Abstract
Evidence of successful predation or scavenging in the fossil record represents important palaeobiological data to more thoroughly understanding extinct ecosystems. Shelly coprolites are particularly useful indications of durophagous predation in deposits, as they can have a higher preservational potential than their producers. Here we present a new shelly coprolite from the Silurian (Přídolí) Wallace Shale of New South Wales, Australia. This specimen contains abundant fragments of the trilobite Denckmannites rutherfordi Sherwin, 1968 that show limited disarticulation across exoskeletal sections. We propose that a pterygotid eurypterid was the most likely producer of this coprolite, although trilobites and fishes are not completely excluded as possible trace-makers. In documenting this specimen, we highlight that the Wallace Shale likely preserves a more complex palaeoecosystem than previously thought and renewed efforts to understand this deposit are needed in light of this new insight.
R.D.C. Bicknell [[email protected]], Palaeoscience Research Centre, School of Environmental and Rural Science, University of New England, Armidale, New South Wales, 2351, Australia;
P.M. Smith [[email protected]], Palaeontology Department, Australian Museum Research Institute, Sydney, New South Wales, 2010, Australia; Department of Biological Sciences, Macquarie University, Sydney, New South Wales, 2109, Australia;
J. Kimmig [[email protected]], Abteilung Geowissenschaften, Staatliches Museum für Naturkunde Karlsruhe, Karlsruhe, 76133, Germany.
RECORDS of predation within the fossil record present important information regarding predator–prey dynamics in palaeoecosystems (Brett Citation1990, Citation2003, Kowalewski Citation2002, Klompmaker et al. Citation2019). Injured specimens (Babcock Citation1993, Citation2003, Vinn Citation2009, Citation2017, Citation2018, Bicknell & Paterson Citation2018, Bicknell & Pates Citation2020, Bicknell et al. Citation2018b, Citation2023), drill holes (Kowalewski et al. Citation2000, Hoffmeister Citation2002, Amano Citation2003, Hoffmeister et al. Citation2004, Vinn et al. Citation2021), gut contents (Richter Citation1992, Sues Citation1993, Jago et al. Citation2016, Zacaï et al. Citation2016), and coprolites (Häntzschel et al. Citation1968, Hunt Citation1992, Toom et al. Citation2020, Kimmig & Strotz Citation2017, Kimmig & Pratt Citation2018, Knaust Citation2020, Hunt & Lucas Citation2021) all represent useful evidence of predation. These different records present varying degrees of insight into possible trophic interactions, with the rarer specimens (such as prey within gut contents) presenting much more palaeoecological information (Babcock Citation1993, Zacaï et al. Citation2016, Bicknell & Paterson Citation2018).
Coprolites containing fragmentary animal parts record predation or scavenging and are very useful for reconstructing trophic interactions. Shelly coprolites often reflect shell crushing (durophagous) activity and have a higher preservation potential than their producers (Vannier & Chen Citation2005). Shelly coprolites are well-documented in early to middle Paleozoic deposits (e.g., Vannier & Chen Citation2005, Klompmaker et al. Citation2019) and such examples are usually attributed to activity by durophagous animals, such as trilobites and other euarthropods (e.g., Vannier & Chen Citation2005, Bicknell & Paterson Citation2018, Bicknell et al. Citation2022a), with few examples possibly being produced by early vertebrates (Hunt et al. Citation2012). While the Cambrian coprolite record is excellent (e.g., Vannier & Chen Citation2005, Kimmig & Strotz Citation2017, Kimmig & Pratt Citation2018, Knaust Citation2020), the Ordovician and Silurian records are comparatively poor (Hunt et al. Citation2012). The identification of shelly coprolites within deposits of these time periods therefore presents new evidence for possible durophagous animals. To expand the record of Silurian coprolites (e.g., Rolfe Citation1973, Bischoff Citation1990, Gilmore Citation1992, Edwards et al. Citation1995), and demonstrate novel examples of durophagous predation within late Silurian deposits of Australia, we report a shelly aggregation from the Silurian (Přídolí)-aged Wallace Shale.
Materials and methods
The specimen (AM F158002) reported herein was collected by PMS from near Mirrabooka ‘homestead’ along a tributary of Wattle Creek, at approximately 33°12′27.00ʺS, 148°51′48.72ʺE (originally collected by Sherwin Citation1968) within the Wallace Shale (). This location is slightly north of the old township of Cheesemans Creek (near Orange), at the midpoint between Bathurst and Parkes, central New South Wales (NSW), Australia. The specimen was collected from a single bedding plane that contained numerous Denckmannites rutherfordi Sherwin, Citation1968 (), preserved in articulation, or in the Salterian moulting arrangement. Hence, this specimen was somewhat aberrant for having been crushed, disarticulated, and containing multiple individuals within a constrained region (compare to typical specimens from the same horizon, such as and Sherwin Citation1968, pl. 133). The specimen was coated in magnesium oxide and photographed under low angle LED light with a Canon EOS 5DS. Images were stacked using Helicon Focus 7 (Helicon Soft Limited) stacking software.
Figure 1. Geography, geology, stratigraphy, and bedding plane information for specimen locations within the Wallace Shale. A, Map of Australia showing specimen location (red star) in New South Wales. B, Geological map showing rocks proximal to Mirrabooka ‘homestead’. Red stars indicate specimen location. A simplified stratigraphic column is shown on the right. C, Panoramic view of located where specimens were collected, from exposure of left creek bank – small tributary running east of Wattle Creek. D, Typical specimen of Denckmannites rutherfordi Sherwin, Citation1968 found at the specimen site with Salterian moulting arrangement. Hundreds of individuals comparable to this specimen are uncovered on bedding surfaces.
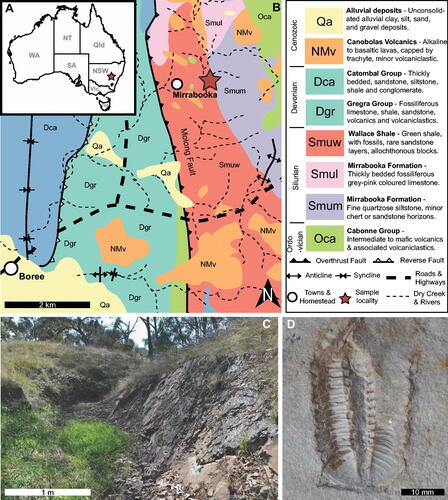
Geological and geographical context
The geological context of this Wallace Shale site was discussed in Sherwin & Rickards (Citation2002, p. 87); hence, a summary is provided here. The unit at the considered site outcrops primarily as a green-grey to olive shale that occasionally splits along bedding planes, although more often splits conchoidally along cleavage planes (Wood Citation1955, Tuckerson Citation1966, Partridge Citation1967). The shale is medium to thickly bedded, with internal laminations only apparent in distinct marker horizons (typically containing course, angular volcanic feldspar and quartz grains). The unit also appears to be enriched in heavy minerals like rutile, zircon, and tourmaline compared to the underlying sequences (Pickett Citation1982). Near Mirrabooka ‘homestead’, and in the surrounding Cheesemans Creek-Spring Creek area, the Wallace Shale conformably overlies the Mirrabooka Formation. Slightly northeast of this, the shale also interfingers with (and may conformably overly) the Borenore and Molong limestones. The unit is conformably overlain by the Bulls Camp Volcanics and disconformably overlain in local patches by Miocene basaltic volcanics (Pogson & Watkins Citation1998).
The Wallace Shale generally hosts boulder beds representing slump deposits. These range in size from relatively small to extremely large (3–450 m) and of various different ages (although locally derived blocks tend to be of a similar age). Most are contemporaneous with the Wallace Shale. However, four outcrops near Mirrabooka ‘homestead’ appear to be Ordovician, hosting conodonts, graptolites, brachiopods, and trilobites similar to those of the basal Malongulli Formation (Sherwin Citation1966, Percival Citation1978, Citation1979, and observations by PMS). The upper part of the unit near Mirrabooka ‘homestead’ also hosts a turbiditic sandstone with subordinate interbeds of shale. This sandstone is reddish-brown or greenish-grey in colour and contains flute casts and invertebrate trails on the bedding surfaces. This upper sandstone sequences was termed the Nyrang Sandstone Member by Sherwin (Citation1971a), who expanded on work by Wood (Citation1955). Presence of turbidites, major slumping, and allochthonous blocks in the unit, along with common planktonic graptolites and small-eyed Denckmannites rutherfordi, suggest that the environment was a relatively deep marine basin. This is further supported by a rather depauperate benthic fauna consisting of Batocara cf. robustus (Mitchell, Citation1924) and an unidentified odontopleurid trilobite, as well as several species of dendroidal graptolites, molluscs, brachiopods, conularids, and corals (Sherwin Citation1968, Citation1971b, Citation1976, Strusz Citation1980, Pickett Citation1982, see Pogson & Watkins Citation1998, table A1.18 for an overview).
Graptolites from the site sampled in the Wallace Shale near Mirrabrook ‘homestead’ give a definitive Přídolí age (Sherwin & Rickards Citation2002). The shale contains a fauna, mainly dominated by monograptid species, that are very similar to those described from the Rosebank Shale and Cowridge Siltstone at Yass, NSW (Jenkins Citation1982, Rickards & Wright Citation1999) and the Humevale Formation, Ghin Ghin, Victoria (Rickards & Garratt Citation1990, Rickards Citation2000, Packham et al. Citation2001). The conodont species Belodella anomalis Cooper, Citation1974 described from an allochthonous (likely contemporaneous) block within the unit at Boree Creek, NSW (Cockle Citation1999) supports a Přídolí age (Farrell Citation2004) for the Wallace Shale. Finally, the upper portion of the unit potentially extends into the Early Devonian further along Wattle Creek, as indicated by the presence of Monograptus cf. uniformis (Tuckerson Citation1966, Sherwin Citation1976).
Description
The shelly aggregation is elongated, 28.9 mm long and 15.1 mm wide (). The edge of AM F158002 is sharp and defined by the dense aggregation of tens of trilobite sections. Due to the shelly composition, the specimen has at least 2 mm relief. Identifiable trilobite sections include pygidia, thoracic segments, and cephala. All fragments belong to Denckmannites rutherfordi. No soft-tissue is preserved.
Discussion
Shelly aggregates within the invertebrate fossil record are typically considered examples of cololite and coprolite bromalites. Comparing AM F158002 with the most recent systematic work on bromalites (Knaust Citation2020), we conclude that the specimen does not completely conform with diagnoses of the described ichnospecies. This has limited our ability to present a formal taxonomic assessment of AM F158002 and we have therefore chosen to leave the specimen in open nomenclature. However, the lack of any evidence for a gut tract surrounding the specimen excludes AM F158002 from the cololite category. Furthermore, the specimen is morphologically comparable to other trilobite-rich aggregates considered coprolites (see Babcock Citation2003, Daley et al. Citation2013, Ding et al. Citation2020, Bicknell et al. Citation2022a). As such, we suggest AM F158002 is likely a coprolite, illustrating the presence of a durophagous predator or scavenger within the Wallace Shale.
Previous examples of eurypterid and fish-rich shelly coprolites have been noted from Silurian-aged deposits (Caster & Kjellesvig-Waering Citation1964, Rolfe Citation1973). Further, Silurian-aged coprolites that show primarily eurypterid fragments also contain trilobite fragments (Caster & Kjellesvig-Waering Citation1964) and are up to four times longer than AM F158002. Both eurypterid and fish-rich shelly coprolites have been attributed to predation by large eurypterids (Caster & Kjellesvig-Waering Citation1964, Rolfe Citation1973, Selden Citation1984, Schmidt et al. Citation2022), based on the co-occurrence of sea scorpions with the coprolites. Large pterygotid eurypterids are known from other Silurian deposits of Australia (McCoy Citation1899, Bicknell et al. Citation2020) and would have been capable of capturing prey with hypertrophied chelicerae (Bicknell et al. Citation2022b) for subsequent shell crushing with re-enforced gnathobasic spines on the large coxal regions of swimming legs (Clarke & Ruedemann Citation1912, Miller Citation2007, Poschmann et al. Citation2017, Haug Citation2020). This contrasts the structurally weaker gnathobasic spines of smaller eurypterid genera that were likely limited to shredding soft prey (Selden Citation1981, Bicknell et al. Citation2018a). Eurypterid fossils have not been identified within the Wallace Shale to date. However, this likely reflects a preservational bias towards biomineralized structures within the formation and a notable lack of soft-bodied fauna. An alternative to eurypterids as producers of this coprolite could be trilobites. The trilobites of the Wallace Shale may have consumed each other, likely targeting smaller individuals, using gnathobasic spines on walking legs (Bicknell et al. Citation2021). However, coprolites produced by Cambrian-aged trilobites show marked disarticulation along exoskeletal sections (Daley et al. Citation2013, Bicknell et al. Citation2022) and AM F158002 lacks this degree of breakage. As such, a trilobite producer is less likely when compared to the eurypterid explanation.
One final possibility is that AM F158002 represents a fish coprolite. Fishes, while rare in Silurian deposits of Australia, have been recorded from some localities (Burrow & Young Citation1999, Burrow & Turner Citation2000), and possible Přídolí-aged ancanthodians and thelodontidid scales are known from the so-called ‘Carribuddy’ Formation (Turner Citation1993). However, ancanthodians may not have been effective at consuming trilobites and antiarch placoderm fishes are not known from Gondwana until the Emsian (Lebedev et al. Citation2022). As such, it is unlikely that fishes produced the coprolite.
Taken together, the most likely coprolite producer was a pterygotid eurypterid. This presents important insight into the fauna that may not be preserved within the formation. This palaeoecosystem was more complex than previously thought and we suggest that additional sampling from other sections of the shale may yield novel fossil material to expand the known palaeodiversity of the deposit.
Disclosure statement
No potential conflict of interest was reported by the authors.
Additional information
Funding
References
- Amano, K., 2003. Predatory gastropod drill holes in Upper Miocene cold seep bivalves, Hokkaido, Japan. The Veliger 46, 90–96.
- Babcock, L.E., 1993. Trilobite malformations and the fossil record of behavioral asymmetry. Journal of Paleontology 67, 217–229.
- Babcock, L.E., 2003. Trilobites in Paleozoic predator-prey systems, and their role in reorganization of early Paleozoic ecosystems. In Predator-Prey Interactions in the Fossil Record. Kelley, P., Kowalewski, M. & Hansen, T.A., eds, Springer, New York, 55–92.
- Bicknell, R.D.C., Holmes, J.D., Edgecombe, G.D., Losso, S.R., Ortega-Hernández, J., Wroe, S. & Paterson, J.R., 2021. Biomechanical analyses of Cambrian euarthropod limbs reveal their effectiveness in mastication and durophagy. Proceedings of the Royal Society of London B: Biological Sciences 288, 20202075.
- Bicknell, R.D.C., Holmes, J.D., Pates, S., García-Bellido, D.C. & Paterson, J.R., 2022. Cambrian carnage: trilobite predator-prey interactions in the Emu Bay Shale of South Australia. Palaeogeography, Palaeoclimatology, Palaeoecology 591, 110877.
- Bicknell, R.D.C., Holmes, J.D., García-Bellido, D.C. & Paterson, J.R., 2023. Malformed individuals of the trilobite Estaingia bilobata from the Cambrian Emu Bay Shale and their palaeobiological implications. Geological Magazine 1–10. DOI:10.1017/S0016756822001261.
- Bicknell, R.D.C. & Paterson, J.R., 2018. Reappraising the early evidence of durophagy and drilling predation in the fossil record: implications for escalation and the Cambrian Explosion. Biological Reviews of the Cambridge Philosophical Society 93, 754–784.
- Bicknell, R.D.C., Paterson, J.R., Caron, J.-B. & Skovsted, C.B., 2018a. The gnathobasic spine microstructure of recent and Silurian chelicerates and the Cambrian artiopodan Sidneyia: Functional and evolutionary implications. Arthropod Structure & Development 47, 12–24.
- Bicknell, R.D.C. & Pates, S., 2020. Exploring abnormal Cambrian-aged trilobites in the Smithsonian collection. Peerj 8, e8453.
- Bicknell, R.D.C., Pates, S. & Botton, M.L., 2018b. Abnormal xiphosurids, with possible application to Cambrian trilobites. Palaeontologia Electronica 21, 1–17.
- Bicknell, R.D.C., Simone, Y., Van der Meijden, A., Wroe, S., Edgecombe, G.D. & Paterson, J.R., 2022. Biomechanical analyses of pterygotid sea scorpion chelicerae uncover predatory specialisation within eurypterids. Peerj 10, e14515.
- Bicknell, R.D.C., Smith, P.M. & Poschmann, M., 2020. Re-evaluating evidence of Australian eurypterids. Gondwana Research 86, 164–181.
- Bischoff, G.C.O., 1990. Coprolites from early and middle Silurian strata of New South Wales. Senckenbergiana Lethaea 70, 209–237.
- Brett, C.E., 2003. Durophagous predation in Paleozoic marine benthic assemblages. In Predator—Prey Interactions in the Fossil Record. Kelley, P.H., Kowalewski, M. & Hansen, H.J., eds, Springer, Boston, MA, 401–432.
- Brett, C.E., 1990. Predation. In Palaeobiology: A Synthesis. Briggs, D.E.G. & Crowther, P.R. eds, Blackwell Press, Oxford, 368–372.
- Burrow, C.J. & Turner, S., 2000. Silurian vertebrates from Australia. In Palaeozoic Verte brate Biochronology and Global Marine/Non–Marine Correlation. Final Report of IGCP 328 (1991–1996). Blieck, A. & Turner, S. eds, Courier Forschungsinstitut Senckenberg, Frankfurt 223, 169–174.
- Burrow, C.J. & Young, G.C., 1999. An articulated teleostome fish from the Late Silurian (Ludlow) of Victoria, Australia. Records of the Western Australian Museum 57, 1–14.
- Caster, K.E. & Kjellesvig-Waering, E.N., 1964. Upper Ordovician eurypterids of Ohio. Palaeontographia Americana 3, 301–358.
- Clarke, J.M. & Ruedemann, R., 1912. The Eurypterida of New York. New York State Museum Memoir 14, 1–628.
- Cockle, P., 1999. Conodont data (Silurian: latest Llandovery–Ludlow) in relation to formations and facies in the Boree Creek area, east-central New South Wales, Australia. Abhandlungen der Geologischen Bundesanstalt 54, 107–133.
- Cooper, B.J., 1974. New forms of Belodella (Conodonta) from the Silurian of Australia. Journal of Paleontology 48, 1120–1125.
- Daley, A.C., Paterson, J.R., Edgecombe, G.D., García‐Bellido, D.C. & Jago, J.B., 2013. New anatomical information on Anomalocaris from the Cambrian Emu Bay Shale of South Australia and a reassessment of its inferred predatory habits. Palaeontology 56, 971–990.
- Ding, Y., Liu, J. & Chen, F., 2020. Ichnology, palaeoenvironment, and ecosystem dynamics of the early Cambrian (Stage 4, Series 2) Guanshan Biota, South China. Geological Journal 55, 77–94.
- Edwards, D., Selden, P.A., Richardson, J.B. & Axe, L., 1995. Coprolites as evidence for plant–animal interaction in Siluro–Devonian terrestrial ecosystems. Nature 377, 329–331.
- Farrell, J.R., 2004. Siluro‐Devonian conodonts from the Camelford Limestone, Wellington, New South Wales, Australia. Palaeontology 47, 937–982.
- Gilmore, B., 1992. Scroll coprolites from the Silurian of Ireland and the feeding of early vertebrates. Palaeontology 35, 319–333.
- Häntzschel, W., El-Baz, F. & Amstutz, G.C., 1968. Coprolites an Annotated Bibliography. Geological Society of America, Boulder, CO.
- Haug, C., 2020. The evolution of feeding within Euchelicerata: data from the fossil groups Eurypterida and Trigonotarbida illustrate possible evolutionary pathways. Peerj 8, e9696.
- Hoffmeister, A.P., 2002. Quantitative Analysis of Drilling Predation Patterns in the Fossil Record: Ecological and Evolutionary Implications. PhD thesis, Virginia Polytechnic Institute and State University, Blacksburg, VA.
- Hoffmeister, A.P., Kowalewski, M., Baumiller, T.K. & Bambach, R.K., 2004. Drilling predation on Permian brachiopods and bivalves from the Glass Mountains, west Texas. Acta Palaeontologica Polonica 49, 443–454.
- Hunt, A.P., 1992. Late Pennsylvanian coprolites from the Kinney Brick Quarry, central New Mexico, with notes on the classification and utility of coprolites. New Mexico Bureau of Mines and Mineral Resources, Bulletin 138, 221–229.
- Hunt, A.P. & Lucas, S.G., 2021. The ichnology of vertebrate consumption: dentalites, gastroliths and bromalites. New Mexico Museum of Natural History Bulletin 87, 1–216.
- Hunt, A.P., Milàn, J., Lucas, S.G. & Spielmann, J.A., 2012. Vertebrate coprolite studies: status and prospectus. New Mexico Museum of Natural History and Science Bulletin 57, 5–24.
- Jago, J.B., García-Bellido, D.C. & Gehling, J.G., 2016. An early Cambrian chelicerate from the Emu Bay Shale, South Australia. Palaeontology 59, 549–562.
- Jenkins, C.J., 1982. Late Pridolian graptolites from the Elmside Formation near Yass. Proceedings of the Linnean Society of New South Wales 106, 167–172.
- Kimmig, J. & Pratt, B.R., 2018. Coprolites in the Ravens Throat River Lagerstätte of Northwestern Canada: implications for the middle Cambrian food web. Palaios 33, 125–140.
- Kimmig, J. & Strotz, L.C., 2017. Coprolites in mid-Cambrian (Series 2-3) Burgess Shale-type deposits of Nevada and Utah and their ecological implications. Bulletin of Geosciences 92, 297–309.
- Klompmaker, A.A., Kelley, P.H., Chattopadhyay, D., Clements, J.C., Huntley, J.W. & Kowalewski, M., 2019. Predation in the marine fossil record: studies, data, recognition, environmental factors, and behavior. Earth-Science Reviews 194, 472–520.
- Knaust, D., 2020. Invertebrate coprolites and cololites revised. Papers in Palaeontology 6, 385–423.
- Kowalewski, M., 2002. The fossil record of predation: an overview of analytical methods. Paleontological Society Papers 8, 3–42.
- Kowalewski, M., Simões, M.G., Torello, F.F., Mello, L. & Ghilardi, R.P., 2000. Drill holes in shells of Permian benthic invertebrates. Journal of Paleontology 74, 532–543.
- Lebedev, O.A., Johanson, Z., Kuznetsov, A.N., Tsessarsky, A., Trinajstic, K. & Isakhodzayev, F.B., 2022. Feeding in the Devonian antiarch placoderm fishes: a study based upon morphofunctional analysis of jaws. Journal of Paleontology 96, 1413–1430.
- McCoy, F., 1899. Note on a new Australian Pterygotus. Geological Magazine 6, 193–194.
- Miller, R.F., 2007. Nineteenth century collections of Pterygotus anglicus Agassiz (Chelicerata; Eurypterida) from the Campbellton Formation, New Brunswick, Canada. Atlantic Geology 43, 197–209.
- Mitchell, J., 1924. New trilobites from Bowning, with notes on Encrinurus and Cordania gardneri. Proceedings of the Linnean Society of New South Wales 49, 46–54.
- Packham, G.H., Percival, I.G., Rickards, R.B. & Wright, A.J., 2001. Late Silurian and Early Devonian biostratigraphy in the Hill End Trough and the Limekilns area, New South Wales. Alcheringa 25, 251–261.
- Partridge, A.D., 1967. Geology of Boree Creek, west of Borenore, N.S.W. BSci (Hons), University of Sydney.
- Percival, I.G., 1978. Inarticulate brachiopods from the Late Ordovician of New South Wales, and their palaeoecological significance. Alcheringa 2, 117–141.
- Percival, I.G., 1979. Ordovician plectambonitacean brachiopods from New South Wales. Alcheringa 3, 91–116.
- Pickett, J.W., 1982. The Silurian System in New South Wales. Geological Survey of New South Wales Bulletin 29, 1–264.
- Pogson, D.J. & Watkins, J.J., 1998. Bathurst 1:250 000 Geological Sheet SI/55-8: explanatory Notes. Geological Survey of New South Wales, Sydney.
- Poschmann, M., Bergmann, A. & Kühl, G., 2017. First record of eurypterids (Chelicerata, Eurypterida) from the Lower Devonian (Lower Emsian) Hunsrück Slate (SW Germany). PalZ 91, 163–169.
- Richter, G., 1992. Fossilized gut contents: analysis and interpretation. In Messel - An Insight into the History of Life and of the Earth. Schaal, S. & Ziegler, W. eds, Oxford University Press, Oxford, 285–289.
- Rickards, R.B., 2000. The age of the earliest club mosses: the Silurian Baragwanathia flora in Victoria, Australia. Geological Magazine 137, 207–209.
- Rickards, R.B. & Garratt, M.J., 1990. Přídolí graptolites from the Humevale Formation at Ghin Ghin and Cheviot, Victoria, Australia. Proceedings of the Yorkshire Geological Society 48, 41–46.
- Rickards, R.B. & Wright, A.J., 1999. Systematics, biostratigraphy and evolution of the late Ludlow and Pridoli (Late Silurian) graptolites of the Yass district, New South Wales, Australia. Records of the Australian Museum 51, 187–214.
- Rolfe, W.D.I., 1973. Excursion 15: Silurian arthropod and fish assemblages from Lesmahagow, Lanarkshire. In Excursion Guide to the Geology of the Glasgow District. Bluck, B.J. ed., Geological Society of Glasgow, Glasgow, 119–126.
- Schmidt, M., Melzer, R.R., Plotnick, R.E. & Bicknell, R.D.C., 2022. Spines and baskets in apex predatory sea scorpions uncover unique feeding strategies using 3D-kinematics. iScience 25, 103662.
- Selden, P.A., 1981. Functional morphology of the prosoma of Baltoeurypterus tetragonophthalmus (Fischer) (Chelicerata: Eurypterida). Transactions of the Royal Society of Edinburgh: Earth Sciences 72, 9–48.
- Selden, P.A., 1984. Autecology of Silurian eurypterids. Special Papers in Palaeontology 32, 39–54.
- Sherwin, L., 1968. Denckmannites (Trilobita) from the Silurian of New South Wales. Palaeontology 11, 691–696.
- Sherwin, L., 1966. Some Geological Observations in the Cheesemans Creek District. BSci (Hons), University of Sydney.
- Sherwin, L., 1976. Stratigraphic boundaries of the Mirrabooka Formation and the Wallace Shale. Geological Survey of New South Wales, Palaeontological Report 76/23, GS1976/281 (unpublished).
- Sherwin, L., 1971a. Stratigraphy of the Cheesemans Creek district. Geological Survey of New South Wales Records 13, 199–237.
- Sherwin, L., 1971b. Trilobites of the subfamily Phacopinae from New South Wales. Records of the Geological Survey of New South Wales 13, 83–99.
- Sherwin, L. & Rickards, B., 2002. Late Silurian (Pridoli) graptolites from the Wallace Shale, New South Wales. Alcheringa 26, 87–101.
- Strusz, D.L., 1980. The Encrinuridae and related trilobite families, with a description of Silurian species from southeastern Australia. Palaeontographica Abteilung A 168, 1–68.
- Sues, H.-D., 1993. Gut contents of Parasaurus (Pareiasauria) and Protorosaurus (Archosauromorpha) from the Kupferschiefer (Upper Permian) of Hessen, Germany. Paläontologische Zeitschrift 67, 169–176.
- Toom, U., Vinn, O., Isakar, M., Madison, A. & Hints, O., 2020. Small faecal pellets in Ordovician shelly fossils from Estonia, Baltoscandia. Estonian Journal of Earth Sciences 69, 1–19.
- Tuckerson, M., 1966. Some Aspects Ofthe Geology Northwest of Cheesemans Creek Post Office. BSci (Hons), University of Sydney.
- Turner, S., 1993. Palaeozoic microvertebrates from eastern Gondwana. In Palaeozoic Vertebrate Biostratigraphy and Biogeography. Long, J.A. ed., Belhaven Press, London, 174–207.
- Vannier, J. & Chen, J., 2005. Early Cambrian food chain: new evidence from fossil aggregates in the Maotianshan Shale biota, SW China. Palaios 20, 3–26.
- Vinn, O., 2009. Attempted predation on Early Paleozoic cornulitids. Palaeogeography, Palaeoclimatology, Palaeoecology 273, 87–91.
- Vinn, O., 2017. Predation in the Ordovician and Silurian of Baltica. Historical Biology 29, 11–16.
- Vinn, O., 2018. Traces of predation in the Cambrian. Historical Biology 30, 1043–1049.
- Vinn, O., Holmer, L.E., Wilson, M.A., Isakar, M. & Toom, U., 2021. Possible drill holes and pseudoborings in obolid shells from the Cambrian/Ordovician boundary beds of Estonia and the uppermost Cambrian of NW Russia. Historical Biology 33, 3579–3584.
- Wood, K.G., 1955. Geology of the Amaroo District. BSci (Hons), University of Sydney.
- Zacaï, A., Vannier, J. & Lerosey-Aubril, R., 2016. Reconstructing the diet of a 505-million-year-old arthropod: Sidneyia inexpectans from the Burgess Shale fauna. Arthropod Structure & Development 45, 200–220.