ABSTRACT
Soil moisture and organic matter level affects soil respiration and microbial activities, which in turn impact greenhouse gas (GHG) emissions. This study was conducted to evaluate the effect of irrigation levels (75% [deficit], 100% [full], and 125% [excess] of reference crop evapotranspiration requirements), and organic amendments (OA) type (chicken manure [CM] and bone meal [BM]) and OA application rates (0,168, 336 and 672 kg total N ha−1) on (i) soil physical properties (bulk density, organic matter content and soil moisture content) and (ii) soil carbon dioxide (CO2) emissions from a highly weathered tropical Hawai'ian soil. Carbon dioxide readings were consistently taken once or twice a week for the duration of the cropping season. A drip irrigation system was used to apply the appropriate amount of irrigation water to the treatment plots. Treatments were randomly selected and corresponding organic amendments were manually incorporated into the soil. Plots were cultivated with sweet corn (Zea mays ‘SS-16’). Soil moisture content within and below the rootzone was monitored using a TDR 300 soil moisture sensor (Spectrum Technologies, Inc., Plainfield, IL, USA) connected with 12 cm long prongs. Soil bulk density and organic matter content were determined at the end of the cropping season. Analysis of variance results revealed that OA type, rate, and their interaction had significant effect on soil CO2 flux (P < 0.05). Among the OA rates, all CM mostly resulted in significantly higher soil CO2 fluxes compared to BM and control treatment (p < 0.05). The two highest rates of BM treatment were not significantly different from the control with regard to soil CO2 flux. In addition, organic amendments affected soil moisture dynamics during the crop growing season and organic matter content measured after the crop harvest. While additional studies are needed to further investigate the effect of irrigation levels on soil CO2 flux, it is recommended that in order to minimize soil CO2 emissions, BM soil amendments could be a potential option to reduce soil CO2 fluxes from agricultural fields similar to the one used in this study.
Introduction
Global warming, due to the increased atmospheric concentration of major greenhouse gases (GHGs) including carbon dioxide (CO2), methane (CH4), and nitrous oxide (N2O), is currently a major concern around the globe.Citation[1–4] Fossil-fuel combustion, deforestation, and agricultural practices are the main anthropogenic contributions to the increase of these GHGs. Among the major GHGs, CO2 has the highest atmospheric concentration followed by CH4, and N2O.Citation[3] Agricultural soils contribute about 25% of the total anthropogenic CO2 emissions.Citation[5,6] On a global scale, Smith et al. Citation[7] estimated that agriculture contributes 52–84% of world's combined anthropogenic methane and nitrous oxide emissions. Due to its large area and increasingly intensive management, agricultural lands have a significant negative impact on the Earth's carbon and nitrogen cycles. At the same time, through better management practices, such as organic and sustainable farming, agriculture has tremendous potential to reduce the atmospheric concentration of GHGs and sequester carbon in the soil.Citation[2,7,8] Cayuela et al.Citation[9] reported that conventional mineral fertilizers produce significantly higher N2O emissions than organic (animal waste derived) soil amendments.
Globally, crop-based agriculture comprises 1.7 billion ha with a soil C stock of about 170 Giga ton.Citation[10] The loss of soil organic matter (SOM) from cultivated soils significantly contributed to past anthropogenic CO2 emissions to the atmosphere. Citation[11] According to Rastogi et al.,Citation[12] there are three biological processes of respiration, which cause the release of carbon dioxide from the soil. These processes are microbial respiration, root respiration, and faunal respiration. Several studies have shown that soil texture, temperature, moisture content, pH, and available soil C and N content are factors that influence CO2 production and emissions from soil. Citation[12,13] While the use of organic amendments could assist with building a healthier soil and providing crops needed nutrients, they also contribute to soil CO2 emission.Citation[14–18] Cayuela et al.Citation[9] reported that chicken manure has the highest CO2 emissions compared with other amendments. Although reports show that organic amendments could potentially increase soil CO2 emissions, Muller Citation[19] argued that organic farming produces lower N2O emission due to its lower nitrogen input. Compared to temperate soils, CO2 emissions from tropical soils were not adequately investigated. Abbas and Fares Citation[18] simulated CO2 emissions from an organically amended (with chicken and dairy manures) Hawai'ian tropical soil. Abbas et al.Citation[20] observed statistically greater CO2 emissions from plots amended with chicken manure compared with plots amended with organic compost and control plots.
Nevertheless, improved management practices (e.g., organic farming) can help mitigate soil CO2 emissions by enhancing carbon sequestration into the soil in a relatively stable form.Citation[21,22] The Intergovernmental Panel on Climate Change (IPCC) Citation[23] has pointed out that increasing soil organic carbon in agricultural systems is an important climate change mitigation option. Organic agriculture is estimated to sequester 3.5–4.8 Giga ton of CO2, approximately 55–80% of the total global GHG emissions from agriculture; and reduce N2O by two-thirds.Citation[24] However, organic farming also requires effective management practices that ensure environmental sustainability (e.g., reduction in soil CO2 emissions).
On the other hand, while irrigation is important to achieve adequate crop yield, it stimulates soil CO2 emissions.Citation[25] When a dry soil is rewetted, microbial activities increase accompanied by the release of air trapped in the soil pores, which contributes to an increase in soil CO2 emission.Citation[12,26] Rewetting of dry soils due to irrigation or rainfall increases CO2 emission by increasing microbial activity, carbon mineralization and respiration.Citation[26,27] Soil moisture content affects soil respiration and hence soil CO2 emission.Citation[28] Ding et al.Citation[29] observed peak soil CO2 emission at 70% soil moisture level; while above 70% soil moisture level, CO2 emission declined sharply. Rochette et al.Citation[30] found that the rise in soil CO2 emissions with increasing soil moisture content was associated with an increase in microbial population and activity.
There is a need to better understand the combined effects of different levels of irrigation and organic amendment type and application rate on soil CO2 flux under tropical conditions. Therefore, the objective of this study was to assess the effect of organic amendment (OA) type (chicken manure and bone meal) and OA application rate (0, 168, 336 and 672 kg total N ha−1) and different irrigation levels (75% [deficit], 100% [full] and 125% [excess] of reference crop evapotranspiration) on (i) some soil physical properties (bulk density, organic matter content) and (ii) soil carbon dioxide (CO2) emissions from a highly weathered tropical Hawai'ian soil.
Material and methods
Study site
This study was conducted at the Waimanalo Research Station (21°20′15″ N, 157°43′30″ W) of the College of Tropical Agriculture and Human Resources (CTAHR), at the University of Hawai‘i at Mānoa. The long-term average precipitation at Waimanalo is 938 mm yr−1, most of which occurs between November and April.Citation[31] The mean annual soil temperature is 23°C. The soil at this location is classified as a Waialua gravelly clay variant (a very fine, mixed, super-active, isohyperthermic Pachic Haplustoll). This soil has 2– 6% slopes and is similar to Waialua clay variant except for the presence of common weathered gravel throughout its profile.Citation[32] Selected soil physical and chemical properties are presented in .
Table 1. Soil texture, saturated hydraulic conductivity (Ksat), available water content (θaw), Organic carbon (OC), bulk density (ρb) and cation exchange capacity (CEC) of Waialua gravelly clay variant (isohyperthermic Pachic Haplustoll) of Waimanalo research station.Citation[38]
Experimental design and site preparation
The effect of irrigation level, and organic amendment (OA) type and rate on CO2 emission was tested using a completely randomized block design with irrigation level as a block factors and organic amendment type and rate as treatments. The irrigation level was the main factor based on which three strips were adopted (75%, 100% and 125% of reference crop evapotranspiration [ETo]). A drip irrigation system was used to apply the appropriate amount of irrigation water to the treatment plots. Surface drip irrigation allows localized application of water reducing water losses as runoff, evaporation and deep percolation etc.
The combination of two organic amendment types (chicken manure and bone meal) and three rates (low, medium, and high) and a control (no amendment) were randomly replicated three times at each irrigation level. Each plot was 1.5 m wide and 2 m long; with a 0.5 m buffer between plots. Organic amendments were manually incorporated into the soil. Amendment application rates were calculated on a dry weight basis based on equivalent nitrogen (N) contents of 3.01 and 10% for chicken manure and bone meal, respectively (). Selected chemical properties of bone meal and chicken manure are given in . Target N application rates of 168, 336 and 672 kg N ha−1 representing low, medium and high rates, respectively, were used based on the recommendations by Abbas and Fares.Citation[18] Alleys and borders of 2.0 m were established between and around the perimeter of replications as buffers.
Table 2. Calculations of the recommended dry weight application rates of chicken manure and bone meal based on 3.01 and 10% N contents, respectively. Rates were calculated based on the recommendations by Abbas and Fares.Citation[18]
Table 3. Chemical properties of bone meal and chicken manure: adapted from Abbas and Fares Citation[18] and Valenzuela et al.Citation[39]
On April 5 and 6, 2011, plots were plowed to a depth of 10 cm, and organic amendment treatments were surface broadcasted and incorporated into the soil. Low, medium, and high amendment rates for chicken manure (CM) and bone meal (BM) are referred to as CM1/BM1, CM2/BM2 and CM3/BM3, which represent amendment rates of 168, 336 and 672 kg ha−1, respectively, while ‘ctrl’ represents non-amended control plots. Then, on April 15, 2011, sweet corn (Zea mays ‘SS-16’) was sowed using a manually operated seed planter. Seeds were sowed in rows with 20 cm between plants and 40 cm between rows. In each plot, PVC collars were permanently installed at a depth of 5 cm to allow for soil CO2 measurements. To ensure uniform soil mass sampling during the duration of the study, all PVC collars were identical and inserted to the same depth. There was no synthetic pesticide or herbicide applied during this field experiment, following the USDA organic growing techniques. Weed pressure was reduced and kept under control by constant hand weeding.
Soil CO2 sampling
Soil CO2 flux measurements were conducted using CIRAS-1 portable photosynthesis machine (PP Systems Inc., Amesbury, MA, USA) through its soil respiration chamber, which was attached to the machine using a 15 pin D connector. The soil chamber was placed on the PVC collar and secured together with a neoprene sleeve to prevent the loss of gas while taking measurements. Soil CO2 readings were taken once or twice a week for the duration of the crop growing season, mostly on Mondays and Fridays at approximately the same time of the day (between10 a.m. to 12:00 p.m.) to ensure consistent soil temperatures and accurate readings. Soil CO2 flux measurements continued for three weeks post-harvest.
Soil physical property measurements
After harvesting, undisturbed soil cores were extracted (using 5.7 cm diameter and 6 cm height cores) to determine bulk density. Soil bulk density was then calculated after oven drying soil cores for 24 h at 105°C following standard procedures. Bulk soil samples were collected from the top 0–10 cm depth for soil organic matter (OM) content analyses. Soil organic matter content was determined using the loss-on-ignition (LOI) method.Citation[33] Soil samples (5 g) from each plot were oven dried at 105°C for 24 h followed by cooling of the samples in a desiccator. Then, soil samples were crushed to < 2 mm diameter size using a mortar and pestle. The samples were then placed in porcelain dishes and combusted in a muffle furnace (Model 550 Isotemp Series, Fisher Scientific, Pittsburgh, PA, USA) at 550°C for 5 h. The samples were then transferred to an oven at 105°C for 2 h and were then allowed to cool in a desiccator. The final weights of the combusted soil were taken for each sample and recorded and soil organic matter content was calculated based on weight loss due to oxidation at the elevated temperature. In addition, soil moisture readings were monitored at each plot using a TDR 300 soil moisture sensor (Spectrum Technologies, Inc., Plainfield, IL, USA) with 12 cm long prongs.
Statistical analysis
Statistical analysis was performed using the Statistix 10 statistical software (Analytical Software, Tallahassee, FL, USA) to assess how irrigation level, organic amendment type, and rate affect soil CO2 flux, bulk density, organic matter content, and moisture level under a sweet corn crop. Data analysis was performed using a completely randomized block design with irrigation levels as block factor and organic amendment type and rate as treatments. Analysis of Variance (ANOVA) tests were performed to test the significance of the effects of the irrigation level and OA type and rate on mean soil CO2 flux, bulk density, organic matter content, and moisture level. When statistically significant differences between two or three parameters were observed, Tukey's HSD post-hoc multiple comparison tests were performed.
Results and discussion
Effect of organic amendments and irrigation on soil CO2 flux
Analysis of variance test results () for soil CO2 emission showed that soil CO2 flux was significantly affected by organic amendment type, rate, and their interaction (p < 0.05). Tukey's HSD post hoc test results showed that soil CO2 flux from chicken manure amended plots was significantly higher than that of bone meal treatments (). These results are consistent with the findings of Abbas and Fares,Citation[18] who observed higher soil CO2 emissions from plots amended with chicken manure compared to those amended with dairy manure. Similarly, Cayuela et al.Citation[9] found that chicken manure had the highest rate of CO2 emission when compared with other organic amendments. The reason for the higher CO2 emission rates from chicken manure could be due to the greater carbon to nitrogen (C:N) ratio of chicken manure (7:1) compared to that of bone meal (3.5:1). This was in agreement with the findings by Heller et al.Citation[13] who reported greater CO2 emission from chicken manure amended soils compared to that of soils amended with corn residue. Organic amendments with higher carbon to nitrogen ratio decompose faster than those with a lower carbon to nitrogen ratio because microorganisms in the former are more active and release more CO2 through respiration than those in organic amendments with a lower carbon-to-nitrogen ratio.
Table 4. Analysis of variance results of soil CO2 flux as a function of irrigation level (IRR), organic amendment type (OA) and rate.
Figure 1. Soil CO2 emission variations between organic amendment (chicken manure and bone meal) types based on the results of the Tukey's HSD post hoc statistical test. Organic amendemnts not sharing the same letter are significantly different at P < 0.05.
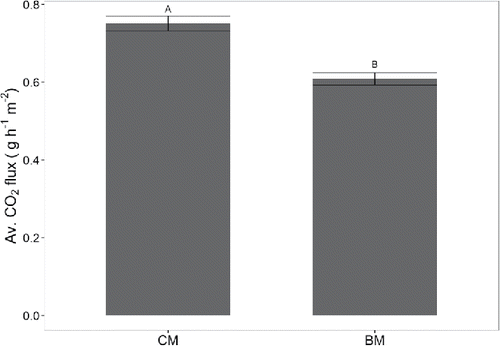
Within irrigation levels, deficit irrigation (75%) emitted a slightly greater CO2 than the full (100%) and excess irrigation (125%) treatments, however results were not statistically significant. Our results agree with those of Kowalenko et al.Citation[34] and Ding et al.Citation[29] who reported that soil 70% soil moisture level would be optimum for soil CO2 emission. They indicated that soil CO2 flux declined sharply when soil moisture content was greater than 70%. Saturated soil conditions could hamper diffusion of CO2 from the soil to the atmosphere; and lead to reduced availability of O2 in soils, which in turn suppresses activity of aerobic microbes. Saturated soil condition also increases the dissolution of soil CO2 in water.Citation[35]
In contrast, however, Raich Citation[25] suggested that increased irrigation would stimulate soil CO2 emissions. Separate effects of organic amendment rates on soil CO2 flux are shown in . While all organic matter amendment rates resulted in significantly higher soil CO2 fluxes compared with the control treatment, differences between the three application rates (low, medium and high) were not significant (). In addition, the effect of two-way interactions of organic amendment type and rate on soil CO2 flux was significant (). Overall, all rates of chicken manure amendments resulted mostly a significantly greater soil CO2 flux compared with bone meal and control treatments (). Our results concur with previous reports by Sainju et al.Citation[28] who indicated that irrigation would significantly increase soil CO2 emissions.
Figure 2. Comparison of soil CO2 emissions between OA rates based on the results of the Tukey HSD post hoc statistical test. Application rates not sharing the same letter are significantly different at P < 0.05.
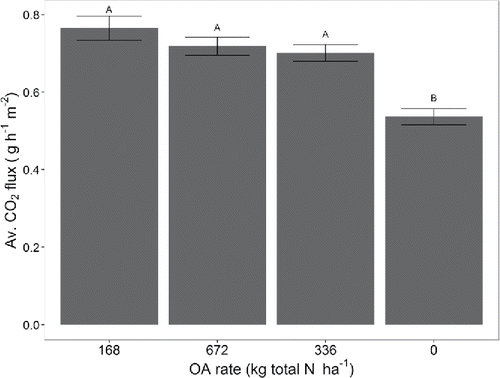
Figure 3. Interaction effects of organic amendment type and rate on soil CO2 emissions based on the results of the Tukey HSD post hoc statistical test. Application rates not sharing the same letter are significantly different at P < 0.05.
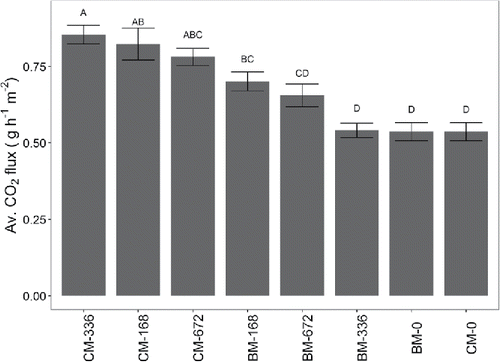
However, even though effects of the irrigation level and its interaction with organic amendemt type and rate were not statistically significant, plotting the cummulative soil CO2 flux indicated that CO2 flux was consistently greater from high amendment rates of both organic amendment types and especially at full and excess irrigation levels (). However, at deficit irrigation level, the CO2 flux tends to be slightly greater from plots amended with 168 kg of chicken manure (). It is worth indicating that CO2 flux from plots amended with chicken manure was consistently greater than that from the control treatment regardless of the OA application rate; however, on the other hand, CO2 flux from control plots was greater than CO2 flux from bone meal amended plots, especially at the low amendment rates ().
Figure 4. Cumulative soil CO2 flux between organic amendemnt rates (a) and between irrigation levels (b).
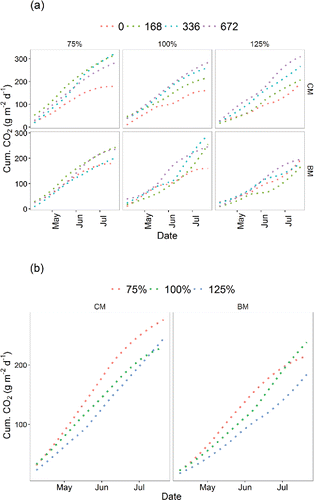
Similarly, the cumulative soil CO2 flux from deficit irrigation levels was mostly greater than those of full and excess irrigation level treatments regardless of amendment type (). However, the differences were only significant between deficit and excess irrigation levels. This could be due to the unusual higher rainfall received during the growing season, which seems to eliminate the deficit irrigation effect intended in this experiment (). This study was conducted during the summer, which is typically a dry time and ideal for irrigation studies. However, frequent occurrences of rainfall that wetted soils in all plots to similar saturation levels are likely to have diminished the effect of irrigation levels.
Effect of organic amendments and irrigation on soil properties
The addition of organic amendments and irrigation levels likely affect soil physical and chemical properties. Analysis of variance results presented in shows that soil moisture content was significantly affected by organic amendment rate (p < 0.05), while irrigation level and organic amendment type did not have significant effects. Furthermore, post hoc Tukey's HSD test results showed that soil moisture content tends to decrease with increases in organic amendment rates (). However, soil moisture content was only significantly different between low amendment rate and control treatments (). Our findings concur with previous finding of Fares et al.Citation[36] who found that soil moisture readings using capacitance sensor were negatively affected by organic matter content. Contrary to previous findings, e.g., Fares et al.,Citation[37] who observed a significant effect of organic amendments on bulk density, we found that bulk density was not affected by any of the treatments (data not shown). Moreover, unexpectedly, the soil moisture level and CO2 flux by organic amendment type were poorly correlated ().
Table 5. Analysis of variance results of soil moisture content as a function of irrigation level (IRR), organic amendment type (OA) and rate.
Figure 6. Effects of OA rates on average soil moisture content based on the results of the Tukey's HSD post hoc statistical test. Application rates not sharing the same letter are significantly different at P < 0.05.
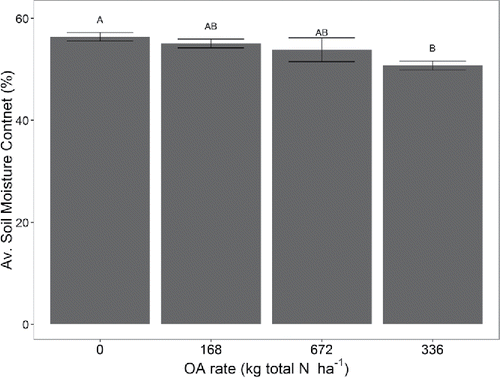
The soil organic matter content at the end of the growing season showed a significant response to the organic amendment application rate (). However, soil organic matter content did not show a consistent trend with increase application rates (). Nevertheless, as expected, control plots had lower organic matter content compared with amended plots (). Surprisingly, the medium application rate treatment was statistically at par with the control treatment (). This could be due to spatial variability of inherent soil organic matter content of the site, which could potentially counterbalance the effects of the organic amendment rate.
Table 6. Analysis of variance results of soil organic matter as a function of irrigation level (IRR), organic amendment type (OA) and rate.
Conclusion
The effect of irrigation level (75% [deficit], 100% [full], and 125% [excess] of reference crop evapotranspiration), organic amendment type (bone meal and chicken manure), and application rates (0, 168, 336, and 672 kg ha−1 equivalent total N) on soil CO2 flux and selected soil physical properties (bulk density, soil moisture content and organic matter content) was investigated to identify irrigation and soil management practices that reduce soil CO2 emission from crop fields.
Reducing atmospheric CO2 levels is imperative to combating climate change. With an increase in consumer consciousness regarding the benefits of consuming organic products, the demand for organic produce continues to increase. With more land area being utilized to grow organic crops, the soil CO2 data collected during this experiment, with regard to organic amendments that are routinely used in the Pacific, would be very important, and could be utilized to develop best management practices for minimizing soil CO2 emissions. Such information is not commonly available for the Pacific region and is only recently starting to be collected. Our findings show that soil CO2 flux was significantly affected by OA type, rate, and their interaction. Significantly highest soil CO2 fluxes compared to the control were observed from the largest application rate of chicken manure. However, the two highest rates of bone meal were not significantly different from the control with regard to soil CO2 flux. Soil moisture and organic matter content were only affected by organic amendment rate. While developing best management practices based on the results of this study, it should be noted that soils should be tested to determine the current nutrient status and plant nutrient requirements, prior to applying organic amendments. This will avoid excessive addition of nutrients, which could lead to nutrient imbalances and to an increase in soil CO2 emissions.
Though additional studies are needed to further investigate the effect of irrigation level on soil CO2 flux, it is recommended that in order to minimize soil CO2 emissions, bone meal soil amendments could be a potential option to reduce soil CO2 emissions.
Acknowledgment
A special mahalo goes out to Dr. Travis Idol, Dr. Helena Zaleski, Ted Evans and Gaoussou Diarra of the University of Hawaii-Manoa.
Funding
This work has been partially funded by Evans Allen NIFA-USDA and T-STAR USDA-NIFA.
References
- IPCC. Climate Change 2013: The Physical Science Basis. Contribution of Working Group I to the Fifth Assessment Report of the Intergovernmental Panel on Climate Change; Stocker, T.F., D. Qin, G.-K. Plattner, M. Tignor, S.K. Allen, J. Boschung, A. Nauels, Y. Xia, V. Bex and P.M. Midgley (eds.). Cambridge University Press: Cambridge, UK, 2013; 1535 pp.
- Watson, R.T.; Meira Filho, L.G.; Sanhueza, E.; Janetos, A. Greenhouse gases: sources and sinks. Clim. Change. 1992, 92, 25–46.
- Watson, R.T.; Rodhe, H.; Oeschger, H.; Siegenthaler, U. Greenhouse gases and aerosols. Clim. Change IPCC Sci. Assess. 1990, 1, 17.
- Emissions Scenarios: Summary for Policymakers: A Special Report of IPCC Working Group III; WMO (World Meteorological Organization): UNEP (United Nations Environment Programme): Geneva, 2000.
- Duxbury, J.M. The significance of agricultural sources of greenhouse gases. Fertil. Res. 1994, 38, 151–163.
- Duxbury, J.M. The significance of agricultural greenhouse gas emissions from soil of tropical agroecosystems. In Soil Management and Greenhouse Effect; Advances in Soil Science; CRC Press: Boca Raton, FL, 1995; 400.
- Smith, P.; Martino, D.; Cai, Z.; Gwary, D.; Janzen, H.; Kumar, P.; McCarl, B.; Ogle, S.; O'Mara, F.; Rice, C.; Scholes, B.; Sirotenko, O.; Howden, M.; McAllister, T.; Pan, G.; Romanenkov, V.; Schneider, U.; Towprayoon, S.; Wattenbach, M.; Smith, J. Greenhouse g:as mitigation in agriculture. Philos. Trans. R. Soc. B: Biol. Sci. 2008, 363, 789–813.
- Chapuis-Lardy, L.; Metay, A.; Martinet, M.; Rabenarivo, M.; Toucet, J.; Douzet, J.M.; Razafimbelo, T.; Rabeharisoa, L.; Rakotoarisoa, J. Nitrous oxide fluxes from Malagasy agricultural soils. Geoderma. 2009, 148, 421–427.
- Cayuela, M.L.; Velthof, G.L.; Mondini, C.; Sinicco, T.; van Groenigen, J.W. Nitrous oxide and carbon dioxide emissions during initial decomposition of animal by-products applied as fertilisers to soils. Geoderma. 2010, 157, 235–242.
- Paustian, K.; Six, J.; Elliott, E.T.; Hunt, H.W. Management options for reducing CO2 emissions from agricultural soils. Biogeochemistry. 2000, 48, 147–163.
- Robertson, G.P.; Paul, E.A.; Harwood, R.R. Greenhouse gases in intensive agriculture: contributions of individual gases to the radiative forcing of the atmosphere. Science. 2000, 289, 1922.
- Rastogi, M.; Singh, S.; Pathak, H. Emission of carbon dioxide from soil. Curr. Sci. 2002, 82, 510–517.
- Heller, H.; Bar-Tal, A.; Tamir, G.; Bloom, P.; Venterea, R.T.; Chen, D.; Zhang, Y.; Clapp, C.E.; Fine, P. Effects of manure and cultivation on carbon dioxide and nitrous oxide emissions from a corn field under Mediterranean conditions. J. Environ. Qual. 2010, 39, 437.
- Rao, D.L.N.; Pathak, H. Ameliorative influence of organic matter on biological activity of salt‐affected soils. Arid Soil Res. Rehabil. 1996, 10, 311–319.
- Moore, T.R.; Dalva, M. The influence of temperature and water table position on carbon dioxide and methane emissions from laboratory columns of peatland soils. J. Soil Sci. 1993, 44, 651–664.
- Rolston, D.E.; Hoffman, D.L.; Toy, D.W. Field measurement of denitrification: I. Flux of N2 and N2O. Soil Sci. Soc. Am. J. 1978, 42, 863–869.
- Egginton, G.M.; Smith, K.A. Nitrous oxide emission from a grassland soil fertilized with slurry and calcium nitrate. J. Soil Sci. 1986, 37, 59–67.
- Abbas, F.; Fares, A. Soil organic carbon and carbon dioxide emission from an organically amended Hawaiian tropical soil. soil sci. Soc. Am. J. 2009, 73, 995–1003.
- Muller, A. Benefits of Organic Agriculture as a Climate Change Adaptation and Mitigation Strategy in Developing Countries. Available at http://www.orgprints.org/14732 (accessed Sep 2016).
- Abbas, F.; Fares, A.; Valenzuela, H.R.; Fares, S. Carbon dioxide emissions from an organically amended tropical soil. J. Sustain. Agric. 2012, 36, 3–17.
- Lal, R. Agricultural activities and greenhouse gas emissions from soils of the tropics. In Soil Management and Greenhouse Effect: Advances in Soil Science; CRC Press: Boca Raton, FL, 1995; 293–307.
- Kotschi, J.; Müller-Sämann, K.M. The Role of Organic Agriculture in Mitigating Climate Change: A Scoping Study; IFOAM: Bonn, Germany, 2004.
- Climate Change The Physical Science Basis: contribution of Working Group I to the Fourth Assessment Report of the Intergovernmental Panel on Climate Change; Solomon, S.; Qin, D.; Manning, M.; Marquis, M.; Averyt, K.; Tignor, M.; Miller, Jr., H. L.; Chen, Z., Eds.; Cambridge University Press: Cambridge, New York, 2007.
- Niggli, U.; Flie/s sbach, A.; Hepperly, P.; Scialabba, N. Low greenhouse gas agriculture: mitigation and adaptation potential of sustainable farming systems. Ökol. Landbau. 2009, 141, 32–33.
- Raich, J.W.; Schlesinger, W.H. The global carbon dioxide flux in soil respiration and its relationship to vegetation and climate. Tellus. 1992, 44B, 81–99.
- Sparling, G.P.; Ross, D.J. Microbial contributions to the increased nitrogen mineralization after air-drying of soils. Plant Soil. 1988, 105, 163–167.
- Calderon, F.J.; Jackson, L.E. Rototillage, Disking, and Subsequent Irrigation. J. Environ. Qual. 2002, 31, 752–758.
- Sainju, U.M.; Stevens, W.B.; Caesar-TonThat, T.; Liebig, M.A. Soil greenhouse gas emissions affected by irrigation, tillage, crop rotation, and nitrogen fertilization. J. Environ. Qual. 2012, 41, 1774.
- Ding, W.; Meng, L.; Yin, Y.; Cai, Z.; Zheng, X. CO2 emission in an intensively cultivated loam as affected by long-term application of organic manure and nitrogen fertilizer. Soil Biol. Biochem. 2007, 39, 669–679.
- Rochette, P.; Desjardins, R.L.; Pattey, E. Spatial and temporal variability of soil respiration in agricultural fields. Can. J. Soil Sci. 1991, 71, 189–196.
- Ahmad, A.A.; Fares, A.; Abbas, F.; Deenik, J.L. Nutrient concentrations within and below root zones from applied chicken manure in selected Hawaiian soils. J. Environ. Sci. Health, Part B. 2009, 44, 828–843.
- US Department of Agriculture USDA. Soil Survey of the Island of Kauai, Oahu, Maui,Molokai, and Lanai, State of Hawaii; USDASCS: Washington, DC, 1972.
- Howard, P.J.A.; Howard, D.M. Use of organic carbon and loss-on-ignition to estimate soil organic matter in different soil types and horizons. Biol. Fertil. Soils. 1990, 9, 306–310.
- Kowalenko, C.G.; Ivarson, K.C.; Cameron, D.R. Effect of moisture content, temperature and nitrogen fertilization on carbon dioxide evolution from field soils. Soil Biol. Biochem. 1978, 10, 417–423.
- Bowden, R.D.; Davidson, E.; Savage, K.; Arabia, C.; Steudler, P. Chronic nitrogen additions reduce total soil respiration and microbial respiration in temperate forest soils at the Harvard Forest. For. Ecol. Manag. 2004, 196, 43–56.
- Fares, A.; Awal, R.; Bayabil, H. Soil water content sensor response to organic matter content under laboratory conditions. Sensors. 2016, 16, 1239.
- Fares, A.; Abbas, F.; Ahmad, A.; Deenik, J.L.; Safeeq, M. Response of selected soil physical and hydrologic properties to manure amendment rates, levels, and types. Soil Sci. 2008, 173, 522–533.
- USDA. Soil Survey of Islands of Kauai, Oahu, Maui, Molokai, and Lanai, State of Hawaii; USDA-SCS: Washington, DC, 1972, 1972.
- Valenzuela, H.R.; Goo, T.; Hamasaki, R.H.; Radovich, T. The effect of bone meal on the Yield of Jicama, Pachyrhizus erosus, in Oahu Hawaii. Proc Fla State Hort Soc. 2000, 113, 222–226.