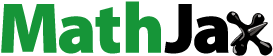
Abstract
Objective
In our previous taste-masking study, we found that Acesulfame K (AK) had a better taste-masking effect than other high-efficiency sweeteners for several representative bitter natural drugs in aqueous decoction. Furthermore, we performed a preliminary taste-masking study of AK for representative bitter API Berberine Hydrochloride (BH) and found that it had a good taste-masking effect. We also found that flocculent precipitation was generated in the BH solution, but it was not clear whether it was related to the good taste-masking effect. This study was conducted to explore the taste-masking effect and mechanism of AK on BH.
Methods
The taste-masking effect of AK on BH was evaluated based on the Traditional Human Taste Panel Method and the electronic tongue evaluation method. DSC, XRD, and molecular simulation techniques were used to explore the mechanism of AK on BH, from the macro level and molecular level, respectively.
Results
When evaluating the taste-masking effect, we found that 0.1% AK had the best taste-masking effect on BH, while higher concentrations had a worse taste-masking effect. DSC and XRD revealed that the flocculent precipitation was a complex AK-BH. Finally, by simulating the binding of AK, BH, and TAS2R46 receptors, we found the unique taste-masking mechanism of AK.
Conclusion
The sweet taste stimulus of AK can mask the bitter taste stimulus of BH, and AK can generate AK-BH with BH to reduce the contact between BH and bitter taste receptors. Additionally, it could block the expression of the TAS2R46 receptors.
Graphical Abstract
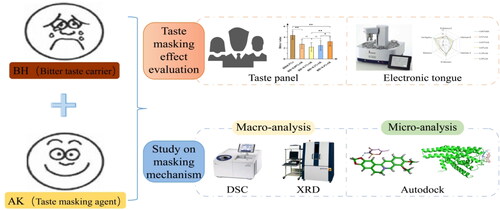
Introduction
Berberine hydrochloride (BH), an isoquinoline alkaloid extracted from Rhizoma coptidis and Cortex phellodendri, is widely used to treat gastrointestinal diseases, including acute gastroenteritis, cholera, and bacillary dysentery [Citation1,Citation2]. However, BH has a strong bitter taste and poor patient compliance, especially for the oral administration of liquid formulations containing BH, making it important to mask its bitter taste [Citation3,Citation4]. So far, some techniques (adding sweeteners, preparing complexes, coating, preparing microcapsules or microspheres, etc.) have been developed to mask the taste of bitter drugs [Citation5–7]. Many scholars have also developed reliable taste-masking techniques for BH, such as preparing microspheres or microcapsules to encapsulate bitter molecules [Citation3,Citation8], adding bitterness inhibitors to inhibit the binding of bitter molecules to the corresponding receptors [Citation9], or using powder modification techniques to mask the bitterness of BH [Citation4,Citation10]. Adding sweeteners directly is the most basic, simplest, and important method of taste masking, and can be easily applied to almost all forms of drugs [Citation5,Citation11]. However, there are few studies on the use of this method to mask the bitterness of BH. We speculate that this could be due to the strong bitterness of BH: some scholars believe that the masking effect is not very good when adding just sweeteners aloneto BH. However, our previous studies found inconsistent phenomena: the use of sweetener alone on BH could also achieve a good taste-masking effect [Citation12].
The artificial sweetener Acesulfame K (AK) is a high-potency sweetener, whose sweetness is 200 times that of sucrose [Citation13]. It is not metabolized or stored after being absorbed into the body and is commonly used as a calorie-free sweetener [Citation14]. It has been widely used in food, beverage, and pharmaceutical applications [Citation15–17]. In a previous study, we assessed the taste-masking effect of AK and aspartame on the aqueous decoction of bitter natural drugs and found that AK had a good taste-masking effect on the aqueous decoction of bitter natural drugs (Lotus plumule and Radix sophorae flavescentis) containing alkaloid bitter components. The highest bitterness inhibition rates were 63.38 and 55.84%, respectively [Citation12], and we speculated that AK could also have a better taste-masking effect on BH. As such, we designed this experiment to explore the taste-masking effect and mechanism of AK on BH.
The existing mechanisms of taste-masking are broadly classified into three categories: taste-masking by confusing gustatory or olfactory stimuli; reducing or blocking the contact of bitter molecules with taste receptors; and inhibiting bitter taste receptors expression [Citation18]. During the pre-experiments, we found that a yellow flocculent precipitate would appear when AK was added to the BH solution. Through the optical microscope, we observed fine, villous crystals in the precipitate that were different from BH, which led us to speculate that AK could have formed a complex AK-BH with BH; some studies consider this to be a new salt with low solubility [Citation19]. The formation of the complex could attenuate the bitter taste by reducing the contact between the bitter molecules and the taste receptors. Combined with AK on BH taste-masking effect and this phenomenon, we speculated that the mechanism of AK on BH taste-masking could contain the following three aspects: the sweet taste stimulus of AK masks the bitter taste stimulus of BH; the contact of BH with taste receptors is reduced by the formation of precipitation; AK competes with TAS2R46 receptors (a bitter TAS2R receptors that BH can activate) for the binding site of BH, and thereby reducing the expression of the TAS2R46 receptors.
Based on the above, this study evaluates the taste-masking effect and regularity of AK on BH using the THTPM and E-tongue evaluation method. The macroscopic taste-masking mechanism of AK on BH is explored with the help of DSC and other techniques, and the taste-masking mechanism is explored at the molecular level in combination with molecular simulation software. Based on previous studies, the taste-masking effect and potential taste-masking mechanism of AK on BH will be further explored to provide a reference for bitter API taste masking.
Materials and methods
Materials
Berberine hydrochloride was purchased from Sichuan Yuxin Pharmaceutical Co., Ltd. AK was purchased from Suzhou Haobo Technology Co., Ltd. Hydrochloric acid was purchased from Luoyang Chemical Reagent Factory. Absolute alcohol was purchased from Tianjin Yongda Chemical Reagent Co., Ltd. Water (The water was purified water).
Evaluation of taste masking effect
Preparation of samples
Division of bitterness intensity
Bitterness intensity was divided into five grades, and each grade corresponded to a certain range of bitterness values [Citation20–23]. The BH solutions of different mass concentrations were used as the bitterness reference solution, which corresponded to different bitterness grades, as shown in .
Table 1. Qualitative description, grade, and quantitative range of bitterness value (I).
Preparation of positive and negative reference solution
Preparation of positive reference solution: took 0.05 g BH, added 800 ml purified water, and stirred to dissolve; after completed dissolution, transferred to a 1000 ml volumetric flask and added purified water to fix the volume to 1000 ml. 0.05 g·L−1 BH solution was obtained as the positive reference solution, and the bitterness value was set to 3.0, according to previous experiment [Citation21].
Preparation of negative reference solution: 1000 ml of purified water was taken and placed in a 1000 ml volumetric flask, which was used as the negative reference solution.
Preparation of mixed solutions with different concentrations of AK and a certain concentration of BH
According to the literature [Citation21] and the clinical dosage of BH, the BH concentrations were set as a low-concentration solution group (0.005% g·mL−1) and the high-concentration solution group (0.5% g·mL−1).
Low concentration solution group: 0.20 g of BH was weighed and dissolved in 4000 ml purified water to yield the low concentration solution of BH (0.005% g·mL−1), and six portions were divided from the stock (600 ml per portion). Five of the above aliquots were added with AK at doses of 0.06, 0.15, 0.30, 0.60, or 1.20 g, and stirred for a sufficient reaction until the yellow flocculent precipitate was observed.
High-concentration solution group: 15 g of BH was weighed and dissolved into 3000 ml purified water to yield the high-concentration solution of BH (0.5% g·mL−1). Then the solution was divided into five parts of 600 ml each. Four of these aliquots were added with AK at doses of 0.30, 0.60, 1.20, or 2.40 g, and stirred for a sufficient reaction until the yellow flocculent precipitate was observed. The above two groups of samples were tested according to the ‘Traditional Human Taste Panel Method’ and the ‘E-tongue evaluation method.’
Preparation of mixed solutions of AK with a certain concentration and BH with different concentrations
BH solutions with concentrations of 0.4, 0.2, and 0.1% were prepared and recorded as a, b, and c, respectively. Take 500 ml of a, b, and c solution, respectively, without treatment, as the control group. Another equal amount of a, b, and c solution was taken, and 0.5 g AK was added to the solution as the experimental group. The samples were tested in accordance with the ‘Traditional Human Taste Panel Method’ and the ‘E-tongue evaluation method.’
Preparation of AK-BH complex
The solubility of BH was determined to be ∼0.5% by the preliminary study. Added 2.5 g BH to 400 ml of hot water, stirred to completely dissolve, cooled and transferred to a volumetric flask, and fixed to 500 ml. Weighed 2.0001 g AK, and added 200 ml of purified water to make a 1% (g·mL−1) solution. Took 5 parts of 100 ml of BH solution, and added 7.2, 10.0, 16.7, 25.0, and 50.0 ml of the above AK solution; the molar ratio of BH to AK in the 5 solution was about 3.5:1, 2.5:1, 1.5:1, 1:1, 0.5:1, respectively. Stirred to make it fully react, filtered to obtain the precipitate, and dried to obtain the complex AK-BH.
Traditional human taste panel method
Volunteers screening
After the ethics committee of The First Affiliated Hospital of Henan University of Chinese Medicine reviewed and approved this study (Ethics Number: YFYKYLL2011-002-03), volunteers were strictly screened. Fourteen healthy volunteers (six males and eight females) were selected as subjects and signed an ‘Informed consent form’ before the trial.
Taste evaluation method
In this study, based on the Traditional Human Taste Panel Method [Citation21,Citation22,Citation24], a combination of ranking (bitterness scale) and scoring (relative reference score) was used to evaluate bitterness intensity [Citation25]. First, each volunteer received five different mass concentrations of the bitter reference solution and held it in his/her mouth for 15 s. During this time, the volunteer gargled several times so that the taste perception area at the root and side of the tongue could perceive the taste. At the same time, they were informed of the bitterness grade and specific bitterness value of the solution to form a standardized sensory memory for bitterness. Volunteers spat the reference solution out and gargled their mouths with purified water at least three times until the mouth was free of the bitter taste. With the same method as above, the test of other mass concentration bitter reference solution was carried out, and the test interval of every two reference solutions was 15 min.
Determination of samples
The test procedure was evaluated in the same way as the reference solution. Approximately 5–6 samples were evaluated in each round. All volunteers were instructed to rank the bitterness of these samples in ascending order, provided a specific bitterness value for each sample by referring to the bitterness of the positive reference solution, and recorded it in the ‘drug bitterness sort and score table’ (). Each sample could be repeatedly evaluated, and the mouth was rinsed three times before evaluating the next sample. A break of 1 h was taken between each round of samples. In addition, the samples were tested by volunteers in the experiment using a randomized, single-blind method.
Table 2. Drug bitterness sort and score table.
Data processing
Due to the experimental volunteers being biological samples, there could be individual differences between different volunteers. At the same time, there were individual outliers in the test data of volunteers, but they could not be taken arbitrarily. Therefore, the Spearman correlation coefficient test was first used to compare the correlation between the bitterness grade of each round of samples judged by volunteers and the theoretical bitterness grade of samples. The Spearman coefficient of each volunteer was calculated according to EquationEq.(1)(1)
(1) in National Standard GBT12315-2008 [Citation26]. The data of volunteers with low Spearman coefficient correlation (coefficient <0.5) were excluded to eliminate the impact of partial volunteers on the whole round of results. The data of volunteers who passed the Spearman coefficient test were subjected to round-robin testing and elimination of outliers using the Grubbs test [Citation27,Citation28]. Only one outlier was eliminated in each round, and multiple rounds of cyclic inspection were performed. In addition, oversized outliers and undersized outliers were both likely to exist in taste experiments, so a two-sided test was used in this study. Taking into account the characteristics of the biological sample, the rejection level of this experiment was chosen to be 0.05, and no new values were inserted after the outliers were eliminated.
(1)
(1)
In the above formula, rs represents the Spearman Correlation Coefficient, di represents the difference between two ranks of the sample, and p represents the number of samples participating in sorting.
Calculation of Ir (%) (Bitterness Inhibition rate)
(2)
(2)
In the above formula, Ir (%) is the intensity of the bitterness reduction rate. Ia is the intensity of bitterness after taste masking. Ib is the intensity of bitterness before taste masking.
E-tongue evaluation method
Samples preparation
According to sample preparation.
Selection of E-tongue sensors
The TS-5000Z electronic tongue has seven sensors: C00, AN0, BT0, AE1, GL1, CA0, and CT0. Of these, the C00 and AE1 sensors have two types of bitterness information, called first taste and aftertaste, while the AN0 and BT0 sensors only output the aftertaste value [Citation22]. Therefore, this experiment selected 4 sensors related to bitterness (C00, AN0, BT0, and AE1), which outputted a total of 6 taste information values [Citation29,Citation30]. Specific information is shown in .
Table 3. Sensor taste information.
TS-5000Z E-tongue (Intelligent Sensor Technology, Insent, Japan) measurement procedures
First, the sensors were cleaned for 90 s in a cleaning solution, followed by 120 s with a reference solution, and continued with another reference solution for 120s. The sensors were zeroed in the equilibrium position for 30 s. After reaching the equilibrium condition, the test started with a test time of 30 s, and the sensors outputted the first taste value. Then, the sensors were briefly cleaned in the two groups of reference solutions for 3 s, and the sensors were inserted into the new reference solution to test the aftertaste for 30 s. It was cycled 4 times. The result of the first cycle was removed, and the average value of the last 3 times was considered the test result. Each cleaning, equilibrating, and testing of the sensors were performed in different sample cups [Citation21,Citation22].
Exploring the mechanism of taste-masking based on macroscopic characterization
Differential scanning calorimetry (DSC) analysis
The differential scanning calorimeter (DSC, Q100 type, American TA company) was used to analyze the thermal characteristics of the complex formed by BH and AK. BH, AK, and the complex formed by BH and AK at molar ratios of 0.5:1, 1:1, 1.5:1, 2.5:1, and 3.5:1, and the physical mixture of BH and AK in the molar ratio of 1:1 were obtained and placed into aluminum dishes, covered, pressed, and placed in the sample determination cell of DSC. The reference cell was placed in a blank aluminum dish, sample weight 4–5 mg, the upper limit temperature was 250 °C, and the test was performed at a heating rate of 10 °C/min.
X-ray diffraction analysis
BH, AK, the physical mixture of AK and BH (molar ratio 1:1), and the recrystallized samples of the complex formed by BH and AK (molar ratio 1:1) were performed with X-ray powder diffraction. X-ray diffraction patterns were obtained using an X-ray diffractometer (D8 Focus, Bruker AXS, Germany) with Cu-K radiation at a voltage of 40 kV, 40 mA. The samples were scanned in increments of 0.02° from 3.400° to 40.001° (diffraction angle 2θ/θ) at a room temperature of 25 °C, and the counting time was 12.8 s.
Study on the mechanism of AK taste-masking based on molecular simulation
AK and BH generated new complexes in the solution state, and macroscopically they existed in the form of flocculent precipitation. To explore the binding mode of AK and BH at the microscopic level and the potential taste-masking mechanism, and to develop a new taste-masking technique, we simulated the possible binding mode of the two molecules and the binding mode of BH and AK to the bitter receptors TAS2R46 with the help of molecular simulation software, such as Autodock.
The docking of AK and BH
Molecular structure acquisition and processing
The 2D molecular structures of AK and BH were downloaded from https://pubchem.ncbi.nlm.nih.gov/ and the chemical structures were shown below in . AK was imported into Chem 3D 19.0 for energy minimization.
Docking parameters setting
The parameters were set to x = 40, y = 28, z = 14, spacing = 0.375, and x, y, and z denoted the number of grid points in each direction. Spacing denoted the length of each grid point, and the center coordinate of the Grid box was (1.892, 0.043, 0.005). The Genetic Algorithm was chosen, and the search parameter ‘Maximum Number of evals medium’ was set to 2,500,000. All other parameters used the system’s default parameters. After that, the Genetic Algorithm was used to output the docking parameter file. Finally, Discovery Studio 4.5 software was used to visualize and analyze the molecular docking results.
Docking of small molecules to TAS2R46 protein
Protein structure acquisition
TAS2R46 receptors protein [Citation31] was acquired from https://bitterdb.agri.huji.ac.il/dbbitter.php.
Docking parameters setting
The docking parameters of AK and TAS2R46 protein were x = 65, y = 73, z = 93, spacing = 0.772, and the center coordinate of the Grid box was (4.831, −5.444, −3.078). The docking parameters of BH and TAS2R46 were x = 74, y = 72, z = 96, spacing = 0.761, and the center coordinate of the Grid box was (2.821, −4.900, −2.405). Before docking, AK and BH were imported into Chem 3D 19.0 for energy minimization. Other parameters were set in the same way as ‘The docking of AK and BH.’ The molecular docking results were visualized and analyzed using Pymol 2.6.0.
Results
Evaluation of taste-masking effect: traditional human taste panel method
The results of the ranking method were first subjected to Spearman’s correlation coefficient test, and the values with coefficients <0.5 were excluded. Then the data of the remaining volunteers were subjected to the Grubbs test, and the rating data were processed by a t-test after excluding the outliers.
Evaluation of the taste-masking effect of different concentrations of AK on a certain concentration of BH
Low-concentration solution group
The data of this group had two abnormal values (rs = 0.286, rs = 0.071), which could be because individual volunteers’ perceptions and tolerance of bitterness and the bitterness of sweetness were different from others, so the bitterness ranking of the samples was different from most volunteers. There were large differences based on the Spearman correlation coefficient test and were eliminated by Spearman coefficient test. The Grubbs test had no outliers, and the experimental results, after eliminating outliers, are shown in .
Figure 2. (A) The taste-masking effect of 0.005% g·mL−1 BH by different concentrations of AK. (B) The taste-masking effect of 0.5% g·mL−1 BH by different concentrations of AK. (C) The taste-masking effect of 0.1% g·mL−1 AK on different concentrations of BH. Note. nsp > 0.05; *p < 0.05; **p < 0.01.
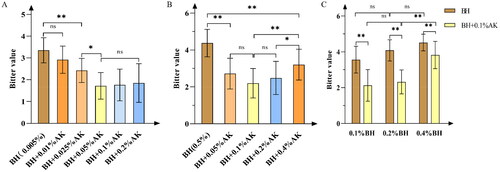
Although the bitterness value of the BH solution with 0.01% AK added decreased compared with the original solution, there is no significant difference between the two groups (p > 0.05, n = 12). The bitterness value of the sample solution added with 0.025% AK and the original solution have a very significant difference (p < 0.01, n = 12), and the bitterness value decreased from 3.25 ± 0.63 to 2.48 ± 0.50, and Ir (%) is 23.69%. There are no significant differences in the bitterness values of the three groups of sample solutions of 0.05, 0.1, and 0.2% AK (p > 0.05, n = 12). Among them, 0.05 and 0.1% AK have the best taste-masking effect, and the bitterness values reduced from 3.25 ± 0.63 to 1.78 ± 0.57 and 1.78 ± 0.63, respectively, and the Ir (%) are both 45.23%.
High-concentration solution group
The data in this group had 1 outlier (rs = 0.30) by Spearman’s correlation coefficient test, which was eliminated by Spearman’s coefficient test. Two outliers were found by Grubbs’ test, and the experimental results after eliminating the outliers are shown in .
There is a very significant difference between the BH solution adding AK and the BH solution without AK (p < 0.01, n = 11). Among them, adding 0.1% AK solution has the best taste-masking effect, which reduces the bitterness value of 0.5% BH from 4.41 ± 0.73 to 1.96 ± 0.53, and Ir (%) is 55.56%. There is no significant difference between the BH solution with 0.1, 0.05, and 0.2% AK (p > 0.05, n = 10). The bitterness value of the BH solution with 0.4% AK is 3.34 ± 0.71, and the bitterness value of the BH solution with 0.1% AK is 1.96 ± 0.53, which has a very significant difference (p < 0.01, n = 11) between the two groups. The results indicated that the bitterness value of the solution had an increasing trend when AK is excessively added.
Evaluation of the taste-masking effect of a certain concentration of AK on different concentrations of BH
There were no outliers in this group of data, and the experimental results are shown in .
The bitterness values of the three concentrations of BH solutions significantly decreased after adding 0.1% AK (p < 0.01, n = 13): from 3.56 ± 0.75, 4.08 ± 0.59, 4.52 ± 0.47 to 2.20 ± 0.88, 2.32 ± 0.67, 3.82 ± 0.76, respectively, with Ir (%) of 38.20, 43.14, and 15.49%. The bitterness values of 0.1% and 0.2% BH solution after adding 0.1% AK are significantly lower than that of 0.4% BH solution after adding 0.1% AK (p < 0.01, n = 13). This is because there are more bitter substances in the 0.4% BH solution, and adding 0.1% AK does not make it sweet enough to mask the bitterness and cannot completely convert BH into precipitate. As such, there is much BH in the solution, and it remains bitter.
Evaluation of taste-masking effect: E-tongue evaluation
Evaluation of the taste-masking effect of different concentrations of AK on a certain concentration of BH
Low-concentration solution group
shows the taste radar diagram of the taste-masking effect of different concentrations of AK on low concentrations of BH (0.005%), where the units of the horizontal and vertical coordinates indicate units of taste. According to the literature [Citation32], the sensors of TS-5000Z responding to BH are the AN0 sensor and BT0 sensor. Therefore, the taste information value of the BT0 sensor decreases after adding AK. When AK is added at 0.05 and 0.1%, the taste information value of the BT0 sensor is the lowest, indicating that the best taste-masking effect is achieved at this time. However, the value of taste information increases when AK reaches a certain concentration, which was consistent with the evaluation results of THTPM. We also found that the first taste of the AE1 sensor and the first taste and aftertaste information value of the C00 sensor significantly changed, and the information value generally increased as the AK-added concentration increased. It is possible that these three sensors were more sensitive to the change in AK concentration, and subsequent experiments will also be performed to investigate this further.
Figure 3. (A) The taste radar plot of 0.005% g·mL−1 BH by different concentrations of AK. (B) The taste radar plot of 0.1% g·mL−1 AK on different concentrations of BH. And a, b, and c are BH solution without AK, their concentrations are 0.4, 0.2, and 0.1% g·mL−1, respectively. Aa, Ab, and Ac are BH solutions with 0.1% g·mL−1 AK, respectively.
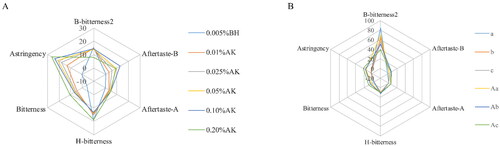
High-concentration solution group
There was no significant difference in the information value of the sensors between the samples of 0.5% BH concentration during the test. After consulting the relevant technical personnel, we found that this situation could be caused by the high concentration of the sample, making it impossible to evaluate the taste-masking effect of different concentrations of AK on 0.5% BH by E-tongue.
Evaluation of the taste-masking effect of a certain concentration of AK on different concentrations of BH
shows the taste radar diagram of the taste-masking effect of a certain concentration of AK on different concentrations of BH. The taste information of the AN0 sensor of three different concentrations of BH all decreased after adding a certain concentration of AK, and the bitterness information values are ranked from largest to smallest: Aa > Ab > Ac. When the mass concentration ratio of BH and AK is 1:1, the taste information value of the AN0 sensor decreased the most. The potential value was the lowest, indicating that the bitterness value of the solution was the lowest at this time, which was consistent with the evaluation results of THTPM.
Exploring the mechanism of AK taste-masking based on macroscopic characterization
DSC analysis
The DSC curves of complexes generated by BH and AK in different ratios are very similar, and the attached figure only shows the DSC curve of the complex formed by a molar ratio of 1:1 (). AK has been in the glass transition stage without a melting peak, which because the melting point of AK is 250 °C (). The DSC curves of the physical mixture of BH with AK and BH have four roughly identical endothermic peaks at ∼100 °C (), in which the peak is attributed to the loss of water of BH. The third endothermic peak at ∼200 °C is the melting peak of BH, which is consistent with the melting point of BH (204–206 °C). There are also two endothermic peaks around 152 and 220 °C, though we are unsure what causes them and will further explore this using other approaches. The DSC curve of the complex AK-BH relative to AK, BH, has an exothermic peak at about 227 °C. This is a crystallization process, indicating the formation of new crystals (). The difference in the characteristic peaks between the complex AK-BH and the other substances indicates that the complex was different from either AK, BH, or the physical mixture of the two. This is a completely new substance, considering that AK and BH could have generated a new complex by forming the hydrogen bond.
X-ray diffraction analysis
demonstrates that the characteristic peaks of AK, BH, their physical mixture, and the complex AK-BH are significantly different, and the characteristic peaks of the physical mixture of AK and BH cover all the characteristic diffraction peaks of AK and BH. AK has three major characteristic peaks when 2θ is 8.5, 17, and 25.5. BH has four characteristic peaks when 2θ is 6.9, 9.1, 14, and 26. The physical mixture of the two has characteristic peaks when 2θ is 6.9, 9, 14, 17, 25.5, and 26, which includes all the characteristic peaks of AK and BH. AK-BH has new characteristic peaks when 2θ are 5.7 and 17.5, which was different from AK and BH. This indicates that AK and BH generate a new complex, which was consistent with the results of the DSC analysis. AK-BH existed in the form of precipitation, which can achieve taste-masking by reducing the contact between BH and bitter taste receptors.
Study on the mechanism of taste-masking based on molecular simulation
The docking of AK and BH
Among the 50 docked conformations, 27 docked conformations formed the optimal conformational clusters, indicating that the docking test converged well. Two of the 27 optimal conformations of AK-BH docking are shown in . The intermolecular hydrogen bond is formed between AK and BH, where the nitrogen and hydrogen atoms in the AK structure form an intermolecular hydrogen bond with the oxygen atom in the BH structure. The binding energies of the two conformations were −2.36 and −2.33 kcal·mol−1, respectively, and the van der Waals forces were −2.34 and −2.32 kcal·mol−1.
Docking of small molecules to proteins
Docking of BH with TAS2R46 protein
Since no relevant studies have found the active center of the TAS2R46 receptors and the corresponding activation site, it was impossible to set the scope of molecular docking and accurately identify the target binding site. From the molecular docking results, two types of docking had been simulated: the first is the formation of one hydrogen bond between BH and the amino acid (LYS) at position 156 of the receptors () with binding energy was −4.22 kcal·mol−1 and the van der Waals force was −4.91 kcal·mol−1. The second is the formation of two hydrogen bonds between BH and the amino acids (ASN and GLN) at positions 150 and 151 of the receptors (). The binding energy was −3.92 kcal·mol−1 and the van der Waals force was −4.63 kcal·mol−1. The first binding mode had lower binding energy and a more stable structure, which was more likely to occur. On the premise of accurate and reliable docking results, the activation sites of the TAS2R46 receptors should theoretically be one or two of ASN, GLN, and LYS.
Docking of AK to TAS2R46 protein
Among the 50 docking results, a total of 35 times AK bounded to the TAS2R46 receptors by forming hydrogen bonds, and there were cases of AK docking with multiple amino acids, including the binding to the amino acids TRP, LYS, HIS, PHE, ARG, and GLN. Since the active center and active site of the receptors could not be determined, and the docking results were complex and diverse, we could not derive accurate and referable docking results from this limited theoretical basis.
Based on the analysis of the docking results of AK and BH, and the docking results of small molecules and the TAS2R46 receptors, if the binding of BH and the TAS2R46 receptors was the first case, the formation of the complex will affect the binding of BH to the TAS2R46 receptors, which could affect the activation of bitter taste receptors. In this case, the third mechanism of taste masking could exist, in which the formation of the complex inhibited the activation of bitter receptors, weakening the perception of bitterness. If BH binding to the TAS2R46 receptors was the second case, then the formation of the complex will not affect the binding of BH to the TAS2R46 receptors. As such, it did not affect the activation of bitter taste receptors, at which point there was no third conjectured taste-masking mechanism.
Discussion
BH, as the representative of alkaloid bitterness components, has a strong bitterness. Alkaloid bitter components are common in many bitter natural drugs/herbs. Therefore, we hope to explore a general method used for the taste-masking of bitter alkaloid components and bitter natural drugs by studying the effect and mechanism of AK on BH taste-masking.
When evaluating the taste-masking effect, we selected the THTPM and E-tongue evaluation methods. From the results in and , it was not difficult to find that when the added concentration of AK was 0.1%, the taste-masking effect was the best. The conclusions obtained by the two methods are also consistent. The combination of two methods not only makes up for certain shortcomings (such as the strong subjectivity of the THTPM method [Citation33–35]) but also validates the results of THTPM. The two-way inspection of the results of the two evaluation methods makes the conclusion more accurate and convincing. However, the evaluation data of THTPM of the BH high concentration group could not be further verified by the E-tongue test due to the sub-optimal design. Fortunately, the evaluation data of THTPM was sufficient for us to evaluate the taste-masking effect of AK and analyze its taste-masking regularity.
In this study, the effect of oral taste evaluation was best when the mass concentration of added AK was 0.1%. However, when the mass concentration of AK continued to increase, the bitterness value no longer decreased but tended to increase. Some scholars have concluded that high concentrations of AK can activate bitter taste receptors in humans [Citation36,Citation37]. The lowest concentrations of AK activating human bitter receptors TAS2R43 and TAS2R44 were 4.7 × 10−3 and 0.34 × 10−3 mol·L−1, respectively, per the bitter molecular database (https://bitterdb.agri.huji.ac.il/compound.php?id=122). When the concentrations of AK were 0.2 and 0.4%, the masking effect worsened instead of improved. This could be because the concentration of AK was high, and the AK that did not bind with BH activated the bitter receptors TAS2R43 and TAS2R44, enhancing the bitter taste. Therefore, when choosing AK for a taste masking agent, it is necessary to control the amount of AK added to avoid making the taste more bitter.
We simulated the binding sites of AK and BH with the help of microstructure analysis software, such as Autodock. We found that AK and BH could generate a new complex in the form of hydrogen bonding, which existed as a flocculent precipitate. The formation of the precipitate further enhanced the taste-masking effect of the sweetener AK and attenuated the bitter taste by reducing the contact between BH and bitter taste receptors. This study not only provides a new research idea for the taste-masking of bitter natural drugs containing alkaloid components but also provides a reference for the development of novel taste-masking agents or high-potency taste-masking agents. Such as other bitter substances, can we try to achieve the purpose of taste masking by forming new complexes with lower solubility between the taste-masking agents and bitter molecules through hydrogen bonding or other binding methods? This would allow some bitter molecules to be separated from the solution, attenuating the bitter taste of the bitter solution. Meanwhile, it was noteworthy that similar studies have found that AK reacts with BH to form a new salt with very low solubility, named berberine acesulfame (BbAs). The crystal structure was analyzed by Single Crystal X-ray Diffraction, in which there were no classical hydrogen bonds; only some weak C–H···O hydrogen bonds were used to stabilize the crystal structure [Citation19]. This is different from the structure of the complex obtained by molecular docking in this experiment. On the one hand, we speculate that this could be due to the difference in crystal structure caused by the inconsistent reaction conditions of the two experiments. On the other hand, we have some doubts about the results of BbAs speculated by Chen Guangwang [Citation19]. Is the N- of AK stable? Will there be a positive ion or proton binding with it? This is an important reason for the large differences between the two experimental results. Additionally, while we have achieved the docking of AK and BH, and the molecular docking has a low binding energy of −2.36 kcal·mol−1 (theoretically these docking results are reliable), it is still not enough to determine that this is the specific binding mode of AK and BH. In the future, we will attempt to use some other structural analysis methods to explore whether the complex AK-BH has a completely consistent crystal structure with BbAs, to further verify the conclusions obtained in the experiment.
Additionally, by analyzing the docking of AK and BH, two small molecules, and the TAS2R46, we found that the formation of the complex could compete with the TAS2R46 protein for the binding site with BH, inhibiting the activation of bitter taste receptors. This potential mechanism could also enhance the taste-masking effect of AK on BH. However, this is only speculation, and no relevant study has found the active site of TAS2R46. Further research and discussion are still needed. Similarly, we cannot ignore another possible binding mode of BH to the TAS2R46 receptors, which will not have any effect on the binding of BH to the TAS2R46 receptors. However, compared to other sweeteners, AK still has two effective taste-masking mechanisms, which help mask the bitterness of BH.
In this study, we found a new complex and explored the mechanism of its formation and potential taste-masking mechanism, but the chemical structure of the complex was not analyzed by mass spectrometry to further determine its composition ratio. More in-depth studies will be performed to explore its chemical composition and chemical properties and to optimize the additional concentration of AK. This will provide a reference for the better development of AK-related bitterness taste-masking agents.
Conclusions
AK can effectively mask the bitter taste of BH. On the one hand, the sweet taste stimulus of AK could mask the bitter taste stimulus of BH; on the other hand, AK could also generate the AK-BH with BH, which further attenuates the bitter taste during oral administration of BH solution by reducing the contact between BH and taste receptors. We also found that AK could compete with the TAS2R46 receptors for the binding site of BH, decreasing the expression of the TAS2R46 receptors to enhance the taste masking effect. When AK is added for BH taste masking, the AK concentration makes a difference. When the AK concentration is too high, it will activate some bitter taste receptors to increase the intensity of the bitterness. As such, the AK concentration should be fully considered when AK is chosen as a sweetener.
Author contributions
Ruixin Liu conceptualized and designed the research and drafted the initial manuscript. Junhan Shi, Yousong Yue, and Chenxu Li made contributions to the study of THTPM. Xiangxiang Wu, Haiyang Li, and Xinjing Gui contributed to the E-tongue evaluation and DSC analysis. Jing Yao, Lu Zhang, and Junming Wang contributed to the X-ray diffraction analysis. Haiyang Li, Xuehua Fan, and Yanli Wang contributed to the study of taste-masking mechanisms at the molecular level. Xuelin Li provided some assistance in the drafting and revision of the article. Haiyang Li, Xuehua Fan, and Xiangxiang Wu also contributed to the data analysis, drafting, and revising of the paper, so Haiyang Li, Xuehua Fan, and Xiangxiang Wu contributed equally to this work. Ruixin Liu and Junhan Shi reviewed and critically revised the manuscript, and agreed to be accountable for all aspects of the work.
Acknowledgments
Thanks to Professor Zhongyi Zhang of the Zhengzhou University of Light Industry for assisting in the DSC analysis. And thank Associate Professor Bing Xu of Beijing University of Chinese Medicine, Associate Researcher Zhaozhou Lin of Beijing Institution of Tongrentang, and Dr. Xinguang Liu of Henan University of Chinese Medicine for their linguistic assistance during the preparation of this manuscript.
Disclosure statement
No potential conflict of interest was reported by the author(s).
Additional information
Funding
References
- Zhong CP, Qian Y, Shu C, et al. Research progress in antimicrobial effects of berberine and its derivatives. Food Sci. 2013;34(7):321–325.
- Guo YY, Feng Q, Yang YX, et al. Effects of berberine on the injury of gastric mucosal epithelial cells in mice infected with Helicobacter pylori. World Chinese Med. 2021;16(18):2703–2705.
- Hu XL, Li YB, Zhang EJ, et al. Preparation and evaluation of orally disintegrating tablets containing taste-masked microcapsules of berberine hydrochloride. AAPS PharmSciTech. 2013;14(1):29–37.
- Jiang H, Zhang D, He J, et al. A novel method to mask the bitter taste of berberine hydrochloride: powder surface modification. Pharmacogn Mag. 2018;14(54):253–260.
- Kaushik D, Dureja H. Recent patents and patented technology platforms for pharmaceutical taste masking. Recent Pat Drug Deliv Formul. 2014;8(1):37–45.
- Zheng X, Wu F, Hong Y, et al. Developments in taste-masking techniques for traditional Chinese medicines. Pharmaceutics. 2018;10(3):157.
- Harmik, Sohi, Yasmin, et al. Taste masking technologies in oral pharmaceuticals: recent developments and approaches. Drug Dev Ind Pharmacy. 2004;30(5):429–448.
- Yu Y, Fan LY, Gao JD, et al. Preparation of berberine hydrochloride microcapsules and its in vitro release behavior. China Pharmacy. 2015;26(01):109–112.
- Liu RX, Wang YL, Tian LY, et al. Masking efficiency and regularity of bitterness suppressants to berberine hydrochloride based on tongue taste and electronic tongue taste. Chinese Pharm J. 2019;54(3):208–218.
- He J. Research on masking the bitterness of berberine hydrochloride and sanhuang tablets by the powder modification technology [Master]. Chengdu:Chengdu University of TCM; 2016.
- Ley JP. Masking bitter taste by molecules. Chemosens Percept. 2008;1(1):58–77.
- Li XL, Kang H, Tian LY, et al. Evaluation of different types of bitterness masking on inhibition of bitterness efficiency and law of four Chinese materia medicine decoctions of Gentianae radix et rhizoma, Sophorae flavescentis radix, Andrographis herba, and Nelumbinis plumula. Chinese Traditional and Herbal Drugs. 2018;49(22):5280–5291.
- Lin C-H, Li H-Y, Wang S-H, et al. Consumption of non-nutritive sweetener, acesulfame potassium exacerbates atherosclerosis through dysregulation of lipid metabolism in ApoE−/− mice. Nutrients. 2021;13(11):3984.
- Qurrat-Ul-Ain, Khan SA. Artificial sweeteners: safe or unsafe? J Pak Med Assoc. 2015;65(2):225–227.
- Chattopadhyay S, Raychaudhuri U, Chakraborty R. Artificial sweeteners – a review. J Food Sci Technol. 2014;51(4):611–621.
- Shahriar S, Ahsan T, Khan A, et al. Aspartame, acesulfame K and sucralose-influence on the metabolism of Escherichia coli. Metabol Open. 2020;8:100072.
- Belton K, Schaefer E, Guiney PD. A review of the environmental fate and effects of Acesulfame-Potassium. Integr Environ Assess Manage. 2020;16(4):421–437.
- Liu BB, Jian H, Tian JM, et al. Research progress and problem analysis on flavoring and taste masking technology for traditional Chinese medicine. Chinese J Exp Tradit Med Formulae. 2016;22(16):229–234.
- Wang C, Perumalla SR, Lu R, et al. Sweet berberine. Crystal Growth Des. 2016;16(2):933–939.
- Ishizaka T, Miyanaga Y, Mukai J, et al. Bitterness evaluation of medicines for pediatric use by a taste sensor. Chem Pharm Bull. 2004;52(8):943–948.
- Liu RX, Zhang XD, Zhang L, et al. Bitterness intensity prediction of berberine hydrochloride using an electronic tongue and a GA-BP neural network. Exp Ther Med. 2014;7(6):1696–1702.
- Zhang P, Zhang Y, Gui XJ, et al. Study on superposition rule of bitterness of decoction of Chinese materia medica based on traditional human taste panel method and electronic tongue method. Chinese Tradit Herbal Drugs. 2021;52(03):653–668.
- Choi Du H, Kim NA, Nam TS, et al. Evaluation of taste-masking effects of pharmaceutical sweeteners with an electronic tongue system. Drug Dev Ind Pharm. 2014;40(3):308–317.
- Drašković M, Medarević D, Aleksić I, et al. In vitro and in vivo investigation of taste-masking effectiveness of eudragit E PO as drug particle coating agent in orally disintegrating tablets. Drug Dev Ind Pharm. 2017;43(5):723–731.
- Liu RX, Zhang XF, Li XL, et al. Drug evaluation of bitterness intensity by three kinds of THTPM. Chinese J Exp Tradit Med Formulae. 2013;19(20):118–122.
- Schiffman SS, Booth BJ, Losee ML, et al. Bitterness of sweeteners as a function of concentration. Brain Res Bull. 1995;36(5):505–513.
- Kang BY, Liu RX, Meng XL, et al. A comprehensive evaluation of the masking effect of traditional Chinese medicine companion based on ISEM and FSEM. Chinese J New Drugs. 2016;25(12):1385–1390.
- Liu RX, Wang YL, Zhang Y, et al. Establishment of elimination method of outliers based on Grubbs rule and MATLAB language and its application in evaluating drug bitterness. China Pharmacy. 2019;30(2):176–182.
- Zhang W, Li G, Xiao C, et al. Mesoporous silica carrier-based composites for taste-masking of bitter drug: fabrication and palatability evaluation. AAPS PharmSciTech. 2022;23(2):75.
- Li S, Zhang Y, Khan AR, et al. Quantitative prediction of the bitterness of atomoxetine hydrochloride and taste-masked using hydroxypropyl-β-cyclodextrin: a biosensor evaluation and interaction study. Asian J Pharm Sci. 2020;15(4):492–505.
- Di Pizio A, Niv MY. Promiscuity and selectivity of bitter molecules and their receptors. Bioorg Med Chem. 2015;23(14):4082–4091.
- Li XL, Chen PJ, Gui XJ, et al. Application of electronic tongue in pattern study about bitterness inhibition by hydroxypropyl-β-cyclodextrin. Chinese Tradit Herbal Drugs. 2017;48(20):4235–4244.
- Li XL, Zhang Y, Chen PJ, et al. Study on superposition law of drug bitterness based on tongue taste evaluation and electronic tongue evaluation. China J Chinese Mater Med. 2019;44(23):5134–5142.
- Cheung LC, Nguyen M, Tang E, et al. Taste evaluation of a novel midazolam tablet for pediatric patients: in vitro drug dissolution, in vivo animal taste aversion and clinical taste perception profiles. Int J Pharm. 2018;535(1–2):194–200.
- Liu Y, Wu X, Tahara Y, et al. A quantitative method for acesulfame K using the taste sensor. Sensors. 2020;20(2):400.
- Kuhn C, Bufe B, Winnig M, et al. Bitter taste receptors for saccharin and acesulfame K. J Neurosci. 2004;24(45):10260–10265.
- Lossow K, Hübner S, Roudnitzky N, et al. Comprehensive analysis of mouse bitter taste receptors reveals different molecular receptive ranges for orthologous receptors in mice and humans. J Biol Chem. 2016;291(29):15358–15377.