Abstract
The quality of hops is dictated in large part by the content of flavor and aroma compounds. These compounds vary in concentration and are affected by agricultural practices. Previous research has found that the application of Bordeaux Mixture may impact the aroma quality of hops by altering concentrations of varietal thiols. The impact of copper fungicides other than Bordeaux Mixture and in hop production areas outside of the Pacific Northwest in the United States has yet to be evaluated. Herein we study the effects of application frequency of a copper (II) hydroxide fungicide on markers of hop quality in hops grown in the Northeastern United States. Copper fungicide application was found to significantly increase the total copper content of hop cones, regardless of application frequency; however, traditional markers of hop quality, including yield, essential oil composition, and acid content, were not affected. Beer consumers were unable to discriminate between beers dry-hopped with conventional or copper-treated hops based on aroma but were able to discriminate based on in-mouth perception. These findings suggest that the use of copper (II) hydroxide for the mitigation of fungal pathogens in hops grown in the Northeastern United States is unlikely to adversely affect final hop yield, acid content, or thiol quality.
Introduction
Over 50,000 tons of hops, valued at $618 million, were produced in the United States in 2019.[Citation1] Hops, most commonly used in the production of beer, contain a myriad of aroma-active compounds, including terpenoids, esters, alkanes, and thiols, as well as crucial taste components, including the class of organic acids known as humulones and lupulones, which impart the characteristic bitterness of a beer. While it is difficult to define quality in hops or any agricultural product, it is often characterized by yield, disease resistance, and macro- and micronutrient content. In horticultural products such as fruits, herbs, and flowers, the aroma and flavor must be elevated to a primary attribute of quality as they are often drivers of consumer acceptability and preference.[Citation2–4] Markers of quality are influenced and improved through agricultural practices. Herein, we investigate the use of a copper-based fungicide on the aroma and flavor quality of hops and hop products in addition to more traditional markers of hop quality.
The control of plant diseases is one critical facet of pesticide functionality. Downy mildew, fire blight, brown rot, and powdery mildew are just a few of the numerous diseases that can have deleterious effects on crops.[Citation5,Citation6] Organic fungicides rely on several naturally occurring, active ingredients for disease control as they cannot be synthetically derived. These active ingredients include, but are not limited to, oils, bicarbonates, elemental sulfur, and elemental copper. Copper-based fungicides (CBFs) contain copper hydroxide, copper sulfate, copper oxychloride, and/or other copper-based compounds as their active ingredients. Copper (II) hydroxide, in the form of the Bordeaux Mixture (copper sulfate and calcium hydroxide, a.k.a. slaked lime), was one of the earliest fungicides used in agriculture.[Citation7] Modern-day preparations of cupric hydroxide are the second most utilized organic fungicides in the United States.[Citation8] CBFs are broad spectrum fungicides and are commonly used alongside single-site mode-of-action fungicides in hop production to prevent diseases, especially downy mildew.[Citation9] The need for fungicides is much greater in areas with increased disease pressure due to greater rainfall and humidity such as the Eastern United States or coastal regions of the Western United States.[Citation10–13] While copper functions effectively as a fungicide, it is a double-edged sword as copper ions can function as pro-oxidants, catalyzing the production of reactive oxygen species (ROS) in the hop plant. The generation of ROS may lead to the loss of quality in the host plant through the direct oxidation of important flavor compounds or through the induction of an oxidative stress response, which alters the normal metabolic functions of the plant.[Citation14–18]
A body of evidence exists linking copper to the degradation of aroma and flavor compounds in several food systems. Previous studies have demonstrated the binding of thiols by copper in the environment as well as foods and beverages, most notably wine.[Citation15,Citation19] Although often found in low concentrations in plants and food products, thiols are potent aroma compounds with odor detection thresholds at ng/L levels.[Citation20] Surface applications and increased soil concentrations of copper have been shown to decrease concentrations of beneficial varietal thiols in hops, wine, and beer.[Citation16,Citation21,Citation22] Increased soil concentrations of copper and exposure to copper during the brewing process have been correlated with reductions in thiol concentrations in hops and wort, respectively.[Citation17,Citation20] When varietal thiols are complexed and oxidized to disulfides or polysulfanes by copper, they no longer contribute their characteristic aromas due to the decreased volatility of these higher molecular weight species.[Citation16] While the question of whether this phenomenon occurs in hops has been addressed to a minor degree by the global hops industry, it has yet to be investigated in non-traditional and re-emerging hop production regions such as the Northeastern United States. Approximately 97% of all hops grown in the United States were produced in the Pacific Northwest.[Citation23] Over the past decade, hop production has been expanding outside of the Pacific Northwest into Northeastern States such as Michigan, Vermont, and Pennsylvania.[Citation1] Production areas in the Northeastern United States experience relatively higher levels of rainfall and humidity during the hop growing season, and a greater need for fungicides such as CBFs exists. Furthermore, with the current popularity of heavily hopped (“hop-forward”) styles of beers (e.g., India Pale Ales and New England Pale Ales), hop aroma stability is arguably a more important marker of hop quality to many brewers than the more traditional quality marker of hop bittering capacity. Further research is necessary to evaluate the effects of foliar applications of CBFs on hop quality in emerging hop production areas such as the Northeastern United States.
The objective of the current study was to evaluate the effects of a copper (II) hydroxide-based fungicide applied to hops at different frequencies throughout the growing season in the Northeastern United States on various markers of hop quality. Markers of quality evaluated included yield, final elemental composition, bittering acid content, essential oil composition, thiol content, and consumer perception of aroma and taste.
Experimental
Materials
A hop acid standard extract was purchased from the American Society of Brewing Chemists (St. Paul, MN, U.S.A.). The internal standard for the essential oil analysis, 2-octanol, was purchased from Thermo Fisher Scientific (Waltham, MA, U.S.A.). The compounds 3-mercaptohexanol, 3-mercaptohexyl acetate, 4-mercapto-4-methylpentan-2-one, and 4-methoxy-2-methyl-2-butanethiol were purchased from Sigma-Aldrich (St. Louis, MO, U.S.A.). Liquid malt extract and hop extracts used for the preparation of beer for sensory analysis were purchased from Northern Brewer (Roseville, MN, U.S.A.). Nitrogen (99.995%) and carbon dioxide (99.5%) were purchased from Praxair (Danbury, CT, U.S.A.) and supplied through Penn State General Stores (University Park, PA, U.S.A.). All other solvents and acids used were of reagent grade or better and were purchased from VWR International (Radnor, PA, U.S.A). All hops were grown at Penn State’s Russell E. Larson Research Farm (Pennsylvania Furnace, PA, U.S.A.) in the 2018 and 2019 seasons. The pesticide, Champ WG (copper (II) hydroxide), was procured from Nufarm Americas Inc. (Alsip, IL, U.S.A.).
Hop field trials
Hops were grown in the research hopyard at Penn State’s Russell E. Larson Research and Education Center at Rock Springs (40°42’48.546” N, 77°56’28.648” W) during the 2018 and 2019 seasons. The hop variety cv. Cascade was used exclusively for these trials. Hop plants were purchased from Great Lakes Hops (Zeeland, MI, U.S.A.) and planted in 2016. Two experimental blocks, each containing ten replicates of seven plants each, were randomly assigned to treatment groups. Hops were trained onto coconut husk rope that was suspended from a 20-foot trellis based on plans provided from Schmidt Hops (Farmington, NY, U.S.A.). For the 2018 season, treatments consisted of a copper group and a control group, with ten replicates (five per block) assigned to each treatment. Copper-treated plants were sprayed with a copper (II) hydroxide formulation every ten days from the first week of June to the last week of July using a tow sprayer while the control received no copper treatment. For the 2019 season, hop treatments consisted of no (control), low frequency (low copper), or high frequency (high copper) application of the copper (II) hydroxide fungicide “Champ WG” (Nufarm Americas, Inc., Alsip, IL, U.S.A.). High copper treated plants were sprayed with a foliar application of copper (II) hydroxide formulation every 14 days using a tow sprayer while low copper plants were sprayed every 28 days. For the 2019 application treatments, six replicates were included in each treatment with three replicates per block. The two end plants in every experimental unit were left as border plants to reduce treatment carryover from the adjoining replicate and were not included in any of the analyses for both seasons. Both the control and CBF-treated plants were sprayed regularly with conventional copper-free fungicides (Ranman 400SC, Summit Agro, Durham, NC, U.S.A., and Zampro, BASF Corporation, Florham Park, NJ, U.S.A.) according to label instructions using an air blast sprayer to ensure crop viability. The recommended labeled application rates for the fungicide’s use on hops were followed, and the final application in both years was completed one month before harvest, which occurred in mid to late August (August 24, 2018 and August 13, 2019).
Hop processing
Hops were harvested mechanically using a Hopster® Harvester (HopsHarvester LLC, Honeoye Falls, NY, U.S.A.) and transferred to 15” x 25” polypropylene mesh bags. Hops from the 2018 season were dried in a hot-air oast at 60 °C to a final moisture content of approximately 10% as determined by an F-2000/H moisture meter equipped with a short pin-prod electrode (Delmhorst Instrument Co, Towaco, NJ, U.S.A.). The oast was built based on plans published by the University of Vermont Extension[Citation24] with minor modifications. Hops were allowed to cool to room temperature and were then placed in sealed plastic bags and frozen at −80 °C for further analysis. Hops from the 2019 season were harvested in the same fashion but were immediately frozen in vacuum-sealed bags after harvest rather than dried in the oast in an attempt to retain more aroma compounds. Hop samples were then freeze-dried using a Labconco FreeZone 2.5 lyophilizer (Kansas City, MO, U.S.A.), taking approximately 48 h, and then stored at −80 °C prior to analysis. For both harvest years, hop cones from all five plants in each replicate were pooled for analysis. No statistical comparisons were made between harvest years due to differences in post-harvest processing.
Hop essential oil analysis
Essential oil fractions were prepared from each replicate for all treatments from both harvest years using official methods with minor modifications.[Citation25] In short, 50 g of oast-dried or freeze-dried hops were placed in a 2-L round bottom flask with 500 mL of distilled water. The flask was secured in a heating mantle and a graduated distillation receiver coupled to an Allihn condenser (VWR International, Radnor, PA, U.S.A.) to prevent the loss of volatile oils. Cold water from an ice-cooled reservoir was pumped through the condenser. The contents of the flask were brought to a boil, and the temperature was adjusted such that approximately 30 drops of distillate condensed into the receiver every minute. The essential oils evolved with the steam were condensed and collected in the calibrated, graduated receiving flask. The flask was allowed to reflux for 3 h for complete extraction of essential oils. The oil was carefully collected with a borosilicate Pasteur pipette to avoid the collection of water, blanketed with nitrogen gas in a sample tube, and stored at −80 °C before analysis.
Select terpenoids were quantified by gas chromatography-mass spectrometry (GC-MS) according to the standard method with some modifications.[Citation26] A stock solution of 1% (v/v) 2-octanol solution was prepared in hexane. Distilled essential oils (100 µL) were diluted in the internal standard stock (900 µL) prior to injection. Essential oil components were separated on a Supelcowax® 10 capillary GC column (L × I.D. 30 m × 0.25 mm, df 0.25 µm) using a 7890 A GC system (Agilent Technologies, Santa Clara, CA, U.S.A.) with an Agilent 5975C mass spectrometer. The inlet was held at 200 °C; the liquid injection volume was 1 µL using helium as the carrier gas with a split ratio of 1:50. The column transfer line to the mass spectrometer was held at 280 °C. The flow rate was held at 0.6 mL/min, and the oven temperature program was as follows: 0–1 min, 50 °C followed by a ramp to 260 °C at a rate of 3 °C/min and then holding at 260 °C for 15 min. The most abundant terpenoids, including myrcene, humulene, β-farnesene, caryophyllene, and geranyl acetate, were identified using the National Institute of Standards and Technology fragmentation library and the relative retention times reported in the published literature.[Citation26] The relative quantity of each terpenoid was expressed in 2-octanol equivalents on a dry weight basis.
Hop acid analysis
The α- and β-acids were extracted using the standard methanol and diethyl ether extraction method and subsequently quantified by high-performance liquid chromatography (HPLC) coupled to a diode array detector (DAD).[Citation27] In short, a 10 g sample of dried hops was ground using a universal No. 2 food chopper and placed in a 250-mL Erlenmeyer flask. The initial mass of each sample was recorded. For the extraction, 100 mL of diethyl ether was added to each flask, followed by 20 mL of methanol. The flasks were capped with rubber stoppers and placed on a rotary shaker at 200 rpm for 30 min. After 30 min, stoppers were removed, and 40 mL of 1 M hydrochloric acid was added to each flask, swirled to mix, stoppered, and then returned to the shaker at the same speed for an additional 10 min. Flasks were then removed, and the two phases were allowed to separate on the benchtop for 5 min. Approximately 40 mL of the ether (top) phase was decanted into a 50-mL centrifuge tube and then spun down for 5 min at 3,220 x g. A 1 mL aliquot of the extract was transferred to a 15-mL centrifuge tube with a positive displacement pipette. The sample was diluted with 9 mL of methanol and mixed thoroughly. Samples were stored for up to 24 h at −80 °C prior to analysis per the recommendation of the established method.[Citation27] Sample acid concentrations were quantified using an external standard of known humulone and lupulone content. Approximately 500 mg of standard extract was added to a 100-mL volumetric flask and dissolved and brought to the mark with methanol to create the external standard solution. The standard solution was then diluted by a factor of 10 with methanol (1 mL of stock to 9 mL methanol) to match the dilution of the samples. Acids were separated using a Shimadzu HPLC system equipped with a photodiode array detector (DAD) set to 314 nm using an Eclipse Plus C18, 2.1 × 150 mm, 5 µm column (Agilent Technologies, Inc., Santa Clara, CA, U.S.A.). The elution was isocratic using a single solvent consisting of 82% (v/v) aqueous methanol containing 1% (v/v) phosphoric acid at a flow rate of 0.9 mL/min. The injection volume of dilute samples and standards was 3 µL, and all separations were run at 25 °C. All diluted samples were injected in triplicate, and the standard was injected five times.
Semi-quantitative analysis of hop thiols
Thiols were quantified using methods adapted from Capone et al.[Citation28] Hops were ground in a food processor, and 10 g were extracted in 300 mL 0.1 M glycine buffer (pH 2.2) containing 100 µM 4,4′-dithiodipyridine and 100 ng of 4-MMB for 12 h at room temperature with stirring. Solid-phase extraction columns containing strong cation exchange resin (Strata™-X-C 33 µm Polymeric Strong Cation, 500 mg/6 mL, Phenomenex, Torrance, CA, U.S.A.) were conditioned with 1 volume (6 mL) of methanol, followed by 1 volume of phosphate buffer (pH 2.2). Two volumes of buffered hop extract (12 mL) were loaded onto the column and subsequently washed with 1 volume of 0.1 M hydrochloric acid and 1 volume of methanol containing 0.1 M hydrochloric acid. The column was dried under vacuum (10 mmHg) for 20 min before elution with 4 mL of methanol containing 5% of a 28% ammonium hydroxide solution. The eluted samples were passed through polytetrafluoroethylene (PTFE) (13 mm; 0.45 µm) syringe filters (CELLTREAT Scientific Products, Pepperell, MA, U.S.A.). Filtered samples were then dried in a CentriVap Benchtop Vacuum Concentrator (Labconco Corporation, Kansas City, MO, U.S.A.) at ambient temperature and resuspended in 120 μL of initial mobile phase (12:88, acetonitrile:water containing 0.5% formic acid). Thiols were separated using reverse-phase, HPLC, and quantified using a mass spectrometer (MS). The aqueous solvent (A) consisted of 0.5% (v/v) formic acid in LC-MS grade water. The organic phase (B) was LC-MS grade acetonitrile containing 0.5% (v/v) formic acid. The gradient elution program was as follows: 0 min, 12% solvent B; 13 min, 50% solvent B; 15 min, 80% solvent B; 17.5 min, 80% solvent B; 18 min, 12% solvent B; 30 min, 12% solvent B. The following parameters were optimized for MS detection in method development: positive electrospray ionization; desolvation flow rate, 450 L/h; cone gas flow rate, 150 L/h; capillary voltage, 4.5 kV; cone voltage, 20 V; extractor voltage 2 V; source temperature, 120 °C; desolvation temperature, 350 °C; collision voltage, 35 eV with argon as the collision gas. The derivatized thiols were detected using multiple reaction monitoring (MRM). MRM pairs included the mass to charge (m/z) of the parent ion of the derivatized thiol and the daughter ion of the protonated thiopyridine ring (). The use of MRM allowed for the removal of interference from potential co-eluting peaks. All chromatograms were analyzed using PeakView 2.2 (AB Sciex, Framingham, MA, U.S.A.). Thiol content was measured relative to the signal of the internal standard (4-MMB).
Table 1. Multiple reaction monitoring parent-daughter ion pairs.
Production of dry-hopped beer for sensory analysis
Hops from the 2018 season were used to dry-hop beers, which were later subjected to sensory analysis. The base of the pilot-scale beer produced for dry hopping was modeled after an American Pale Ale (original gravity (OG): 1.044–1.050, international bitterness units (IBU): 30–50). It was determined that ∼4 L of finished beer was necessary to conduct the designed sensory test. Given the volume limitations of the pilot-scale equipment available, the following wort preparation procedure was repeated twice, and the resultant worts were pooled to eliminate the variation between batches.
Municipal water (19 L) was brought to a boil in a LEE Industries CWD, 57 L jacketed steam kettle (Philipsburg, PA, U.S.A.) to prepare the wort. Once the water was boiling, 3.4 kg. of liquid, golden malt extract (LME) (Northern Brewer, Roseville, MN, U.S.A.) was added. Cascade Hop-Shot extract (6 ml) (Northern Brewer, Roseville, MN, U.S.A.), a supercritical CO2 extract of Cascade hops, was added to the boil to achieve the desired IBU value. Addition rates for LME and hop extract were based on supplier-provided instructions to achieve the target values for OG and IBU, given the volume of the production. The wort was boiled for 1 h and then cooled to 25 °C in the kettle by running cold water through the jacket. Both batches of wort were pooled in a 38 L stainless steel vessel, and samples were taken to determine initial specific gravity and pH. Specific gravity was measured using a Densito 30 PX digital density meter (Mettler Toledo, Columbus, OH, U.S.A.) and was found to be 1.057 ± 0.001. The initial pH, as measured by a FiveGo handheld pH meter (Mettler Toledo, Columbus, OH, U.S.A.), was 5.55 ± 0.01 pH units. American ale type yeast (Wyeast 1056, Wyeast Laboratories Inc., Odell, Or, U.S.A.) was added to the cooled wort at a rate of 5.7 × 104 viable cells/mL, based on manufacturer-reported cell count and estimates of cell viability. The inoculated wort was divided into eight fermentation replicates consisting of 4 L of wort in a 5 L glass carboy (Little Big Mouth Bubbler, Northern Brewer, Roseville, MN, U.S.A.). The beer was allowed to ferment at room temperature (∼18 °C) for 14 days in the absence of light to complete primary fermentation. At the end of primary fermentation, samples were taken to determine final gravity and pH, ensuring that no significant variations in fermentation occurred. The average specific gravity across all replicates was found to be 1.017 ± 0.0006, and the average pH was 4.64 ± 0.016, indicating consistent fermentations across replicates.
Each carboy was randomly assigned to be dry-hopped with control or copper-treated hops with four replicates for each treatment. Hop mixtures for the control and copper-treated hops were prepared by combining 10 g of dried, whole-hop cones from each of the ten field replicates within an experimental group. The hop cones were blended in a food processor for 30 s to increase the homogeneity of the mixtures. Ground hops were placed in nylon mesh bags and added to the carboys at a concentration of 2 g/L or 8 g per carboy. The beers were blanketed in nitrogen gas to prevent oxidation, and the hops were left in contact with the beers for 12 days. At the end of the dry-hopping period, the hops were removed from the beers, and benchtop sensory was conducted on each replicate to make sure that no anomalous variations in color, aroma, or taste existed between replicates within a given treatment. Finished beers were then pooled according to treatment into 38 L stainless steel brite tanks (CM Brewing Technologies, Inc., Tustin, CA, USA). The beers were refrigerated (4 °C) and carbonated to 2.5 volumes of CO2 over 7 days.
Consumer sensory analysis of dry-hopped beers
Sensory analysis was conducted at the Penn State Sensory Evaluation Center, located in the Rodney A. Erickson Food Science Building (University Park, PA, U.S.A.). Procedures were deemed exempt by Penn State’s Institutional Review Board under category 6: Taste and Food Quality Evaluation (protocol no. 33164). Beer consumers (21–61 years of age; n = 92, 56 females, 36 males) were presented with two sets of three beer samples of equal volume in 30 ml covered plastic sample cups, labeled with 3-digit blinding codes. Presentation order of the samples was counterbalanced across participants. For the first set of samples, participants were instructed to smell all three beer samples and then identify the odd sample. For the second set of samples, participants were instructed to taste and expectorate the sample and then pick the odd sample based on the perceived flavor. Panelists were compensated for their time with a $5 cash payment and gave informed consent via a yes/no question on a computer screen at the beginning of the test. All testing was conducted in individual tasting booths at room temperature under red lighting, such that the appearance of the beers does not influence the evaluation. Beers were served directly from the brite tanks, which were kept refrigerated and carbonated using a CO2 cannister to ensure there were no differences in beer temperature or carbonation for a given sample set.
Statistical analyses
Statistical analyses were performed using GraphPad Prism 6 (GraphPad Software Inc., San Diego, CA, U.S.A.). The Student’s t test was used to determine differences between treatments in the analyses performed on the hops harvested in 2018. The χ2 test was used to determine if significant differences were detected in triangle test evaluations using the analysis tools included with the survey software, Compusense Cloud (Academic Consortium, Guelph, ON, Canada). Differences among treatments in the 2019 season were determined using a one-way analysis of variance (ANOVA) with Tukey’s honestly significant differences (HSD) post hoc test. The Brown–Forsythe test was used to ensure equal sample variances (α = 0.05). P values less than 0.05 were considered statistically significant.
Results and discussion
Hop yield
Hop cones were pooled according to field replicate during harvest, and each replicate, consisting of the cones of the five bines, was weighed before drying. No significant differences in yield were found between the treatments indicating that the use of CBFs had no apparent impact on the mass of hop cones produced by a single plant (). These findings are consistent with data previously published regarding the use of the copper hydroxide fungicide on Cascade hops in the Northeastern United States, which compared the yield and quality of hops treated with several different organic fungicides and found no significant effect on hop yield.[Citation29] Our findings corroborated the previously published data and also found that yield was not negatively impacted when CBF was applied in combination with a conventional, synthetic fungicide program for disease management.
Figure 1. Hop yield. The yield of wet hops in grams from each bine for the 2018 (Panel A) and 2019 (Panel B) harvests. Error bars represent the standard deviation of the samples for 2018 (n = 10 per treatment) and 2019 (n = 6 per treatment). No statistically significant differences were found between treatments within harvest years (α = 0.05).
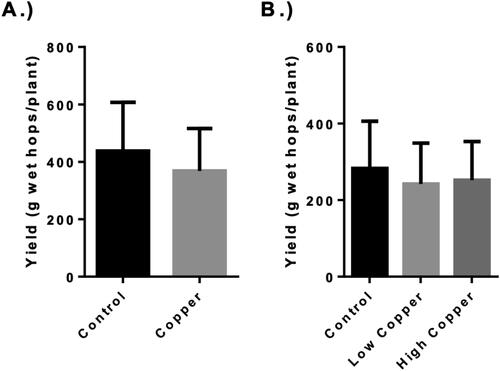
Hop elemental and metal content
Various metals and other elements, including phosphorus, potassium, calcium, magnesium, sulfur, manganese, iron, copper, boron, zinc, aluminum, and sodium were quantified in the hop cones (). As was expected, of all of the elements analyzed, only the copper content varied among treatments. In the 2018 season, content hop cones contained an average of 7.22 ± 0.48 mg/kg total copper, while CBF-treated cones contained an average of 18.71 ± 1.70 mg/kg on a dry weight basis. These copper levels represent a significant increase (p < 0.0001) in post-harvest copper content, amounting to a 159% average increase over a single season.
Figure 2. Hop elemental analysis. The elemental analysis of dried hop cones from the 2018 (Panel A and B) and 2019 (Panel C and D) seasons. Copper was significantly elevated in copper-based fungicides (CBF)-treated hops regardless of season and treatment frequency. Error bars represent the standard deviation of the samples for 2018 (n = 10 per treatment) and 2019 (n = 6 per treatment). Difference in single elemental content between treatments was evaluated by the Student’s two-sample t test for 2018 data (* indicates a significant difference, p < 0.05) and using a one-way analysis of variance (ANOVA) with Tukey’s HSD post hoc test for the 2019 data (significant differences are indicated by dissimilar letters above the bar, p < 0.05).
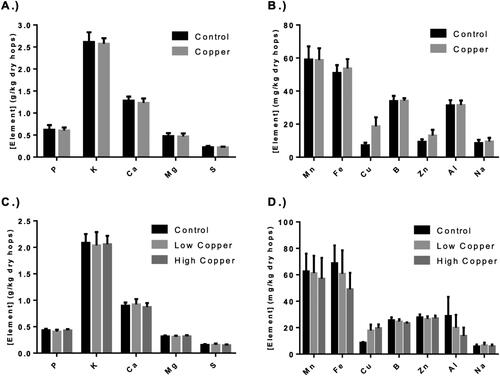
Similar results were observed during the 2019 harvest. A single sample (Control replicate 2) was excluded from the analysis due to excessive variation from all other replicates. The significance of its outlying properties was confirmed using Grubbs’ test for outliers (α = 0.05). Both the low and high copper treatments had significantly elevated levels of total copper compared with the control hops (p < 0.0001). The low copper treatment was, on average, 114% greater than the control (17.83 ± 4.36 mg/kg vs. 8.33 ± 0.82 mg/kg, respectively) while the high copper treatment was 136% greater than the control (19.67 ± 2.81 mg/kg vs. 8.33 ± 0.81 mg/kg, respectively). While the increase in the total copper content of the high copper treatment was greater in magnitude compared with the low copper treatment, the difference was not significant. The low copper application rate was half of the maximum frequency recommended by the supplier (28-day rotation), while the high copper application rate was at the maximum frequency recommended by the supplier (14-day rotation). The fact that the total copper did not vary significantly between these treatments indicates that either the frequency of application had no effect on the total increase in the post-harvest copper content of the hops or that the total copper content is more dependent upon the timing of application relative to harvest. Rainfall, which has the potential to physically remove fungicides residue, may also have affected total copper concentrations, but weather events were not monitored in the current study. The final applications of both the low and high copper treatments occurred on the same day; as a result, it is possible that the observed copper contents may be due to residual fungicide on the cones rather than endogenous copper absorbed throughout the season.
The latter hypothesis is supported by previous research on the persistence of CBFs on hops. Krofta et al. applied copper oxychloride fungicides to hop plants and monitored the copper content of the hop cones and leaves for an extended period following the applications.[Citation30] It was found, generally, that the highest concentrations of elemental copper in hop cones were in those cones sampled closest to the date of the fungicide application, and the amount of copper decreased gradually over time. This trend was much more apparent in the copper content of the leaves as the increased foliar surface area resulted in greater fungicide deposition. The authors reported no clear correlation between the amount of copper applied and the final copper content of the hop cones. The increased copper content of cones at the point of harvest may pose a risk to the quality of products produced with these hops. Copper is known to act as an oxidation catalyst, generating oxygen radicals through Fenton-like reactions (i.e., peroxide reduction to hydroxyl or alkoxyl radical species) and activating ground state oxygen.[Citation31] This increase in pro-oxidative potential may negatively impact the stability of hopped products, mainly beers, produced with the CBF-treated hops as many reactions resulting in quality loss are oxidation dependent.[Citation32] The potential for excess copper found in treated hops to be transferred into products utilizing those hops must be investigated.
Across both seasons, the data indicate that the use of CBFs significantly increases the total elemental copper content of treated hop cones. This appears to be independent of application frequency and is likely dependent on the date of application relative to harvest. Similar findings of significantly increased copper concentrations have been reported in the application of copper sulfate[Citation17] and copper oxychloride[Citation30] in hops. The distribution of other elements measured in the present study does not appear to be impacted by the use of this copper hydroxide fungicide.
Hop acid quantification
Hop acids consist of two major groups: humulones, also known as α-acids, and lupulones, also known as β-acids. The compounds belonging to each group are bitter and are, in their isomerized forms, responsible for contributing the characteristic bitterness of a beer. The major α- and β-acids (humulone, adhumulone, cohumulone, lupulones, adlupulone, and colupulone) were extracted from hops and quantified by HPLC-DAD. The data indicate that CBF treatment, regardless of frequency, had no impact on the acid concentrations of the hops in either year (, p > 0.05 for all comparisons). The values reported here are comparable to the expected values for α- and β-acids in Cascade hops reported elsewhere.[Citation17,Citation33] Variations in the acid content across years are likely due to the fact that the hops in the 2019 season were completely dry for the analysis while the 2018 hops contained around 10% moisture.
Figure 3. The α-acid and β-acid content of copper-based fungicides (CBF)-treated hops. Pooled and individual acid analysis of hops from 2018 (Panel A and B) and 2019 (Panel C and D) expressed as weight percent of hops analyzed. Error bars represent the standard deviation of the samples for 2018 (n = 10 per treatment) and 2019 (n = 6 per treatment). Difference in acid content between treatments was evaluated by the Student’s two-sample t test for 2018 data and using a one-way analysis of variance (ANOVA) for the 2019 data. No significant differences in individual acids or pooled humulones and lupulones were observed between treatments in either harvest year.
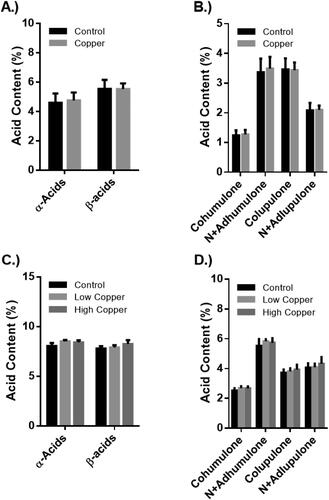
During boiling, hop α-acids are isomerized to iso-α-acids. The bitter taste of beer is predominantly attributable to these isomerized acids, but their concentration is dependent on the initial acid content of the hops. Therefore, the CBF-treated hops were expected to perform similarly to conventionally treated hops in the role of a bittering agent.
Hop essential oil characterization
Hop essential oils are the volatile, non-polar fraction of hop compounds and contain over 1,000 unique compounds, including terpenoids, alkanes, alcohols, and esters.[Citation34] Many of these molecules are aroma active and contribute floral, citrus, coniferous, and fruity notes that are characteristic of hops. The aroma of hop flowers is an important factor in consideration of the overall hop quality. Hop essential oils were extracted by steam distillation, and select terpenoids were quantified by gas chromatography. The most abundant terpenoids, including myrcene, humulene, caryophyllene, β-farnesene, and geranyl acetate, were identified by their retention times, relative quantities, and mass spectra. Their concentrations were expressed relative to that of the 2-octanol internal standard. Regardless of the harvest year or CBF treatment, no significant differences were found in the concentrations of these terpenoids (, p > 0.05). This result was expected as no previous research has linked excess copper content to the modulation of terpenoid content in hops. Morimoto et al. found no significant difference in the terpenoid content of untreated hops and those treated with the Bordeaux Mixture.[Citation17] If differences were detected between untreated and copper-treated hops, they might not correlate with a loss of hop aromas in many traditional beers as quantities of these non-polar compounds extracted from the hops during brewing are generally below their detection thresholds.[Citation35] This may, however, be dependent on a number of processing factors as the oxygenated derivatives of these terpenes may significantly contribute to the hoppy aromas in beer, but their occurrence is altered by hop storage conditions, boiling conditions, and yeast metabolism.[Citation35]
Figure 4. Hop essential oil profiles of copper-based fungicides (CBF)-treated hops. Essential oil component concentration expressed as internal standard equivalents (2-octanol) for the 2018 (Panel A) and 2019 (Panel B) seasons. Error bars represent the standard deviation of the samples for 2018 (n = 10 per treatment) and 2019 (n = 6 per treatment). Difference in terpenoid content between treatments was evaluated by the Student’s two-sample t test for 2018 data and using a one-way analysis of variance (ANOVA) for 2019. No significant differences in the quantified essential oil compounds were observed between treatments regardless of CBF-application frequency or harvest year.
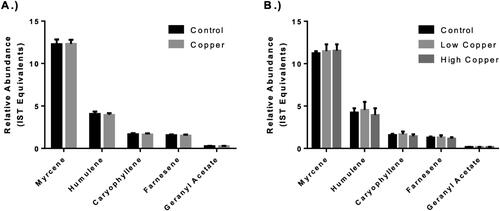
Hop thiol content
Thiols are a class of chemical compounds that contain a sulfhydryl (R–SH) moiety. While these sulfur-containing compounds are often associated with unpleasant odors such as alliaceous (2-mercapto-3-methyl-1-butanol) or burnt rubber (2-mercaptoethanol) some thiols, impart desirable aromas such as grapefruit, passionfruit, and black currant.[Citation36] The three major thiols found to impart these pleasant aromas in hops and in finished beer are 4-mercapto-4-methyl-2-pentanone (4-MMP: muscat, black currant), 3-mercaptohexanol (3-MH: passion fruit, grapefruit), and 3-mercaptohexyl acetate (3-MHA: grapefruit). Unlike the odor-active terpenoids found in hops, thiols are commonly found in beers above their detection thresholds.[Citation38,Citation39] This is because thiols tend to have exceedingly low detection thresholds (low ng/L). Therefore, these thiols and their conjugated precursors must be maintained in hop production as they directly impact the aroma of the finished beer.
Free thiols were extracted from dried hop cones and quantified by LC-MS. No significant differences between treatments were found in either harvest year (). The differences in thiol content across years are likely due to the differences in drying processes, although no comparisons are made between the years in this study. The lack of difference in thiol content between control and CBF-treated hops is contrary to previously published research. Morimoto et al. found that the application of Bordeaux Mixture resulted in a significant loss of 4-MMP in hop cones.[Citation17] This was likely attributable to a well-characterized reaction of cupric ions with free thiols resulting in the reduction of copper and the formation of a disulfide linkage.[Citation15,Citation19] Other work by Kishimoto et al. found that hops grown in Germany, a country in which there is a long history of using Bordeaux Mixture as a fungicide, had a minimal to no 4-MMP present in varieties that contained 4-MMP when grown elsewhere.[Citation21] It is unclear whether this was a result of long-term CBF use, increased soil levels of copper, or changes in hop physiology.
Figure 5. Hop thiol content of copper-based fungicides (CBF)-treated hops. 4-Mercapto-4-methylpentan-2-one (4MMP), 3-mercaptohexanol (3MH), and 3-mercaptohexyl acetate (3MHA) of hops from 2018 (Panel A) and 2019 (Panel B) expressed as ng/kg. Error bars represent the standard error of the mean for 2018 (n = 10 per treatment) and 2019 (n = 6 per treatment). Difference in individual thiol between treatments was evaluated by the Student’s two-sample t test for 2018 data and using a one-way analysis of variance (ANOVA) for the 2019 data. No significant differences in the quantified thiols were observed between treatments regardless of CBF-application frequency or harvest year.
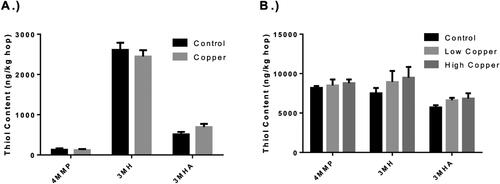
The fact that no differences were observed in the present study may be attributable to several different factors. The CBF used in work by Morimoto and colleagues contained copper sulfate as an ingredient in the CBF mixture.[Citation17] Copper sulfate is highly soluble and readily dissociates in an aqueous environment, releasing cupric ions that may readily react with free thiols. The CBF used in the present study contained only copper (II) hydroxide, which has limited solubility. The intent of this limited solubility is that it persists longer on the plant surface, providing antimicrobial activity over a more extended period. The limited solubility may inhibit the reaction of copper with the thiols to such a degree that the loss of thiol content does not occur or is immeasurable. Another explanation may be the term of CBF exposure in the hopyard. The hopyard used in the present study had, at the time of this study, only been treated with CBFs over two seasons, and the plants themselves were only three years old. The application history of the hopyard and age of the hop plants used by Morimoto et al. were not reported, but the work by Kishimoto et al. indicated that the loss of thiol content may be closely related to long-term CBF usage.[Citation17,Citation21]
Sensory analysis of dry-hopped beers
Hops from the 2018 season were used to dry hop American Pale Ale-style beers and were subjected to sensory analysis to determine if CBF-treatments had an impact on the sensory properties of beers prepared with those hops. Subjects were asked to evaluate the aroma and taste of the beers, which had been dry hopped at a rate of 2 g/L, which represents a modest hopping rate compared with industry-reported rates of 1–60 g/L depending on the style.[Citation35] No significant differences were found in the aroma of the beers (p = 0.09), with only 37 participants out of a total of 92 being able to identify the odd sample in a triangle test, indicating a pure chance event (d’= 0.89). The results for in-mouth flavor, however, were significantly above the chance level (p = 0.02), with 41 participants of the group able to identify the odd sample out. Based on this result, we conclude that there was either a perceptible (d’= 1.17) difference in basic taste (i.e., bitterness, sourness, sweetness, etc.) or the aroma as perceived through retronasal olfaction. This result was unexpected based on the other analyses performed on the hops that indicated the only detectable differences were in the copper content of the hops. There may be differences that are not detectable by the chemical analyses performed but are detectable through human sensory perception. It has been demonstrated that humans are capable of detecting aroma and flavor components in the parts-per-billion and even parts-per-trillion ranges.[Citation39] Such levels of sensitivity are often far beyond the detection capabilities of commonly employed chemical or instrumental analyses. The human sensory evaluation also accounts for the perception of numerous compounds as a single, more complex aroma or flavor, so perceived differences cannot always be correlated with differences in a single compound or class of compounds. Therefore, the detected differences in the sensory testing may not be readily apparent in the chemical analysis of the hops themselves.
While the evaluation of the hops in beer is readily applicable to understanding how they differ in their most likely mode of usage, the beer itself may confound the evaluation of the hop-specific sensory properties. While efforts were taken to minimize differences in beer production, minor differences in beer preparation, serving temperature, or carbonation may result in perceived differences not attributable to the hops themselves but would not likely affect the result of this test as the triads were counterbalanced across participants. These differences become likely when a beer is produced in small batches at a pilot-scale. Further evaluation of the hop cones themselves is necessary to confirm the perceived differences detected in the initial sensory study. In addition to performing discriminatory testing, descriptive testing must also be conducted to identify not only if the hops differ, but in what manner.
Conclusions
In summary, we found that the treatment of hops with a copper (II) hydroxide-based fungicide significantly increased the copper content of hop cones regardless of treatment frequency. Across both seasons, we found that the use of CBFs increased the total copper content of the hops by 114–159% compared with those hops treated only with a copper-free fungicide. The frequency of application was evaluated and found to have no significant effect on the final copper content, indicating that application timing relative to harvest may have a greater impact on total copper content rather than total seasonal copper load. While the increase in copper concentrations is relatively small (parts per billion), one must consider the widespread use of CuBFs in the agricultural system. A minimal amount of research has been published regarding the increases in the copper content of agricultural goods due to the use of copper fungicides. Further research into the increased levels of copper in our food supply, its bioavailability, and any potential negative impacts is necessary.
Beyond the increased copper content, no significant changes in markers of hop quality were identified. Yield, essential oil composition, α- and β-acid content, and varietal thiol content were all evaluated, and no significant differences were observed between CBF-treated and control hops regardless of application frequency. Hops were used to dry-hop beers for sensory analysis. No differences in the aromas of beer prepared with CBF-treated and control hops could be detected in a sensory discrimination test by a panel of untrained beer consumers (p = 0.09, n = 92). Significant differences were observed in the in-mouth perception of the beers indicating a noticeable difference in either the basic taste properties or retro-nasal aroma perception. Further sensory evaluation of the hops independently is necessary to ensure the detected differences are attributable to the hops themselves rather than the beers. Descriptive sensory testing is also warranted to identify the ways in which the hop aromas and flavors may differ.
Based on the data presented, we conclude that the use of CBFs, specifically a copper (II) hydroxide formulation, would not have any adverse effect on the quality or functionality of hops and would, therefore, be recommended for use in hop disease prevention strategies. Further investigation into the potential impact of excess copper in products prepared with CBF-treated hops is necessary.
Acknowledgments
The authors would like to acknowledge Timothy Grove, Thomas Butzler, Thomas Ford, Tanner Delvalle, Leah Fronk, and Elsa Sanchez for their help in establishing and maintaining the hopyard at Penn State’s Russell E. Larson Research and Education Center at Rock Springs.
Disclosure statement
The authors report no potential conflict of interest.
Additional information
Funding
Literature cited
- National Hop Report. USDA National Agricultural Statistics Service, 2017.
- Péneau, S.; Hoehn, E.; Roth, H. R.; Escher, F.; Nuessli, J. Importance and Consumer Perception of Freshness of Apples. Food Qual. Prefer. 2006, 17, 9–19. DOI: https://doi.org/10.1016/j.foodqual.2005.05.002.
- Berna, A. Z.; Lammertyn, J.; Buysens, S.; Di Natale, C.; Nicolaï, B. M. Mapping Consumer Liking of Tomatoes with Fast Aroma Profiling Techniques. Postharvest. Biol. Technol. 2005, 38, 115–127. DOI: https://doi.org/10.1016/j.postharvbio.2005.05.013.
- Gilbert, J. M.; Young, H.; Ball, R. D.; Murray, S. H. Volatile Flavor Compounds Affecting Consumer Acceptability of Kiwifruit. J. Sens. Stud. 1996, 11, 247–259. DOI: https://doi.org/10.1111/j.1745-459X.1996.tb00044.x.
- Palti, J.; Cohen, Y. Downy Mildew of Cucurbits (Pseudoperonospora cubensis): The Fungus and Its Hosts, Distribution, Epidemiology and Control. Phytoparasitica 1980, 8, 109–147. DOI: https://doi.org/10.1007/BF02994506.
- Cadle-Davidson, L. Variation within and between Vitis spp. for Foliar Resistance to the Downy Mildew Pathogen Plasmopara viticola. Plant Dis. 2008, 92, 1577–1584. DOI: https://doi.org/10.1094/PDIS-92-11-1577.
- Johnson, G. F. The Early History of Copper Fungicides. Agric. Hist. 1935, 9, 67–79.
- Atwood, D.; Paisley-Jones, C. Pesticides Industry Sales and Usage 2008-2012 Market Estimates; EPA, United States Environmental Protection Agency: Washington, DC, 2017.
- Mahaffee, W. F.; Pethybridge, S. J. Gent DH: Compendium of Hop Diseases and Pests; American Phytopathological Society (APS Press): St. Paul, MN, 2009.
- Gent, D. H.; Ocamb, C. M.; Farnsworth, J. L. Forecasting and Management of Hop Downy Mildew. Plant Dis. 2010, 94, 425–431. DOI: https://doi.org/10.1094/PDIS-94-4-0425.
- Johnson, D. A.; Alldredge, J. R.; Allen, J. R. Weather and Downy Mildew Epidemics of Hop in Washington State. Phytopathology 1994, 84, 524–527. DOI: https://doi.org/10.1094/Phyto-84-524.
- Lizotte, E.; Miles, T. Managing Hop Downy Mildew in Michigan; Michigan State University Extension: East Lansing, MI, 2020.
- Skotland, C.; Johnson, D. A. Control of Downy Mildew of Hops. Plant Dis. 1983, 67, 1183–1186. DOI: https://doi.org/10.1094/PD-67-1183.
- Ferreira, I. C.; Barros, L.; Soares, M. E.; Bastos, M. L.; Pereira, J. A. Antioxidant Activity and Phenolic Contents of Olea europaea L. leaves Sprayed with Different Copper Formulations. Food Chem. 2007, 103, 188–195. DOI: https://doi.org/10.1016/j.foodchem.2006.08.006.
- Kreitman, G. Y.; Danilewicz, J. C.; Jeffery, D. W.; Elias, R. J. Reaction Mechanisms of Metals with Hydrogen Sulfide and Thiols in Model Wine. Part 1: Copper-Catalyzed Oxidation. J. Agric. Food Chem. 2016, 64, 4095–4104. DOI: https://doi.org/10.1021/acs.jafc.6b00641.
- Kreitman, G. Y.; Danilewicz, J. C.; Jeffery, D. W.; Elias, R. J. Copper (II)-Mediated Hydrogen Sulfide and Thiol Oxidation to Disulfides and Organic Polysulfanes and Their Reductive Cleavage in Wine: Mechanistic Elucidation and Potential Applications. J. Agric. Food Chem. 2017, 65, 2564–2571. DOI: https://doi.org/10.1021/acs.jafc.6b05418.
- Morimoto, M.; Kishimoto, T.; Kobayashi, M.; Yako, N.; Iida, A.; Wanikawa, A.; Kitagawa, Y. Effects of Bordeaux Mixture (Copper Sulfate) Treatment on Blackcurrant/Muscat-Like Odors in Hops and Beer. J. Am. Soc. Brew. Chem. 2010, 68, 30–33. DOI: https://doi.org/10.1094/ASBCJ-2009-1118-01.
- Michalak, A. Phenolic Compounds and Their Antioxidant Activity in Plants Growing under Heavy Metal Stress. Polish J. Environ. Stud. 2006, 15, 523–530.
- Laglera, L. M.; van den Berg, C. M. G. Copper Complexation by Thiol Compounds in Estuarine Waters. Mar. Chem. 2003, 82, 71–89. DOI: https://doi.org/10.1016/S0304-4203(03)00053-7.
- Lermusieau, G.; Collin, S. Volatile Sulfur Compounds in Hops and Residual Concentrations in Beer: A Review. J. Am. Soc. Brew. Chem. 2003, 61, 109–113. DOI: https://doi.org/10.1094/ASBCJ-61-0109.
- Kishimoto, T.; Kobayashi, M.; Yako, N.; Iida, A.; Wanikawa, A. Comparison of 4-Mercapto-4-Methylpentan-2-One Contents in Hop Cultivars from Different Growing Regions. J. Agric. Food Chem. 2008, 56, 1051–1057. DOI: https://doi.org/10.1021/jf072173e.
- Vermeulen, C.; Guyot-Declerck, C.; Collin, S. Combinatorial Synthesis and Sensorial Properties of Mercapto Primary Alcohols and Analogues. J. Agric. Food Chem. 2003, 51, 3623–3628. DOI: https://doi.org/10.1021/jf0212340.
- George, A. 2019 Statistical Report; Hop Growers of America, Yakima, WA, 2019.
- Modular Hop Oast or Tray Drier for Herbs. https://farmhack.org/tools/modular-hop-oast-or-tray-drier-herbs.
- ASBC Methods of Analysis. Hops Method 13. Total Essential Oils in Hops and Hop Pellets by Steam Distillation; American Society of Brewing Chemists: St. Paul, MN, 2008. https://www.asbcnet.org/Methods/Pages/default.aspx.
- ASBC Methods of Analysis. Hops Method 17. Hop Essential Oils by Capillary Gas Chromatography-Flame Ionization Detection; American Society of Brewing Chemists: St. Paul, MN, 2004. https://www.asbcnet.org/Methods/Pages/default.aspx.
- ASBC Methods of Analysis. Hops Method 14. α-Acids and β-Acids in Hops and Hop Extracts by HPLC; American Society of Brewing Chemists: St. Paul, MN, 2010. DOI: https://doi.org/10.1094/ASBCMethod-Hops14.
- Capone, D. L.; Ristic, R.; Pardon, K. H.; Jeffery, D. W. Simple Quantitative Determination of Potent Thiols at Ultratrace Levels in Wine by Derivatization and High-Performance Liquid Chromatography–Tandem Mass Spectrometry (HPLC-MS/MS) Analysis. Anal. Chem. 2015, 87, 1226–1231. DOI: https://doi.org/10.1021/ac503883s.
- Darby, H.; Cubins, J.; Lewins, S.; Post, J, Hop Biofungicide Trial. Northwest Crops & Soils Program 2016, https://scholarworks.uvm.edu/nwcsp/99/.
- Krofta, K.; Pokorný, J.; Kudrna, T.; Ježek, J.; Pulkrábek, J.; Křivánek, J.; Bečka, D. J. P. The Effect of Application of Copper Fungicides on Photosynthesis Parameters and Level of Elementary Copper in Hops. Plant Soil Environ. 2012, 58, 91–97.
- Bokare, A. D.; Choi, W. Review of Iron-Free Fenton-like Systems for Activating H2O2 in Advanced Oxidation Processes. J. Hazard Mater. 2014, 275, 121–135. DOI: https://doi.org/10.1016/j.jhazmat.2014.04.054.
- Bamforth, C. W.; Muller, R. E.; Walker, M. D. Oxygen and Oxygen Radicals in Malting and Brewing: A Review. J. Am. Soc. Brew. Chem. 1993, 51, 79–88. DOI: https://doi.org/10.1094/ASBCJ-51-0079.
- Sharp, D. C.; Townsend, M. S.; Qian, Y.; Shellhammer, T. H. Effect of Harvest Maturity on the Chemical Composition of Cascade and Willamette Hops. J. Am. Soc. Brew. Chem. 2014, 72, 231–238. DOI: https://doi.org/10.1094/ASBCJ-2014-1002-01.
- Roberts, M. T.; Dufour, J. P.; Lewis, A. Application of Comprehensive Multidimensional Gas Chromatography Combined with Time‐of‐Flight Mass Spectrometry (GC × GC‐TOFMS) for High Resolution Analysis of Hop Essential Oil. J. Sep. Sci. 2004, 27, 473–478. DOI: https://doi.org/10.1002/jssc.200301669.
- Lafontaine, S. R.; Shellhammer, T. H. Investigating the Factors Impacting Aroma, Flavor, and Stability in Dry-Hopped Beers. Tech. Q. Master Brew. Assoc. Am. 2019, 56, 13–23.
- Vermeulen, C.; Gijs, L.; Collin, S. Sensorial Contribution and Formation Pathways of Thiols in Foods: A Review. Food Rev. Int. 2005, 21, 69–137. DOI: https://doi.org/10.1081/FRI-200040601.
- Vermeulen, C.; Lejeune, I.; Tran, T.; Collin, S. Occurrence of Polyfunctional Thiols in Fresh Lager Beers. J. Agric. Food Chem. 2006, 54, 5061–5068. DOI: https://doi.org/10.1021/jf060669a.
- Takazumi, K.; Takoi, K.; Koie, K.; Tuchiya, Y. Quantitation Method for Polyfunctional Thiols in Hops (Humulus lupulus l.) and Beer Using Specific Extraction of Thiols and Gas Chromatography–Tandem Mass Spectrometry. Anal. Chem. 2017, 89, 11598–11604. DOI: https://doi.org/10.1021/acs.analchem.7b02996.
- Desrochers, R.; Keane, P.; Ellis, S.; Dowell, K. Expanding the Sensitivity of Conventional Analytical Techniques in Quality Control Using Sensory Technology. Food Qual. Prefer. 2002, 13, 397–407. DOI: https://doi.org/10.1016/S0950-3293(02)00078-2.