Abstract
Pacific salmon Oncorhynchus spp. face serious challenges from climate and landscape change, particularly in the southern portion of their native range. Conversely, climate warming appears to be allowing salmon to expand northwards into the Arctic. Between these geographic extremes, in the Gulf of Alaska region, salmon are at historically high abundances but face an uncertain future due to rapid environmental change. We examined changes in climate, hydrology, land cover, salmon populations, and fisheries over the past 30–70 years in this region. We focused on the Kenai River, which supports world-famous fisheries but where Chinook Salmon O. tshawytscha populations have declined, raising concerns about their future resilience. The region is warming and experiencing drier summers and wetter autumns. The landscape is also changing, with melting glaciers, wetland loss, wildfires, and human development. This environmental transformation will likely harm some salmon populations while benefiting others. Lowland salmon streams are especially vulnerable, but retreating glaciers may allow production gains in other streams. Some fishing communities harvest a diverse portfolio of fluctuating resources, whereas others have specialized over time, potentially limiting their resilience. Maintaining diverse habitats and salmon runs may allow ecosystems and fisheries to continue to thrive amidst these changes.
INTRODUCTION
Climate change, habitat degradation, and other stressors pose serious challenges to many Pacific salmon Oncorhynchus spp. populations, and these challenges are particularly acute in the southern portion of their native range (CitationIsaak et al. 2011; CitationLawrence et al. 2014; CitationWard et al. 2015). Conversely, as their climate envelope shifts northward, salmon are apparently expanding into Arctic and subarctic watersheds (CitationNielsen et al. 2013; CitationPoesch et al. 2016), where habitats are generally intact. Between these geographic extremes, in the core of their native range stretching from Kamchatka to Southeast Alaska (CitationAugerot and Foley 2005), wild salmon remain abundant and productive but face an uncertain future.
The biomass and commercial harvest of wild salmon in the North Pacific Ocean are currently near all-time highs, and roughly one-third of these salmon spawn in the Gulf of Alaska region, including the southern Alaska Peninsula, southcentral Alaska, and Southeast Alaska (CitationIrvine and Fukuwaka 2011; CitationIrvine and Ruggerone 2016). Salmon rivers in this region face a complex set of climate-driven changes, including rising summer stream temperatures (CitationMauger et al. 2017), melting glaciers (CitationO'Neel et al. 2015), and increasing streamflows during fall and winter, when developing embryos are vulnerable to streambed scour (CitationShanley and Albert 2014). In combination, these climatic changes may reduce the productivity of some salmon species, populations, and life stages while benefiting others (CitationLeppi et al. 2014; CitationShanley et al. 2015). A patchwork of human development adds further complexity; whereas some salmon rivers are located in protected areas, many others are vulnerable to habitat loss and other human impacts (CitationScannell 2012; CitationAlbert and Schoen 2013; CitationSepulveda et al. 2015). The net effects of these changes on ecosystems and fisheries defy simple prediction, and this uncertainty complicates the tasks of natural resource management, conservation, and habitat restoration (CitationSchindler and Hilborn 2015).
The Kenai River in southcentral Alaska exemplifies the high social, economic, and ecological value of wild salmon and the complex changes they face in the Gulf of Alaska region. Kenai River salmon support several of Alaska's largest recreational salmon fisheries, major commercial gill-net and personal-use dip-net fisheries, as well as small-scale subsistence and educational fisheries (CitationBegich et al. 2013; CitationShields and Dupuis 2016). The river is famous for its large Chinook Salmon O. tshawytscha, including a 44-kg (97 lb) world record fish caught in 1985. However, recent declines in Chinook Salmon returns have prompted fishery closures and exacerbated long-standing conflicts between stakeholders (CitationLoring 2016). These declines, coinciding with state-wide declines in Chinook Salmon returns and body size (CitationADFG 2013; CitationLewis et al. 2015), have raised concerns about the resilience of these salmon runs and their dependent fishing communities to rapid environmental change (CitationLoring et al. 2014).
In this article, we synthesize 70 years of measured changes in climate, hydrology, land cover, freshwater habitat, salmon populations, and fisheries in rivers draining into the Gulf of Alaska, using the Kenai River watershed in southcentral Alaska as a central case study. We focus on the coupled terrestrial and freshwater habitats of large, complex watersheds () rather than small coastal streams or marine ecosystems (e.g., CitationO'Neel et al. 2015). Further, we interpret downscaled climate model projections for the region in light of current knowledge of how landscape features determine the sensitivity of aquatic ecosystems to regional climate trends. Finally, we discuss critical uncertainties and management decisions facing fishing communities in the Kenai River basin. Our objective is to identify trends, vulnerabilities, and management opportunities likely to affect these highly productive ecosystems and valuable fisheries.
STUDY AREA
The Gulf of Alaska drainage basin encompasses the southern Alaska Peninsula, southcentral Alaska, and Southeast Alaska, bordered to the north by the highest mountain ranges in North America. We refer to this area as the Gulf of Alaska region throughout the article. This region is heavily glacierized (ice covered) and receives tremendous inputs of freshwater from precipitation and glacier runoff (CitationNeal et al. 2010). The landscape is drained by a diverse array of salmon-bearing watersheds, ranging from large floodplain rivers to small, steep coastal streams (CitationADFG 2017).
In many respects, the Kenai Peninsula in southcentral Alaska represents a microcosm of the broader region, containing icefields, alpine tundra, boreal forests, temperate rainforests, and wetlands, and straddling two distinct climatic zones. The mountainous eastern peninsula is characterized by a subarctic maritime climate with relatively heavy precipitation, and the western peninsula lies in the rain shadow of the Kenai Mountains and is characterized by a subarctic continental climate (CitationShulski and Wendler 2007). The eastern mountains are covered by glaciers, alpine tundra, and coastal temperate rainforests (CitationO'Neel et al. 2015), and the western lowlands are covered by boreal forests and extensive wetlands (CitationKlein et al. 2005).
The Kenai River flows 182 km through the center of the peninsula and empties into Cook Inlet near the cities of Kenai and Soldotna (). Glaciers descending from the Harding and Sargent icefields cover 11% of its 5,568 km2 watershed (CitationDorava and Milner 2000). Three gauged tributaries illustrate the spectrum of lowland, montane, and glacially influenced salmon streams found throughout the region ( and ). Lowland streams such as Beaver Creek (2% average gradient, 0% glacial coverage) have the least streamflow relative to their basin area, with peak flows occurring during a rapid spring snowmelt period, followed by lower midsummer flows. Montane tributaries such as the Russian River (9% average gradient, <1% glacial coverage) are characterized by a later and longer-lasting spring freshet fed by melting alpine snowpack. Glacially influenced tributaries such as Ptarmigan Creek (14% average gradient, 7% glacial coverage) have the greatest flows relative to their basin area, with midsummer peaks in discharge when glacial melt reaches its maximum. All tributary types typically exhibit secondary peak flows caused by fall rains. The Kenai and many other rivers in the region flow through lakes, which influence their hydrology in important ways detailed below. Discharge of the mainstem Kenai River generally peaks during July and August and reaches a minimum between January and March ().
Figure 2. Kenai River watershed (area within grey border) including three focal subbasins (green polygons) and glaciers (white polygons) within the watershed. Red lines are major highways and orange lines are secondary roads.
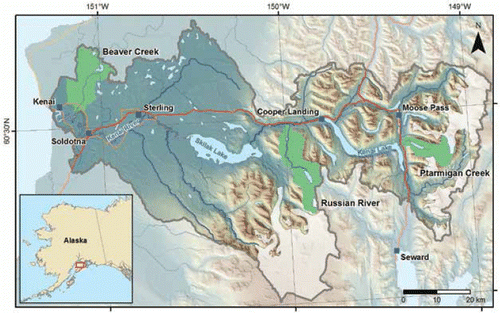
Figure 3. Seasonal hydrographs of the mainstem Kenai River and three tributaries representing lowland (Beaver Creek), montane (Russian River), and glacially influenced montane (Ptarmigan Creek) flow regimes. Colored lines represent daily mean discharge standardized by drainage area, and shaded bands represent variability (±1 SD) among years. Data record covers 51 years for the main stem and 8–12 years for each tributary. The large spike in December reflects a single storm event captured in the period of record for two of the tributaries. Data from the U.S. Geological Survey.
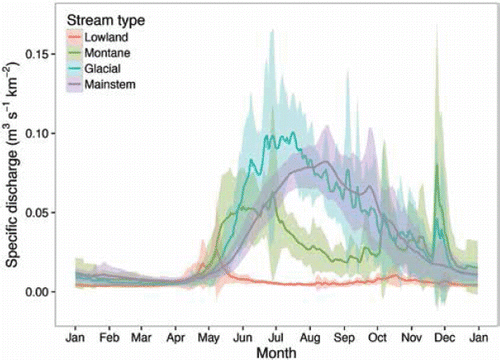
CLIMATE CHANGE AND SENSITIVITY OF FRESHWATER HABITATS
Alaska has warmed at more than twice the rate of the rest of the United States over the past 60 years, and rapid warming is projected to continue through the end of this century (CitationChapin et al. 2014). Historical trends in precipitation are largely unclear, due to high interannual variability, data gaps, and changes in methodology and location of some weather stations (CitationMcAfee et al. 2013, Citation2014a; CitationKane and Stuefer 2015). Climate models predict that Alaska will receive more total annual precipitation as the climate warms but that water availability will decrease during summer due to increasing evapotranspiration during a longer growing season (CitationChapin et al. 2014). Further, in coastal regions bordering the Gulf of Alaska, more winter precipitation is projected to fall as rain rather than snow (CitationMcAfee et al. 2014b).
Climate change is well documented at the Kenai Municipal Airport, near the mouth of the Kenai River (e.g., CitationBerg et al. 2009; CitationBauret and Stuefer 2013). This station has a >70-year, continuous, homogenous, and reliable weather record (CitationMcAfee et al. 2013), representative of coastal areas west of the Kenai Mountains. We illustrated the scope and seasonality of the changes at Kenai by plotting the monthly mean air temperature and precipitation recorded during two historical time periods, 1943–1969 and 1970–2012, with climate model projections for two future time periods, 2030–2049 and 2070–2089 (). We set 1970 as the cutoff between historical time periods to align with documented climatic regime shifts beginning in 1968 in the Kenai Lowlands (CitationBerg et al. 2009) and during the mid-1970s in the North Pacific (CitationMantua et al. 1997; CitationMeehl et al. 2009). Climate projections were based on a five-model average using the most recent global circulation models (AR5) from the International Panel on Climate Change, statistically (delta) downscaled for Kenai to allow comparison with the historical record (CitationSNAP 2016).
Figure 4. Observed and projected changes in (A) air temperature and (B) precipitation at the city of Kenai and (C) observed changes in discharge in the Kenai River at the town of Cooper Landing. Observed data from the National Weather Service (air temperature and precipitation) and U.S. Geological Survey (discharge). Climate projections from the Scenarios Network for Alaska and Arctic Planning (2016). Intermediate emissions scenario (RCP 6.0) shown.
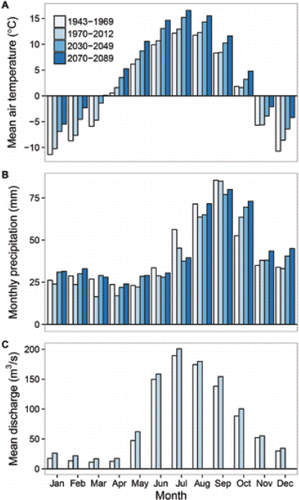
Mean annual air temperatures at Kenai have increased significantly, and all seasons except fall have become warmer on average (; CitationBauret and Stuefer 2013). Total annual precipitation has decreased substantially, spring and summer have become drier, and fall has become wetter (; CitationBauret and Stuefer 2013; CitationMcAfee et al. 2013). Warmer, drier summers have caused a 55% decline in available water for plant growth, streamflow, and groundwater recharge in the Kenai Lowlands since 1968 (CitationBerg et al. 2009). Climate models predict further increases in air temperatures during all months of the year and increased precipitation during fall and winter, but models differ on future trends in summer precipitation.
Climate change is also altering the flow and temperature regimes of Alaska salmon streams. On average, spring snowmelt and peak stream runoff occur several weeks earlier now than in the 1950s (CitationStewart et al. 2005). Hydrological models predict that drier summers and warmer, wetter winters will increase the risks of flooding and drought in many salmon streams across southern Alaska (CitationLeppi et al. 2014; CitationShanley and Albert 2014; CitationWobus et al. 2016). However, streams are not responding uniformly, because varied landscapes filter the effects of regional climate drivers on aquatic habitat characteristics like streamflow and water temperature (CitationSchindler et al. 2008). For example, as flood size increases, higher-gradient upland streams are more sensitive to streambed scour than unconfined floodplain channels (CitationSloat et al. 2017). Conversely, lowland streams are more sensitive to low midsummer flows than streams fed by high-elevation snowpack or glaciers (CitationFellman et al. 2015). Indeed, despite the trend of drier summers in the surrounding Kenai Lowlands (CitationBerg et al. 2009), average summer discharge has increased modestly in the glacially influenced Kenai River over the past 70 years (), likely due to increasing glacial melt. Glacial meltwater will not buffer flow regimes forever though; runoff has probably already peaked and begun to decline for most glaciers in Alaska (CitationBliss et al. 2014).
Landscape features also influence the sensitivity of stream temperature to rising air temperature, making some streams more vulnerable to climate warming than others. Stream temperatures of nonglacial Alaska streams are more sensitive to air temperatures in lower-elevation, larger, rain-dominated watersheds with lower average watershed slope (CitationLisi et al. 2015; CitationMauger et al. 2017; CitationWinfree 2017). Forecasting future stream temperatures is challenging because the thermal sensitivities of watersheds can change over time, introducing nonlinear thresholds into warming trends (CitationArismendi et al. 2014). For example, streams that are currently buffered by glacial or snowmelt inputs may suddenly become more sensitive to air temperature as snowlines rise and smaller glaciers disappear from their upper watersheds (CitationLisi et al. 2015). More information is needed to understand how stream temperature sensitivity changes on seasonal and interannual time-scales, in order to identify and conserve habitats that will be thermally suitable for productive salmon runs in a warmer world. The role of landscapes in filtering climate change effects on salmon is only part of the picture, however, because many of these landscapes themselves are also rapidly changing.
LANDSCAPE CHANGE AND ITS EFFECTS ON FRESHWATER COMMUNITIES
Glacial Mass Loss
Freshwater systems flowing into the Gulf of Alaska are strongly influenced by glaciers, which are rapidly retreating and losing mass overall (; CitationArendt et al. 2002; CitationHill et al. 2015). For example, every glacier in a sample of 347 in southcentral Alaska receded from 1948 to 2009, based on satellite images, with an overall loss of 23% of glaciated area (CitationLe Bris et al. 2011). Harding Icefield, the largest source of glacial runoff to the Kenai River, thinned by 30 m from 1950 to 1999 (CitationVanLooy et al. 2006). Wolverine Glacier, a representative coastal glacier in the Kenai Mountains, has rapidly lost mass since the late 1980s, based on a long-term time series of field observations (; CitationO'Neel et al. 2014).
Figure 5. Perspective rendering showing retreat of the Skilak Glacier, a major source of glacial runoff to the Kenai River. Colored lines indicate ice extent for 1952, 1978, and 2013 derived from aerial photographs. The toe of Skilak Glacier retreated only slightly between 1952 and 1978 and then it retreated rapidly over 4 km between 1978 and 2013, creating a new proglacial lake. An interactive slider graphic showing greater detail is available at https://ak-nsf-epscor.github.io/kenai-change (July 2017).
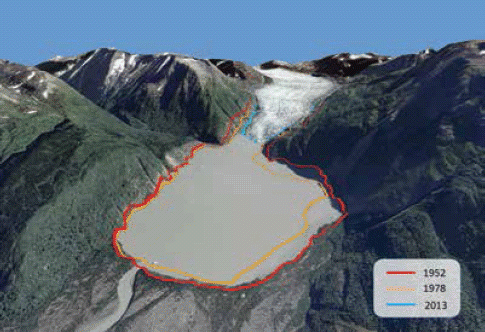
Figure 6. Landscape changes on the Kenai Peninsula: (A) cumulative loss of mass, meters of water equivalent, of Wolverine Glacier, a representative coastal glacier in the Kenai Mountains (data from CitationO'Neel et al. 2014; S. O'Neel, U.S. Geological Survey, unpublished data); (B) cumulative change in herbaceous wetland area at 11 representative sites, Kenai Lowlands, means ± SE (data from CitationBerg et al. 2009); (C) frequency of lightning- and human-caused wildfires (area >40.5 ha) in the Kenai Peninsula Borough (curves represent Poisson regression fits; see Supplemental Information for methods); and (D) increasing footprint of human development within 1 km of the lower Kenai River (determined from aerial photography; see Supplemental Information for methods).
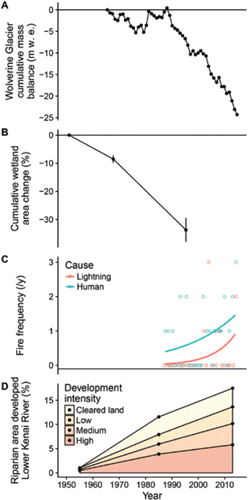
Regional losses of glacial mass likely have both positive and negative implications for freshwater ecosystems and salmon populations (CitationO'Neel et al. 2015), but the net effects remain poorly understood. Most obviously, retreating glaciers expose new streams and proglacial lakes for freshwater biota to colonize (CitationMilner et al. 2000, Citation2007). Less visible, but potentially more important to regional salmon production, are the effects of changing glacial inflows. Loss of glacial mass is unlikely to dramatically alter the total annual discharge of coastal Alaska rivers, which receive most of their streamflow from precipitation and seasonal fluctuations in glacier mass; however, it could cause important changes in seasonal discharge and water quality (CitationO'Neel et al. 2014). Glacially influenced rivers exhibit greater midsummer streamflows, lower water temperatures, enhanced concentrations of nutrients and organic carbon, and elevated sediment loads (and associated turbidity) relative to nonglacial rivers (CitationMilner et al. 2001; CitationHood and Berner 2009). As glaciers lose mass, short-term increases in meltwater inputs can reduce the production of zoo-plankton and Sockeye Salmon O. nerka in lakes (CitationEdmundson et al. 2003); the diversity and production of microorganisms, algae, and benthic macroinvertebrates in rivers; and the quality of fish spawning habitat (CitationMilner et al. 2009). However, moderate levels of glacial discharge can provide important benefits to freshwater ecosystems. Glacial inflows can moderate water temperatures and help maintain dissolved oxygen levels necessary for fish survival during hot, dry summers (CitationFellman et al. 2015). Glacial discharge can also support connectivity within a mosaic of mainstem and off-channel riverine habitats throughout the growing season, providing important rearing habitat for juvenile Chinook Salmon and Coho Salmon O. kisutch (CitationMurphy et al. 1989; CitationRine et al. 2016). Proglacial lakes, including the Kenai and Skilak lakes in the Kenai River watershed, moderate the flow, turbidity, and temperature of glacial rivers (CitationDorava and Milner 2000; CitationKyle and Brabets 2001), providing rearing habitat for juvenile Sockeye Salmon, enhancing the productivity and diversity of freshwater invertebrates and salmon stocks, and prolonging the seasonal availability of salmon for human harvest in downriver reaches (Box 1). Diminishing glacial influence may reduce these benefits and enhance the sensitivity of aquatic ecosystems to warmer air temperatures and other aspects of climate change (CitationKyle and Brabets 2001). It remains unclear whether glacial mass loss will enhance or reduce the overall salmon productivity of the Gulf of Alaska region. As these changes unfold, several simultaneous processes are transforming both glacial and nonglacial watersheds.
Wetland Loss
Wetland loss is occurring throughout the Alaska boreal forest as summers become warmer and drier (CitationRiordan et al. 2006). This pattern is well documented in the Kenai Peninsula lowlands, where large areas of herbaceous wetlands are underlain by peat that has accumulated since the end of the Wisconsin glaciation 18,000 years ago (CitationBerg et al. 2009). As the climate warmed and dried during the past 50 years, lakes and ponds shrank, water tables fell, and wetlands were replaced by shrubs and black spruce Picea mariana forest (CitationKlein et al. 2005). Wetland loss has accelerated since the 1970s, reaching a rate of 9% ± 1.5% per decade (mean surface area ± SE; ; CitationBerg et al. 2009).
The loss of wetlands has important consequences for salmon streams, especially in lowlands. Wetlands export nutrients to habitats downstream (CitationFellman et al. 2009), provide food and habitat for aquatic invertebrates and fish (CitationDavid et al. 2016), and filter sediment and pollutants from surface runoff (CitationZedler and Kercher 2005). Wetlands can also buffer streamflows by storing water and releasing it during dry periods (CitationGracz et al. 2015). Consequently, loss or disruption of these wetlands could magnify the effects of climate change on Alaska salmon by further reducing streamflows during summer (CitationGracz et al. 2015).
Accelerating Disturbance Regimes
Warmer, drier conditions are also accelerating the frequency and severity of disturbances such as wildfires and insect outbreaks in temperate forests worldwide (CitationMillar and Stephenson 2015). Humans have been the primary cause of fire on the Kenai Peninsula since European settlement began around 1850, which was marked by a 10-fold increase in soil charcoal accumulation (CitationLynch et al. 2002; CitationBerg and Anderson 2006). Lightning strikes were historically rare (CitationLynch et al. 2002), but lightning-caused fires have occurred with increasing frequency during the past 20 years (; see Supplemental Information). Spruce bark beetle Dendroctonus rufipennis outbreaks have also increased in extent following recent strings of consecutive warm summers, including a major region-wide outbreak in the 1990s (CitationBerg et al. 2006). This beetle outbreak is thought to have increased the likelihood of fire in mixed stands of black spruce and white spruce P. glauca, which are common in the lower Kenai River watershed (CitationHansen et al. 2016).
The alteration of natural disturbance regimes will affect riverine ecosystems in important ways (CitationWolken et al. 2011). Riverine networks are inextricably linked to and dependent upon riparian habitats (CitationVannote et al. 1980; CitationWallace et al. 1997; CitationWipfli and Richardson 2015). Riparian trees shade streams, moderating their temperatures, and provide large wood that creates pool habitats for rearing salmonids (CitationRoni and Quinn 2001). Canopy loss from fire and forest insect outbreaks can be problematic for small streams sensitive to heating (CitationDunham et al. 2007). Fire can also provide benefits, for example, by increasing wood delivery to rearing habitats of juvenile salmon (CitationFlitcroft et al. 2016). However, a rapid increase in fire and other disturbances in Alaska boreal forests will not necessarily improve salmon habitat. Fire and beetle outbreaks are projected to initially increase wood and fine organic debris inputs on Kenai Peninsula rivers (CitationRinella et al. 2009) but over the long term could reduce the rate and size of wood input, especially if fires become more frequent and intense, inhibiting forest regrowth. Forested riparian zones also provide leaf litter and terrestrial insect inputs to freshwater food webs (CitationWipfli 1997). Leaf litter is an essential source of energy and nutrients for aquatic insects, which in turn are food for rearing salmonids in Alaska streams (CitationWipfli 1997; CitationAllan et al. 2003). Terrestrial plants supply close to half of the food ingested by salmonids in coastal forested streams in Alaska through direct inputs of terrestrial insects sourced from forest canopies and understories (CitationWipfli 1997; CitationAllan et al. 2003; CitationRoon et al. 2016). Wildfires may increase food supplies for salmonids in the short term (CitationMellon et al. 2008), but longer term effects are unknown. Terrestrial vegetation also moderates surface runoff, and loss of vegetation cover at more frequent intervals will increase sediment loading and routing into streams.
Human Development and Activities
Despite Alaska's reputation as a sparsely populated wilderness, the expanding footprint of human development is now a significant disturbance process influencing many salmon-bearing watersheds. In southcentral Alaska, home to 62% of the state's population (CitationAlaska Department of Labor and Workforce Development 2016), cities and roads are clustered in lowlands and often along rivers, increasing the potential for impacts on salmon ecosystems. We quantified the expanding footprint of human development in the Kenai River watershed over the past six decades using high-resolution aerial photography and the National Land Cover Database (see Supplemental Information for methods). The area occupied by human development increased more than 20-fold, from 3.5 km2 in the 1950s to 57 km2 in the 1980s and 76 km2 in 2013. Despite this rapid growth, less than 2% of the overall Kenai River watershed was considered developed in 2013. However, this development was highly concentrated within the lower Kenai River riparian zone (), which we defined as land within 1 km of the river between Skilak Lake and Cook Inlet. Though this represents less than 3% of the watershed area, nearly half of the medium- and high-density development and more than one-third of all development were concentrated within the lower Kenai River riparian zone. The human footprint covered 1% of the lower river riparian zone in the 1950s, expanding to 12% in the 1980s and 17% in 2013 (). This pattern is reinforced by the distribution of land ownership: for example, protected federal lands (Chugach National Forest and Kenai National Wildlife Refuge) comprise 88% of the watershed area but only 10% of the lower Kenai River banks (CitationArendt et al. 2010).
Figure 7. The expanding footprint of human development in the lower Kenai River watershed based on analysis of aerial photographs. Inset in top panel shows location within entire Kenai River watershed (area within black line). Most development is concentrated in the lowlands near the river main stem and tributaries. An interactive slider graphic showing greater detail is available at https://ak-nsf-epscor.github.io/kenai-change (July 2017).
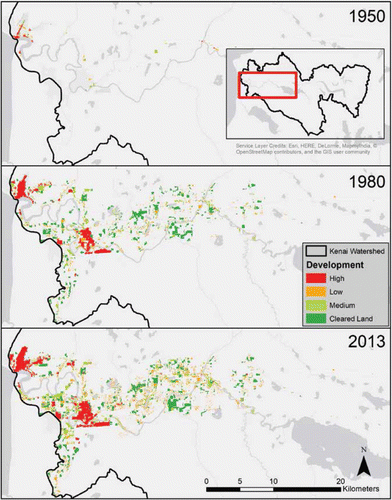
Human development has contributed to the introduction and dispersal of at least 109 nonnative flora (CitationACCS 2017) and 30 nonnative fauna (CitationUSFWS 2016) on the Kenai Peninsula, surprisingly high numbers considering the relative remoteness and isolation of Alaska versus other parts of North America. Several of these species can cause injury to salmonids or their habitats and have prompted rapid management responses: statewide banning of felt-soled waders in 2012 to control the spread of didymo or rock snot Didymosphenia geminata and whirling disease Myxobolus cerebralis (CitationArsan and Bartholomew 2008), the ongoing use of herbicides to eradicate the aquarium plant Elodea from lakes on the peninsula (CitationMorton et al. 2014), and the ongoing use of rotenone to eradicate Northern Pike Esox lucius from the peninsula (CitationDunker et al. 2016). Other introduced organisms have the potential to be novel ecosystem drivers: green alder sawfly Monsoma pulveratum, which defoliates shade-producing thinleaf alder Alnus tenuifolia along the banks of nonglacial streams; white sweetclover Melilotus alba, a nitrogen fixer that can displace native flora on gravel bars; softshell clam Mya arenaria, which competes with native shellfish in the Cook Inlet; and earthworms, which alter soil nutrient dynamics (CitationSaltmarsh et al. 2016) and provide a new food resource for salmonids (D. J. Rinella, unpublished data). Under rapid climate change, ecologists expect novel species assemblages to develop unlike any found today (CitationWilliams and Jackson 2007). Novel assemblages on the Kenai Peninsula may be based not on a simple reshuffling of native boreal species but of human-dispersed species from the Old World and temperate North America (Morton et al., in press). We address several other impacts of human development below in the Conservation and Management section.
SALMON POPULATION TRENDS: LINKS TO CLIMATE AND LANDSCAPE
During the past decade, the numbers of both wild and hatchery-origin salmon have reached historic high levels in the North Pacific (CitationIrvine and Ruggerone 2016). Overall, the numbers of Pink Salmon O. gorbuscha, Chum Salmon O. keta, and Sockeye Salmon have recently increased, due to a combination of generally favorable climatic conditions in the ocean and increased hatchery production, whereas Chinook and Coho salmon populations have decreased (CitationIrvine and Fukuwaka 2011; CitationIrvine and Ruggerone 2016). Similar trends hold in the Gulf of Alaska region, which produces roughly one-third of the world's wild Pacific salmon (CitationIrvine and Ruggerone 2016). Hatchery salmon production in the region has recently reached all-time highs of roughly 1.5 billion (1.5 × 109) salmon released per year, mostly in Prince William Sound and Southeast Alaska (CitationStopha 2017).
In the Kenai River, Sockeye Salmon have been relatively abundant during the past decade, whereas Chinook Salmon runs have declined. Total run sizes have fluctuated 10-fold for Sockeye Salmon and 7-fold for Chinook Salmon during the 30- to 40-year period of record (). These numbers include fish that were harvested as well as those that escaped the fisheries to spawn. Hatchery supplementation produces less than 2% of the Sockeye Salmon and none of the Chinook Salmon returning to the Kenai River (CitationTobias and Willette 2013). The average run size of Sockeye Salmon was slightly above the 40-year average during 2006–2015, the 10 most recent years with available data (CitationTobias and Willette 2013; CitationMcCarthy 2016). In contrast, Chinook Salmon populations declined sharply in the Kenai and many other Alaska rivers during the past decade (CitationADFG 2013). Two distinct populations of Chinook Salmon return to the Kenai River (Box 1; CitationBurger et al. 1985). In recent years, adult returns from both populations have declined to the lowest levels in the 30-year record (; CitationFleischman and Reimer 2017). The mean body size of adult Chinook Salmon has also declined substantially since the 1980s in many Alaska rivers, including the Kenai, due to slower growth rates and earlier maturity (CitationLewis et al. 2015), raising concerns about recruitment and productivity. Although the specific mechanisms driving these changes are poorly understood, they are linked to climate and landscape change in at least two key ways.
Figure 8. Returns (total run size: escapement + harvest) of adult Chinook and Sockeye salmon to the Kenai River and a major tributary, the Russian River (data from Alaska Department of Fish and Game). Returns are plotted separately for populations or runs of each species that differ in spawning habitat and timing. The early runs of each species spawn in tributaries not influenced by lakes or glaciers (N). The late runs spawn predominantly in tributaries influenced by lakes (L) or in the main stem influenced by glaciers and lakes (G + L). Returns have varied by roughly an order of magnitude (7- to 13-fold) over the period of record for all five runs (note the log-scaled y-axis). Chinook Salmon returns data exclude small fish <75 cm in length from mid-eye to tail fork, which cannot be distinguished from Sockeye Salmon on imaging sonar (CitationFleischman and Reimer 2017).
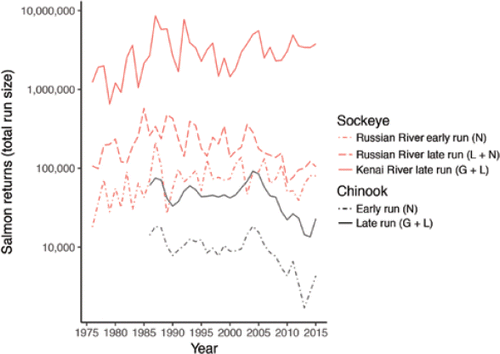
First, salmon populations have fluctuated widely over past decades and centuries in association with large-scale climatic oscillations. For example, decadal-scale cycles in Chinook and Coho salmon productivity in North America, including the recent downturn, are associated with an indicator of marine climatic conditions, the North Pacific Gyre Oscillation (CitationKilduff et al. 2015; CitationOhlberger et al. 2016). On regional and local scales, Chinook Salmon productivity is also associated with changes in the freshwater environment, such as summer stream discharge (CitationNeuswanger et al. 2015) and the timing of spring river ice breakup (CitationOhlberger et al. 2016). Reaching further back in time, lake sediment records reveal that Sockeye Salmon populations in Bristol Bay and the Gulf of Alaska have cycled between high- and low-productivity periods lasting 100 years or more over the past 500 years, with different populations rising and falling asynchronously until the onset of commercial fishing (CitationRogers et al. 2013). The longest available paleolimnological record for Alaska salmon, from the Russian River drainage, suggests that Sockeye Salmon were generally abundant over the past 3,700 years but nearly absent during a 600-year period beginning in 50 BCE (CitationMcCarthy 2016).
Second, diverse, intact landscapes enhance the stability of salmon returns. Individual salmon populations respond in different ways to regional climate patterns, potentially because they rely on different types of freshwater habitats (CitationRogers and Schindler 2008). This asynchrony among populations stabilizes the dynamics of salmon returns across broader geographic regions, delivering more reliable benefits to fisheries and ecosystems via the portfolio effect (CitationHilborn et al. 2003; CitationSchindler et al. 2010). Alaska salmon populations are more asynchronous, and thus provide more reliable portfolios, than salmon at lower latitudes where habitats are more heavily impacted and homogenized by human development and dams (CitationMoore et al. 2010; CitationGriffiths et al. 2014).
Habitat diversity also increases the benefits of salmon runs to ecosystems and fisheries by causing diversity in the timing of their spawning migrations. In diverse, intact landscapes, the peak migration timing of the same species in nearby streams can vary by 3 weeks or more due in part to temperature differences (Box 1; CitationLisi et al. 2013). This variability allows trout, bears, and gulls to eat salmon and salmon eggs for months rather than weeks by moving progressively from colder to warmer streams (CitationRuff et al. 2011; CitationSchindler et al. 2013; CitationDeacy et al. 2016). Diversity in migration timing can also extend fishing seasons and improve food security for humans (CitationNesbitt and Moore 2016). In the Kenai River watershed, Sockeye Salmon return to the Russian River in two runs: an early run returns in early June to spawn in cold water upstream of two rearing lakes and a late run returns in July to spawn throughout the drainage, predominantly in warmer water downstream from the lakes. In part due to this extended window of fishing opportunity, the Russian River supports one of the most popular sport fisheries in Alaska (CitationBegich et al. 2013). This vigorous 3-mile (∼5 km) fishery at times draws more than 1,000 anglers per day in elbow-to-elbow “combat fishing” conditions (; CitationPawluk 2015).
Figure 9. Trends in harvest and effort in the (A) commercial gill-net, (B) recreational hook-and-line, and (C) personal-use dip-net and gill-net fisheries on the northern Kenai Peninsula. Commercial fisheries harvest more salmon than the other fisheries combined (note different y-axis scales), but the sport and personal-use fisheries have grown substantially. Commercial harvest includes central district drift gill-net and east-side set gill-net fisheries. “Other” harvest includes Rainbow Trout Oncorhynchus mykiss, Dolly Varden Salvelinus malma, Arctic Grayling Thymallus arcticus, and Northern Pike Esox lucius. Sources: Alaska Department of Fish and Game and Alaska Commercial Fisheries Entry Commission. Photo credits (top to bottom): Madeline Jovanovich, Ron Neibrugge (via Creative Commons), jodyo.photos.
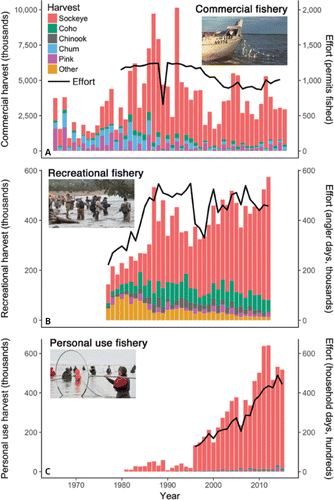
Box 1. Glaciers + Lakes = High Salmon Productivity?
Box 1. Rivers flowing into the Gulf of Alaska are strongly influenced by glaciers. Roughly 7% of freshwater discharge into the Gulf of Alaska is meltwater from rapidly thinning glaciers (CitationHill et al. 2015). In general, glacial rivers support low productivity of algae, invertebrates, and fish due to their low bed stability, high sediment loads, and cold temperatures (CitationMilner et al. 2001). However, proglacial lakes moderate these harsh physical conditions by stabilizing flow regimes, trapping sediment, and buffering temperatures (CitationDorava and Milner 2000; CitationKyle and Brabets 2001; see below).
Many rivers below proglacial lakes support highly productive salmon populations, including those in the Kenai, Kasilof, and Klutina rivers in southcentral Alaska. CitationDorava and Milner (2000) proposed that lake-mediated glacial rivers produce more salmon per unit channel length than glacial or nonglacial rivers lacking lake influence, citing examples in the Cook Inlet basin. Lake-regulated rivers also exhibit diversity in salmon run timing, prolonging the availability of salmon to fisheries and ecosystems. Many lake-regulated rivers support two genetically distinct populations of Chinook and Sockeye salmon: early runs spawning in tributaries and late runs spawning in the main stem below the lake (e.g., Burger et al. 1986, Citation1997; CitationSavereide 2005).
The distribution of proglacial lakes is changing as glaciers recede. For example, a large new proglacial lake formed at the toe of the Melbern Glacier in the Alsek River drainage, YT, Canada, ca. 2005. Melbern Lake and its outlet river are now developing characteristics suitable for colonization by Chinook and Sockeye salmon, which could ultimately be harvested in U.S. fisheries (A. von Finster, Fisheries and Oceans Canada, retired, pers. comm.). Conversely, in 2016, Kluane Lake, a major proglacial lake in the Yukon River basin, lost its main glacial tributary when the Kaskawulsh Glacier receded to the point that its drainage flowed south towards the Pacific Ocean instead of north into the lake (CitationShugar et al. 2017). The loss of glacial inflow may transform the ecology of the lake and its salmon-bearing outlet river.
Photo credits (L-R): Marli Miller, University of Oregon; John Schoen; National Weather Service.
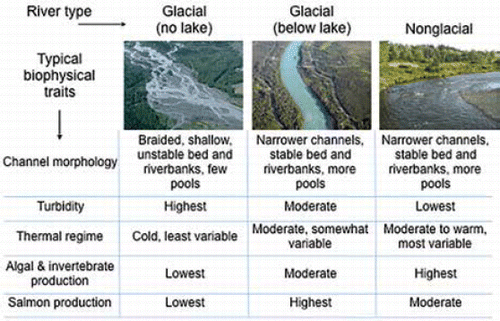
SALMON FISHERIES: RECENT TRENDS AND RESILIENCE TO CHANGE
Salmon are a tremendously valuable resource, providing food, employment, and cultural value to Alaska residents and visitors. We estimate that commercial and recreational fisheries for Alaska salmon support over US$1 billion (1 × 109) in combined annual economic output, based on recent economic studies (see Supplemental Information). Beyond its monetary value, salmon fishing is a treasured annual tradition for a broad cross section of Alaskans (CitationLord 2016). The Kenai River has historically produced 35% of the salmon commercially harvested in the Cook Inlet basin, including 80% of the Sockeye Salmon, despite occupying less than 6% of the basin area (CitationDorava and Milner 2000). The Kenai River also supports world-famous recreational fisheries. In recent years, these have included Alaska's largest sport fisheries for Chinook, Coho, and Sockeye salmon as well as Rainbow Trout O. mykiss (CitationBegich et al. 2013). Together these species support hundreds of thousands of days of fishing effort by guided as well as nonguided anglers. Kenai River salmon are also harvested in personal-use fisheries, which provide Alaska residents an opportunity to efficiently “fill their freezers” for their annual sustenance via dip nets or gill nets, as well as in small-scale subsistence and educational fisheries (CitationFall et al. 2015). Commercial fisheries harvest more northern Kenai Peninsula salmon than the other fisheries combined, but the recreational and personal-use fisheries have grown substantially in recent decades (; see Supplemental Information).
Despite the high value of Alaska salmon, the inherent variability of their returns can make fishing an economically risky business. Some fishers and fishing communities mitigate these risks and smooth their incomes by targeting a diverse portfolio of species and stocks (CitationKasperski and Holland 2013). However, fishing communities differ in opportunities for diversifying their fishery portfolios due to their proximity to commercial fisheries and fleet characteristics that promote or constrain participation in diversified fisheries. In general, communities along the Gulf of Alaska have more diverse fishing portfolios than those in western and interior Alaska (CitationSethi et al. 2014; CitationCline et al. 2017).
Commercial fisheries in Cook Inlet harvest salmon returning to the Kenai River and neighboring drainages using two types of fishing gear: drift and set gill nets (CitationShields and Dupuis 2016). Both fisheries are limited entry programs with tradable permits (CitationGho 2014). Encouragingly, residents of the Kenai Peninsula Borough own most of these permits and have maintained these permit holdings over time (see Supplemental Information), contrary to a state-wide trend of diminishing local control of fishing permits (CitationKnapp 2011). Further, Kenai Peninsula residents participate in many other commercial fisheries, providing a diverse revenue base at the regional scale (Supplemental Information: Figure S3). However, some communities on the peninsula (e.g., Kenai and Soldotna) have become increasingly reliant on fewer fisheries over the past 35 years, as measured by applying CitationSimpson's (1949) diversity index to permit holdings data (). These communities have experienced more variability in commercial fishery revenues over time, relative to other communities that have maintained a more stable and diversified portfolio of permits (e.g., Homer and Anchor Point, ). The concentration of permit holdings on fewer types of fisheries may limit the capacity of these communities to adapt to changing patterns of productivity and withstand future cyclical downturns in harvest or market prices.
Figure 10. Concentration of (A) fishery permits and (B) variability in fishery revenues for selected communities of the Kenai Peninsula Borough (source: Alaska Commercial Fisheries Entry Commission). Fisheries concentration is quantified using Simpson's diversity index, with higher values indicating more concentration (less diversity). The coefficient of variation measures the SD (relative to the mean) of communities' fishery revenue over time (1980–2014).
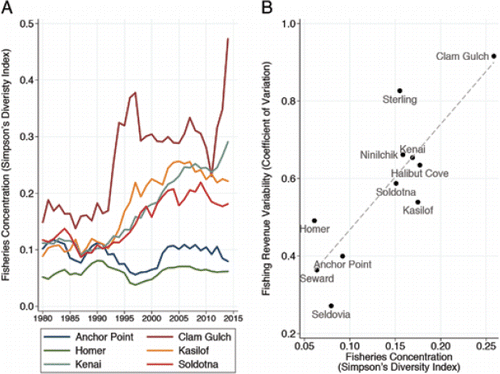
Although harvesting a diversity of resources generally stabilizes revenues and enhances resilience, it can also create challenges for management, particularly when fisheries capture multiple species or stocks at the same time and place. In these mixed fisheries, managers often must choose between restricting harvest of abundant stocks or overfishing weak stocks; thus, total harvest can be limited by the weakest component (CitationNRC 1996; CitationKnudsen 2000; CitationWalters and Martell 2004). Due to the overlap in run timing and migration routes of the five salmon species present in Cook Inlet, the commercial salmon fisheries are mixed fisheries, in terms of both species and stocks. Fishery managers are charged with meeting their primary biological objectives (e.g., spawning escapement goals) as well as social objectives (e.g., sport and personal-use fishing allocations) for multiple species simultaneously (CitationShields and Dupuis 2016). This has resulted in a complicated and contentious network of regulations for both commercial and recreational fisheries, designed to allow harvest of abundant Sockeye Salmon while minimizing impacts to weak Chinook Salmon runs. During recent poor return years, managers have closed the set gill-net and sport fisheries (CitationLoring 2016). To enhance the resilience of this complex and valuable system, an important additional challenge for policymakers is to promote entry opportunities to fisheries and encourage economic diversification, potentially outside of commercial fishing (CitationSethi et al. 2014).
CONSERVATION AND MANAGEMENT IN THE FACE OF ENVIRONMENTAL CHANGE
Existing research documenting the responses of fish to climate change largely focuses on shifts in distribution (CitationLynch et al. 2016), emphasizing the leading and trailing edges of species' climate envelopes. In contrast, we synthesized changes occurring within the highly productive core of the worldwide distribution of wild Pacific salmon (CitationAugerot and Foley 2005; CitationIrvine and Fukuwaka 2011). Despite the high economic and cultural value of salmon in the Gulf of Alaska region and a growing number of prospective modeling studies (e.g., CitationLeppi et al. 2014; CitationWobus et al. 2016; CitationSloat et al. 2017), relatively few observed responses to climate change have yet been documented in this region (CitationLynch et al. 2016). These responses include colonization of newly deglaciated habitats (CitationMilner et al. 2000), changes in juvenile growth (CitationEdmundson et al. 2003), and altered migration timing (CitationKovach et al. 2015). The net effects of these myriad changes on ecosystem dynamics and salmon productivity may be too complex to predict with mechanistic models (CitationSchindler and Hilborn 2015), and scientists and policymakers should expect unprecedented future conditions to produce ecological surprises (CitationWilliams and Jackson 2007). The changing environment will likely affect salmon species, populations, and life history variants differently, producing winners and losers and potentially driving a redistribution of productive salmon runs and profitable fisheries across the region. To manage ecosystems in the face of such uncertainty, research suggests applying multiple strategies, such as the restoration of degraded habitats, adding vulnerable habitats to conservation networks, facilitating species movements into new habitats, or transforming parts of the ecosystem into novel forms to provide more desired benefits to society (e.g., CitationMillar et al. 2007; CitationAplet and McKinley 2017).
Riparian lowlands emerge as a key priority for restoration and conservation throughout the region because of their high value as salmon habitat, sensitivity to environmental change, exposure to human development, and underrepresentation within conservation networks. On the Kenai Peninsula, lowland streams fed by groundwater and wetlands are considered the most vulnerable category of fish habitat, facing threats from a warming climate, invasive species, riparian development, and road crossings lacking adequate fish passage (CitationKPFHP 2014). The lower mainstem Kenai River appears to be buffered from some climatic changes by upstream glaciers and lakes (Box 1), but it is also impacted by riparian development () and other human activities. The lower main stem is a crucial spawning and rearing habitat for late-run Chinook Salmon and a migration corridor for all Pacific salmon species. Lowland salmon habitats in other parts of Alaska face similar challenges (CitationSepulveda et al. 2015; CitationMatanuska–Susitna Basin Salmon Habitat Partnership 2016).
Lowland streams are also vulnerable in less populated parts of the Gulf of Alaska region, including the temperate rainforests of Southeast Alaska. Although only 12% of the productive old-growth forest in Southeast Alaska has been harvested, disproportionate logging in valley bottoms and floodplains has reduced forest cover with the highest volume of contiguous old growth by 67% (CitationAlbert and Schoen 2013). These lowland habitats have high potential for salmon productivity (CitationEdwards et al. 2013; CitationSloat et al. 2017). Many higher-elevation streams remain more pristine, but these are generally less productive habitats due to higher gradients, confined channels, and a lack of floodplain connectivity (CitationSloat et al. 2017). Further, several rivers with important salmon runs are vulnerable to large proposed mines, both in Alaska (e.g., CitationLeppi et al. 2014; CitationWobus et al. 2016) and upstream on transboundary rivers in Canada (e.g., CitationScannell 2012). Riparian lowlands attract human development worldwide (CitationCarpenter et al. 1992), and many of the world's formerly productive lowland salmon streams are now highly degraded (CitationMontgomery 2003). However, much of Alaska remains unmodified by permanent development (CitationTrammell and Aisu 2015; CitationCrawford et al. 2016), and it is not too late to conserve the lowland component of the habitat portfolio in many salmon-bearing watersheds.
Conversely, salmon are colonizing new habitats following glacial loss and retreat, potentially allowing for productivity gains in some watersheds. Many rivers in the region receive too much glacial runoff to support salmon. For example, rearing habitat for juvenile Chinook Salmon is only available in a small fraction of the Copper River watershed, in subbasins with ≤10% glacier coverage (CitationBidlack et al. 2014). New proglacial lakes (e.g., ; Box 1) may provide particularly valuable opportunities for salmon productivity gains (CitationMilner et al. 2007; CitationO'Neel et al. 2015). Salmon will readily colonize watersheds following glacial recession when hydroecological conditions become suitable (CitationMilner et al. 2000), so to facilitate these movements, managers may need only to conserve glacial habitats that are likely to become more productive in the future. For example, several subbasins in the upper Kenai River watershed could potentially produce more salmon in the future, including the Snow River, which has a wide floodplain with ample low-gradient habitat suitable for anadromous fish but where high (30%) glacier coverage currently contributes to channel instability (CitationMacFarlane 2005). A hydropower dam has recently been proposed on the Snow River, and we suggest that the impact of such a project on salmon production might be much greater under future conditions than it appears at present.
Salmon hatcheries are an example of a strategy attempting to transform the ecosystem into a novel form to provide greater benefits (i.e., enhanced fish harvests) to society, but record-high hatchery production poses risks to wild salmon. Hatchery-origin salmon accounted for one-third of the salmon commercially harvested in Alaska over the past decade (CitationStopha 2017). Most hatchery salmon return to Prince William Sound (56%), Southeast Alaska (36%), and Kodiak (11%), whereas only 2% return to Cook Inlet (CitationStopha 2017). Alaska hatchery operations are regulated to minimize impacts on wild populations, and wild runs have increased along with hatchery production since the 1970s (CitationStopha 2017). However, wild Pink Salmon production has increased far less in Prince William Sound than in other regions of Alaska lacking large hatcheries, suggesting a tradeoff between hatchery and wild production (CitationAmoroso et al. 2017). Further, recent studies have documented substantial proportions of strayed hatchery-origin fish on wild spawning grounds (CitationBrenner et al. 2012; CitationHabicht et al. 2013), which could reduce dissolved oxygen levels to lethal limits when streamflows are low (CitationSergeant et al. 2017); genetic introgression into wild populations (CitationJasper et al. 2013), which can reduce the fitness of wild salmon (CitationAraki et al. 2007); and competition between hatchery-origin and wild fish in the ocean, which reduces their growth rates (e.g., CitationCross et al. 2005; CitationRuggerone et al. 2016).
Climate and landscape change are likely to intensify some of the interactions between hatchery-origin and wild salmon. Low streamflows driven by climate change or human-caused water diversions increase the risk of hypoxia, making wild spawners more sensitive to the density of hatchery-origin fish (CitationSergeant et al. 2017). As the ocean warms during this century, Chinook and Sockeye salmon are projected to face greater metabolic constraints than other salmon species (CitationAbdul-Aziz et al. 2011), and these stresses could be exacerbated by greater competition with hatchery-enhanced Pink Salmon populations. Large-scale hatchery releases involve important policy tradeoffs, including potential reductions in the resilience of wild salmon to climate and landscape changes.
MITIGATING THE IMPACTS OF GLOBAL CHANGE WITH LOCAL ACTION
In many cases, the risks facing Alaska salmon can be mitigated by quantifying tradeoffs and adjusting management strategies. One notable success story is the reduction of hydrocarbon pollution in the Kenai River. Petroleum hydrocarbons and metals in urban runoff can cause high mortality of adult salmon before they are able to spawn (CitationScholz et al. 2011; CitationSpromberg et al. 2016). During the 1990s and early 2000s, the sport fishery for Chinook Salmon attracted more than 700 boats per day to the lower Kenai River during July, and petroleum hydrocarbon concentrations exceeded state clean water standards due to discharge from older, carbureted two-stroke outboard motors. The lower river is a major spawning and rearing area for Chinook Salmon and a migration corridor for other Pacific salmon species, making it particularly sensitive to pollution. In response, a buy-back program was initiated for the most polluting motors, these motors were prohibited on the lower river beginning in 2008, and hydrocarbon levels dropped dramatically (CitationOasis Environmental 2010).
Other risks have proven more challenging to mitigate, but governmental agencies and nongovernmental organizations (NGOs) are making progress with coordinated action. To mitigate the impacts of invasive species, the highest priority conservation concern in local freshwater ecosystems (CitationKPFHP 2014), management agencies have taken several aggressive steps documented previously. Research from Oregon suggests that climate change impacts to salmon can be mitigated in part by restoration of riparian vegetation (CitationJustice et al. 2017) and the availability of coldwater refuge habitats fed by subsurface flow (CitationTorgersen et al. 1999). To protect riparian vegetation, the Kenai Peninsula Borough restricted new development within 50 ft (15 m) of salmon-bearing waters (CitationCzarnezki and Yaeger 2014). This riparian buffer regulation was recently upheld by local ballot initiative, demonstrating strong popular support for river conservation. In addition, public and private landowners have now preserved or restored more than 20% of the land parcels bordering the river (CitationArendt et al. 2010). To conserve coldwater refugia in Kenai Peninsula salmon streams, Cook Inletkeeper, a local NGO, uses thermal imagery to identify priority sites and works with land trusts to protect them (CitationMauger et al. 2015). Road crossings lacking adequate fish passage are also a high priority for restoration because large areas of disconnected habitat can be restored at relatively low cost. During the late 2000s, only 18% of assessed road culverts on the Kenai Peninsula provided adequate anadromous fish passage, but several agencies and the Kenai Watershed Forum NGO are now replacing problematic culverts and other stream crossings (CitationArendt et al. 2010).
More than a dozen federal, state, and local agencies have some degree of jurisdiction over the Kenai River fisheries or habitat supporting the fisheries. Additionally, more than a dozen NGOs actively exert influence over management of the fisheries (CitationKrupa 2016). Both collaboration and conflict abound among these diverse stakeholders (CitationHarrison and Loring 2014). Changes in allocation, closures of specific fisheries, and habitat protection measures that restrict development are frequent sources of conflict. Considering the importance of these fisheries, it is not surprising that local elected officials and NGO leaders share a similarly high level of local knowledge about the fisheries as the professional fishery managers (J. E. Powell and colleagues, unpublished data). In a scenario assessment, area resource managers identified climate change, economic development, changing marine conditions, and local human population growth as key threats to salmon abundance (E. J. Trammell and colleagues, unpublished data). Additionally, they raised local issues related to allocation, specifically whether the recreational and personal-use fisheries would continue to grow and whether participation in the personal-use fishery would continue and/or increase, as important uncertainties in the future of salmon management on the Kenai River.
In order to design effective local conservation measures, which primarily affect freshwater habitats, it is important to understand how freshwater life stages affect salmon population productivity. In general, high-quality freshwater habitat is necessary but not sufficient for highly productive salmon runs, because a substantial and variable fraction of salmon lifecycle mortality occurs in the ocean (CitationBradford 1995; CitationQuinn 2005). To separately quantify freshwater and marine survival rates, researchers must monitor juvenile salmon abundance—for example, by counting smolts during their spring outmigration to the ocean—in addition to counting adults during their return spawning migration. Smolt counts are logistically challenging in Alaska due to river ice, and only a few wild stocks have been monitored, mostly in Southeast Alaska. After identifying juvenile monitoring as a critical knowledge gap in addressing the statewide decline of Chinook Salmon, the Alaska Department of Fish and Game recently expanded these programs to the Copper River and other systems (CitationADFG 2013). If maintained over the long term, these data sets could be extremely valuable in teasing out specific factors in the freshwater and marine environments that influence salmon production.
CONCLUSIONS
Salmon productivity is not stable over time but cyclical, as shown by decades of fish counts, a century of commercial harvest, and lake sediment records dating back for millennia. Salmon have recovered from massive climatic and landscape disturbances in the past, including glaciations, wildfires, and floods (CitationAugerot and Foley 2005; CitationWaples et al. 2008). However, human development and habitat degradation have proven overwhelming in much of the Atlantic basin and Pacific Northwest, where wild salmon abundance today is a tiny fraction of historic levels (CitationNRC 1996; CitationLenders et al. 2016; CitationGibson 2017). Future trends will not necessarily resemble the past, especially as climate change and ocean acidification alter the environment in unprecedented ways, with unknown and perhaps surprising outcomes (CitationWilliams and Jackson 2007; CitationFabry et al. 2008). Policymakers and fishing communities would be prudent to maintain their flexibility in preparation for further large-scale shifts in salmon productivity (CitationCline et al. 2017). Encouragingly, many important factors influencing the long-term sustainability of salmon populations are under local control, such as restoration efforts guided by knowledge of historical conditions, enforcement of protections critical to salmon habitat, and coordination among managers and stakeholders (CitationMontgomery 2003). An important question for managers is whether current policies and land use patterns sufficiently protect the habitats that will be necessary to support resilient salmon runs in a warmer world, which could differ from the most productive habitats today. Maintaining and, when possible, restoring a diversity of options may allow wild salmon and fishing communities alike to continue to thrive amidst the challenges and opportunities posed by climate and landscape change.
SUPPLEMENTARY MATERIALS
Additional supplementary materials are available online. can be downloaded at http://www.alaska.edu/epscor/pdfs/Salmon-Ecosystems.pdf. All data used in this article are freely available from the Alaska EPSCoR Central Portal (epscor.alaska.edu).
REFERENCES
All references for the article are available as supplementary materials on the publisher's website at http://tandfonline.com/doi/full/10.1080/03632415.2017.1374251
SPECIAL NOTE ABOUT THIS SUBMISSION
This issue of Fisheries has been structured around this Schoen et al. Feature, which is one of the longer and more complex manuscripts to ever appear in Fisheries. Fisheries published it in its entirety because it illustrates the broad range of complexity and uncertainty about climate change from both ecological and human perspectives. Although it focuses on Alaska salmon in a particular river, we believe that fisheries scientists confronting climate change will find it useful as an example of a landscape-level approach on this topic.
ufsh_a_1374251_sm3043.zip
Download Zip (803.8 KB)ACKNOWLEDGMENTS
We thank Robert Begich, Edward Berg, Branden Bornemann, Jeff Falke, Steve Fleischman, Rudiger Gens, Shad O'Neel, Robert Ruffner, Daniel Schindler, Al von Finster, and Alaska EPSCoR program researchers for providing data, field and lab assistance, and valuable discussions that improved this article. We also thank Dixon Jones for producing . Peter Westley and four anonymous reviewers provided valuable feedback on the article. The findings and conclusions in this article are those of the authors and do not necessarily represent the views of the U.S. Fish and Wildlife Service. This study was funded by Alaska EPSCoR National Science Foundation award #OIA-1208927 with matching funds from the state of Alaska.
REFERENCES
- Abdul-Aziz, O. I., N. J. Mantua, and K. W. Myers. 2011. Potential climate change impacts on thermal habitats of Pacific Salmon (Oncorhynchus spp.) in the North Pacific Ocean and adjacent seas. Canadian Journal of Fisheries and Aquatic Sciences 68 (9): 1660–1680.
- ADFG (Alaska Department of Fish and Game). 2013. Chinook Salmon stock assessment and research plan. Alaska Department of Fish and Game, Anchorage.
- ADFG (Alaska Department of Fish and Game). 2017. Anadromous waters catalog. Available: http://adfg.alaska.gov/sf/SARR/AWC. (July 2017).
- Alaska Center for Conservation Science. 2017. Alaska exotic plants information clearinghouse (AKEPIC). Available: http://accs.uaa.alaska.edu/invasive-species/non-native-plants. (July 2017).
- Alaska Department of Labor and Workforce Development. 2016. 2015 Population estimates by borough, census area, and economic region. Available: http://live.laborstats.alaska.gov/pop/index.cfm7. (July 2017).
- Alaska EPSCoR. 2017. Environmental change on the Kenai Peninsula from 1950 to 2013. Available: https://ak-nsf-epscor.github.io/kenaichange. (August 2017).
- Albert, D. M., and J. W. Schoen. 2013. Use of historical logging patterns to identify disproportionately logged ecosystems within temperate rainforests of southeastern Alaska. Conservation Biology 27 (4): 774–784.
- Allan, J. D., M. S. Wipfli, J. P. Caouette, A. Prussian, and J. Rodgers. 2003. Influence of streamside vegetation on inputs of terrestrial invertebrates to salmonid food webs. Canadian Journal of Fisheries and Aquatic Sciences 60 (3): 309–320.
- Amoroso, R. O., M. D. Tillotson, and R. Hilborn. 2017. Measuring the net biological impact of fisheries enhancement: Pink Salmon hatcheries can increase yield, but with apparent costs to wild populations. Canadian Journal of Fisheries and Aquatic Sciences 74 (8): 1233–1242.
- Aplet, G. H., and P. S. McKinley. 2017. A portfolio approach to managing ecological risks of global change. Ecosystem Health and Sustainability 3 (2): e01261.
- Araki, H., B. Cooper, and M. S. Blouin. 2007. Genetic effects of captive breeding cause a rapid, cumulative fitness decline in the wild. Science 318 (5847): 100–103.
- Arendt, A. A., K. A. Echelmeyer, W. D. Harrison, C. S. Lingle, and V. B. Valentine. 2002. Rapid wastage of Alaska glaciers and their contribution to rising sea level. Science 297 (5580): 382–386.
- Arendt, K., R. Ericksen, R. Beamesderfer, and G. Johnston. 2010. Kenai River habitat assessment. Report to the Alaska Department of Fish and Game and Kenai River Sport Fishing Association. Cramer Fish Sciences and Inter-fluve. Available: http://www.fishsciences.net/reports/2010/Kenai_Habitat_Report_Review_2010.pdf. (July 2017).
- Arismendi, I., M. Safeeq, J. B. Dunham, and S. L. Johnson. 2014. Can air temperature be used to project influences of climate change on stream temperature? Environmental Research Letters 9 (8): 084015.
- Arsan, E. L., and J. L. Bartholomew. 2008. Potential for dissemination of the nonnative salmonid parasite Myxobolus cerebralis in Alaska. Journal of Aquatic Animal Health 20 (3): 136–149.
- Augerot, X., and D. N. Foley. 2005. Atlas of Pacific Salmon: the first map-based status assessment of salmon in the north Pacific. University of California Press, Berkeley.
- Bauret, S., and S. L. Stuefer. 2013. Kenai Peninsula precipitation and air temperature trend analysis. Pages 35–44 in S. L. Stuefer, and W. R. Bolton, editors. 19th International Northern Research Basins Symposium and Workshop, Southcentral Alaska, University of Alaska Fairbanks.
- Begich, R. N., J. A. Pawluk, J. L. Cope, and S. Simons. 2013. 2010–2012 Annual management report and 2013 recreational fisheries overview for northern Kenai Peninsula: fisheries under consideration by the Alaska Board of Fisheries, 2014. Alaska Deparment of Fish and Game, Anchorage.
- Berg, E. E., and R. S. Anderson. 2006. Fire history of white and Lutz spruce forests on the Kenai Peninsula, Alaska, over the last two millennia as determined from soil charcoal. Forest Ecology and Management 227 (3): 275–283.
- Berg, E. E., J. D. Henry, C. L. Fastie, A. D. De Volder, and S. M. Matsuoka. 2006. Spruce beetle outbreaks on the Kenai Peninsula, Alaska, and Kluane National Park and Reserve, Yukon Territory: relationship to summer temperatures and regional differences in disturbance regimes. Forest Ecology and Management 227 (3): 219–232.
- Berg, E. E., K. M. Hillman, R. Dial, and A. DeRuwe. 2009. Recent woody invasion of wetlands on the Kenai Peninsula Lowlands, south-central Alaska: a major regime shift after 18,000 years of wet Sphagnum–sedge peat recruitment. Canadian Journal of Forest Research 39 (11): 2033–2046.
- Bidlack, A. L., L. E. Benda, T. Miewald, G. H. Reeves, and G. McMahan. 2014. Identifying suitable habitat for Chinook Salmon across a large, glaciated watershed. Transactions of the American Fisheries Society 143 (3): 689–699.
- Bliss, A., R. Hock, and V. Radić. 2014. Global response of glacier runoff to twenty-first century climate change. Journal of Geophysical Research: Earth Surface 119 (4): 717–730.
- Bradford, M. J. 1995. Comparative review of Pacific Salmon survival rates. Canadian Journal of Fisheries and Aquatic Sciences 52 (6): 1327–1338.
- Brenner, R. E., S. D. Moffitt, and W. S. Grant. 2012. Straying of hatchery salmon in Prince William Sound, Alaska. Environmental Biology of Fishes 94 (1): 179–195.
- Burger, C. V., W. J. Spearman, and M. A. Cronin. 1997. Genetic differentiation of Sockeye Salmon subpopulations from a geologically young Alaskan lake system. Transactions of the American Fisheries Society 126 (6): 926–938.
- Burger, C. V., R. L. Wilmot, and D. B. Wangaard. 1985. Comparison of spawning areas and times for 2 runs of Chinook Salmon (Oncorhynchus tshawytscha) in the Kenai River, Alaska. Canadian Journal of Fisheries and Aquatic Sciences 42 (4): 693–700.
- Carpenter, S. R., S. G. Fisher, N. B. Grimm, and J. F. Kitchell. 1992. Global change and freshwater ecosystems. Annual Review of Ecology and Systematics 23: 119–139.
- Chapin, F. S. I., S. F. Trainor, P. Cochran, H. Huntington, C. J. Markon, M. McCammon, A. D. McGuire, and M. Serreze. 2014. Alaska. Pages 514–536 in J. M. Melillo, T. Richmond, and G. W. Yohe, editors. Climate change impacts in the United States: the third National Climate Assessment. U.S. Global Change Research Program, Washington, D.C.
- Cline, T. J., D. E. Schindler, and R. Hilborn. 2017. Fisheries portfolio diversification and turnover buffer Alaskan fishing communities from abrupt resource and market changes. Nature Communications 8: 14042.
- Crawford, S., G. Whelan, D. M. Infante, K. Blackhart, W. M. Daniel, P. L. Fuller, T. Birdsong, D. J. Wieferich, R. McClees-Funinan, S. M. Stedman, K. Herreman, and P. Ruhl. 2016. Through a fish's eye: the status of fish habitats in the United States 2015. Available: http://assessment.fishhabitat.org. (July 2017).
- Cross, A. D., D. A. Beauchamp, J. L. Armstrong, M. Blikshteyn, J. L. Boldt, N. D. Davis, L. J. Haldorson, J. H. Moss, K. W. Myers, and R. V. Walker. 2005. Consumption demand of juvenile Pink Salmon in Prince William Sound and the coastal Gulf of Alaska in relation to prey biomass. Deep-Sea Research Part II—Topical Studies In Oceanography 52 (1–2): 347–370.
- Czarnezki, J., and J. Yaeger. 2014. On the River: A guide to owning and managing waterfront property on the Kenai Peninsula, 2nd edition. Kenai Peninsula Borough, River Center, Soldotna, Alaska.
- David, A. T., C. A. Simenstad, J. R. Cordell, J. D. Toft, C. S. Ellings, A. Gray, and H. B. Berge. 2016. Wetland loss, juvenile salmon foraging performance, and density dependence in Pacific Northwest estuaries. Estuaries and Coasts 39 (3): 767–780.
- Deacy, W., W. Leacock, J. B. Armstrong, and J. A. Stanford. 2016. Kodiak brown bears surf the salmon red wave: direct evidence from GPS collared individuals. Ecology 97 (5): 1091–1098.
- Dorava, J. M., and A. M. Milner. 2000. Role of lake regulation on glacierfed rivers in enhancing salmon productivity: the Cook Inlet watershed, south-central Alaska, USA. Hydrological Processes 14 (16–17): 3149–3159.
- Dunham, J. B., A. E. Rosenberger, C. H. Luce, and B. E. Rieman. 2007. Influences of wildfire and channel reorganization on spatial and temporal variation in stream temperature and the distribution of fish and amphibians. Ecosystems 10 (2): 335–346.
- Dunker, K. J., A. J. Sepulveda, R. L. Massengill, J. B. Olsen, O. L. Russ, J. K. Wenburg, and A. Antonovich. 2016. Potential of environmental DNA to evaluate Northern Pike (Esox lucius) eradication efforts: an experimental test and case study. PLoS One 11 (9): e0162277.
- Edmundson, J., T. Willette, J. Edmundson, D. Schmidt, S. Carlson, B. Bue, and K. Tarbox. 2003. Sockeye Salmon over escapement (Kenai River component). Alaska Department of Fish and Game, Anchorage.
- Edwards, R. T., D. V. D'Amore, E. Norberg, and F. Biles. 2013. Riparian ecology, climate change, and management in North Pacific coastal rainforests. Pages 43–72 in G. H. Orians and J. W. Schoen, editors. North Pacific temperate rainforests: ecology and conservation. University of Washington Press, Seattle.
- Fabry, V. J., B. A. Seibel, R. A. Feely, and J. C. Orr. 2008. Impacts of ocean acidification on marine fauna and ecosystem processes. ICES Journal of Marine Science 65 (3): 414–432.
- Fall, J. A., C. L. Brown, S. S. Evans, R. A. Grant, H. Ikuta, L. Hutchinson-Scarbrough, B. Jones, M. A. Marchioni, E. Mikow, J. T. Ream, L. A. Sill, and T. Lemons. 2015. Alaska subsistence and personal use salmon fisheries 2013 annual report. Alaska Department of Fish and Game, Division of Subsistence, Anchorage.
- Fellman, J. B., E. Hood, D. V. D'Amore, R. T. Edwards, and D. White. 2009. Seasonal changes in the chemical quality and biodegradability of dissolved organic matter exported from soils to streams in coastal temperate rainforest watersheds. Biogeochemistry 95 (2): 277–293.
- Fellman, J. B., E. Hood, W. Dryer, and S. Pyare. 2015. Stream physical characteristics impact habitat quality for Pacific Salmon in two temperate coastal watersheds. PLoS One 10 (7): e0132652.
- Fleischman, S. J., and A. M. Reimer. 2017. Spawner-recruit analysis and escapement goal recommendations for Kenai River Chinook Salmon. Alaska Department of Fish and Game, Divisions of Sport Fish and Commercial Fisheries, Anchorage.
- Flitcroft, R. L., J. A. Falke, G. H. Reeves, P. F. Hessburg, K. M. McNyset, and L. E. Benda. 2016. Wildfire may increase habitat quality for spring Chinook Salmon in the Wenatchee River subbasin, WA, USA. Forest Ecology and Management 359: 126–140.
- Gho, N. 2014. Overview of permit holdings, harvests, and estimates of gross earnings in the Cook Inlet salmon drift gillnet fishery, 1975–2012. Commercial Fisheries Entry Commission, Juneau, Alaska.
- Gibson, R. J. 2017. Salient needs for conservation of Atlantic Salmon. Fisheries 42: 163–174.
- Gracz, M. B., M. F. Moffett, D. I. Siegel, and P. H. Glaser. 2015. Analyzing peatland discharge to streams in an Alaskan watershed: an integration of end-member mixing analysis and a water balance approach. Journal of Hydrology 530: 667–676.
- Griffiths, J. R., D. E. Schindler, J. B. Armstrong, M. D. Scheuerell, D. C. Whited, R. A. Clark, R. Hilborn, C. A. Holt, S. T. Lindley, J. A. Stanford, and E. C. Volk. 2014. Performance of salmon fishery portfolios across Western North America. Journal of Applied Ecology 51 (6): 1554–1563.
- Habicht, C., T. M. Tobias, G. Fandrei, N. Webber, B. Lewis, and W. S. Grant. 2013. Homing of Sockeye Salmon within Hidden Lake, Alaska, can be used to achieve hatchery management goals. North American Journal of Fisheries Management 33: 777–782.
- Hansen, W. D., F. S. Chapin III, H. T. Naughton, T. S. Rupp, and D. Verbyla. 2016. Forest-landscape structure mediates effects of a spruce bark beetle (Dendroctonus rufipennis) outbreak on subsequent likelihood of burning in Alaskan boreal forest. Forest Ecology and Management 369: 38–46.
- Harrison, H. L., and P. A. Loring. 2014. Larger than life: the emergent nature of conflict in Alaska's Upper Cook Inlet salmon fisheries. SAGE Open 4 (4): 1–14.
- Hilborn, R., T. P. Quinn, D. E. Schindler, and D. E. Rogers. 2003. Biocomplexity and fisheries sustainability. Proceedings of the National Academy of Sciences 100 (11): 6564–6568.
- Hill, D., N. Bruhis, S. Calos, A. Arendt, and J. Beamer. 2015. Spatial and temporal variability of freshwater discharge into the Gulf of Alaska. Journal of Geophysical Research: Oceans 120 (2): 634–646.
- Hood, E., and L. Berner. 2009. Effects of changing glacial coverage on the physical and biogeochemical properties of coastal streams in southeastern Alaska. Journal of Geophysical Research: Biogeosciences 114: G03001.
- Irvine, J. R., and M. Fukuwaka. 2011. Pacific Salmon abundance trends and climate change. ICES Journal of Marine Science 68 (6): 1122–1130.
- Irvine, J. R., and G. T. Ruggerone. 2016. Provisional estimates of numbers and biomass for natural-origin and hatchery-origin Pink, Chum, and Sockeye Salmon in the North Pacific, 1952–2015. North Pacific Anadromous Fish Commission, Vancouver, B.C., Canada.
- Isaak, D. J., S. Wollrab, D. Horan, and G. Chandler. 2011. Climate change effects on stream and river temperatures across the northwest U.S. from 1980–2009 and implications for salmonid fishes. Climatic Change 113 (2): 499–524.
- Jasper, J. R., C. Habicht, S. Moffitt, R. Brenner, J. Marsh, B. Lewis, E. Creelman Fox, Z. Grauvogel, S. D. Rogers Olive, and W. S. Grant. 2013. Source–sink estimates of genetic introgression show influence of hatchery strays on wild Chum Salmon populations in Prince William Sound, Alaska. PLoS One 8 (12): e81916.
- Justice, C., S. M. White, D. A. McCullough, D. S. Graves, and M. R. Blanchard. 2017. Can stream and riparian restoration offset climate change impacts to salmon populations? Journal of Environmental Management 188: 212–227.
- Kane, D. L., and S. L. Stuefer. 2015. Reflecting on the status of precipitation data collection in Alaska: a case study. Hydrology Research 46 (4): 478–493.
- Kasperski, S., and D. S. Holland. 2013. Income diversification and risk for fishermen. Proceedings of the National Academy of Sciences 110 (6): 2076–2081.
- Kenai Peninsula Fish Habitat Partnership. 2014. Strategic plan. Available: http://www.kenaifishpartnership.org/?page_id=303. (July 2017).
- Kilduff, D. P., E. Di Lorenzo, L. W. Botsford, and S. L. H. Teo. 2015. Changing central Pacific El Niños reduce stability of North American salmon survival rates. Proceedings of the National Academy of Sciences 112 (35): 10962–10966.
- Klein, E., E. E. Berg, and R. Dial. 2005. Wetland drying and succession across the Kenai Peninsula Lowlands, south-central Alaska. Canadian Journal of Forest Research 35 (8): 1931–1941.
- Knapp, G. 2011. Local permit ownership in Alaska salmon fisheries. Marine Policy 35 (5): 658–666.
- Knudsen, E. E. 2000. Managing Pacific Salmon escapements: the gaps between theory and reality. Chapter 17 in E. E. Knudsen, C. R. Steward, D. D. MacDonald, J. E. Williams, and D. W. Reiser, editors. Sustainable fisheries management: Pacific Salmon. CRC Press, Boca Raton, Florida.
- Kovach, R. P., S. C. Ellison, S. Pyare, and D. A. Tallmon. 2015. Temporal patterns in adult salmon migration timing across southeast Alaska. Global Change Biology 21 (5): 1821–1833.
- Krupa, M. B. 2016. Who's who in the Kenai River Fishery SES: a streamlined method for stakeholder identification and investment analysis. Marine Policy 71: 194–200.
- Kyle, R. E., and T. P. Brabets. 2001. Water temperature of streams in the Cook Inlet basin, Alaska, and implications of climate change. U.S. Geological Survey, Anchorage, Alaska.
- Lawrence, D. J., B. Stewart-Koster, J. D. Olden, A. S. Ruesch, C. E. Torgersen, J. J. Lawler, D. P. Butcher, and J. K. Crown. 2014. The interactive effects of climate change, riparian management, and a nonnative predator on stream-rearing salmon. Ecological Applications 24 (4): 895–912.
- Le Bris, R., F. Paul, H. Frey, and T. Bolch. 2011. A new satellite-derived glacier inventory for western Alaska. Annals of Glaciology 52 (59): 135–143.
- Lenders, H. J. R., T. P. M. Chamuleau, A. J. Hendriks, R. C. G. M. Lauwerier, R. S. E. W. Leuven, and W. C. E. P. Verberk. 2016. Historical rise of waterpower initiated the collapse of salmon stocks. Scientific Reports 6: 29269.
- Leppi, J. C., D. J. Rinella, R. R. Wilson, and W. M. Loya. 2014. Linking climate change projections for an Alaskan watershed to future Coho Salmon production. Global Change Biology 20 (6): 1808–1820.
- Lewis, B., W. S. Grant, R. E. Brenner, and T. Hamazaki. 2015. Changes in size and age of Chinook Salmon Oncorhynchus tshawytscha returning to Alaska. PLoS One 10 (6): e0130184.
- Lisi, P. J., D. E. Schindler, K. T. Bentley, and G. R. Pess. 2013. Association between geomorphic attributes of watersheds, water temperature, and salmon spawn timing in Alaskan streams. Geomorphology 185: 78–86.
- Lisi, P. J., D. E. Schindler, T. J. Cline, M. D. Scheuerell, and P. B. Walsh. 2015. Watershed geomorphology and snowmelt control stream thermal sensitivity to air temperature. Geophysical Research Letters 42: 3380–3388.
- Lord, N., editor. 2016. Made of salmon: Alaska stories from the Salmon Project. University of Alaska Press, Fairbanks.
- Loring, P. A. 2016. Toward a theory of coexistence in shared social–ecological systems: the case of Cook Inlet salmon fisheries. Human Ecology 44 (2): 153–165.
- Loring, P. A., H. L. Harrison, and S. C. Gerlach. 2014. Local perceptions of the sustainability of Alaska's highly contested Cook Inlet salmon fisheries. Society and Natural Resources 27 (2): 185–199.
- Lynch, A. J., B. J. E. Myers, C. Chu, L. A. Eby, J. A. Falke, R. P. Kovach, T. J. Krabbenhoft, T. J. Kwak, J. Lyons, C. P. Paukert, and J. E. Whitney. 2016. Climate change effects on North American inland fish populations and assemblages. Fisheries 41: 346–361.
- Lynch, J. A., J. S. Clark, N. H. Bigelow, M. E. Edwards, and B. P. Finney. 2002. Geographic and temporal variations in fire history in boreal ecosystems of Alaska. Journal of Geophysical Research: Atmospheres 107 (D1): 8152.
- MacFarlane, W. 2005. Snow River watershed hydrologic condition assessment. U.S. Department of Agriculture, Forest Service, Chugach National Forest, Anchorage, Alaska.
- Mantua, N. J., S. R. Hare, Y. Zhang, J. M. Wallace, and R. C. Francis. 1997. A Pacific interdecadal climate oscillation with impacts on salmon production. Bulletin of the American Meteorological Society 78 (6): 1069–1080.
- Matanuska–Susitna Basin Salmon Habitat Partnership. 2016. Healthy salmon/healthy communities 2014–2015. 12pp. Available: matsusalmon.org (July 2017).
- Mauger, S., M. McCarty, M. Bernard, and B. Bornemann. 2015. Science-based land conservation: conservation strategies to protect key salmon habitat in lower Kenai Peninsula watersheds. Cook Inlet-keeper, Homer, Alaska.
- Mauger, S., R. Shaftel, J. C. Leppi, and D. Rinella. 2017. Summer temperature regimes in southcentral Alaska streams: watershed drivers of variation and potential implications for Pacific Salmon. Canadian Journal of Fisheries and Aquatic Sciences 74: 702–715.
- McAfee, S. A., G. Guentchev, and J. K. Eischeid. 2013. Reconciling precipitation trends in Alaska: 1. Station-based analyses. Journal of Geophysical Research: Atmospheres 118 (14): 7523–7541.
- McAfee, S., G. Guentchev, and J. K. Eischeid. 2014a. Reconciling precipitation trends in Alaska: 2. Gridded data analyses. Journal of Geophysical Research: Atmospheres 119 (24): 13820–13837.
- McAfee, S. A., J. Walsh, and T. S. Rupp. 2014b. Statistically downscaled projections of snow/rain partitioning for Alaska. Hydrological Processes 28 (12): 3930–3946.
- McCarthy, M. D. 2016. Past and present Sockeye Salmon production in the Kenai River watershed, Alaska. Master's thesis. University of Alaska Anchorage.
- Meehl, G. A., A. Hu, and B. D. Santer. 2009. The mid-1970s climate shift in the Pacific and the relative roles of forced versus inherent decadal variability. Journal of Climate 22 (3): 780–792.
- Mellon, C. D., M. S. Wipfli, and J. L. Li. 2008. Effects of forest fire on headwater stream macroinvertebrate communities in eastern Washington, USA. Freshwater Biology 53 (11): 2331–2343.
- Millar, C. I., and N. L. Stephenson. 2015. Temperate forest health in an era of emerging megadisturbance. Science 349 (6250): 823–826.
- Millar, C. I., N. L. Stephenson, and S. L. Stephens. 2007. Climate change and forests of the future: managing in the face of uncertainty. Ecological Applications 17 (8): 2145–2151.
- Milner, A. M., J. E. Brittain, E. Castella, and G. E. Petts. 2001. Trends of macroinvertebrate community structure in glacier-fed rivers in relation to environmental conditions: a synthesis. Freshwater Biology 46 (12): 1833–1847.
- Milner, A. M., L. E. Brown, and D. M. Hannah. 2009. Hydroecological response of river systems to shrinking glaciers. Hydrological Processes 23 (1): 62–77.
- Milner, A. M., C. L. Fastie, F. S. Chapin, D. R. Engstrom, and L. C. Sharman. 2007. Interactions and linkages among ecosystems during landscape evolution. Bioscience 57 (3): 237–247.
- Milner, A. M., E. E. Knudsen, C. Soiseth, A. L. Robertson, D. Schell, I. T. Phillips, and K. Magnusson. 2000. Colonization and development of stream communities across a 200–year gradient in Glacier Bay National Park, Alaska, USA. Canadian Journal of Fisheries and Aquatic Sciences 57 (11): 2319–2335.
- Montgomery, D. 2003. King of fish: the thousand-year run of salmon. Westview Press, Cambridge, Massachusetts.
- Moore, J. W., M. McClure, L. A. Rogers, and D. E. Schindler. 2010. Synchronization and portfolio performance of threatened salmon. Conservation Letters 3 (5): 340–348.
- Morton, J., B. Blackburn, E. Bella, M. Steffy, C. Anderson, R. Massengill, J. Blackwell, L. Ka'aihue, R. Zulueta, and J. Chumley. 2014. Integrated pest management plan for eradicating Elodea from the Kenai Peninsula. Prepared for the Kenai Peninsula Cooperative Weed Management Area, Homer, Alaska.
- Morton, J. M., D. Reid, D. Beach, and D. Clark. In press. Novel community and trophic assemblages in the northwest boreal region. Chapter 4.2 in A. L. Sesser, A.P. Rockhill, D. R. Magness, D. Reid, J. DeLapp, P. Burton, E. Schroff, V. Barber & C. Markon, editors. Drivers of landscape change in the northwest boreal region of North America: Implications on policy and land management. U.S. Geological Survey Press. Reston, Virginia.
- Murphy, M. L., J. Heifetz, J. F. Thedinga, S. W. Johnson, and K. V. Koski. 1989. Habitat utilization by juvenile Pacific Salmon (Onchorynchus) in the glacial Taku River, Southeast Alaska. Canadian Journal of Fisheries and Aquatic Sciences 46 (10): 1677–1685.
- National Research Council. 1996. Upstream: salmon and society in the Pacific Northwest. National Academies Press, Washington, D.C.
- Neal, E. G., E. Hood, and K. Smikrud. 2010. Contribution of glacier runoff to freshwater discharge into the Gulf of Alaska. Geophysical Research Letters 37: L06404.
- Nesbitt, H. K., and J. W. Moore. 2016. Species and population diversity in Pacific Salmon fisheries underpin indigenous food security. Journal of Applied Ecology 53 (5): 1489–1499.
- Neuswanger, J. R., M. S. Wipfli, M. J. Evenson, N. F. Hughes, and A. E. Rosenberger. 2015. Low productivity of Chinook Salmon strongly correlates with high summer stream discharge in two Alaskan rivers in the Yukon drainage. Canadian Journal of Fisheries and Aquatic Sciences 72 (999): 1–13.
- Nielsen, J. L., G. T. Ruggerone, and C. E. Zimmerman. 2013. Adaptive strategies and life history characteristics in a warming climate: salmon in the Arctic? Environmental Biology of Fishes 96 (10–11): 1187–1226.
- Oasis Environmental. 2010. Kenai River hydrocarbon assessment, 2009. Final report prepared for Alaska Department of Environmental Conservation, Anchorage.
- Ohlberger, J., M. D. Scheuerell, and D. E. Schindler. 2016. Population coherence and environmental impacts across spatial scales: a case study of Chinook Salmon. Ecosphere 7 (4): e01333.
- O'Neel, S., E. Hood, A. Arendt, and L. Sass. 2014. Assessing streamflow sensitivity to variations in glacier mass balance. Climatic Change 123 (2): 329–341.
- O'Neel, S., E. Hood, A. L. Bidlack, S. W. Fleming, M. L. Arimitsu, A. Arendt, E. Burgess, C. J. Sergeant, A. H. Beaudreau, and K. Timm. 2015. Icefield-to-ocean linkages across the northern Pacific coastal temperate rainforest ecosystem. Bioscience 65 (5): 499–512.
- Pawluk, J. A. 2015. Operational plan: Sockeye Salmon escapement studies at the Russian River. Alaska Department of Fish and Game, Anchorage.
- Poesch, M. S., L. Chavarie, C. Chu, S. N. Pandit, and W. Tonn. 2016. Climate change impacts on freshwater fishes: a Canadian perspective. Fisheries 41: 385–391.
- Quinn, T. 2005. The behavior and ecology of Pacific Salmon and Trout. University of Washington Press, Seattle.
- Rine, K. M., M. S. Wipfli, E. R. Schoen, T. L. Nightengale, and C. A. Stricker. 2016. Trophic pathways supporting juvenile Chinook and Coho Salmon in the glacial Susitna River, Alaska: patterns of freshwater, marine, and terrestrial food resource use across a seasonally dynamic habitat mosaic. Canadian Journal of Fisheries and Aquatic Sciences 73 (11): 1626–1641.
- Rinella, D. J., M. Booz, D. L. Bogan, K. Boggs, M. Sturdy, and M. J. Rinella. 2009. Large woody debris and salmonid habitat in the Anchor River basin, Alaska, following an extensive spruce beetle (Dendroctonus rufipennis) outbreak. Northwest Science 83 (1): 57–69.
- Riordan, B., D. Verbyla, and A. D. McGuire. 2006. Shrinking ponds in subarctic Alaska based on 1950–2002 remotely sensed images. Journal of Geophysical Research: Biogeosciences 111: G04002.
- Rogers, L. A., and D. E. Schindler. 2008. Asynchrony in population dynamics of Sockeye Salmon in southwest Alaska. Oikos 117 (10): 1578–1586.
- Rogers, L. A., D. E. Schindler, P. J. Lisi, G. W. Holtgrieve, P. R. Leavitt, L. Bunting, B. P. Finney, D. T. Selbie, G. Chen, I. Gregory-Eaves, M. J. Lisac, and P. B. Walsh. 2013. Centennial-scale fluctuations and regional complexity characterize Pacific Salmon population dynamics over the past five centuries. Proceedings of the National Academy of Sciences 110 (5): 1750–1755.
- Roni, P., and T. P. Quinn. 2001. Density and size of juvenile salmonids in response to placement of large woody debris in western Oregon and Washington streams. Canadian Journal of Fisheries and Aquatic Sciences 58 (2): 282–292.
- Roon, D. A., M. S. Wipfli, T. L. Wurtz, and A. L. Blanchard. 2016. Invasive European bird cherry (Prunus padus) reduces terrestrial prey subsidies to urban Alaskan salmon streams. Canadian Journal of Fisheries and Aquatic Sciences 73 (11): 1679–1690.
- Ruff, C. P., D. E. Schindler, J. B. Armstrong, K. T. Bentley, G. T. Brooks, G. W. Holtgrieve, M. T. McGlauflin, C. E. Torgersen, and J. E. Seeb. 2011. Temperature-associated population diversity in salmon confers benefits to mobile consumers. Ecology 92 (11): 2073–2084. Ruggerone, G. T., B. A. Agler, B. M. Connors, E. V. FarleyJr, J. R. Irvine, L. I. Wilson, and E. M. Yasumiishi. 2016. Pink and Sockeye Salmon interactions at sea and their influence on forecast error of Bristol Bay Sockeye Salmon. North Pacific Anadromous Fish Commission Bulletin 6: 349–361.
- Saltmarsh, D. M., M. L. Bowser, J. M. Morton, S. Lang, D. Shain, and R. Dial. 2016. Distribution and abundance of exotic earthworms within a boreal forest system in southcentral Alaska. NeoBiota 28: 67–86.
- Savereide, J. W. 2005. Inriver abundance, spawning distribution, and run timing of Copper River Chinook Salmon, 2002–2004. Alaska Department of Fish and Game, Anchorage.
- Scannell, P. W. 2012. Taku-Tulsequah River mining activity: background environmental monitoring and potential mining effects. Alaska Department of Fish and Game, Douglas.
- Scenarios Network for Alaska and Arctic Planning. 2016. Available: http://snap.uaf.edu. (July 2017).
- Schindler, D. E., J. B. Armstrong, K. T. Bentley, K. Jankowski, P. J. Lisi, and L. X. Payne. 2013. Riding the crimson tide: mobile terrestrial consumers track phenological variation in spawning of an anadromous fish. Biology Letters 9 (3): 20130048.
- Schindler, D. E., X. Augerot, E. Fleishman, N. J. Mantua, B. Riddell, M. Ruckelshaus, J. Seeb, and M. Webster. 2008. Climate change, ecosystem impacts, and management for Pacific Salmon. Fisheries 33 (10): 502–506.
- Schindler, D. E., and R. Hilborn. 2015. Prediction, precaution, and policy under global change. Science 347 (6225): 953–954.
- Schindler, D. E., R. Hilborn, B. Chasco, C. P. Boatright, T. P. Quinn, L. A. Rogers, and M. S. Webster. 2010. Population diversity and the portfolio effect in an exploited species. Nature 465 (7298): 609–612.
- Scholz, N. L., M. S. Myers, S. G. McCarthy, J. S. Labenia, J. K. McIntyre, G. M. Ylitalo, L. D. Rhodes, C. A. Laetz, C. M. Stehr, B. L. French, B. McMillan, D. Wilson, L. Reed, K. D. Lynch, S. Damm, J. W. Davis, and T. K. Collier. 2011. Recurrent die-offs of adult Coho Salmon returning to spawn in Puget Sound lowland urban streams. PLoS One 6 (12): e28013.
- Sepulveda, A. J., D. S. Rutz, A. W. Dupuis, P. A. Shields, and K. J. Dunker. 2015. Introduced Northern Pike consumption of salmonids in south-central Alaska. Ecology of Freshwater Fish 24 (4): 519–531.
- Sergeant, C. J., J. R. Bellmore, C. McConnell, and J. W. Moore. 2017. High salmon density and low discharge create periodic hypoxia in coastal rivers. Ecosphere 8 (6): e01846.
- Sethi, S. A., M. Reimer, and G. Knapp. 2014. Alaskan fishing community revenues and the stabilizing role of fishing portfolios. Marine Policy 48: 134–141.
- Shanley, C. S., and D. M. Albert. 2014. Climate change sensitivity index for Pacific Salmon habitat in Southeast Alaska. PLoS One 9 (8): e104799.
- Shanley, C. S., S. Pyare, M. I. Goldstein, P. B. Alaback, D. M. Albert, C. M. Beier, T. J. Brinkman, R. T. Edwards, E. Hood, A. MacKinnon, M. V. McPhee, T. M. Patterson, L. H. Suring, D. A. Tallmon, and M. S. Wipfli. 2015. Climate change implications in the northern coastal temperate rainforest of North America. Climatic Change 130 (2): 155–170.
- Shields, P., and A. Dupuis. 2016. Upper Cook Inlet commercial fisheries annual management report, 2015. Fishery Management Report 16–14. Alaska Department of Fish and Game, Anchorage.
- Shugar, D. H., J. J. Clague, J. L. Best, C. Schoof, M. J. Willis, L. Copland, and G. H. Roe. 2017. River piracy and drainage basin reorganization led by climate-driven glacier retreat. Nature Geoscience 10 (5): 370–375.
- Shulski, M., and G. Wendler. 2007. The climate of Alaska. University of Alaska Press, Fairbanks.
- Simpson, E. H. 1949. Measurement of diversity. Nature 163: 688–688.
- Sloat, M. R., G. H. Reeves, and K. R. Christiansen. 2017. Stream network geomorphology mediates predicted vulnerability of anadromous fish habitat to hydrologic change in southeast Alaska. Global Change Biology 23 (2): 604–620.
- Spromberg, J. A., D. H. Baldwin, S. E. Damm, J. K. McIntyre, M. Huff, C. A. Sloan, B. F. Anulacion, J. W. Davis, and N. L. Scholz. 2016. Coho Salmon spawner mortality in western U.S. urban watersheds: bio-infiltration prevents lethal storm water impacts. Journal of Applied Ecology 53 (2): 398–407.
- Stewart, I. T., D. R. Cayan, and M. D. Dettinger. 2005. Changes toward earlier streamflow timing across Western North America. Journal of Climate 18 (8): 1136–1155.
- Stopha, M. 2017. Alaska fisheries enhancement annual report 2016. Alaska Department of Fish and Game, Anchorage.
- Tobias, T. M., and T. M. Willette. 2013. An estimate of total return of Sockeye Salmon to Upper Cook Inlet, Alaska, 1976–2008. Alaska Department of Fish and Game, Division of Commercial Fisheries, Anchorage.
- Torgersen, C. E., D. M. Price, H. W. Li, and B. A. McIntosh. 1999. Multiscale thermal refugia and stream habitat associations of Chinook Salmon in northeastern Oregon. Ecological Applications 9 (1): 301–319.
- Trammell, E. J., and M. Aisu. 2015. Development of a landscape integrity dataset for the Alaska crucial habitat assessment tool. Prepared for the Alaska Department of Fish and Game, Anchorage.
- U.S. Fish and Wildlife Service. 2016. Species checklist. Kenai National Wildlife Refuge, Soldotna, Alaska.
- VanLooy, J., R. Forster, and A. Ford. 2006. Accelerating thinning of Kenai Peninsula glaciers, Alaska. Geophysical Research Letters 33: L21307.
- Vannote, R. L., G. W. Minshall, K. W. Cummins, J. R. Sedell, and C. E. Cushing. 1980. The river continuum concept. Canadian Journal of Fisheries and Aquatic Sciences 37 (1): 130–137.
- Wallace, J. B., S. L. Eggert, J. L. Meyer, and J. R. Webster. 1997. Multiple trophic levels of a forest stream linked to terrestrial litter inputs. Science 277 (5322): 102–104.
- Walters, C. J., and S. J. D. Martell. 2004. Fisheries ecology and management. Princeton University Press, Princeton, New Jersey.
- Waples, R. S., G. R. Pess, and T. Beechie. 2008. Evolutionary history of Pacific Salmon in dynamic environments. Evolutionary Applications 1 (2): 189–206.
- Ward, E. J., J. H. Anderson, T. J. Beechie, G. R. Pess, and M. J. Ford. 2015. Increasing hydrologic variability threatens depleted anadromous fish populations. Global Change Biology 21 (7): 2500–2509.
- Williams, J. W., and S. T. Jackson. 2007. Novel climates, no-analog communities, and ecological surprises. Frontiers in Ecology and the Environment 5 (9): 475–482.
- Winfree, M. 2017. The influence of geomorphic and landscape characteristics on stream temperature and streamwater sensitivity to air temperature in the coastal temperate rainforest of Southeast Alaska. M.S. Thesis. University of Alaska Fairbanks.
- Wipfli, M. S. 1997. Terrestrial invertebrates as salmonid prey and nitrogen sources in streams: contrasting old-growth and young-growth riparian forests in southeastern Alaska, USA. Canadian Journal of Fisheries and Aquatic Sciences 54 (6): 1259–1269.
- Wipfli, M. S., and J. S. Richardson. 2015. Riparian management and the conservation of stream ecosystems and fishes. Pages 270–291 in G. P. Closs, M. Krkosek, and J. D. Olden, editors. Conservation of freshwater fishes. Cambridge University Press, Cambridge, U.K.
- Wobus, C., R. Prucha, D. Albert, C. Woll, M. Loinaz, and R. Jones. 2016. Hydrologic alterations from climate change inform assessment of ecological risk to Pacific Salmon in Bristol Bay, Alaska. PLoS One 11 (1): e0147242.
- Wolken, J. M., T. N. Hollingsworth, T. S. Rupp, F. S. Chapin, S. F. Trainor, T. M. Barrett, P. F. Sullivan, A. D. McGuire, E. S. Euskirchen, P. E. Hennon, E. A. Beever, J. S. Conn, L. K. Crone, D. V. D'Amore, N. Fresco, T. A. Hanley, K. Kielland, J. J. Kruse, T. Patterson, E. A. G. Schuur, D. L. Verbyla, and J. Yarie. 2011. Evidence and implications of recent and projected climate change in Alaska's forest ecosystems. Ecosphere 2 (11): 1–35.
- Zedler, J. B., and S. Kercher. 2005. Wetland resources: status, trends, ecosystem services, and restorability. Annual Review of Environment and Resources 30: 39–74. AFS