tip designs, catheter cross-sectional areas, and resulting SPR. There was a statistically significant difference in SCS stress between catheters (p < 0.001). Differences in mean SCS stresses between catheter tip design are provided in . Briefly, tips “C” and olive provided less stress to the SCS than tips “A” and blunt (p < 0.001). While there was no statistically significant difference in SCS stress between tips “B”, “C”, and olive, the difference between tip “C” and “B” approached statistical significance (p = 0.062). The SPR of tip “C” was 10.9% and 13.8% lower than “B” and olive, respectively.
Table 1. Stress data from dynamics simulations of catheter tip designs in the suprachoroidal space.
Table 2. Differences in mean suprachoroidal space stresses between catheter tip designs.
We also assessed the effect of catheter cross-sectional ratio on SPR. Since catheter tip “C” had the lowest SPR, we evaluated models of that tip design at different ratios, as shown in . There was a statistically significant difference in SCS stress between ratios (p = 0.05). Differences in mean SCS stresses with catheter tip “C” at multiple cross-sectional ratios are provided in . Briefly, SCS stress was statistically significantly greater with the 1:1.5 ratio than the 1:1 ratio (p = 0.04). However, SPR revealed considerable differences between ratios, with 1:2 being 51.2% and 31.8% lower than 1:1 and 1:1.5, respectively.
Table 3. Stress data from dynamics simulations of catheter tip “C” at various ratios in the suprachoroidal space.
Table 4. Differences in mean suprachoroidal space stresses between catheter tip cross-sectional ratios.
The assembled catheter system
Catheter tip “C” in a 1:2 ratio was selected for fabrication due to its ability to impart minimal stress to the SCS and its superior SPR. The catheter system was fabricated and assembled according to the Methods section. Foot pedal actuation of the servomotor, which drove the translational element of the electromechanical mount, resulted in a ±35 degrees range of catheter tip articulation about the neutral position. With the adjustable angle of servomotor rotation per trigger set to 5 degrees via Arduino software, the catheter tip achieved a resolution of 2.5 degrees per foot pedal step over a central range of ±30 degrees. After removal of the collar, the sheath and catheter can be decoupled. Upon withdrawal of the catheter, delivery of a payload via the remaining embedded sheath was possible.
Visual demonstration of the catheter system
The in vitro model was cannulated and internal pressure was set between 10 and 21 mmHg, comparable to normal human intraocular pressure. Insertion of the sheathed catheter into the membrane-gel interface was performed through a 3–5 mm incision on the external face of the ballistics gel, roughly 15 mm superior to the equator. The sheathed catheter was then driven through the interface to generate an SCS analog through blunt dissection of the membrane from the ballistics gel. The combined action of catheter tip articulation and drive was used to successfully navigate along the curvature of the model through the interface. shows the catheter and sheath of the system embedded in the model and at varying catheter tip articulations. Notably, failure to appropriately articulate the catheter tip during navigation resulted in bunching against one material or the other and subsequent stalling.
Figure 5. Catheter tip articulation in the in vitro model. From left to right, the catheter at maximum articulation towards the chorioretinal analog, articulation to match the curvature of the model, and maximum articulation towards the scleral analog.
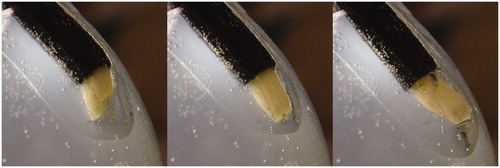
The catheter was also navigated through the model with spatial restrictions imposed on the fluid-filled membrane (). In this example, the posterior pole of the model was used as a target with the spatial restrictor being located approximately at the equator. The local changes in curvature caused by this restrictor were impossible to overcome without catheter tip articulation, as repeated attempts resulted in stalling and the inability to drive the catheter farther. After articulation and navigation beyond the restrictor, the mechanism for targeted delivery of a payload was demonstrated. The collar was removed and the catheter was withdrawn while the sheath was held in place. The remaining sheath provided a direct port to the targeted location in the model. A red dye was injected through the sheath to visualise the potential of the catheter system to deliver payloads to a targeted location.
Figure 6. Catheter tip articulation in the in vitro model as it is advanced over a spatial restrictor between the fluid-filled membrane and the ballistics gel. From left to right, the catheter approaching the obstacle while matching curvature of model, re-articulation and drive of the catheter to overcome the obstacle, and targeted delivery of a payload. For delivery of the payload, the catheter was withdrawn to leave behind the sheath, which was used to inject a red dye for illustration purposes.
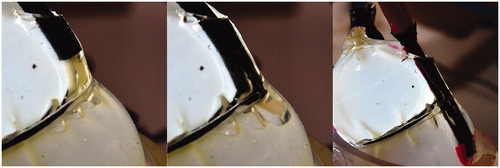
Discussion
Diseases of the posterior segment may result in blindness, and the delivery of novel therapeutics through intravitreal injection is often precluded. The SCS is an alternative route to deliver therapeutics to the posterior segment, however, to the best of our knowledge, there are no navigational catheter systems for the delivery of large payloads designed specifically for use in the SCS. Therefore, we designed a system that met the following criteria: 1) the catheter tip must minimise stress applied to SCS-defining tissues, 2) the catheter tip must be controllably articulated to permit navigation, and 3) the catheter must facilitate the targeted delivery of large payloads.
To balance the first and third criteria, a preferential catheter tip design was determined using computer-based modelling. Five catheter tips and an eye model were generated using computer-aided design software and imported to ANSYS, wherein the catheters were inserted into the eye model. Stress data from the choroidal and scleral tissues were exported to assess the effect of catheter tip design. Furthermore, the relative ability of a catheter to deliver greater payloads while minimising stress to the neighbouring opposing tissues was quantified using the stress-payload ratio (SPR). Catheter tips “B”, “C”, and olive induced the least stress, but tip “C” provided the lowest SPR by more than 10%. Tip “C” was then selected to determine the effect of catheter cross-sectional area on the SCS stress and SPR. There was no statistically significant difference in stress between ratios 1:1 and 1:2, and both were lower than 1:1.5, but the 1:2 ratio had a greatly reduced SPR. While a minimal value of SPR indicates an ideal balance between catheter induced stresses to SCS-defining tissues and the ability to deliver large payloads, this does not mean that the preferential catheter tip design induces the least amount of stress to the SCS. Indeed, undue stresses caused to the chorioretinal tissues can result in blindness. Further evaluation of these catheter tip designs and ratios with ex vivo or in vivo animal models is necessary to ensure safety of use. Nevertheless, within the scope of our work and available resources, these results indicate that tip “C” at a 1:2 ratio was the most effective tip design.
To address the second criterion, catheter articulation was accomplished through bifurcation of the shaft and the relative sliding of the resulting two layers. Articulation was confined to the catheter tip alone through the use of a sheath, which extended along the length of the catheter. An electromechanical mount was designed and implemented for precise and accurate control of catheter tip articulation, and a customised case was developed to protect and coordinate all components. Bifurcated catheters with tip design “C” in a 1:2 ratio were fabricated and assembled into the system. To visually demonstrate the ability for the navigational catheter system to reach and deliver a payload to a targeted location, a customised in vitro model was developed to represent the eye and SCS. The use of these in vitro models permitted direct and real-time visualisation of catheter tip articulation which emphasised its necessity in navigating past curvatures and obstacles that occur naturally in the eye. Furthermore, this model permitted visualisation of targeted payload delivery, which occurred via the sheath after withdrawal of the catheter. These valuable visual demonstrations required no prior experience and were performed without perforation of the liquid-filled membrane. Combined hand- and foot-driven control corresponding to catheter drive and catheter tip articulation, respectively, required minimal coordination from the operator, and the resulting navigation occurred with fluidic movements.
There were limitations to the current report. Material properties of the choroid and sclera in the computer-based modelling was based on studies using enucleated eyes and may not faithfully represent in vivo material properties of the SCS. Further, materials for the in vitro model were selected to prioritise visualisation of the catheter during navigation and only qualitatively represented the SCS. Additional studies using the navigational catheter system with ex vivo or in vivo animal models are necessary to establish the utility and safety of the design presented in this report. Nevertheless, this report describes the concept development and prototyping of a novel navigational catheter system for the targeted delivery of payloads within the SCS. This system can enable the delivery of large payloads consistent with modern therapeutics to the posterior segment for the treatment of ocular disorders, thereby impacting the future development and availability of vision-saving treatments.
Acknowledgments
The authors acknowledge the contributions of Mr. Andrew Graham for tooling and fabrication.
Disclosure statement
The authors have a provisional patent issued for the described technology issued 11/2019.
Data availability statement
Data available upon request.
Additional information
Funding
References
- Wong WL, Xinyi S, Xiang L, et al. Global prevalence of age-related macular degeneration and disease burden projection for 2020 and 2040: a systematic review and meta-analysis. Lancet Glob Health. 2014;2:e106–e116.
- American Diabetes Association. Statistics About Diabetes. 2017. Available from: https://www.diabetes.org/resources/statistics/statistics-about-diabetes
- Del Amo EM, Urtti A. Current and future ophthalmic drug delivery systems. A shift to the posterior segment. Drug Discov Today. 2008;13:135–143.
- Stronks HC, Dagnelie G. The functional performance of the Argus II retinal prosthesis. Expert Rev Med Devices. 2014;11:23–30.
- Al-Ubaidi MR, Naash MI, Conley SM. A perspective on the role of the extracellular matrix in progressive retinal degenerative disorders. Invest Ophthalmol Vis Sci. 2013;54:8119–8124.
- Yao J, Tao SL, Young MJ. Synthetic polymer scaffolds for stem cell transplantation in retinal tissue engineering. Polymers. 2011;3:899–914.
- Lee CS, Bishop ES, Zhang R, et al. Adenovirus-mediated gene delivery: potential applications for gene and cell-based therapies in the new era of personalized medicine. Genes Dis. 2017;4:43–63.
- Ding K, Shen J, Hafiz Z, et al. AAV8-vectored suprachoroidal gene transfer produces widespread ocular transgene expression. J Clin Invest. 2019;129:4901–4911.
- Pearson RA, Barber AC, Rizzi M, et al. Restoration of vision after transplantation of photoreceptors. Nature. 2012;485:99–103.
- Singh R, Cuzzani O, Binette F, et al. Pluripotent stem cells for retinal tissue engineering: current status and future prospects. Stem Cell Rev Rep. 2018;14:463–483.
- Touchard E, Berdugo M, Bigey P, et al. Suprachoroidal electrotransfer: a nonviral gene delivery method to transfect the choroid and the retina without detaching the retina. Mol Ther. 2012;20:1559–1570.
- Chiang B, Jung JH, Prausnitz MR. The suprachoroidal space as a route of administration to the posterior segment of the eye. Advanced Drug Delivery Reviews. 2018;126:58–66.
- Olsen TW, Feng X, Wabner K, et al. Cannulation of the suprachoroidal space: a novel drug delivery methodology to the posterior segment. Am J Ophthalmol. 2006;142:777–787.
- Patel SR, Lin ASP, Edelhauser HF, et al. Suprachoroidal drug delivery to the back of the eye using hollow microneedles. Pharm Res. 2011;28:166–176.
- Goldstein DA, Do D, Noronha G, et al. Suprachoroidal corticosteroid administration: a novel route for local treatment of noninfectious uveitis. Transl Vis Sci Technol. 2016;5:14.
- Manasses DT, Au L. The new era of glaucoma micro-stent surgery. Ophthalmol Ther. 2016;5:135–146.
- Stefater JA, Eliott D, Kim LA. Drainage and analysis of suprachoroidal fluid in a patient with acute systemic lupus erythematous. Am J Ophthalmol Case Rep. 2017;5:29–32.
- El Rayes EN, Oshima Y. Suprachoroidal buckling for retinal detachment. Retina. 2013;33:1073–1075.
- Kim YC, Oh KH, Edelhauser HF, et al. Formulation to target delivery to the ciliary body and choroid via the suprachoroidal space of the eye using microneedles. Eur J Pharm Biopharm. 2015;95:398–406.
- Jung JH, Desit P, Prausnitz MR. Targeted drug delivery in the suprachoroidal space by swollen hydrogel pushing. Invest Ophthalmol Vis Sci. 2018;59:2069–2079.
- Edelhauser HF, Verhoeven RS, Burke B, et al. Intraocular distribution and targeting of triamcinolone acetonide suspension administered into the suprachoroidal space. Invest Ophthalmol Vis Sci. 2014;55:5259.
- de Smet MD, Lynch JL, Dejneka NS, et al. A subretinal cell delivery method via suprachoroidal access in minipigs: safety and surgical outcomes. Invest Ophthalmol Vis Sci. 2018;59:311–320.
- Olsen TW, Aaberg SY, Geroski DH, et al. Human sclera: thickness and surface area. Am J Ophthalmol. 1998;125:237–241.
- Manjunath V, Taha M, Fujimoto JG, et al. Choroidal thickness in normal eyes measured using Cirrus HD optical coherence tomography. Am J Ophthalmol. 2010;150:325–329e1.
- Friberg TR, Lace JW. A comparison of the elastic properties of human choroid and sclera. Exp Eye Res. 1988;47:429–436.
- Choh V, Su X, Vesco C, et al. Densities of bovine ocular components. Invest Ophthalmol Vis Sci. 2009;50:5564.
- Wood NJ, Maddocks SE, Grady HJ, et al. Functionalization of ethylene vinyl acetate with antimicrobial chlorhexidine hexametaphosphate nanoparticles. Int J Nanomedicine. 2014;9:4145–4152.