ABSTRACT
Lake Eyre Basin (LEB) rivers are important but under-researched. This study integrates published and new information into a systematic geomorphological overview, subdividing the LEB into nine landscape zones: basin-margin Uplands with relatively high-energy rivers; Rocky Fringe, piedmont with short rivers and floodouts; Western Rivers, notable for neotectonism and stream capture; low-relief Northern Plains drainages and floodouts; Central Plains dunefield; Channel Country-Vertic Downs, cracking-clay interfluves and valley macrochannels; Channel Country-Stony Domes, rocky or sandy interfluves and complex macrochannels; Lakes & Dunes maintained by saline groundwater; and playas in the Mega-lakes. Zone boundary GIS datasets are available. LEB rivers are shown to be diverse and distinctive, indicating a need for locally relevant knowledge in management and monitoring. Fluvial landform suites include sand-bed rivers, macrochannels hosting coexisting anabranching and anastomosing systems in vertic floodplains, floodouts, low-angle alluvial fans, and three types of waterhole. Channels may be discontinuous or not present; floodplains can dominate flow transmission. Contributing hillslopes are heterogenous in their capacity to generate runoff, while drainage networks may have low to high degrees of integration. Management implications include the importance of in-river terrestrial ecosystems and “patchy” drainage networks, and the inapplicability of some morphometric practices.
Introduction
The Lake Eyre Basin (LEB) is a dominating feature of drylands Australia, yet its fluvial geomorphology is under-researched. This lack of local information has encouraged a practice of viewing LEB rivers in terms of information derived from perennial or otherwise non-similar systems. The baseline assumption, that all rivers are sufficiently alike to be treated similarly, is untrue. Since water-distributing landforms control drylands habitats, incorrect information hampers effective land management.
In drylands, landforms that distribute water underpin every ecosystem. Although potable water held in the larger channels and waterholes is clearly important, other landscapes store water for terrestrial ecosystems: hillslopes, plains and small creeks. Habitats are hosted within landforms formed and maintained by geomorphological processes across nested hierarchies of scale (Polvi et al., Citation2020; Thoms et al., Citation2016). It is poor strategy to monitor habitat while ignoring wider geomorphological context; it risks missing problems until they are well advanced (Larsen et al., Citation2016; Pringle & Tinley, Citation2003). Understanding local geomorphology creates better outcomes in land management, regulatory frameworks, infrastructure design, condition assessments, and planning for climate change.
Despite this, specialist geomorphology is not usually a core discipline employed in Australian drylands condition assessments (e.g. LEBSAP, Citation2008). Partly this is because Australian drylands rivers are relatively poorly documented. Drylands catchments are often remote, dangerous and expensive to work in, with few reliable transport routes. The result is a sampling bias in which drylands rivers projects are scarce and clustered around more accessible areas (McMahon & Finlayson, Citation2003; Tooth & Nanson, Citation1995). Past perceptions of drylands rivers have been dominated by studies in the steep, small catchments of northern hemisphere piedmonts (Tooth, Citation2000a), to the detriment of understanding other contexts (Nanson et al., Citation2002).
Australia’s LEB has been the subject of geomorphological research for over 50 years (Mabbutt, Citation1967), including work on Central Australian sand-bed rivers (Baker et al., Citation1983; Bourke & Pickup, Citation1999; Tooth, Citation1999) and the central playas (Amos et al., Citation2012; Croke et al., Citation1996; Waclawik et al., Citation2008). The Channel Country, especially Cooper Creek, has been a research focus since Rust (Citation1981), resolving the nature of its fluvial system (Gibling et al., Citation1998), documenting its hydrology at catchment and reach scales (Knighton & Nanson, Citation1994, Citation2001) revealing palaeoclimates and palaeohydrology (Cohen et al., Citation2010; Maroulis et al., Citation2007), and uncovering the surprising information that two styles of channel can coexist (Nanson et al., Citation1986) and mud can travel as aggregates in bedload (Rust & Nanson, Citation1989). Analyses of mud-aggregate floodplains (Fagan & Nanson, Citation2004; Maroulis & Nanson, Citation1996) and the nature of anabranching (Morón & Amos, Citation2018; Nanson and Knighton, Citation1996) have global application (Huang et al., Citation2002; Marriott & Wright, Citation2004; Wakelin-King & Amos, Citation2016). The Channel Country is therefore often dominant in geomorphological information presented to drylands river managers, including that intended to apply to the whole LEB (e.g. Kingsford, Citation2018; Kingsford et al., Citation2014; Sheldon et al., Citation2005).
However, the Channel Country is not the whole LEB. The most-researched areas (Cooper Plains and Innamincka Plains physiographic regions, sensu Pain et al., Citation2011) are only 3.5% of the Lake Eyre Basin; Cooper Creek has the highest proportion of the Channel Country’s good waterholes (Silcock, Citation2009), rather than being representative of the whole. Additionally, knowledge gaps are sometimes bridged by methodologies developed for non-drylands rivers (Finlayson & McMahon, Citation1988; and e.g. Parsons et al., Citation2004; Thoms et al., Citation2009), or based on assumptions that are inappropriate for the LEB (e.g. Sheldon et al., Citation2004). While these are reasonable interim measures, they aren’t a suitable end point; better geomorphological information is needed (McKenzie-Smith, Citation2004; Tooth & Nanson, Citation2011).
The goal of this paper is to provide better geomorphological information. A comprehensive whole-of-basin description of LEB geomorphology is presented, with a new subdivision of the LEB into landscape zones (, , Supp. Table) based on the elements that create diversity in the surface waters. Each zone’s characteristic features and dominant drivers are identified, and GIS datasets are available from the La Trobe University data repository (https://opal.latrobe.edu.au DOI 10.26181/616cc5686abd5). The landscape zones are intended to be compatible with the River Styles™ perspective (Brierley & Fryirs, Citation2005; Fryirs & Brierley, Citation2013), as a first step towards management-ready and systematic documentation of these systems. This paper’s source information integrates peer-reviewed literature, State government reports, and new data generated during this study. Its target readership is geoscientists, non-geoscience specialists, and land managers, particularly those who have not previously had access to geomorphological information.
Table 1. Landscape Zone (LZ) summary. Climate classification from Australian Bureau of Meteorology; moisture index (MI) is rainfall/potential evaporation. Adjective applied to fluvial gradient (superscript 1) is relative to the Lake Eyre Basin as a whole: very low, <0.01%, low, 0.01–0.02%; moderate, 0.03–0.08%; high, 0.09–0.14%; steep, >0.15%. LZ are listed in order of area. Lower-case “uplands” is local topography, upper case “Uplands LZ” is landscape zone
Methods
This work uses observations and concepts from subcatchment-scale geomorphological projects (Wakelin-Kin Citation2011, Citation2013, Citation2015a, Citation2015b, Citation2017), developed further in a basin-wide study. Methodology is similar to that used in regional geological mapping, which reiteratively integrates information from a variety of datasets and across scales from metres to hundreds of kilometres. A key investigative tool is that spatial relationships can be used to infer causality by tracing process relationships across place and time (location-for-time substitution, or ergodic reasoning; Fryirs et al., Citation2012). Regional data were used to characterise and map the subcatchment-scale external factors that govern rivers (topography, large landforms, surficial geology, climate), creating first-pass interpretations of broad landscape processes. During fieldwork, reach-scale geomorphological processes were illuminated by sediments, landforms, vegetation and human features and their spatial relationships. Local processes were then linked to their regional context, revealing the drivers of LEB river behaviour and allowing process interpretations to be propagated more widely.
This study used Google Earth satellite imagery, Digital Elevation Models (DEM) from the Shuttle Radar Topography Mission (SRTM) data (3-second pixel from the United States Geological Survey, 1-second smoothed from Geoscience Australia), the Atlas of Australian Soils vector dataset (www.asris.csiro.au), Water Observations from Space imagery (Geoscience Australia), Moderate Resolution Imaging Spectroradiometer (MODIS) imagery (NASA), geological (1 million scale) and topographical (10 million scale) vector datasets (Geoscience Australia), and 1:250,000 scale geological maps from Geoscience Australia and the state Geological Surveys. Coordinates are in decimal degrees and WGS84 datum (compatible with Google Earth). Climate classifications and calculations are derived from Australian Bureau of Meteorology maps (www.bom.gov.au/climate, accessed 2018–2020). All place names in this paper are locatable in the Intergovernmental Committee on Surveying and Mapping place-names gazetteer (https://placenames.fsdf.org.au; accessed July 2020). Areas were measured as 2D surfaces using GIS software on the topographic dataset in the projection specified. Gradients were measured from fluvial longitudinal profiles constructed in Excel from SRTM data, and fitted to linear trendlines. Unless otherwise indicated, photos are by Gresley Wakelin-King and maps and diagrams by Wakelin Associates Pty Ltd.
Study area
The LEB is a shallow endorheic basin extending across the Northern Territory (NT), South Australia (SA), New South Wales (NSW), and Queensland (Qld) (). Occupying 15.3% of the Australian mainland, in area (1,166,000 km2) it is more than three times the size of Germany. The LEB is low in elevation (mostly <250 m AHD) and relief, although the perimeter (7,302 km) encompasses rocky ranges to the west and south and a raised plateau to the east. Elevation extremes are ~1389 m AHD at Mt Giles (NT) and −15.6 m AHD (at Madigan Gulf, SA).
Figure 2. Regional context. (a) The Lake Eyre Basin (LEB) is located across four Australian states. Colours within the LEB outline indicate elevation ranges: blue, −15 to + 20 m AHD; grey, 20–250 m; white, 250–500 m; brown, 500–1354 m. (b) LEB major rivers (simplified), and three of the townships (red triangles). The Kati Thanda-Lake Eyre playa complex includes the basin depocentre near the southeast shores.
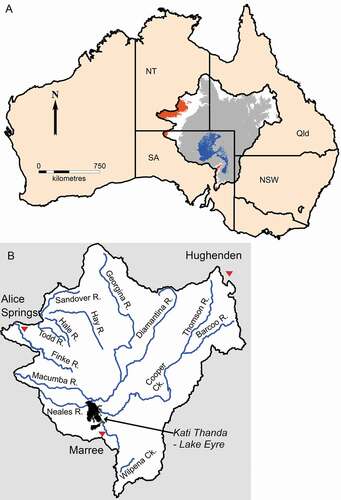
Although thinly populated, the LEB contains towns and indigenous homelands, and supports industries in the pastoral, agricultural, defence, conservation, tourism, and resources sectors. The LEB has widely recognised but loosely defined regions: Central Australia (NT), including Alice Springs township, a number of ranges, and the Finke, Todd, Hale, Hay, and Sandover rivers; the western LEB (NT and SA) including the Neales and Macumba rivers; the southern LEB, including Marree township, the northern Flinders Ranges, and numerous small rivers; and the Channel Country (Qld and SA), including the Georgina, Diamantina, Cooper, Barcoo and Thomson rivers; Hughenden township is nearby but outside the catchment divide.
Climate and palaeoclimate
Most (~75%) of the main Australian continent is drylands. Numerically defined, drylands extent differs according to the climatic parameters used (Trewin, Citation2006), and a qualitative definition is preferred here: drylands are where high evaporation rates and sparse, erratic rainfall create a moisture deficit that governs the distributions and life strategies of the biota. Fluvial processes and surficial geology are influenced by drylands’ effects on weathering, erosion and vegetation–landform relationships.
The LEB climate is hot and arid to semi-arid; winter rainfall dominates the south, summer rainfall is stronger in the north, and moisture index (MI: annual rainfall/potential evaporation) and Köppen climate class vary across the basin () (Bureau of Meteorology www.bom.gov.au; McMahon et al., Citation2008a; Trewin, Citation2006). Interannual rainfall variability is high in the south, consistent with other high latitude winter rainfall deserts, and very high in the north, consistent with low latitude summer rainfall deserts (Van Etten, Citation2009). Rainfall delivery varies from light ineffective rain to heavy downpours from tropical depressions or convective thunderstorms. The conditions most likely to bring substantial rain into the LEB are (Barlow, Citation1988; Bureau of Meteorology ibid., Trewin, Citation2006; Williams, Citation1970) -
in the south, slow-moving winter frontal systems traversing west to east,
in the north and northeast, southward extension of the monsoon trough over the Channel Country,
in the north and west, tropical depressions in the summer and north-west cloud bands in the winter, crossing the continent from northwest to southeast.
Three towns () exemplify climatic conditions across the LEB:
in the south, Marree ~200 mm average annual rainfall, ~2400 mm average annual evaporation, MI 0.08;
in the north-east, just beyond the Channel Country catchment boundary, Hughenden ~600 mm rain, ~2400 mm evaporation, MI 0.25,
in north-west, Alice Springs ~300 mm rainfall, ~3000 mm average annual evaporation, MI 0.1.
Orographic rain falls on the LEB’s western and southern uplands (Central Australia, Flinders Ranges) (McMahon et al., Citation2008a; Trewin, Citation2006). Unusually wet or dry years tend to cluster, and are influenced by longer-term weather cycles such as the El Niño Southern Oscillation and the Inter-decadal Pacific Oscillation (McMahon et al., Citation2008a; Risbey et al., Citation2009). In the recent past, particularly wet times in the LEB have occurred on a roughly 15–40 year cycle, most recently including the mid-1950s, mid-1970s, the late 1990s, and 2010–12 (McKeon et al., Citation2004; The Long Paddock, Citation2020a, Citation2020b).
During Cenozoic global glacial cycles and developing aridity, the LEB climate has oscillated through wetter and drier conditions while becoming progressively drier overall (Benbow et al., Citation1995a; Callen & Benbow, Citation1995; Habeck-Fardy & Nanson, Citation2014). In today’s landscape, the effects of this aridification include stripping of soils to produce the stony deserts from ~2-4 Ma (Fujioka et al., Citation2005), the development of the longitudinal dunefields from ~1 Ma (Craddock et al., Citation2010; Fujioka et al., Citation2009), lakes that no longer fill to their previous extent (e.g. Cohen et al., Citation2011), rivers that now carry less water than the volumes that determined their original channel and meander dimensions (Nanson et al., Citation2008; Wray, Citation2009), and the alternation of fluvial and aeolian activity producing source-bordering dunes (Cohen et al., Citation2010).
Hydrology
The LEB’s rivers drain towards the central playa lakes: Kati Thanda-Lake Eyre (comprising a large northern lake and a smaller southern lake, ), Frome, Blanche, Callabonna, and Gregory. The rivers are ephemeral, being dry for extended periods except for water retained in scattered waterholes and lakes. Flow events are rainfall-generated (McMahon et al., Citation2008b). The highly to extremely variable nature of the flow regimes (Finlayson & McMahon, Citation1988; Knighton & Nanson, Citation2001; McMahon et al., Citation2008b) arises from variable rainfall exacerbated by patchy distribution of the elements that mediate the rainfall-to-river pathway: evapotranspiration (Finlayson & McMahon, Citation1988) and capacity to generate runoff (Dunkerley, Citation2011; Osterkamp & Friedman, Citation2000; Tooth, Citation2000a). Many of the river reaches will experience in-channel flow and/or floodplain-level flow more or less yearly, but the frequency and size of flow events is different for the different catchments and landscape zones. The Channel Country rivers, which have large catchments and very long flow paths, are capable of big floods and sustained flow events with multiple flood peaks (Knighton & Nanson, Citation2001). Smaller creeks or low-order sub-catchments can be expected to have shorter and more flashy flow events. The LEB has few stream gauges, and the length of record is short. McMahon et al. Citation(2008b) have curated and analysed public-sector LEB flow records, and hydrological research from ARDIFLO and other programmes includes Costelloe (Citation2013, Citation2017) and Costelloe et al. (Citation2004, Citation2006, Citation2007). Practical aspects of Channel Country hydrology are presented for the rangeland management community in Phelps et al. (Citation2006a, Citation2006b).
A river’s flow regime includes ordinary flows, which are small and frequent (low Average Recurrence Interval, high Annual Exceedance Probability), and larger and less frequent floods (higher ARI, lower AEP). Engineered structures are designed to withstand big floods (e.g. ARI 50 years, or AEP 2%). Anything beyond ARI 100 years (1% AEP) is a very rare to extreme flow event (Ball et al., Citation2019), sometimes known as a megaflood or palaeoflood. Australian drylands rivers have experienced rainfall-generated megafloods at multi-century to millennial time scales (Baker et al., Citation1983; Bourke & Pickup, Citation1999; Cohen et al., Citation2018; Hollands et al., Citation2006; Jansen & Brierley, Citation2004; Quigley et al., Citation2007a). Megafloods strongly influence drylands landscape development: their deposited sediments persist in a relatively unmodified state, while their scoured-out valleys and plains become the framework for landforms created by more ordinary flows (Bourke, Citation1994; Bourke & Pickup, Citation1999; Shannon et al., Citation2002). Their effect on physical habitat thus influences species distribution (Crowley & Preece, Citation2019).
Megaflood landforms include lee bars, expansion bars, floodouts, undulating sandplains, scours; they can be so large compared to nearby landforms that their flood origin is hard to recognise (see Bourke & Pickup, Citation1999; Pickup, Citation1991; Tooth, Citation1999; Wakelin-King, Citation2011, Citation2013; Wakelin-King & Webb, Citation2007a).
Geology
Basement, sedimentary basins, surface sediments, weathering profiles, and tectonic history have shaped the LEB’s landscape development. This summary is drawn from State Geological Survey publications including Drexel and Preiss (Citation1995), Ahmad and Munson (Citation2013), and Jell (Citation2013).
Geological basement is a term describing the dominantly crystalline rocks that represent the area’s oldest and (usually) deepest rocks and provide the structural framework for the overlying sedimentary basins. Uplifted basement exposures in the LEB are important in defining catchment and subcatchment boundaries; they include the Arunta Block in the north-west, the Mount Isa Province in the north-east, and the Curnamona Province in the south-east. The oldest sedimentary basins in the area are the Adelaide Superbasin in the south and the Amadeus Basin in Central Australia. Around the LEB margins they occur alongside basement rocks, with folded layers exposed in spectacular strike-ridges and -valleys that influence catchment topography.
The LEB is the youngest of a succession of sedimentary basins occupying this region over geological time. The older basins had various spatial extents and their sediments are variably preserved and exposed across today’s LEB. The Proterozoic to Palaeozoic Georgina Basin and the Mesozoic Eromanga Basin are the most extensive. Their significance lies in their lithologies, some of which are parent materials for vertic soils (), and their tectonic histories, which influence drainage network geometry in the Channel Country catchments. In addition, the Eromanga Basin forms part of the Great Artesian Basin (GAB), an important source of bore water and artesian springs and seeps (Habermehl, Citation1982; Mudd, Citation2000). Other basins relevant to LEB landscapes are the Palaeozoic to Mesozoic Galilee Basin in the north-east, and the Cenozoic Tirari and Callabonna basins in the LEB’s central areas. The Cenozoic rocks and sediments with most influence on LEB geomorphology are the quartzose sandstones and conglomerates of the Glendower and Eyre formations (Palaeocene-Eocene age), the permeable sands and sandstones of the Katipiri Formation (Pleistocene age), and the Neogene and younger sediments masking older rocks, such as the Simpson Sands, the vertic soils of the Channel Country, and residual red earth soils on dissected planation surfaces.
Figure 3. Lake Eyre Basin geological context, with reference to the Vertic Downs and Stony Domes landscape zone boundaries. Left, the Georgina and Eromanga sedimentary basins are the dominant pre-Cenozoic geological units. Right, in the Vertic Downs landscape zone the vertisols are broadly located across interfluves, while in the Stony Domes landscape zone vertisol deposits outline alluvial plains. Vertic soils also occur elsewhere in the LEB.
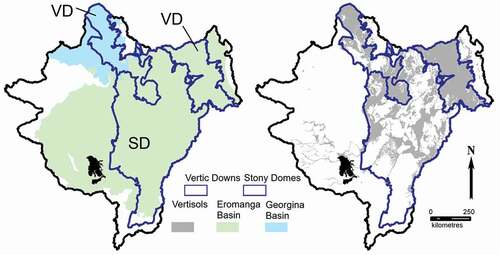
LEB landscapes have been exposed to weathering over geological time. Interactions between surface rock, groundwater and rainwater have leached, mobilised, and re-deposited minerals, creating physical changes to the rock fabric. Softening, brecciation, or replacement of rock elements creates often complex polygenetic weathering profiles (Anand, Citation2005; Anand & de Broekert, Citation2005; Benbow et al., Citation1995b; Pillans, Citation2005; Rey, Citation2013; Thiry et al., Citation2006). Regolith includes everything above fresh rock: altered rock, weathering profiles, sediments and soils. LEB regolith is widespread and distinctive, with brightly coloured scarps and hard flat gibber plains being particularly iconic. Regolith elements play important roles in LEB hydrology and landscape evolution, especially erosion-resistant silcretes, soft saprolite, high-runoff duricrusts, and low-runoff vertic soils and red-earth loams.
Tectonic influences (Habeck-Fardy & Nanson, Citation2014; Sandiford et al., Citation2020) shape many drivers of fluvial styles, including the LEB’s basin relief, depocentre location, subsidence rate, and catchment locations with respect to climate zones. At subcatchment level, smaller scale tectonic features influence sediment sources, drainage geometry, gradient, and local base level. In particular, the drainage geometry of the western rivers is markedly affected by neotectonic faulting, while dome-and-basin structures created by syn-sedimentary folding dominate Channel Country landscapes.
The sedimentary record suggests southwards migration of the LEB depocentre. Cenozoic sediments are thickest near the Qld-NT border where a Palaeogene basin was accumulating sediment (Callen et al., Citation1995; Moussavi Harami & Alexander, Citation1998; Wells & Callen, Citation1986). By the late Neogene (Tirari Formation), the basin depocentre was in what is now the southern Simpson Desert with lacustrine and paludal conditions to the north of the present Kati Thanda-Lake Eyre (Callen & Benbow, Citation1995; Tedford et al., Citation1986). In the present day, the depocentre is in Kati Thanda-Lake Eyre, close to the LEB southern margin where the Cenozoic section is relatively thin (Moussavi Harami & Alexander, Citation1998). The timing of depocentre shift, the present-day topographic asymmetry, and the orientation of erosional landscapes in the northeastern LEB (Wakelin-King & Amos, Citation2016, their ) is consistent with Schellart and Spakman’s (Citation2015) model of mantle-driven basin subsidence.
Common drylands landscape elements
Certain drylands landforms occur across the LEB, and are described here rather than repeating the information for each landscape zone. These landforms are either fluvial in nature, or are relevant to contributing hillslope hydrology.
Vertic soils and vertisols
Vertisols are clay rich, and include a proportion of swelling clays. They can be black, red, grey or brown, and exhibit strong shrink-swell behaviour, expanding and retaining moisture when wet, contracting and becoming hard when dry. Dry vertic soils may present as multiply-fragmented cracking clay soils or as a loose and dusty surface of sand- and silt-sized mud aggregates. Vertic soils are often associated with gilgai landforms: a heaved uneven ground surface centred around large deep cracks (macropores or “crabholes”). Shrink-swell behaviour during seasonal wet-dry cycles churns the soils and can push rocks up to the surface (Driessen et al., Citation2001), sometimes to be sorted into more- and less-stony microtopography (Dunkerley & Brown, Citation1995). Vertisols can form from a wide range of parent rocks under an alternate wet-dry soil water regime, in a context of impeded drainage and high temperatures (Driessen et al., Citation2001; Hubble, Citation1984). Reddish vertisols are created by long weathering, short-period wetting, and severe drying under high temperatures, while black vertisols are created with moderately abundant rainfall, alkaline parent material, and grass-dominant plant communities (Hubble, Citation1984).
Compared to the rest of the world, Australia is unusual in the extent and variety of vertic soils (Hubble, Citation1984), and they occur across the LEB. Red gilgai and stony gilgai are mostly in the LEB south-east, while the dark (black, grey, grey-brown) soils dominate the north and north-east. In the Channel Country upper catchments (especially in the Vertic Downs landscape zone), residual vertic soils occur on hillslopes and interfluves (), with characteristics determined by the underlying lithologies. Above the Georgina Basin’s carbonate rocks, there is a mixed landscape of ferruginous plains, and vertic plains and downs with ferruginous remnants (Edgoose, Citation2005). A more homogenous landscape of undulating black soil plains overlie sedimentary rocks of the Rolling Downs Group (Eromanga Basin) (Vanderstaay Citation2000a, Citation2000b). Pervasive polygonal faulting within these rocks (Ransley et al., Citation2015) produces a characteristic equant vermiform mottled appearance on satellite images (). In contrast, vertic soils in the Channel Country lower catchments are predominantly allochthonous. Their robust character permits them to survive fluvial transport (Maroulis and Nanson, Citation1996; Wakelin-King & Webb, Citation2007b), and in the Stony Domes landscape zone they commonly occur as alluvium (Fagan & Nanson, Citation2004; Gibling et al., Citation1998).
Figure 4. Part of the Thomson River macrochannel, containing a channel (far left), a waterhole, dark grey anastomosing floodways and pale grey braid-like floodplain bars. The valley margin is indistinct (dashed line), and the hillslope (right) shows mottling characteristic of Rolling Downs Group subcrop. Vertic Downs landscape zone, along the Muttaburra-Longreach road (−23.09° 144.48°), flow top to bottom.
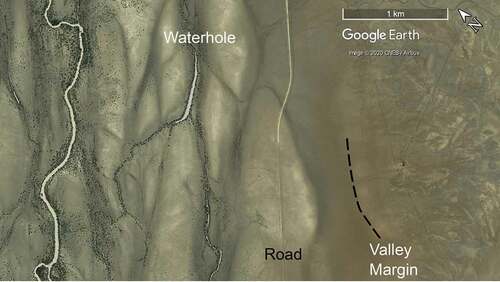
Silcrete and gibber plains
Silcretes are hard silica-rich duricrusts that occur widely across the LEB. Their key geomorphic characteristics are their capacity to protect underlying softer rocks from erosion, and the water-shedding that can significantly influence flow hydrology. Silcretes form during weathering, usually in association with either a palaeosurface or groundwater (Thiry et al., Citation2006; Ullyott & Nash, Citation2016). LEB silcretes were exposed at surface by erosion of softer overlying regolith during continental aridification 2–4 million years ago (Fujioka et al., Citation2005). They crop out as semi-planar rough layers, lumpy boulder piles, or broad areas of rounded rocks (gibber plains or stony mantles). Often, gibber occurs as desert pavement: a single layer of closely packed rocks, underlain by stone-free silty sediments and often associated with a water-repellent near-surface vesicular layer (Dunkerley, Citation2011; Laity, Citation2011).
Multithread macrochannel rivers, sand-bed rivers
In low-variability rivers, it is expected that characteristics such as channel form or floodplain inundation frequencies are relatable to predictable, regularly occurring discharge values (Croke et al., Citation2016). However, high variability rivers such as those of the LEB encompass many flow sizes that form channels, banks and floodplains; this complexity needs to be recognised and named (Croke et al., Citation2017). A macrochannel is a compound form in which smaller channels, active more frequently at smaller flows, are set within a larger channel that can accommodate larger flood discharges. Both scales of channel are part of the current flow regime, and there can be multiple inundation surfaces (Croke et al., Citation2016, Citation2017).
A multithread river is one in which multiple channels coexist. In the LEB, anabranching and anastomosing are widely-occurring multi-thread river styles, most commonly found in macrochannels (e.g. ). Both comprise multiple channels separated by stable floodplain-height islands or bars. Usage of the two terms has been inconsistent (e.g. Gibling et al., Citation1998; Habeck-Fardy & Nanson, Citation2014; Makaske, Citation2001; Nanson, Citation2013), however “anastomosing” sometimes carries a connotation of being low-energy and fine-grained (e.g. Knighton & Nanson, Citation1993; Nanson, Citation2013), whereas rivers described as “anabranching” often have channels that operate fairly independently (e.g. Kemp et al., Citation2017; Taylor, Citation1999). In the present paper, “anastomosing” denotes LEB channels that are closely interlinked and relatively small or shallow, while “anabranching” denotes channels with a degree of independence ().
Figure 5. Four examples of LEB rivers which can appear braid-like, but are not braided. (a) Small trees along the flow paths show the anastomosing channels occupying the Arckaringa Creek valley; Western Rivers landscape zone (−27.94° 134.76°), flow left to right. (b) Two anabranch channels and a waterhole amongst the floodplain bars (mid-grey) and anastomosing floodways (dark grey) of Cooper Creek (−26.97° 141.90°), Stony Domes landscape zone, flow top to bottom. (c) A sand-bed channel contains recently transported white quartz sand, stranded as flat-topped bars at the close of flow. Finke River railway bridge (−29.93° 133.64°), width of white sand = 180 m; Uplands landscape zone, flow right to left. (d) Floodways (medium grey) anastamose around braid-like bars (pale grey); Diamantina River (−24.87° 140.59°), Vertic Downs landscape zone, flow top to bottom.
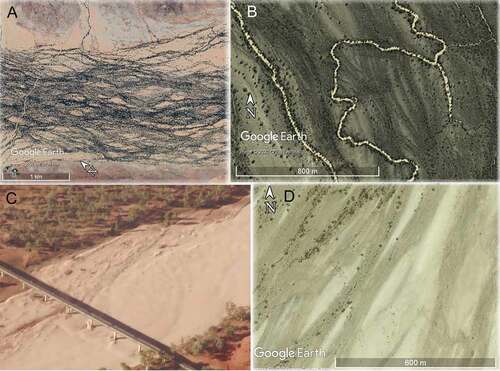
Sand-bed rivers are common in Central Australia and the Flinders Ranges. Most are single-thread and low-sinuosity where semi-confined within a valley; some are anabranching or distributary in other contexts (Bourke & Pickup, Citation1999; Tooth, Citation2000b, Citation2005; Tooth & Nanson, Citation2004). Sandy and silty floodplains commonly hold abandoned channels and flood runners. Channels are wide and shallow with well-defined banks; they carry abundant sandy and gravelly bedload. Sediment moves in pulses; flood peaks, flood routing, and reach-scale variations in flow energy determine the grain sizes under transport and the distance travelled (Bourke & Pickup, Citation1999; Mabbutt, Citation1977; Pickup et al., Citation1988; Wakelin-King, Citation2015a). In a dry sandy river, the channel floor is a palimpsest of bedforms stranded by declining stream power at the end of a flow event () (Wakelin-King & Webb, Citation2007b). The preferential preservation of waning-flow bedforms such as ripples and 2D dunes has prompted statements describing low-energy rivers (Mabbutt, Citation1977; Williams, Citation1971). However, flows can be extremely vigorous; during the 1967 floods, 1 m amplitude stationary breaking waves were observed in the Finke River (Williams, Citation1970), while in Alice Springs, an AEP 2% flood mobilised bedload to a depth of >2.5 m at the thalweg (Barlow, Citation1988).
Braided channels are a type of multithread river once considered to be prevalent in desert systems (Mabbutt, Citation1977). Although sand-bed and Channel Country rivers have been described as braided (e.g. Nanson et al., Citation1986; Williams, Citation1971), the term is generally not appropriate for the LEB. Braided rivers are multithread high-energy dynamic systems in which normal flows include active bedload transport, frequent thalweg shifts and low bars exhibiting lateral instability (Bertoldi, et al., Citation2009; Fryirs & Brierley, Citation2013; Morón et al., Citation2017). In contrast, LEB anastomosing and anabranching rivers are multithread low-energy systems; both channels and bars are stable, and there is generally little bedload transport (Wakelin-King, Citation2011, Citation2013). Their braid-like appearance () relates to the conditions under which anabranching develops (Morón et al., Citation2017). Sand-bed rivers, on the other hand, can be high-energy with active bedload transport, but are not inherently multithread: a braid-like appearance in the dry river results from floodplain topography and the final rivulets winding around bedform margins.
Waterholes
A waterhole is a landform in which water is of better quality, greater abundance, or easier access than other local landforms. Drylands waterholes preserve water after flow ceases, and their distribution and qualities are ecologically, commercially and culturally important (Duguid et al., Citation2005; Fensham et al., Citation2011; Pearson et al., Citation2020; Silcock, Citation2009). To sustain life between flow events, a refuge waterhole must hold more water than it will lose through evaporation or seepage. This depends on water depth at cease-to-flow, length of time between flows, and evaporation rate (Costelloe & Russell, Citation2014; Costelloe et al., Citation2007). Cease-to-flow depth depends on waterhole size and geomorphic context, while flow frequency and evaporation rate are externally forced factors. As a rule-of-thumb, a refuge waterhole that experiences annual flow, no spring input and ~3600 mm/y evaporation loss requires a cease-to-flow depth of >4 m (Costelloe & Russell, Citation2014). Note that the 4 m “rule” only applies to that specific context: waterholes with more frequent flow or less evaporation will require less depth, whereas a deep waterhole may cease to be a refuge if its flow regime declines in frequency or volume.
Geomorphological research on LEB riverine waterholes has focused on Channel Country rivers (Knighton & Nanson, Citation1994, Citation2000) however, similar waterholes also occur in the western LEB (Wakelin-King, Citation2011). Channel Country-type waterholes are reach-scale whole-of-channel features in which the channel is notably wider and deeper than nearby or upstream/downstream channels. They occur at all scales from minor floodplain scours (<0.2 m deep, 2–10 m wide, 5–100 m long), to large and reliable water resources (metres deep, tens of metres wide, kilometres long). Channel Country-type waterholes have semi-straight to sinuous planforms, and can be isolated channel segments or part of more continuous channels. The upstream end is formed by one or more floodways and/or channels coalescing and/or abruptly expanding. The downstream end may be tapered () or blunt in planform, may be associated with a sediment splay, and is often divided into 2–6 smaller and shallower distributaries (Knighton & Nanson, Citation2000; Morón & Amos, Citation2018; Wakelin-King, Citation2017). In longitudinal profile, the upflow end of a waterhole has an increased gradient as the flow path deepens, and the downflow end has a steep reverse slope as it shallows (Knighton & Nanson, Citation2000, their ). From limited observations, cross-section topography suggests a scoured base (Wakelin-King, Citation2011). Banks have a steep upper face () usually with a clear break of slope at the upper bank edge. The roughly level bank top is usually part of a levee or riparian ridge and often has loose, recently deposited sediment or (rarely) a narrow ridge. Bank tops are almost always densely vegetated and easily visible on satellite imagery.
Figure 6. Right-bank of a large waterhole (left) and sketched profile (right). The upper bank slope is steeper than the lower bank. After flow ceases the waterhole’s water level is transient and decreases over time. At the time of visit, water level was approximately 2.5 m below cease-to-flow level. Vegetation zones are linked to hydrology: riparian coolibah occupies bank tops and upper bank slopes, lignum is most dense near the cease-to-flow (C-t-F) depth. White oval is person-sized scale; Diamantina River (−26.261° 139.398°); clip-art from Pixabay.
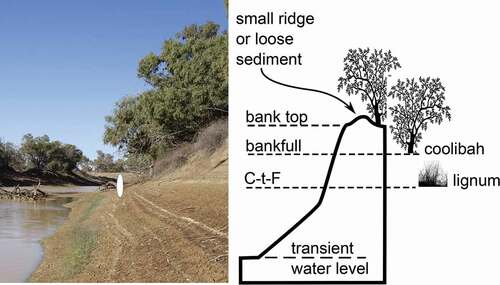
Landforms and hydrology are strongly linked in Channel Country-type waterholes (Costelloe et al., Citation2004; Wakelin-King, Citation2017). Bank tops are always elevated above cease-to-flow depth, and are sometimes elevated above flood inundation. Bankfull, the elevation at which water spills over into secondary channels or floodplains, is frequently a lower elevation than bank top. The cease-to-flow level, at which outflow stops at the close of flow, is determined by a sill somewhere in the downflow distributary channels. Banks may be breached by distributary channel offtakes located above cease-to-flow but below bank top (Wakelin-King, Citation2013, Citation2017). Channel Country waterholes have marked vertical vegetation zonation (): riparian coolibah (Eucalyptus coolabah) occur at and above maximum flood height or around bankfull, red gum (Eucalyptus camaldulensis) occur below coolibah and above lignum, and the base of the lignum is approximately at cease-to-flow (Costelloe et al., Citation2004; Costelloe & Russell, Citation2014). During “ordinary” floods (occurring every few years or so), the river valley may be inundated and flow moves over and through the riparian trees. The additional roughness in the flow path creates boundary shear stress along waterhole bed and banks (Knighton & Nanson, Citation2002, their ), probably maintaining waterhole depth and bank integrity. Many waterholes are located in what would be locally high-energy flow conditions at some flood level (Knighton & Nanson, Citation1994, Citation2000), and it has been suggested that Channel Country-type waterholes originate by macroturbulent scour during megafloods (Wakelin-King, Citation2011, Citation2015a). However, the progressive development of anabranching can also lead to channel segments with waterhole morphology (Morón et al., Citation2017; Morón & Amos, Citation2018).
It is important to recognise other kinds of riverine waterhole. In Central Australia and the Flinders Ranges, rivers cutting across rocky outcrop create narrow “gaps” in which the channel might occupy the entire width of the flow path. During floods, the confined flow has high erosional power, creating deeply scoured sections (gorge waterholes) that occupy the whole channel width. In Central Australia’s less confined intermontane plains, locally high-energy scour of the sandy channel bed creates waterholes that occupy only part of the channel width (sand-bed waterholes). Some are fixed in their location by a turbulence-producing feature (e.g. a spur of outcrop), but others change their location from flow to flow (Unmack, Citation2001; Wakelin-King, Citation2015a). Non-riverine waterbodies (e.g. springs, rockholes; see Silcock, Citation2009), sometimes called waterholes, are not reviewed here.
Channel-free flow paths: floodouts, banded vegetation, infilled valleys
A floodout is a fluvial landform in which the river channel diminishes and disappears, but the flow path continues. The term is widely used across rural Australia. Despite long-term recognition in the literature (e.g. Mabbutt, Citation1977), Australian floodout processes were not investigated until Tooth (Citation1999, Citation2005). Floodouts are created by downstream-decreasing stream power, either through transmission loss or as the flow path widens and shallows. Competency and capacity for sediment transport declines, and the channel diminishes and eventually disappears. Flow continues as shallow sheetflow across what is effectively 100% floodplain. Floodouts are favourable sites for water infiltration, sedimentation and seed deposition, creating a biological and geomorphological feedback process that maintains floodout stability (Bull, Citation1997; Wakelin-King & Webb, Citation2007a). A floodout may be at the river’s terminus (), if rainfall is never sufficient to push flows to catchment base level, or may be an unchannelised reach within a continuous flow path (a mid-creek floodout) (Bull, Citation1997; Tooth, Citation1999; Wakelin-King & Webb, Citation2007a). Mid-creek floodouts create a hiatus in the flow path’s longitudinal integration. They are promoted where a flow path alternates between confined and unconfined reaches and the river’s discharge is only sufficient to maintain a channel within confined reaches.
Figure 7. A terminal floodout, showing the main channel (flow top left to centre right) which splits and becomes distributive (arrow) a short distance upstream from channel termination. The flow path continues, and sheetflow distribution is marked by the dark tone of dense vegetation. Wilpena Creek, Rocky Fringe landscape zone (−31.50° 139.40°).
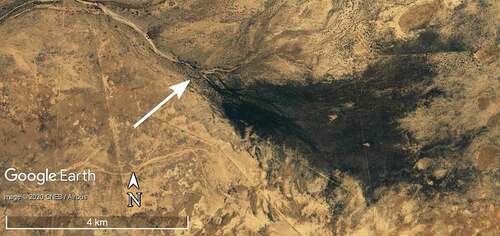
Banded vegetation is a water-harvesting landform found on low-gradient plains and hillslopes, consisting of contour-parallel patterns of vegetated bands and bare interbands (). The vegetation is the visible marker of the runoff-runon geomorphological process, in which overland sheetflow is shed by high-runoff bare ground and intercepted by low-runoff vegetated ground (Wakelin-King, Citation1999). Water-shedding and -retaining processes are governed by gradient, soil surface properties, surface roughness, and distribution of pedogenic and biotic macropores (Deblauwe et al., Citation2012; Dunkerley & Brown, Citation1995, Citation1999; Mabbutt & Fanning, Citation1987; Saco et al., Citation2007; Tongway & Ludwig, Citation1994, Citation2001; Valentin et al., Citation1999). It is most prominent in stable regolith-covered hillslopes unconnected to active fluvial systems, and occurs in a variety of plant communities (e.g. Acacia trees, chenopod scrub, Mitchell grass). More densely vegetated water lanes, where bare interbands are reduced or absent, occupy valley bottoms between banded vegetation hillslopes. In rainfall-limited areas, banded vegetation can develop through self-organisation of plants (Saco et al., Citation2007). It maximises productivity, but can be destroyed if it loses its capacity to capture runoff (Freudenberger & Hiernaux, Citation2001; Valentin and d’Herbès, Citation1999; Valentin et al., Citation1999;Wakelin-King, Citation1999).
Figure 8. Infilled valleys on regolith-blanketed hillslopes, Georgina catchment, Northern Plains landscape zone. (a) On a low-gradient relict land surface, interfluves carry banded vegetation, and the previous drainage lines are now unchanneled and evenly-vegetated water lanes (Sandover River −22.76°, 134.18°). (b) Greater vegetation density (darker image tone) shows a dendritic network of infilled valleys (near Ooratippra Creek −22.22°, 135.89°).
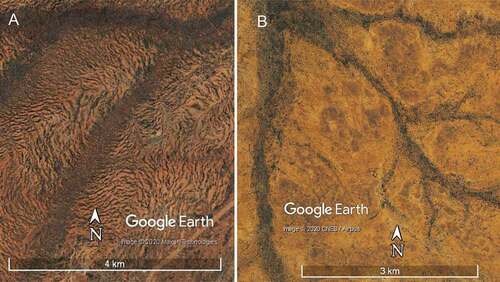
Sedimentary infill of palaeo-topography is widespread and has taken place in multiple episodes (Anand, Citation2005; De Broekert & Sandiford, Citation2005; Chan, Citation2009; Clarke, Citation2005; Fryirs & Brierley, Citation1998; Nawaz & Wasson, Citation2019; Prosser et al., Citation1994). Infilled valleys are the relics of palaeodrainage networks, now blanketed by sediment. Some are deeply buried and have no surface expression (e.g. Krapf et al., Citation2019; Magee, Citation2009), but many are more shallowly buried and visible on satellite imagery as a dendritic distribution of relatively dense vegetation (). The vegetation is responding to better availability of moisture, either because the ground surface dips gently into the buried palaeovalley, or where the underlying rock is acting as a sump for groundwater (George et al., Citation2006), or both. Buried palaeovalleys can also develop into playa lake chains by evaporative discharge of saline groundwater (Clarke, Citation2005; Mernagh, Citation2013). In the LEB, infilled valleys may be unconnected to present-day drainage, or the upslope unincised valley fill may be related topographically to downslope channels and floodplains. There is a continuum of form between unchanneled valley fill, infilled valleys that host discontinuous drainage, and wide alluvial valleys that host underfit streams.
Lake Eyre Basin landscape zones
In this study the Lake Eyre Basin is divided into nine landscape zones (, Supp. Table), providing a framework for catchment management, condition assessments and planning. Each is defined by unifying geomorphological characteristics that influence fluvial behaviour. As ever where natural systems are examined by subdivision and classification, the geographic and conceptual boundaries may be gradational or subject to further refinement. The landscape zones are:
Uplands (elevated, usually rocky hills; relatively high-energy rivers) (, brown)
Rocky Fringe (southern piedmont; moderate-energy rivers and floodouts) (, pale orange)
Western Rivers (cutting through regolith; both high- and low-energy rivers) (, pale grey)
Northern Plains (low-relief sandy and rocky country; low-energy rivers; floodouts) (, tan)
Central Plains (dunefields; interdune floodouts) (, yellow)
Channel Country: Vertic Downs (black soil country; low-energy rivers) (, pale green)
Channel Country: Stony Domes (black soil floodplains and sandy or rocky gibber uplands; the largest zone with the most diverse landscapes; dominantly very low-energy rivers) (, pink)
Lakes & Dunes (closely integrated playa lakes and compound dunes; very few rivers) (, lavender)
Mega-lakes (Kati Thanda-Lake Eyre and the playa lakes Frome, Callabonna, Blanche, Gregory; to the extent of their last palaeo-megalake footprint) (, blue).
Most of the LEB river systems extend across two or more landscape zones:
Flinders Ranges waterways start in the Uplands, extend through the Rocky Fringe, and finish in the Mega-lakes;
Cooper Creek’s main tributaries (Barcoo and Thomson rivers) arise in the Galilee Uplands, traverse the Vertic Downs, and in the Stony Domes country, they join to form Cooper Creek that then finishes in the Mega-lakes;
Diamantina River has tributaries arising in the Vertic Downs, its trunk stream flows through the Stony Domes country, then a different section of Vertic Downs, re-enters Stony Domes, then finishes in the Mega-lakes;
Georgina River has tributaries arising in the Northern Plains and the Mt. Isa Uplands, its trunk stream flows axially through the Vertic Downs before joining with the Diamantina River in the Stony Domes country, then finishes in the Mega-lakes;
Central Australian rivers arise in or near the Central Australian Uplands, or in the rocky country of the Northern Plains; most finish in the sand dunes of the Central Plains, although the Finke River skirts along the edge between the Western Rivers and the Central Plains;
the Western Rivers flow into the Mega-lakes, in some cases via short traverses through the dunefields.
In the descriptions below, Uplands (capitalised) refers to the landscape zone, and uplands (lower case) is local topography (rises, ranges, hills, flat-top hills, domes, elevated plains).
Uplands
Much of the LEB outer catchment divide is low elevation and low relief where the drainage network is expanding headwards, either cutting back into relict land surfaces (e.g. −27.90° 134.05°, near Cadney Park, SA), or competing against the drainage of an adjoining basin (e.g. −21.53° 142.03°, near Kynuna, Qld.). In contrast, five areas of the LEB rim are characterised by higher elevation and greater relief: the uplands near Mount Isa, Lake Galilee, Barrier Range, Flinders Range, and Central Australia. Past or present-day uplift has stripped sedimentary cover and regolith, exposing underlying lithologies to differential weathering, sometimes resulting in striking country with clearly expressed geological structures such as folds or faults. Rocky ranges and hillsides shed talus and colluvium into the fluvial system; high-runoff surfaces rapidly move rainfall into the steep lower-order creeks. Relative to the LEB as whole (), the Uplands downvalley gradients are high (0.09–0.14%) to very steep (>1.5%). Flow events have the potential to be flashy and high-energy, transporting sometimes large amounts of coarse bedload (sand, gravel, cobbles).
Mt Isa Uplands (425 m relief)
Rugged parallel ranges of strongly outcropping metamorphic rocks are separated by low-relief regolith surfaces and overlapped on the west and south by relatively flat-lying sedimentary rocks of the Georgina and Eromanga basins. Siliceous and iron-rich duricrusts and mottled weathering profiles occur in low hills, and flat-topped hills are common. The moisture index (MI: annual rainfall/potential evaporation) is ~0.16 and its Köppen climate zone is hot winter drought grassland /desert. Downvalley gradients are ~0.33% in lower order creeks near basement outcrop, to ~0.13% on regolith or sedimentary rocks. Drainage network geometry is lithologically controlled in basement outcrop areas and influenced by broad basin slope where the rivers traverse the sedimentary rocks.
Barrier Uplands (311 m relief)
The Barrier Ranges are fault-bounded uplift blocks of weathered basement exposed as moderate to low rocky hills and low-relief plains. A blanket of red silty and vertic regolith covers rocky plains, drapes the lower hills and forms valley fill. Downvalley gradients are as high as 1.37% in the lowest-order rocky reaches, and 0.33% elsewhere. The rocky hills and some of the silty soils are high-runoff surfaces, however elsewhere the regolith is low-runoff stony gilgai and/or banded vegetation. In the hills, creeks are semi-confined and discontinuous, with tributary confluence floodouts interrupting their longitudinal drainage integration (Wakelin-King & Webb, Citation2007a). Many waterways are heavily modified by post-European valley incision (Fanning, Citation1999). The Barrier Range climate zone is hot dry desert (MI = ~0.10).
Flinders Uplands (1089 m relief)
The Flinders Ranges are one of the most seismically active landscapes in Australia, with uplift since the Neogene creating a “young” topography (Quigley et al., Citation2007b) of rocky outcrop in high hilltops and ranges, steep rocky hillsides, talus slopes, and narrow gorges. Red silty regolith is present as valley fill or occasional plains; the plains are more dominant in areas of lower and more rounded hills. High-elevation summit surfaces, in some places crowned with Mesozoic fluvial rocks (Quigley et al., Citation2007b), demonstrate old land surfaces now uplifted. Lithologically controlled differential erosion forms striking ridges and peaks, with picturesque gorges where creeks crosscut the lithological trend. The landscape zone is narrow with central peaks, so drainage geometry is radial, creeks are relatively short (tens of kilometres) and the catchments are small. Fluvial gradients can be 4.35% in the lowest-order creeks, and 0.5–0.9% elsewhere. Drainage networks are longitudinally integrated, and the creek channels are generally continuous and closely coupled with high-runoff hillslopes. Coarse quartzose creek sediments (sand, gravel and cobbles) indicate high-energy flows. The Flinders Ranges (MI ~0.10–0.12) are in the warm summer-drought grassland climate zone and receive orographic rainfall.
Central Australian Uplands (1116 m relief)
The Central Australian Uplands is a mixed countryside of rocky ranges and hills, separated by piedmonts of shallow subcrop, alluvial fans, and wide plains of red sandy and silty regolith with low flat-topped hills of Cenozoic sedimentary rocks. The proportion and relief of rocky outcrop decreases towards the south and east. The Central Australian Uplands landscape zone is wide and its drainage networks extend for hundreds of kilometres, so catchments are large. The roughly east–west structural trend of the geology is reflected in the broad-scale distribution of ranges and plains, while at reach scale differential weathering has given pronounced topographic expression to outcrop lithologies. In contrast, higher-order trunk rivers are antecedent (Pickup et al., Citation1988) and cut across the geological trend in response to overall basin slope. These combined influences create drainage networks in which lower-order reaches in the ranges are interconnected through bedrock-driven topography, and are linked to other ranges by higher-order trunk rivers ().
Figure 9. Sand-bed rivers of the Central Australian Uplands landscape zone. Top: The trunk river flowing north to south cuts across the dominantly east-west trend of the topography, forming a gorge-type waterhole (Ellery Creek Big Hole, −23.78°, 133.07°). Bottom: A valley margin of duricrust-capped Mesozoic rock (left foreground) overlooks the white sandy channel of the Finke River (−24.85° 133.48). Vehicle for scale, on sandy floodplain (centre right).
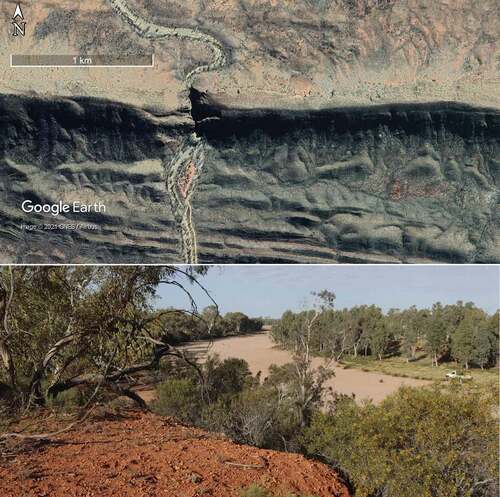
The rivers of the Central Australian Uplands are sand-bed rivers, with low-sinuosity, single-thread planforms, wide shallow channels, usually low-cohesion banks, and sandy and silty floodplains. Lower-order reaches are typical rocky upland systems, with a high drainage density of bedrock creeks, limited or no floodplains, and gradients that are steep by LEB standards (e.g. ~0.45%, Finke River; ). High-runoff hillslopes shed water and pale quartz-rich sandy and gravelly sediment into the fluvial system. The drainage network is longitudinally integrated, delivering water and sediment into major trunk systems (e.g. the Todd, Ross, Finke rivers). Where creeks cut across outcrop they are confined between rocky valley margins, sometimes forming gorge waterholes. Crosscut valleys are favourable sites for flow expansion and slackwater deposition; such sediments have been used in estimation of palaeoflood hydrology (Baker et al., Citation1983).
Exiting the rocky ranges and their associated piedmonts, the higher-order trunk rivers enter the intermontane plains. No longer confined by rocky valley margins, the river may deposit floodplain in its wider valley, experience transmission loss, and sometimes develop floodouts. For example, the Todd River floods out near Amoonguna township (−23.82° 133.96°). Despite this hiatus in longitudinal drainage integration, the Todd flow path continues and the channel re-establishes ~15 km downstream. Such intermontane reaches are likely to contain palaeoflood landforms. Alternatively, trunk rivers crossing intermontane plains may occupy the flow path of a previous and larger version of the river. For example, the Finke River near Brumby Waterhole () is underfit within the sinuous path of the palaeo-Finke; the older and larger channel is set into dune-covered Mesozoic and other sedimentary rocks. In these reaches the Finke River’s downvalley gradient is 0.09%: lower than that of lower-order reaches in rocky country, but still high for an LEB river.
Central Australia’s climate zone is hot dry grassland (MI = 0.09) and it receives orographic rainfall. Intense rainfall can be associated with summertime convective thunderstorms, and large rainfall events may result from tropical depressions (such as the weather that triggered the 1988 Alice Springs flood; Barlow, Citation1988). The climate, topography, steep gradients, high-runoff hillslopes and large and integrated drainage networks can produce high-energy flow events. Evidence of vigorous flow conditions include reach-scale scour releasing pulses of coarse bedload within the channel, large stranded 2D dunes along channel margins, and flood runners and scour pits across floodplains (Wakelin-King, Citation2015a). Quartz sands are transported down the length of the Finke River, and are currently being deposited in the Finke Floodout Complex (Wakelin-King, Citation2015a).
Galilee Uplands (606 m relief)
Along the northeastern LEB margin an elongate plateau comprises parallel sets of low sandstone hills, surrounding low-relief internally draining sandy plains and lakes Galilee, Dunn and Buchanan. The plateau’s western margin is in place bounded by a west-facing escarpment. In the north-west, an adjoining plateau slopes down from the rugged White Hills in the north. This area is unlike the other Uplands in geology and topography. The rocks exposed at surface are quartzose sedimentary rocks of the Galilee and Eromanga basins. The landscape is characterised by sandy plains with banded vegetation, low-relief regolith-mantled hills and small often poorly defined alluvial waterways. Downvalley gradients are high to steep (relative to the LEB as a whole; ) yet not as steep as the other uplands: 0.24% in the upper reaches to 0.09% in the lower plains approaching the zone boundary. Much of the higher-order drainage network is broadly parallel to the outcrop trend, but drainage lines also cross the trend. Most creeks are small and sandy, set within sandy valley fill; some are discontinuous, with channelised reaches separated by floodouts. The low-energy regolith-mantled plains above the escarpment are likely to be susceptible to erosion (Wakelin-King, Citation2015b). The climate is the mildest in the LEB (hot dry grasslands, MI = ~0.21).
Rocky Fringe
The Rocky Fringe landscape zone is a broad piedmont located downslope from the Flinders and Barrier Uplands. The piedmonts comprise subcrop, often thinly mantled by regolith, and Cenozoic rocks and sediments in alluvial fans and low-angle alluvial fans. Relative to the LEB as a whole (), gradients are very steep (0.27–0.57%) in the proximal sections and steep to moderate (0.07–0.22%) distally. Fan distribution and geometry is variable, with steeper and more voluminous fans in areas of more recent uplift (Quigley et al., Citation2007a) and consequently the Rocky Fringe landscape differs from southeast to northwest. The southeastern Rocky Fringe zone descends either from the LEB boundary, or from the relatively small and less elevated Barrier Uplands landscape zone. It has small shallow creeks draining across low-angle alluvial fans with pronounced banded vegetation slopes.
The northwestern Rocky Fringe landscape zone has short drainage networks extending radially from the Flinders Uplands. There may be two generations of alluvial fans, the older with an upper surface dissected by drainage or mantled by silty regolith with banded vegetation. Typically, as creeks exit the Flinders rangefront, they incise the older fans as far as their downslope edge. At that point the creeks become unconfined, forming the present-day low-angle fans and often terminating in a floodout (). The longer creeks (e.g. Frome Creek, near Marree) are shallow alluvial valleys. Reaches may include unchannelled flow paths, floodouts, small discontinuous channels or waterholes, and longer channels that may be single-thread, anabranching or anastomosing. Since these waterways are relatively steep and are fed by the Flinders Uplands they will occasionally carry moderately high-energy flows. However, as their catchments are relatively small and their drainage geometry prevents them from coalescing into large trunk streams, flows are unlikely to become large or sustained. Instead, the prevalence of floodouts indicates that once valleys become less confined, discharge is insufficient to maintain channels.
Channel Country-Vertic Downs
The Georgina, Diamantina, and Cooper rivers (), collectively known as the “Channel Country”, are known for their many interconnected waterways. The name dates from the late 1800s, when the area’s well-watered floodplains were undergoing European settlement (Allen, Citation1968) and displacement of the original indigenous population (Watson, Citation1998). More recently, the undulating black soil country in the north and the more stony country in the south have been divided into separate IBRA regions, Mitchell Grass Downs and Channel Country (Environment Australia, Citation2000). In the present study, the Channel Country landscape zones are differentiated on surficial lithotypes and trunk valley width, geological qualities that have created the fluvial styles. The Vertic Downs landscape zone’s distinguishing features are interfluves dominated by residual vertic soils, and trunk valleys that are moderately wide.
The Vertic Downs zone comprises two elongate belts trending NNW-SSE. The eastern belt encompasses the lower-order reaches of the Cooper and Diamantina catchments, and is a fairly homogenous landscape of undulating black soil plains underlain by Eromanga Basin sedimentary rocks. The western belt encompasses part of the central Diamantina catchment, and the lower-order and central Georgina catchment. Its landscape includes black soil plains or cracking clay downs and ferruginous plains, and it is underlain by sedimentary rocks of the Eromanga and Georgina basins ().
In the lower order reaches, dendritic drainage networks are cutting down into interfluves. In satellite view, valley margins are often indistinct; the drainage may be unchanneled or small discontinuous channels. Gradients can be relatively high (c.f. the LEB as a whole; ) in the lowest-order reaches, but are generally moderate to low. As drainage order increases, network geometry remains dendritic over the Eromanga Basin subcrop but becomes trellis over the carbonate rocks in the west. The small creeks near the Galilee Uplands escarpment carry a little sandy bedload as far as the Muttaburra confluence (Wakelin-King, Citation2015b).
In the middle-order reaches, creeks occupy shallow valleys of irregular width (<1-8 km) and poorly defined margins. They are multithread, with shallow floodways anastomosing around braid-like bars. Many floodways lack channels, but where channels occur they are usually set within floodways (). Channels may occur as one or several primary channels (slightly larger and longer than nearby elements), anastomosing channel networks, discontinuous small channels, or small waterholes. Planform varies from low to high sinuosity, and some channels show evidence of meander migration. Where the valleys are slightly wider and there are several primary channels, they are usually anabranching.
Middle-order channels are widespread and occupy thalweg positions in their valleys, therefore must be active in flow events; However, they are neither continuous nor present in every reach, so they cannot be the primary landform responsible for flow transmission. Vegetation distribution and satellite imagery indicate that floodways are commonly active during flow events; the middle-order reaches are valley macrochannels. In order of increasing flood stage, small flows are carried within channels and floodway thalwegs, larger flows occupy floodways but leave the braid-like bars emergent, and at highest flows the macrochannels are fully inundated and most of the bars are awash ().
Figure 10. Flow stages in the confluence of two Vertic Downs valley macrochannels. (a) The Thomson River (TR) is carrying flow across the whole macrochannel: most of the floodplain and all channels and floodways are submerged. The tributary Acheron Creek (AC) is carrying only small in-channel flows, at least some of which are back-fill from the Thomson River. Flow direction is arrowed, boxes show locations of B and C. (b) Acheron Creek’s in-channel flow (top left) is hydraulically dammed by floodway back-filling from the Thomson River (right) (−23.82° 143.59°). (c) Composite satellite image captures the Thomson River at two stages: sub-bankful in-channel flow (right of dashed line), and fully inundated macrochannel (left) (−23.84 143.63).
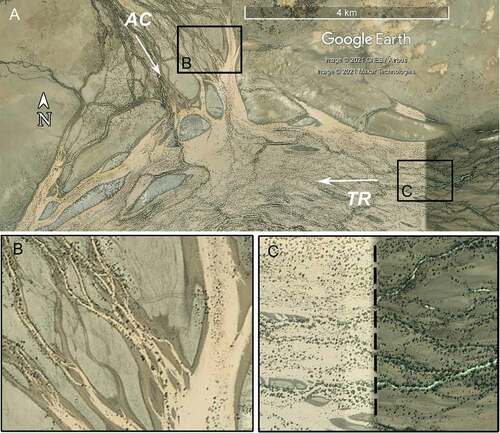
At some tributary-trunk confluences the valley widens, with downstream-divergent channel geometry indicating low-angle alluvial fans (e.g. Burke and Hamilton rivers, near Boulia; −22.90° 140.09°). Tributary-trunk confluences can also be the location of hydraulic damming as a result of asynchronous flood routing in the drainage network ().
In the highest-order trunk drainage, gradients are generally low (~0.02%) and the shallow valleys are a little wider (3–10 km wide) than upstream reaches. Fluvial landforms are larger, and the spatial relationships between them are more complex. The valley macrochannels contain floodways anastomosing around braid-like bars; in comparison with middle-order reaches, there are more and larger long channels, more anabranching channel networks, and the larger channels are less likely to be set into floodways. Channel width is variable along a flow path as channels join or divide. Channel planform may be sinuous but signs of active meandering are not common. The trunk reaches are more likely to contain waterholes large enough to be useful water resources (e.g. Longreach Waterhole, Muttaburra Broadwater), probably because they carry the gathered discharge of several tributaries. Floodplain sediments are transported vertic soils, dominantly mud aggregates but also including quartzose silt and medium-fine to fine sand, depending on upstream and local sediment sources.
Channel Country-Stony Domes
This is the largest of the LEB’s landscape zones, extending >1000 km and occupying an area larger than Germany. The Channel Country catchments pass through the zone, and Sturt Stony Desert and the Strzelecki Desert are located within it. In this study, the two Channel Country zones are differentiated based on surface lithotype and trunk valley width: the Stony Domes landscape zone interfluves are dominated by silica-rich sediments and duricrusts, and its trunk valleys can be exceptionally wide. The key landscape components are the syn-sedimentary anticlines and synclines forming the elongate NNE-SSW trending dome-and-basin topography. The tectonism is current and affects rivers and sediments, including local base levels created by uplift (Jansen et al., Citation2013), subsidence producing floodplain asymmetry (Knighton & Nanson, Citation1994), and unfilled accommodation space (Wakelin-King & Amos, Citation2016). The resulting drainage geometry links the semi-monsoonal north to the arid south. High-volume northern rainfall creates large flows able to persist over great distances despite transmission loss (Knighton & Nanson, Citation2001) and low downvalley gradients (). Regular inundation thus maintains high-value aquatic ecosystems (Costelloe, Citation2013, Citation2017; Schmarr et al., Citation2013, Citation2017; Silcock, Citation2009) and supports communities and industries (Phelps et al., Citation2006a, Citation2006b).
The Stony Domes zone is quite diverse, and the uplands, lowlands, river landforms and river behaviour are here described separately.
Stony Domes uplands
The dominant Stony Domes uplands are elevated low-relief surfaces: broad gibber plains or tabletop hills/mesas topped by duricrust. Landform margins range from steep scarps to gentle slopes. There may be several elevated levels (e.g. tabletop hills overlook gibber plains that are themselves dissected by drainage), reflecting uplift history, differential weathering across lithotypes, and differential weathering in response to area exchange between migrating subcatchment boundaries (Beeson et al., Citation2017; Willett et al., Citation2014). Undulating hillslopes of residual vertic soils also occur. Quartz-rich lithologies at surface are an important landscape component; they protect uplands from erosion and in some reaches govern valley width and local base levels. They include silcretes, and the sandstones and conglomerates of the Palaeocene-Eocene Glendower and Eyre Formations, with which the silcretes are often associated (Cook & Jell, Citation2013). Stony Domes upland areas are an intricately patterned mix of silica-rich and sometimes ferruginous duricrusts, some veneered in sand or silt, and silty or shaley and gypseous soft regolith, often displaying shrink-swell cracking. On satellite imagery, the duricrust, gibber and sand country is red-brown to dark brown; residual vertic soils are grey to blackish brown; and pale tan colours indicate where drainage networks have cut through siliceous lithotypes, revealing underlying silty and sandy regolith and moving it into fluvial transport.
Some Stony Domes uplands show relict land surfaces, especially in the more basin-marginal areas. These are typically loamy red earths, partially dissected by lower-order drainage and hosting poorly defined banded vegetation. In the more southern areas, sparse longitudinal dunes cross some stony uplands. Sandy country also occurs, associated with stony regolith but at an intermediate elevation between uplands and lowlands (e.g. sand sheets NNE and SSW from Lake Yamma Yamma, dunes west of Lake Machattie, and Benagerie Ridge dunefields east of Strzelecki Creek; Callen et al., Citation1995, their Fig. 10.28; dunes examined in Stevens, Citation1991).
Some uplands landforms and their plant communities have been influenced by diffuse GAB seeps (e.g. Sturt Stony Desert, −26.9°, 139.5°). Unusual semi-circular features in ferruginous duricrust have low centres displaying characteristics similar to the lignum swamps in the Stony Domes lowlands: dark colour, relatively dense vegetation and reticulate channel pattern. There may be nearby small gullies that appear to have more vegetation than would be expected in such low-order reaches. Upwards leakage of GAB water is known to support vegetation (Ransley & Smerdon, Citation2012), and it has been suggested (Wakelin-King, Citation2017) that stony uplands with these features result from a combination of ongoing domal uplift, a groundwater mound (Ransley & Smerdon, Citation2012), polygonal fault structures (Ransley et al., Citation2015), and hydraulic connectivity between units (Winton Formation, Eyre Formation; Radke & Ransley, Citation2020; Ransley & Smerdon, Citation2012) truncated by erosion (Alley et al., Citation2011).
Stony Domes lowlands
The Stony Domes lowlands are broad shallow valleys that host the main trunks of the Channel Country rivers. The valleys are extremely but variably wide (5–60 km), and their downvalley gradients are low (0.01–0.02%, ). They are of tectonic origin, for example, the southwest Queensland valley of Cooper Creek (Windorah township to Maapoo Waterhole) is the surface expression of the Cooper and Wilson synclines (Senior, Citation1968). It contains up to 160 m of Neogene sediments (Whitula Formation; Cook & Jell, Citation2013), and the Pleistocene Katipiri Formation that can be tens of metres thick, overlain by variable thickness of modern floodplain muds (Gibling et al., Citation1998; Maroulis et al., Citation2007; Nanson et al., Citation2008). Ongoing subsidence influences the location and depth of fluvial sediment accumulation. On satellite imagery, the striking mix of colours indicates sediment origin: pale tan to cream is silty and sandy regolith and remobilised Katipiri Formation, dark grey to black is vertic soils and their associated vegetation, and orange-brown is sand dunes.
The two exceptions to this suite of lowland landforms are Eyre Creek, in which the original south-southwesterly drainage network has been diverted by the longitudinal dune field (Wakelin-King, Citation2017), and the Strzelecki Desert between Sturt Stony Desert and Strzelecki Creek. Much of the Strzelecki lowland is occupied by the Cooper Creek Fan (Callen & Bradford, Citation1992), formed where Cooper Creek exits the Innamincka Dome. The river splits into multiple independent flow paths, including the Cooper Creek main branch, Strzelecki Creek, and the Cooper Creek north-west branch which supports the Ramsar-listed Coongie Lakes. The area is occupied by compound dunes, in which source-bordering dunes formed from alluvium were themselves the source of later longitudinal dunes (Cohen et al., Citation2010; Fitzsimmons, Bowler et al., Citation2007; Fitzsimmons, Rhodes et al., Citation2007; Stevens, Citation1991). Deflation pans commonly occur downwind of the larger compound dunes. Those located on a flow path experience lacustrine and nearshore processes during flow events, thus developing into lakes with smooth beds and shoreline berms (Wakelin-King, Citation2013). On satellite imagery, the spatial relationships between fluvial and aeolian landforms are most visible along the present-day drainage networks as dark tones of the vegetated floodplains contrasting with red-brown dunes. Palaeodrainage networks (Wakelin-King, Citation2013; Wakelin-King & Amos, Citation2016) are better seen in Digital Elevation Models where source-bordering dunes and alluvial fan topography are more clearly displayed.
In places, landforms and ecosystems of the Stony Domes lowlands are influenced by the regional unconfined aquifer, through access that plants have to the aquifer’s water (). The aquifer water is of variable salinity (Cendón et al., Citation2010; Costelloe, Citation2017), and is dominantly hosted in Pleistocene fluvial sands (Katipiri Formation and correlatives; Alley, Citation1998; Callen & Benbow, Citation1995; Nanson et al., Citation2008). The meandering rivers depositing these sands left behind a palaeotopography which is now shallowly buried by floodplain muds, but nonetheless sometimes visible in satellite imagery. Characteristic patterns and scales identify infilled channels (broad loops) and buried scroll plains (narrow parallel arcs) (Wakelin-King, Citation2017). The effect is particularly striking when crosscut by overlying present-day landforms (e.g. Eyre Creek −26.27°, 139.04°). The aquifer’s influence on vegetation growth varies according to thickness of overlying muds, aquifer salinity, proximity to surface flow (presumably reflecting low-salinity recharge), and plant species (Wakelin-King, Citation2017). The strongest expression is found in Eyre Creek, Goyder Lagoon and adjoining dunefields, and includes bare ground, lines of shrubs and trees on alluvial plains and interdunes, and orientation of reticulate channels in the lignum swamps (). The aquifer’s shallow subcrop has been identified in the Tirari Desert (Callen & Benbow, Citation1995; Tedford et al., Citation1986), Cooper Creek valley (Gibling et al., Citation1998) and floodout near Lake Kopperamanna (Wakelin-King, Citation2013), and South Australian reaches of the Diamantina and Georgina rivers (Wakelin-King, Citation2017; Wakelin-King & Amos, Citation2016). It can be traced in the Stony Domes lowlands as far upstream as the boundary with the Vertic Downs landscape zone.
Figure 11. The unconfined aquifer can influence landforms and plant communities; Goyder Lagoon, Stony Domes landscape zone. (a) Conceptual model: root penetration through the floodplain muds is influenced by palaeotopography’s effect on depth of burial. (b) Lignum swamp channel geometry is normally reticulate to dendritic (top left), but can be aligned to flow direction (arrow) or influenced by aquifer subcrop (dashed lines); −26.72° 138.99°. (c) View from dune top (foreground, below white dashed line) across lagoon plain. The boundary between vegetated (upper left) and unvegetated (upper right) plain is not a result of structures that modify grazing pressure. (d) Satellite image of the location (dot, on orange dune) and view direction (arrow) of the photo shown in (c). The unvegetated area (pale grey) is a buried channel; top left, curved traces of a buried scroll plain. Near Yelpawaralinna Waterhole (−27.12°, 138.70°); after Wakelin-King (Citation2017).
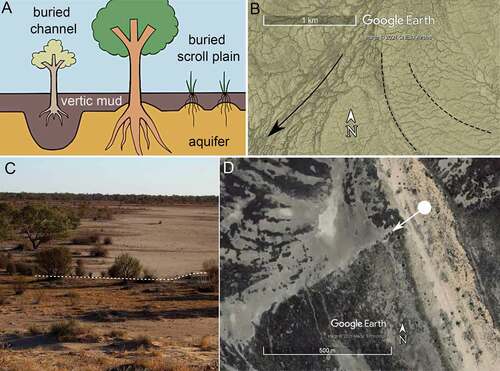
Stony Domes Rivers
In the Stony Domes uplands, dendritic and radial drainage networks of relatively high gradient (relative to the LEB as a whole; ) bedrock streams cut down into regolith. Lowest-order flow paths are typically narrow and confined within gullies or small valleys. Medium-order uplands streams occupy wider shallow valleys floored in mostly pale alluvium. Their flow paths tend to fully occupy their valley, either as anabranching channels/channel sets separated by poorly vegetated floodplain, or as more closely integrated anastomosing channels. Whether uplands reaches are anabranching or anastomosing appears to relate to width of the alluvium in floodplain storage (e.g. Farrars Creek, Diamantina catchment, −24.86° 141.47°), suggesting that the transport-limited nature of these rivers influences fluvial style. Channels are generally small, with a range of degrees of sinuosity and upstream-downstream continuity. Where uplands creeks enter the trunk (e.g. the Barcoo/Thompson or Whitula/Cooper confluences), valley-width distributary channel networks and pale sediments indicate silty and sandy low-angle alluvial fans.
The Stony Domes lowlands host the Channel Country’s trunk river valley macrochannels. Flows in the wide and low-gradient valleys () encounter irregular low-relief topography (Knighton & Nanson, Citation2000, Citation2001), obstacles such as sand dunes and floodplain bars, and roughness elements such as lignum swamps, coolibah communities, and gilgai. The fluvial landforms (e.g. channels, floodways, waterholes) are similar to those of the Vertic Downs macrochannels, however in the Stony Domes macrochannels they attain greater size, and have greater variety in their shapes and in the ways in which the elements relate to each other spatially. Generally a Channel Country-Stony Domes lowland river is a valley macrochannel containing a broad dusty multi-level floodplain of cracking clays and silty mud, in which coexisting fluvial systems include shallow floodways anastomosing around braid-like bars, anabranching networks of one to several main channels and a number of secondary and smaller channels, Channel Country-type waterholes, wide areas of unconfined shallow flow, and lignum swamps (Habeck-Fardy & Nanson, Citation2014; Knighton & Nanson, Citation2000; Nanson et al., Citation1986; Rust & Nanson, Citation1989; Wakelin-King, Citation2015a, Citation2017, inter alia). The lignum swamps (locally called lagoons) occur in backwaters and unconfined diffuse flow paths (e.g. Cooper Creek Fan, Eyre Creek, Goyder Lagoon; Wakelin-King, Citation2013, Citation2017) and have a distinctive pattern of small, closely-spaced usually reticulate channels (). They occur in areas of regular but low-energy inundation where throughflow is insufficient to maintain floodways (Fagan & Nanson, Citation2004).
The modern floodplain is transported vertic soil and quartzose silt and fine sand, deposited over sandy Pleistocene sediments (Maroulis et al., Citation2007; Nanson et al., Citation1986). The vertic soils are mud aggregates, transported fluvially and deposited from bedload; once consolidated they form dense and impermeable muds that are strong enough to sustain steep channel banks (Knighton & Nanson, Citation2000; Maroulis & Nanson, Citation1996) (). Floodplains are characterised by gilgai heave, large and deep macropores, cracking and fragmentation, especially where mud content is high. Most Stony Domes lowlands floodplains are mud-rich; however, it’s likely that the sand/silt/mud ratios vary according to contributing hillslope geology and/or local conditions of sediment deposition. There is a sandy floodplain east of Andrewilla Waterhole, sandy patches on the Goyder Lagoon (Wakelin-King, Citation2017), and sandy muds downvalley from the Thomson/Barcoo confluence (Wakelin-King, Citation2015a).
Floodplain-level flow takes place in broad shallow floodways. Their character varies from well-defined anastomosing networks around pale braid-like bars (e.g. Georgina River −23.89° 139.81°), to broad areas of unconfined flow (e.g. Eleanor Creek −26.13° 139.33°; see Wakelin-King, Citation2017). Floodways have low (≤1 m) relief, gentle bank slopes and usually rounded or indistinct bank tops (). They lack levees and usually lack riparian trees, although banks may be lined with lignum. Floodway bottoms may show gilgai or vegetation, whereas the less frequently inundated adjacent bar tops usually do not.
Channels are narrower and deeper than the floodways; their banks are clearly defined and all but the smallest have riparian trees. Larger channels usually have steeper upper banks, levees, and bank-slope and bank-top coolibah (Eucalyptus coolabah) communities (Gibling et al., Citation1998; Knighton & Nanson, Citation1994; Wakelin-King, Citation2013, Citation2017). Channel sinuosity varies; generally smaller channels can be very sinuous and larger channels tend to be less sinuous. Many sinuous channels are not currently actively meandering. Diamantina River (Birdsville to Clifton Hills outstation reaches) is unusual both in its degree of channel continuity and in the extent to which the main channel shows active meandering (Wakelin-King, Citation2017). The Stony Domes lowlands trunk rivers have more and larger Channel Country-type waterholes than other Channel Country areas. In the Cooper Creek valley, waterholes are typically 20–100 m (maximum 240 m) wide, 100 m to >20 km long and the larger waterholes are 6–10 m deep (Knighton & Nanson, Citation1994); Cullyamurra Waterhole is exceptionally deep at 18.1 m (Costelloe et al., Citation2004).
Flow in Stony Domes Rivers
Flow events in the Stony Domes lowlands are complex. Floods may have one or many flood peaks (Knighton & Nanson, Citation2001), while rainfall distribution and the contrasts between high- and low-runoff surfaces influence flood peak transmission times, contributing to tributary asynchronicity and influencing flood routing (Phelps et al., Citation2006a, Citation2006b; Wakelin-King, Citation2017). As a generalisation, channels are activated at lower flows, and floodways at higher flows; at large floods, when much of the floodplain is covered in a sheet of water, the wider macrochannel is activated. At flood stage the anabranching channels and the anastomosing floodways coexist, each operating according to its nature (Nanson et al., Citation1986). This conceptual model is straightforward but a little misleading, as it doesn’t convey the interplay between floodplain and channel. Individual channels vary greatly in size and interlink across a multi-level floodplain (Knighton & Nanson, Citation2000); many waterholes and channels are discontinuous, beginning or ending in floodways, while many small channels are located within floodways. At least some waterholes require floodplain flow as well as input from their feeder channels to account for their cross-sectional area (Knighton & Nanson, Citation2000). Stony Domes macrochannels are highly integrated longitudinally, yet individual channels are not; most channels are unlikely to flow without the involvement of floodplain flows somewhere along the path.
From the perspective of a single reach, a flow event might start with water coming downstream in the deeper or lower-elevation channels. The initial flow front passes, and water levels rise; overtopping the small channel banks, water is distributed into floodways (Phelps et al., Citation2006a) and the wider floodplain (Knighton & Nanson, Citation2000). Presumably, channels and waterholes fed only by floodways are not activated until at least some of the floodplain is inundated. As flow reaches bankfull in waterholes and larger channels, water is transmitted onto the adjacent floodplain though low-lying anabranches and bank offtake distributaries (Wakelin-King, Citation2017). If the rising limb of the flood is rapid, channels tend to scour, whereas slower rises in flood level tend to result in sediment deposition (Phelps et al., Citation2007). By the time in-channel water is high enough to overtop the bank top, the floodplain is already inundated. Floodplain landforms illustrate that at high flood stage, the floodplain is actively transmitting flow, often in places and directions that are independent of the channels (Gibling et al., Citation1998; Knighton & Nanson, Citation1994; Wakelin-King, Citation2017). Macrochannel inundation moves down-system as a valley-wide flood front (e.g. the Diamantina and Georgina rivers, January–March 2009; Wakelin-King, Citation2017). The advance of the flood front is promoted by good antecedent moisture conditions (Phelps et al., Citation2006a). Transmission losses become greater as flows enter the wide valleys downstream from tributary confluences (Knighton & Nanson, Citation2002; McMahon et al., Citation2008b), and floodwaters may be slowed by filling depleted waterholes (Knighton & Nanson, Citation2000), and abstraction into swamps, lakes or unconfined floodplain flow paths (Knighton & Nanson, Citation1994; Wakelin-King, Citation2017). Floodwaters also recharge groundwater and bank storage (Cendón et al., Citation2010; Costelloe, Citation2013, Citation2017). The flood pulse passes, and at some point the upstream water source ceases to supply new flow; transmission loss reduces flood stage, and the flood front stalls. As water level drops below the cease-to-flow point, channels become disconnected, and floodplain water infiltrates the soil, is impounded in waterholes and swamps, or drains back into the channel.
Geomorphological processes continue during the river’s no-flow phase. Evaporative pumping brings salts into the soil’s capillary zone, influencing vegetation distribution. Soil desiccation maintains gilgai landforms (Driessen et al., Citation2001; Fagan & Nanson, Citation2004), including macropores that are influential in groundwater recharge (Costelloe et al., Citation2009). Importantly, the no-flow phase allows substantial vegetation to grow in the flow path, creating roughness elements () that affect stream energy, flood routing, sedimentation patterns, and landform evolution (Corenblit et al., Citation2009; Graeme & Dunkerley, Citation1993; Nanson et al., Citation2002).
Figure 12. Even during times of no-flow, drylands rivers are working systems. (a) Dense clumps of lignum grow around shallow remnant puddles, downflow from a small waterhole’s distributary terminus. They will be roughness elements during flooding. Goyder Lagoon, Stony Domes landscape zone (−26.604°, 139.158°); 1.8 m person for scale. (b) Small plants benefit from moisture brought to near-surface by a coolibah tree’s roots. Ungrazed interdune, Lakes and Dunes landscape zone (−27.75°, 138.20°).
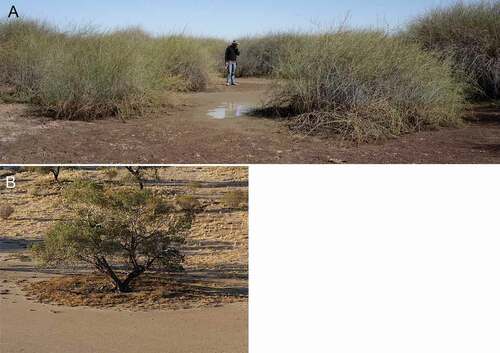
Although flow has ceased, the ecological work of the river continues. Aquatic biota shelter in the waterholes, from whence they will repopulate the floodplains and channels when next connected by floods (Bunn et al., Citation2006; McNeil et al., Citation2011, Citation2015; Schmarr et al., Citation2017). Non-waterhole reaches will have access to water in riverine soil and sediments, supporting growth and reproduction in the terrestrial plants and their associated ants, reptiles, birds, and mammals. Although biological productivity declines the longer the dry spell lasts, it still continues and the ecosystems’ resilience is due to the biota’s adaptation to flood/drought fluctuations (Bunn et al., Citation2006; Colloff & Baldwin, Citation2010). A particular adaptation is that of the keystone tree species Eucalyptus coolibah, which has a dimorphic root structure with the capacity to switch between shallow soil moisture stores and deeper groundwater stores. This nurtures both the tree and the biota beneath its canopy () (Costelloe, Citation2017; Gillen, Citation2017).
Western Rivers
The southwestern LEB is an erosion-dominated landscape hosting eastward-flowing rivers: the Macumba and Finke catchments exit to the Central Plains, and the Neales and Anna Creek catchments terminate in the Mega-lakes. Proterozoic metamorphic and sedimentary rocks crop out in the west as low hills amongst red-earth plains, and in the centre as the uplift blocks of the Peake and Denison Ranges. However, the area is dominated by Eromanga Basin sedimentary rocks, including GAB aquifers and aquitards, and Cenozoic sedimentary deposits. Surface expression of geological faulting includes uplift of the Peake and Denison Ranges, and Pleistocene disruption of drainage geometry (Waclawik et al., Citation2008). Faults influence location and output of GAB springs and seeps (Habermehl, Citation1982; Mudd, Citation2000), including the heritage-listed Witjira-Dalhousie Springs complex. The Western Rivers landscape zone also has older drainage networks now presenting as infilled valleys, and the sand-bed Finke River passes through as it traverses from the Central Australian Uplands to the Finke River Floodout Complex in the Central Plains landscape zone.
In the Neales catchment, strongly developed weathering profiles produce a varied and colourful landscape. Higher-elevation flat plains of gibber, often as desert pavement, are edged by steep slopes and scarps and surrounded by lower irregular stony and shaley rises. In some areas sand sheets with infilled drainage lines occupy low-relief sloping plains between stony country. River catchments, extending headwards into relict land surfaces outside the LEB, cut into silicious duricrusts, and a down-section traverse encompasses all levels of the weathering profile. Blackish-red maghemite pebbles (“buckshot gravel”; see Clarke & Chenoweth, Citation1995) are scattered on the uneroded land surface near the catchment boundary; exposures at decreasing elevation reveal nodular and massive silcrete or the equivalent in gibber plain, and then decreasing degrees of silicification to leached saprolith. The duricrusts and gibber plains are resistant to erosion, but the underlying saprolith is soft.
Lower- and middle-order reaches of the Neales catchment have contrasting river styles in juxtaposition. At higher elevations, low-relief gibber plains in wide shallow basins (e.g. the upper Neales River, ) undergo broad-scale slow downwearing in dendritic drainage networks. Topographically below (but occupying a similar place in the drainage network’s stream ordering), some creeks have cut deeply into the weathering profile, exposing soft rock. Scarp retreat undercuts protective hilltop cappings and produces elongate steep-sided valleys (e.g. Arckaringa Creek, ). All the subcatchments are actively expanding and cutting back into each other; where these two types of landscape are juxtaposed, conditions are favourable for stream capture. Stream capture following tectonic drainage network disruption can result in in situ development of high-elevation low-relief surfaces (Beeson et al., Citation2017; Willett et al., Citation2014), and that is likely to be a factor here.
Figure 13. The Neales catchment (Western Rivers landscape zone). (a) Looking NNE across the Arckaringa Creek macrochannel towards the elevated low-relief gibber plain that carries the Neales River lower-order reaches. The trees indicate the extent of the macrochannel, white dashed line is the floodplain-hillslope boundary. Photo point −27.75° 134.60°. (b) Topographic profile of the landscape shown in (a). AC, Arckaringa Creek; NN and SS, the north and south branches of the upper Neales River; arrow indicates photo point; elevation range 180–260 m; width of profile 25 km. (c) Broad diffuse flow paths and discontinuous channel segments in middle-order reaches, upper Neales River (−27.59° 134.78). White dashed lines are floodplain-hillslope boundaries.
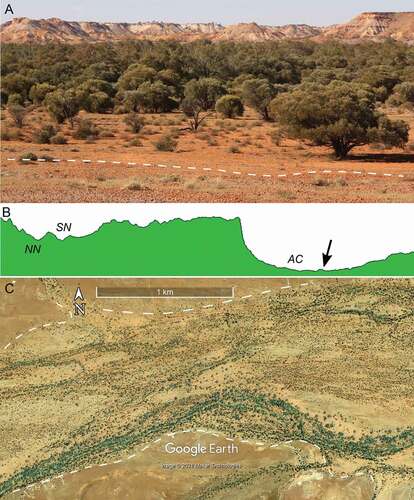
The lower- and most of the middle-order reaches are bedrock streams, in valleys carrying a thin veneer of alluvium. Valley macrochannels contain anastomosing small channels (), or broad multithread flow paths containing discontinuous channel segments, isolated waterholes, and floodways (). Small anabranching channels occur in places but are a minor component. The distribution of scours, shadow bars and vegetation across the macrochannel floodplains indicates that flows regularly occupy the full valley width.
The middle-order Neales catchment drainage networks are confined where they pass through the Peake and Denison Ranges, and large waterholes are associated with the narrowed flow paths. The main tributaries (Neales River and Peake Creek) join to form the Neales trunk valley, which extends ~ 40 km before dividing into distributary channels at the apex of the Neales Delta. The fluvial style is anastomosing networks in valley macrochannels, except three places where the Neales River is dominated by a single-thread continuous channel: downflow from the Ockenden Creek confluence, extending up-slope from Tardetakarrina Waterhole, and downflow from the Wood Duck Creek confluence. Of these, the first two are associated with propagating gully networks and recently desiccated floodplain vegetation, and are likely to be post-European valley-floor incision (Wakelin-King, Citation2011).
Sediment supply and stream order influence the Neales catchment fluvial character. Lower-order reaches in scarp-retreat valleys can be steep and fed by high-runoff surfaces, and the channels carry abundant coarse sediment in active bedload transport. Pulses of silty and sandy sediment become stranded where the narrow valleys widen in the medium-order reaches, creating a broad area of shallow alluvium in which the fluvial style becomes less anastomosing and more anabranching. In contrast, low and medium order gibber plains do not appear to have coarse sediment under active transport, waterholes have scoured bases, and sandy and silty sediments are deposited as floodplain-level shadow bars, all suggesting finer sediment supply transported in suspension during high flows. The majority of river reaches are dominated by floodplain flow. In reaches with clusters of waterholes (e.g. −27.58° 135.44°), flow moves from floodplain to waterhole and back out to the floodplain.
Northern Plains
The Northern Plains landscape zone lies between the Central Australian Uplands and the low erosional plains of the Vertic Downs landscape zone. Its overall character is sandy pediments with relict and discontinuous drainage and low-runoff contributing surfaces. Elevated areas in the west and southeast show planated and weathered outcrop with lithologically controlled and dendritic drainage etched into the plantation surfaces, or shallow subcrop covered by banded vegetation red-earth plains () or other relict land surfaces. Infilled valleys are common; many are parallel to or connect to present-day drainage. Sandsheets and stony sandplains occupy the central and northern areas; sand thickness varies from being thick enough to conceal underlying surfaces, to a thin veneer over outcrop and through which pre-existing drainage networks may be traced. The zone’s watercourses are the Bundy and Sandover rivers and Ooratippra Creek, which flow east towards the Georgina River; the Plenty and Hay rivers and Marqua Creek, which flow southeast into the Central Plains; and the headwaters of the culturally important (Watson, Citation1983) Mulligan River.
The Bundey–Sandover–Ooratipra system is the major drainage network. Tributary channels are generally confined to semi-confined in narrow valleys, becoming anabranching where the valleys are wider. In higher-order reaches, the rivers are well-defined straight to sinuous sand-bed channels, carrying quartzose sandy and gravelly bedload. Long ridges separate in-channel flow paths in a characteristic form of anabranching (Tooth & Nanson, Citation2004). Rivers emerging from valley confinement become wide and shallow; the channels become distributive before flooding out as sandy splays () (Tooth, Citation1999, Citation2005). Large floodouts occur at the Sandover-Bundey confluence, between Ooratippra Creek and the Sandover-Bundey river, and at the Bundey River terminus, with smaller floodouts at tributary confluences elsewhere.
Figure 14. A channel’s active terminal splay in a sand-bed river floodout (centre); the left-bank branch is an abandoned splay. Oblique view looking downflow, Sandover River, Northern Plains landscape zone (−21.80° 135.31°; same location as Tooth, Citation2005, his Fig. 4A).
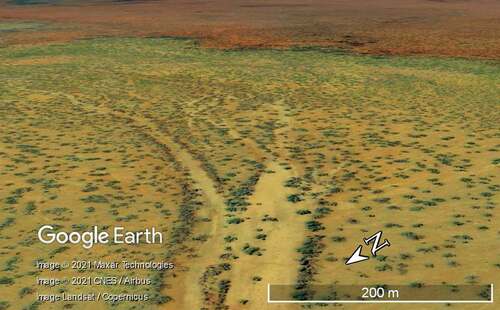
The drainage geometry, topography and palaeodrainage traces indicate that the Bundey–Sandover–Ooratipra catchment was once integrated with the Georgina River. However, in the present day the system has insufficient stream power to maintain channels that far. Since the valleys have moderate-to-high downvalley gradients (relative to the LEB as a whole, ), it is likely that low stream power is a result of low discharge, resulting from both low-runoff contributing surfaces, and the interception of flow by floodouts. Consequently, higher-order reaches in the sandplains are not receiving floods sufficiently large to overcome transmission loss.
Central Plains
The Central Plains landscape zone is currently dominated by the Simpson Desert sand sheets and dunefield. The plains slope evenly from the Central Australian Uplands southeast towards the Lakes & Dunes. The area is best known for its quartzose longitudinal dunes characterised by great length, regular spacing and narrow crests (Wasson et al., Citation1988). Closer examination reveals the Simpson Desert sands have unexpected variety of crest type, dune orientation and spacing (Hesse, Citation2010, Citation2011). The red/brown colour arises from the underlying weathered alluvium or piedmont from which the sands were derived (Callen & Benbow, Citation1995; Craddock et al., Citation2010; Hesse, Citation2010; Pell et al., Citation2000; and similarly; Fitzsimmons, Citation2007). Sand cover of variable thickness extends across the Central Plain. Dune height (interdune to crest) ranges from ~10 m to ~40 m, but the sand depths beneath the interdunes are largely unknown; up to 35 m thickness of sand is reported from bore data (Craddock et al., Citation2010; Wopfner & Twidale, Citation1967). Sand cover is visibly thinnest along the higher-elevation northwest area, where underlying rocks are poorly exposed or shallowly subcropping, and the southeast, where the effect of the regional aquifer’s buried topography is visible in the interdunes (Wakelin-King, Citation2017; Wakelin-King & Amos, Citation2016).
Rivers enter the Central Plains from the west, north and east. Downslope gradients are not particularly low relative to the LEB as a whole (); however, low flow volumes prohibit rivers from crossing the plains. All diminish in size with distance downstream, and eventually flood out in the dunefields, supporting local ecosystems. Past rivers have built low-angled alluvial fans out onto the plain (visible in DEM); previous flow paths outlining distributive drainage geometry are indicated by sand colour and dune morphology (; and see Hollands et al., Citation2006). Scattered small source-bordering dunes also show palaeodrainage geometry. For rivers entering from the north, dune orientation is approximately the same as downslope direction; flow paths are generally long, narrow, and follow interdune corridors unless blocked by other landforms (, and see Tooth, Citation1999). In contrast, the Finke and Macumba rivers and the Dalhousie Springs outflow enter from the west. Dune orientations are at a high angle to northerly or easterly downfan flow directions, so drainage networks are persistently deflected southwards.
Figure 15. Rivers exiting the Central Australian Uplands enter the Simpson Desert dunefield but do not cross it. The Hale River floodout is wide because its flow path is impeded by outcrop (dashed white line); the Todd River floods out in narrow interdunes. Dunes are red-brown, paler sand (asterisks) denotes old flood alluvium, dense vegetation along flow paths is dark grey to black, double-headed arrows indicate dune trends, flow is towards SSE; Central Plains landscape zone (−24.43° 135.60°).
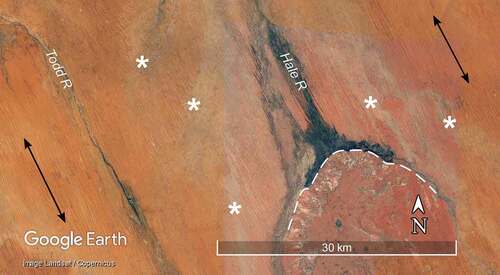
Three influences create the present distinctive landscape. Tectonism has developed the open and broadly planar topography, the evenness of which may contribute to the dune crest consistency over such a large area. Secondly, weathering of the parent lithologies has imparted rich colour to the sand grains. Finally, Quaternary aridification has both decreased flow volumes and created a dunefield from piedmonts and alluvial plains. As a consequence, the fluvial topography that once spanned the Central Plains (Craddock et al., Citation2010) is now concealed beneath the sand, and rivers that used to cross the plains have been deflected from their paths by dunes and reduced to nothing by transmission loss.
Lakes & Dunes
This landscape zone is located in the Tirari and Simpson Deserts and consists of mingled playas and dunefield. The playas are usually elongate and range in size from 0.5 to 45 km in length; many are interconnected. Compound dunes are emplaced to the north and east of playas: basal dunes trending W-E to NNW-SSE, overprinted by the more visually prominent NNW-SSE longitudinal dunes. As with elsewhere in the LEB (Cohen et al., Citation2010; Maroulis et al., Citation2007), the original landscape will have been rivers developing source-bordering dunes from seasonally exposed sandy alluvium. Two distributary branches of the Diamantina River extend through the landscape zone: Warburton River and Kallakoopah Creek. They interconnect across the upslope 50 km of the zone, after which they are hydrologically independent until their entry into the Mega-lakes. The modern sediments and landforms lie on, or are set into, the flat topography of past paludal and lacustrine depositional environments (Plio-Pleistocene centrally, and mid- to late Pleistocene closer to Kati Thanda-Lake Eyre).
The number and size of the playas results from the interaction between the regional unconfined aquifer’s capillary zone, the ground surface, and past fluvial landforms. The aquifer is saline, shallow, and increasingly close to the ground surface (Costelloe, Citation2017) as it slopes towards the LEB depocentre. The ground surface is very low-relief: the ~30 m elevation difference in interdunes 300 km apart is less than the elevation difference (interdune to crest) within many playa-dune sets. The palaeodrainage networks oriented towards those earlier lakes are now represented by playa chains and compound dunes (Callen & Benbow, Citation1995).
In an arid setting, precipitation, deflation and evaporative pumping of the groundwater leads to dynamic evolution of low-lying landforms into playas (Mernagh, Citation2013). Under certain circumstances of groundwater salinity and depth, intrasedimentary evaporites will be precipitated from the capillary fringe, fracturing surface sediments into easily deflated pellets (Bowler, Citation1983; Teller et al., Citation1982). The lowest-elevation landforms (lake beds, valley bottoms, interdunes) are closest to the capillary fringe and most vulnerable to deflation. Their deflated sediment will contribute to compound dune development (Cohen et al., Citation2010; Fitzsimmons, Citation2007; Fitzsimmons et al., Citation2009; Stevens, Citation1991; Wells & Callen, Citation1986), while the process of sediment fracturing and removal increases the landforms’ exposure to the capillary fringe.
The conditions under which this process occurs are narrow: if the groundwater is too deep, the fractured sediments remain buried; if too shallow, the ground surface is either wet or covered in salt crust. The Lakes and Dunes landscape zone is unusual in the area across which the groundwater and ground surface are in the effective spatial relationships for this process to occur. Differences in interdune elevation (in response to local conditions; see Stevens, Citation1991) mean that some are above the influence of the saline groundwater; they become claypans that collect rainfall and support local ecosystems.
Warburton River and Kallakoopah Creek are too small and the dunes are too big to allow the modern rivers to develop a straightforward path towards Kati Thanda-Lake Eyre. Instead, they occupy the existing palaeodrainage topography, pursuing independent paths in a zigzag course towards the Mega-lakes landscape zone. On a reach scale, valley context varies between wide playas, narrow palaeodrainages filling with modern alluvium, and palaeodrainages where channels are incising into older formations. Diverse valley contexts encompass variations in river confinement and local downvalley gradient (Wakelin-King, Citation2017), leading to reach-scale planform variety. The rivers can carry abundant white sandy and gypseous bedload, and their fluvial style is often aggrading and avulsive with small mobile channels (Wakelin-King, Citation2017).
Mega-lakes
Kati Thanda-Lake Eyre, Australia’s largest playa lake, is linked to a chain of smaller playas (lakes Frome, Callabonna, Blanche, and Gregory) which lie in an arc around the rocky fringe of the Flinders Uplands. During previous wetter climates they were permanent lakes, with shorelines extending beyond their present locations; the smaller playas were coalesced into Lake Mega-Frome which at times drained towards Lake Mega-Eyre via a shallow broad swale (Cohen et al., Citation2011, Citation2012; DeVogel et al., Citation2004; Nanson et al., Citation1998). The multiple filling and drying histories of the large palaeolakes are complex and not necessarily synchronous, and the last time the two were hydrologically connected was ~47 ka (Cohen et al., Citation2012). In the present day this area is arid (); the lakes are usually dry and only fill partially during modern flood events.
The Mega-lakes landscape zone is here defined on the basis of ~125 ka palaeolake shorelines (Leon & Cohen, Citation2012; Nanson et al., Citation1998), that is ~+10 m AHD around Kati Thanda-Lake Eyre, +16 to +18 m AHD around lakes Frome, Callabonna, Blanche, and Gregory, and equivalents along the waterways that linked them. Kati Thanda-Lake Eyre contains Australia’s lowest point (–15.6 m AHD; Leon & Cohen, Citation2012), and this technically represents today’s base level for LEB rivers. However, only some of the LEB rivers extend as far as the playas, and for those that do, the functional base level is today’s shoreline (between −5 and −10 m AHD in Kati Thanda-Lake Eyre, and ~2 m AHD in Lake Blanche). This is the point at which landforms reflect nearshore or lacustrine conditions, rather than a fluvial setting.
As basin depocentre, the Mega-lakes zone receives water and accumulates sediment. Its most visible characteristic is the lake floors, which are composed of clays, evaporites and some organic sediments in a distribution related to the very low-relief topography (Callen & Benbow, Citation1995; Dulhunty, Citation1986). Small irregular waterways link the Mega-Frome lakes, and Kati Thanda-Lake Eyre has two broad shallow swales (the Warburton and Kalaweerina grooves) that transmit water from the Macumba and Diamantina catchments down to the central and southern parts of the lake, largely bypassing the north.
Kallakoopah Creek, Warburton Creek, and the Macumba River approach Kati Thanda-Lake Eyre from the north, east and west across an area of relict palaeolake floor now overprinted by dunes. In the present day, they meet in an area that would once have been a shallow bay; the downvalley gradient is effectively zero and flow paths are disorganised. Cooper Creek approaches Kati Thanda-Lake Eyre from the east via Lake Killamperpunna and a floodout near Lake Kopperamanna, an area with an effectively zero gradient. Cooper Creek’s channel re-establishes at the downvalley end of the floodout, and the lower Cooper Creek cuts across the dunefield as a mobile sandy channel and discontinuous sandy floodplain underfit within the sinuous pathway of an older larger creek.
Creeks entering the lakes’ west or south are characterised by river-dominated constructional features such as deltas and terminal splays (Croke et al., Citation1996; Fisher et al., Citation2008), filled or partially filled embayments (Amos et al., Citation2012) and low-angle alluvial fans, whereas on the east and northeast the creek outflows are diverted behind an apparently wave- and aeolian-dominated shoreline (beaches or platforms, low cliffs, and beach ridges or source-bordering dunes). On the north and east of Kati Thanda-Lake Eyre, only the Diamantina catchment’s outlets are currently emplacing sediment beyond the shoreline. This is probably due to the Diamantina River’s proportionally greater flow volume (Habeck-Fardy & Nanson, Citation2014), and the local supply of remobilised Pleistocene sands from the lower reaches (Wakelin-King, Citation2017).
Discussion
Drivers of LEB fluvial Styles
The determinants of LEB fluvial style are gradient, runoff, discharge, flow regime variability in space and time, bank strength, roughness in the flow path, valley confinement and type and amount of sediment load, all ultimately arising from geology and climate. In the LEB, the different expressions and combinations of these factors are the drivers of the LEB’s landscape zones and fluvial styles.
Downvalley and downflow gradient
The LEB’s downvalley gradients are generally low (less that 0.08%), and in some places even flat. Consequently, low-energy flow is a recurring theme, and many LEB rivers show little or no present-day inter-reach bedload transport. In the lowest-gradient areas, local landform topography can have a stronger influence on flow direction than overall downvalley gradient. Such conditions promote channel avulsion (e.g. Warburton and Kallakoopah creeks) and complex flow routing (e.g. Burt and Pelican waterholes) (Diamantina catchment; Wakelin-King, Citation2017).
However, moderate-to-steep gradients (0.1–4.3%) are found in areas of uplift (e.g. the Central Australian Uplands landscape zone), or where scarp retreat is taking place in breakaway country (e.g. the Western Rivers landscape zone). These areas usually feature high-runoff hillslopes, and bedrock streams with close hillslope-channel coupling. High-energy flows can be the result, with transport of coarse bedload, or of high volumes of bedload. This is especially the case in Central Australia and the Flinders Ranges, which rise from the surrounding plains and intercept westward-moving weather bands, making them likely places for intense convective thunderstorms.
At a sub-reach scale, reverse gradients (uphill in the downflow direction) are a common feature at the downflow ends of waterholes. Indeed, since waterholes retain water at the close of flow and are deeper than nearby water-carrying landforms, a reverse gradient at the downslope end is a defining feature of waterholes.
Flow variability and the relative importance of floodplains
The rainfall across Australia’s drylands is sparse, unpredictable in timing and location, and varies in quantity from almost nothing to short intense falls or extended voluminous rain events. The runoff quality of contributing hillslopes varies between extremes of very high runoff (e.g. gibber) to little or no runoff (e.g. dunes, or banded vegetation). The spatial distribution of hillslope runoff qualities is irregular, from broad (e.g. the Central Plains dunefields), to a closely spaced mosaic (e.g. parts of the Stony Domes country). Once in fluvial transport, surface waters experience transmission loss, and the degree to which flow is reduced and the point at which flow disappears depends on the size of the initial flood, and the geology and vegetation through which it flows. Both hillslope runoff and flow transmission loss may be greater or lesser according to the soil’s antecedent moisture, which itself depends on the patchy mosaic of rainfall. All these factors contribute to extremely variable flow regimes. A flow event may occur at any scale, and may start and finish almost anywhere within the drainage network.
An important result of this variability is that it blurs the functional distinction between channel and floodplain. In a low-variability perennial river under normal flow conditions, most flow takes place in the channel; floodplains are not essential to water and sediment transport. In contrast, drylands floodplains can carry flows of significant volume or stream power, and in some cases are the only continuous flow path. In the Channel Country, there is no one “channel forming discharge”: channelised flow takes place in many different forms and elevations, from deep waterholes to floodplain-level shallow floodways. The floodplain is an integral part of the flow path, an active transmitter of flow not a passive recipient of floodwaters. In the Channel Country-Stony Domes landscape zone, there are many places where downstream waterholes and channels rely on upstream floodplain-level flow, and continuous channels are the exception not the rule. In the Western Rivers landscape zone, most reaches are dominated by floodplain-level flow, or are anastomosing channels so shallow that the floodplain would be involved in any flow larger than a trickle. The Finke River (Central Australian Uplands and Western Rivers landscape zones) apparently has a more straightforward delineation between channel and floodplain; satellite imagery and on-ground experience show a visual distinction between pale channel sands and darker vegetated floodplain (). However, spatial relationships between channel types, variety of bank types, and floodplain sedimentation and scour (Wakelin-King, Citation2015a: and accompanying text) indicate a very active role for floodplain flow during flood stages. The Finke River channel is differentiated from its floodplain by being the sediment transport route, not the transmission of flow.
In high-variability rivers, the channel:floodplain distinction is also blurred ecologically. Aquatic organisms are not confined to channels, and channels and flow paths often contain terrestrial ecosystems. Flood cycles link channel and floodplain biota laterally and longitudinally, watering terrestrial ecosystems, bringing organics into aquatic habitats, providing pathways for feeding, spawning and migration of aquatic biota, and supporting boom-and-bust ecosystems (Bunn et al., Citation2006; Leigh et al., Citation2010; McNeil, et al., Citation2011, Citation2015).
Flow size and drainage networks
The various LEB catchments have characteristic flow sizes, from small floods with local effects to large floods that travel long distances. Climate is certainly a factor, for example, the Channel Country rivers (known for large and far-travelling floods) arise in the least harsh climate zones (Mount Isa Uplands, moisture index 0.16; Galilee Uplands, MI = 0.21), whereas the Flinders Uplands (not known for long-lasting flow events) have a more harsh climate (MI = 0.1–0.12). However, Central Australia, despite having the harshest climate of all the Uplands (MI = 0.09), nonetheless supplies long rivers with large and far-travelling floods. In comparison, the Barrier Range with a similar climate (MI = 0.1) supplies relatively short discontinuous rivers (see Wakelin-King & Webb, Citation2007a). Therefore, other factors must be involved: drainage network geometry and degree of integration.
Drainage network geometry determines whether runoff in a flow path has the opportunity to coalesce with other flows downvalley. Drainage networks can be influenced by tectonism, such as the uplift disrupting previously existing drainage lines (Western Rivers landscape zone; Waclawik et al., Citation2008). In the Flinders Ranges area, uplift has created radial drainage; drainage networks diverge rather than coalesce. In both cases, tectonism has created relatively short drainage networks. In contrast, the Channel Country’s tectonically driven topography constrains tributaries to drain into axial trunk rivers, allowing collection of runoff from a wide area and regularly delivering large flows. This tectonism also drives the fluvial style: wide valleys promote the development of anabranching and anastomosing (Morón et al., Citation2017; Morón & Amos Citation2018). In the Central Australian Uplands landscape zone, bedrock geology influences drainage geometry in two ways. Firstly, most lower-order reaches are in rocky ranges with high-runoff surfaces and close hillslope-channel coupling. Secondly, medium order drainage networks are shaped by structured bedrock with a dominantly east–west character, while higher-order reaches are transverse to the structural trend (). Lower-order drainage brings abundant runoff into strike-valley streams, which are then intersected by trunk rivers that cut through the ranges. Thus trunk reaches receive waters from broad subcatchments, enabling them to transmit flow from one range to the next. In contrast, drainage networks of the Barrier Range are less influenced by lithology; they do not coalesce into large networks. The trunk rivers are not shaped by high-discharge floods, and thus terminate only a few tens of kilometres from the rangefront.
Drainage integration expresses the degree to which the landscape causes flow paths to coalesce (c.f. connectivity, which is how much of the drainage network is flowing at a given time). The pathway from hillslope to channel (lateral integration) is governed by hillslope runoff character and fluvial landform distribution. For example, many creeks in the Central Australian and Flinders Uplands have a high degree of lateral integration since the channels and high-runoff rocky surfaces are closely linked across narrow floodplains. The rainfall to runoff to channelised flow progression occurs with relatively little loss. Although little rainfall is retained in situ, riverine and downvalley ecosystems are supported. In contrast, the Northern Plains rivers have very low-runoff contributing surfaces, so the hillslope to channel pathway has a low degree of lateral integration. In this case, the hillslope ecosystems are well supported by rainfall retention, but contribute little flow to the trunk channel.
The degree of continuity in the downvalley flow path is its longitudinal integration. A high degree of longitudinal integration can be achieved by a continuous channel, such as the Finke River (Ormiston to Apatula reaches). A valley macrochannel can have strong longitudinal integration even if it lacks continuous channels, as is the case in Cooper Creek or the Neales River. Drainage networks with strong longitudinal integration may carry large and far-travelling flows that benefit downvalley ecosystems: the Finke River supports terrestrial ecosystems in the Simpson Desert, and Cooper Creek supports Ramsar-listed wetlands in the Strzelecki Desert. Conversely, a low degree of longitudinal integration will occur if the channel network is interrupted by floodouts, for example, where the Todd River floods out near Amoonguna township in Central Australia. Rivers with hiatuses in their longitudinal integration may have small flows that do not travel far, but their floodouts benefit local ecosystems, such as the piedmont floodouts of the Rocky Fringe landscape zone ().
Many Australian drylands ecosystems are built around “patch” dynamics: small-scale spatial heterogeneity that promotes efficient local resource use (Ludwig et al., Citation1996; Pickup, Citation1985; Tongway & Ludwig, Citation1994). In this context, it is desirable to avoid value-implicit language for describing drainage integration. The phrases “well-integrated” and “poorly-integrated” imply that one kind of river is more desirable than another. In realty, both high and low degrees of longitudinal integration have benefits to offer drylands ecosystems.
Aridity, vegetation, and flow path roughness
Drylands biota must maximise their access to water. For plants, this can mean growing on or near frequently inundated landforms. The variable flow regimes of dryland rivers lend themselves to this: a river that is often dry can be colonised by terrestrial vegetation. Life strategies that facilitate this include seed dispersal by flood and ability to withstand fluctuations in salinity and water availability (Casanova, Citation2015; Costelloe, Citation2016; Costelloe et al., Citation2016; Roberts & Marston, Citation2011). The tendency for trees and large plants to grow in flow paths may be unique to drylands rivers (Nanson et al., Citation2002). Importantly, this places large vegetation in locations of vigorous fluvial processes.
In the Australian drylands, large trees and shrubs in sometimes dense plant communities commonly occur in macrochannels (), bank tops () floodplains and other flow path locations (). In-channel trees form physical barriers of their trunks, branches and foliage, as well as the barriers of flood debris formed against them (Graeme & Dunkerley, Citation1993). Such impediments to flow act to decrease velocity and stream power, and their distribution influences locations of turbulence and shear stress. Plant communities in riverine corridors are thus significant bioengineers, creating and maintaining landforms through their influence on erosion, sediment deposition, and seed distribution (Corenblit et al., Citation2009, Citation2007, Citation2020). The obstructive effect of vegetation increases with taller or more stiff plants, greater vegetation density, or plant groupings that are not aligned in a streamwise direction (Sandercock et al., Citation2007).
Roughness along the flow path is an important determinant of fluvial behaviour; the parameter Manning’s n is commonly used to represent roughness in calculations. Typical values vary from 0.02 for small simple streams to 0.06 for larger or more complex streams, while irregular, rocky or heavily timbered flow paths can have values of 0.11–0.20 (Ball et al., Citation2019; Fryirs & Brierley, Citation2013). However, the standard values are largely derived from northern hemisphere rivers; there have been few Australian studies. Tooth (Citation2000b) estimated Manning’s n values of 0.030–0.045 in rivers of the Northern Plains landscape zone. Graeme and Dunkerley (Citation1993) established that in-channel Eucalyptus communities increased the Manning’s n by 2.3–82.1%, depending on the site’s other elements of boundary roughness. In the Australian drylands, vegetation’s effect on flow patterns and landforms includes the development of anabranching (Morón et al., Citation2017; Tooth & Nanson, Citation2004), tributary-confluence floodout formation and maintenance (Wakelin-King & Webb, Citation2007a), channel width:depth ratios (Pietsch & Nanson, Citation2011), and the flow velocity gradients that maintain Channel Country waterhole depth and bank steepness (Knighton & Nanson, Citation2000, Citation2002).
Management implications
If landscape processes are not understood properly, then management and regulatory decisions are based on incorrect assumptions, with consequences extending throughout the public and private sectors. The information presented here has broad implications for land and catchment management.
There is a very high degree of diversity in Lake Eyre Basin rivers, both between and sometimes within the landscape zones. It is inappropriate to manage rivers as if they were all the same. In particular, assessment systems for river condition should start with site-specific knowledge of natural (pre-colonial) river behaviour.
In catchments with highly variable flow regimes (like the LEB) channels are not the only (or even the most important) fluvial elements. Rivers in which significant volumes of water can be transmitted along floodplains should be recognised in hydrological measurement, cartography, infrastructure design and environmental flow calculations. Fluvial monitoring and management should include floodplains, unchanneled water-bearing landforms, and contributing hillslopes.
Drylands rivers are active and “working” even during the no-flow stages. Terrestrial ecosystems are major components of riverine (including in-channel) corridors: assessment systems for drylands river condition should not overemphasise the aquatic at the expense of the terrestrial. Drylands rivers have value beyond their potential as water resources: even small creeks support ecosystems.
Large vegetation exists in many drylands river flow paths, constituting considerable resistance to flow, and consequently acting as an important bioengineer.
Low-integration drainage networks are a critical part of the LEB fluvial architecture. Throughflow rivers have high degrees of drainage integration and transfer water to downflow ecosystems, while “patch” landscapes with low degrees of integration retain water locally. Both provide valuable services to their biota and must be considered as equally important.
Some common morphometric practices are likely to suit LEB rivers poorly. An assumption that at-a-point discharge scales with upstream catchment size is built into hydrological calculations (Ball et al., Citation2019; Shilton et al., Citation2015), and contributing area plots are used as a surrogate indicator of relative discharge in geomorphological analysis (Brierley & Fryirs, Citation2005). However, this is not the case in the LEB, where floodouts interrupt some flow paths, and all the rivers experience transmission loss.
Conclusions
This study defines nine landscape zones for the Lake Eyre Basin, based on detailed analyses of multi-scale geomorphic domains. Particular attention is given to those attributes that define LEB fluvial styles: channel types, floodplain–channel relationships, valley confinement, flow path integration, sediment, and flow energy. The overview presented here is the first basin-wide geomorphological study of the LEB, and the first catchment-scale baseline geomorphological study of any of its rivers as whole-of-landscape entities.
Aridity, variable flow regimes and generally low-gradient topography are well-known drivers of LEB fluvial styles. However, they are not homogenous across the basin: there are different climate zones, spatial and temporal fluctuations in rainfall, and slope values ranging from steep to extremely low across reach to sub-catchment scales. Other drivers of fluvial style are similarly inhomogeneous. Surficial geology supplies rivers with sediments ranging in size from boulders to muds, in quantities ranging from abundant to negligible. Different pathways of landscape evolution have created some contributing hillslopes that shed rainwater and others that retain it. The life strategies that co-locate plant communities with water-adjacent landforms create spatially variable roughness elements in the flow path. The outcome of all these factors is a diversity of fluvial styles within the Lake Eyre Basin.
The landscape zones (LZ) are Uplands, Rocky Fringe, Western Rivers, Northern Plains, Central Plains, Channel Country-Vertic Downs, Channel Country-Stony Domes, Lakes & Dunes, and Mega-lakes (). Most LEB catchments occur over at least two landscape zones, as do the LEB’s loosely defined regions (Central Australia, western LEB, the Channel Country, and southern LEB).
Central Australian rivers are usually wide sand-bed rivers in a sandy floodplain. They arise in rocky country (Uplands LZ) that is arid but can receive voluminous rainfall. Rapid runoff and close hillslope–channel coupling promotes high-energy flows and transport of quartzose sandy bedload, while a closely integrated drainage network allows flows to coalesce in main trunk reaches. Gorge-type waterholes may be present in rocky country, and within-channel waterholes may occur elsewhere. Those that drain towards the south-east (e.g. the Todd River, Uplands LZ) have relatively steep gradients, rocky contributing hillslopes and a high degree of longitudinal integration; they amass flows large enough to push long distances into the Simpson Desert (Central Plains LZ). Those that drain towards the east (e.g. the Sandover River, Northern Plains LZ) have low-runoff contributing hillslopes and drainage networks that are not highly integrated; the main trunk channel floods out before reaching base level.
In the western LEB (e.g. the Neales River, Western Rivers LZ), tectonism and stream capture contribute to a landscape notable for breakaways and elevated low-relief gibber plains. Rivers cut back and down through regolith profiles rich in duricrust; contributing hillslopes and fluvial character are strongly influenced by local geology. The flow paths are in valley macrochannels, in many of which floodplain flow is the dominant mode of water transmission. Channel Country-type waterholes are present. Rivers of the western LEB flood out in the Central Plains or Mega-lakes LZ. Drainage networks are not especially long, dominated by tributary rather than trunk reaches, and some are disarranged by tectonic activity. The climate is more arid than the Central Australian ranges to the north, and less likely to receive orographic rainfall. Climate and drainage geometry make these rivers less likely to experience large floods.
The Channel Country catchments are strongly influenced by both surficial geology and underlying tectonism. Some upper-catchment reaches (Mt Isa and Galilee Uplands LZ) have less harsh climates than other Uplands LZ. Channel Country drainage networks are long and highly integrated, allowing monsoonal rain to be delivered deep into the most arid areas. Upper and mid-catchment (Vertic Downs LZ) are low-gradient rivers with moderately wide shallow valley macrochannels hosting floodways, small Channel Country-type waterholes, discontinuous channels, and braid-like bars. Interfluves are undulating downs of residual vertic soils. The mid- to lower catchment (Stony Domes LZ) is very low-gradient, with wide valley macrochannels floored by transported vertic soils, and underlain by Neogene sands. The macrochannels contain anastomosing multi-level floodways, braid-like bars, discontinuous channels in complex anabranching networks, small to large Channel Country-type waterholes, and lignum swamps. Interfluves are stony or sandy. Channel Country rivers flood out in the Stony Domes or Central Plains LZ, or terminate at base level in the Mega-lakes LZ. Rivers of the Diamantina catchment traverse the Lakes & Dunes LZ before terminating at base level in the Mega-lakes LZ.
The landscapes of the southern LEB are dominated by rocky uplands and regolith-mantled alluvial fans. Active uplift in the upper reaches (Flinders Uplands LZ) has produced steep gradients and peaks that sometimes receive voluminous rainfall. The drainage networks are relatively short and do not coalesce, while the lower reaches (Rocky Fringe LZ) have low-runoff contributing hillslopes. Consequently, these river are less likely to generate large or long-lasting floods, and their flow paths are interrupted by or terminate in floodouts. Many of the rivers terminate near the playas of the Mega-lake LZ.
One aim of this study was to redress an existing information imbalance overwhelmingly in favour of Channel Country lowlands rivers. This aim has been achieved within the scope of this investigation, yet the Channel Country lowlands continue to dominate the information presented here. In part, this is because those areas and their geomorphological processes are complex and important. However, it also reflects the available information. There will be other important landforms and processes waiting to be uncovered across the Lake Eyre Basin.
Acknowledgments
Early concepts were developed during discussions with ecologists Dale McNeil, David Schmarr and Rupert Mathwin (South Australian Research and Development Institute) and Angus Duguid (Northern Territory Department of Primary Industries and Resources). Field observations were collected while consulting to the South Australian public sector, and Henry Mancini (Natural Resources SA Arid Lands) is thanked for creating and maintaining an interdisciplinary research group. Many thanks to Dr. C Krapf and an anonymous reviewer for helpful reviews and Alissa Flatley for discussions.
Disclosure statement
No potential conflict of interest was reported by the author(s).
Additional information
Funding
References
- Ahmad, M., & Munson, T. J. (2013). Geology and mineral resources of the Northern Territory: Northern Territory Geological Survey, Special Publication 5. Northern Territory Department of Mines and Energy: Darwin.
- Allen, A. C. B. (1968). Marginal settlement‐a case study of the Channel Country of southwest Queensland. Australian Geographical Studies, 6(1), 1–23. https://doi.org/https://doi.org/10.1111/j.1467-8470.1968.tb00174.x
- Alley, N. F., Sheard, M. J., White, M. R., & Krieg, G. W. (2011). The sedimentology and stratigraphic significance of Cretaceous sediments at Mt Howie, NE South Australia. Transactions of the Royal Society of South Australia, 135(1), 12–25. https://doi.org/https://doi.org/10.1080/03721426.2011.10887147
- Alley, N. F. (1998). Cainozoic stratigraphy, palaeoenvironments and geological evolution of the Lake Eyre Basin. Palaeogeography, Palaeoclimatology, Palaeoecology, 144(3–4), 239–263. https://doi.org/https://doi.org/10.1016/S0031-0182(98)00120-5
- Amos, K., Goodwin, C., & Soria Jauregui, A. (2012). Incised valleys in marginal-lacustrine depositional environments: A new reservoir analogue from Lake Eyre, Central Australia. APPEA Journal, 52(1), 513–524. https://doi.org/https://doi.org/10.1071/AJ11040
- Anand, R. R. (2005). Weathering history, landscape evolution, and implications for exploration. In R. R. Anand & P. de Broekert (Eds.), Regolith landscape evolution across Australia: A compilation of regolith landscape case studies with regolith landscape evolution models (pp. 2–40). Cooperative Research Centre for Landscape Environment and Mineral Exploration (CRC LEME). http://crcleme.org.au/Pubs/monographs.html
- Anand, R. R., & de Broekert, P. (Eds.). (2005). Regolith landscape evolution across Australia: A compilation of regolith landscape case studies with regolith landscape evolution models. Cooperative Research Centre for Landscape Environment and Mineral Exploration (CRC LEME). http://crcleme.org.au/Pubs/monographs.html
- Baker, V. R., Pickup, G., & Polach, H. A. (1983). Desert palaeofloods in Central Australia. Nature, 301(5900), 502–504. https://doi.org/https://doi.org/10.1038/301502a0
- Ball, J. E., Babister, M. K., Nathan, R., Weeks, W., Weinmann, P. E., Retallick, M., & Testoni, I. (Eds). (2019). Australian Rainfall and Runoff - A guide to flood estimation. Geoscience Australia. http://pid.geoscience.gov.au/dataset/ga/127626
- Barlow, F. T. H. (1988). Hydrology of the Todd River flood of March 1988 (Technical report #31, WRD88031). Northern Territory Power and Water Authority.
- Beeson, H. W., McCoy, S. W., & Keen-Zebert, A. (2017). Geometric disequilibrium of river basins produces long-lived transient landscapes. Earth and Planetary Science Letters, 475, 34–43. https://doi.org/https://doi.org/10.1016/j.epsl.2017.07.010
- Benbow, M. C., Alley, N. F., Callen, R. A., & Greenwood, D. R. (1995a). Geological History and Palaeoclimate. J. F. Drexel & W. V. Preiss Eds., The geology of South Australia: Vol. 2, The Phanerozoic Bulletin 54. (208–218). Geological Survey of South Australia.
- Benbow, M. C., Callen, R. A., Bourman, R. P., & Alley, N. F. (1995b). Deep weathering, ferricrete and silcrete. J. F. Drexel & W. V. Preiss Eds., The geology of South Australia: Vol. 2, The Phanerozoic Bulletin 54. (201–207). Geological Survey of South Australia.
- Bertoldi, W., Zanoni, L., & Tubino, M. (2009). Planform dynamics of braided streams. Earth Surface Processes and Landforms, 34(4), 547–557. https://doi.org/https://doi.org/10.1002/esp.1755
- Bourke, M. C. (1994). Cyclical construction and destruction of flood dominated flood plains in semiarid Australia. In L. J. Olive, R. J. Loughran, & J. A. Kesby Eds., Variability in Stream Erosion and Sediment Transport; Publication 224 (pp. 113–124). Oxfordshire: International Association of Hydrological Sciences
- Bourke, M. C., & Pickup, G. (1999). Fluvial form variability in arid central Australia. In A. J. Miller & A. Gupta (Eds.), Varieties of Fluvial Form (pp. 249–271). John Wiley and Sons.
- Bowler, J. M. (1983). Lunettes as indices of hydrologic change: A review of Australian evidence. Proceedings of the Royal Society of Victoria, 95(3), 147–168.
- Brierley, G. J., & Fryirs, K. A. 2005. Geomorphology and river management: Applications of the river styles framework. Blackwell Publishing.
- Bull, W. B. (1997). Discontinuous ephemeral streams. Geomorphology, 19(3–4), 227–276. https://doi.org/https://doi.org/10.1016/S0169-555X(97)00016-0
- Bunn, S. E., Thoms, M. C., Hamilton, S. K., & Capon, S. J. (2006). Flow variability in dryland rivers: Boom, bust and the bits in between. River Research and Applications, 22(2), 179–186. https://doi.org/https://doi.org/10.1002/rra.904
- Callen, R. A., Alley, N. F., & Greenwood, D. R. (1995). Lake Eyre Basin. In J. F. Drexel & W. V. Preiss (Eds.). The geology of South Australia: Vol. 2, The Phanerozoic; Bulletin 54 (pp. 188–194). Geological Survey of South Australia.
- Callen, R. A., & Benbow, M. C. (1995). The deserts – Playas, dunefields, and watercourses. In J. F. Drexel & W. V. Preiss (Eds.). The geology of South Australia: Vol. 2, The Phanerozoic; Bulletin 54 (pp. 244–251). Geological Survey of South Australia.
- Callen, R. A., & Bradford, J. (1992). Cooper Creek fan and Strzelecki Creek - hypsometric data, Holocene sedimentation, and implications for human activity. Mines and Energy Review South Australia, 158, 52–57. ISSN 1034-8794.
- Casanova, M. T. (2015). Review of water requirements for key floodplain vegetation for the northern basin: Literature review and expert knowledge assessment, Report by Charophyte Services to the Murray–Darling Basin Authority.
- Cendón, D. I., Larsen, J. R., Jones, B. G., Nanson, G. C., Rickleman, D., Hankin, S. I., Pueyo, J. J., & Maroulis, J. (2010). Freshwater recharge into a shallow saline groundwater system, Cooper Creek floodplain, Queensland, Australia. Journal of Hydrology, 392(3–4), 150–163. https://doi.org/https://doi.org/10.1016/j.jhydrol.2010.08.003
- Chan, R. A. (2009). Evolution of the Girilambone regolith landscape, central western New South Wales. Australian Journal of Earth Sciences, 56:(1), S105–S123. https://doi.org/https://doi.org/10.1080/08120090902871135
- Clarke, J. D. A. (2005). Complex depositional landscapes of the Western Australian wheatbelt. In I. C. Roach (Ed.), Proceedings of the CRC LEME Regolith Symposia: Regolith 2005 – Ten Years of CRC LEME (pp. 49–54). Bentley, WA: Cooperative Research Centre for Landscape Environments and Mineral Exploration. http://crcleme.org.au/Pubs/monographs.html
- Clarke, J. D., & Chenoweth, L. (1995). Classification, genesis and evolution of ferruginous surface grains. AGSO Journal of Australian Geology & Geophysics, 16(3), 213–222. ISSN 1320-1271.
- Cohen, T. J., Meyer, M. C., & May, J. H. (2018). Identifying extreme pluvials in the last millennia using optical dating of single grains of quartz from shorelines on Australia’s largest lake. The Holocene, 28(1), 150–165. https://doi.org/https://doi.org/10.1177/0959683617715700
- Cohen, T. J., Nanson, G. C., Jansen, J. D., Jones, B. G., Jacobs, Z., Larsen, J. R., May, J.-H., Treble, P., Price, D. M., & Smith, A. M. (2012). Late Quaternary mega-lakes fed by the northern and southern river systems of central Australia: Varying moisture sources and increased continental aridity. Palaeogeography, Palaeoclimatology, Palaeoecology, 356, 89–108. https://doi.org/https://doi.org/10.1016/j.palaeo.2011.06.023
- Cohen, T. J., Nanson, G. C., Jansen, J., Jones, B. G., Jacobs, Z., Treble, P., Price, D. M., May, J.-H., Smith, A. M., Ayliffe, L. K., & Hellstrom, J. C. (2011). Continental aridification and the vanishing of Australia’s megalakes. Geology, 39(2), 167–170. https://doi.org/https://doi.org/10.1130/G31518.1
- Cohen, T. J., Nanson, G. C., Larsen, J. R., Jones, B. G., Price, D. M., Coleman, M., & Pietsch, T. J. (2010). Late Quaternary aeolian and fluvial interactions on the Cooper Creek Fan and the association between linear and source-bordering dunes, Strzelecki Desert, Australia. Quaternary Science Reviews, 29(3–4), 455–471. https://doi.org/https://doi.org/10.1016/j.quascirev.2009.09.024
- Colloff, M. J., & Baldwin, D. S. (2010). Resilience of floodplain ecosystems in a semi-arid environment. The Rangeland Journal, 32(3), 305–314. https://doi.org/https://doi.org/10.1071/RJ10015
- Cook, A. G., & Jell, J. S. (2013). Palaeogene and Neogene. In P. A. Jell (Ed.). Geology of Queensland. (pp. 577–652). Geological Survey of Queensland.
- Corenblit, D., Steiger, J., Gurnell, A. M., Tabacchi, E., & Roques, L. (2009). Control of sediment dynamics by vegetation as a key function driving biogeomorphic succession within fluvial corridors. Earth Surface Processes and Landforms, 34(1413), 1790–1810. https://doi.org/https://doi.org/10.1002/esp.1876
- Corenblit, D., Tabacchi, E., Steiger, J., & Gurnell, A. M. (2007). Reciprocal interactions and adjustments between fluvial landforms and vegetation dynamics in river corridors: A review of complementary approaches. Earth-Science Reviews, 84(1–2), 56–86. doi:https://doi.org/10.1016/j.earscirev.2007.05.004.
- Corenblit, D., Vautier, F., González, E., & Steiger, J. (2020). Formation and dynamics of vegetated fluvial landforms follow the biogeomorphological succession model in a channelized river. Earth Surface Processes and Landforms, 45(9), 2020–2035. https://doi.org/https://doi.org/10.1002/esp.4863
- Costelloe, J. F. (2013). Hydrological assessment and analysis of the Cooper Creek catchment, South Australia. Report by the University of Melbourne to the South Australian Arid Lands Natural Resources Management Board. Retrieved (2021) from www.land scape.sa.gov.au/saal/water/managing-water-resources/Lake_Eyre_Basin_catchment_projects .
- Costelloe, J. F. (2016). Water use strategies of a dominant riparian tree species (Eucalyptus coolabah) in dryland rivers. In J. A. Webb, J. F. Costelloe, R. Casas-Mulet, J. P. Lyon, and M. J. Stewardson (Eds.). Proceedings of the 11th International Symposium on Ecohydraulics, Melbourne. Barton: Engineers Australia; retrieved (2021) from www.researchgate.net/profile/Justin-Costelloe
- Costelloe, J. F. (2017). Hydrological assessment and analysis of the Diamantina River catchment, South Australia. Report by the University of Melbourne to the South Australian Arid Lands Natural Resources Management Board: Retrieved (2021) from http://www.landscape.sa.gov.au/saal/water/managing-water-resources/Lake_Eyre_Basin_catchment_pro jects .
- Costelloe, J. F., Grayson, R. B., & McMahon, T. A. (2006). Modelling streamflow in a large anastomosing river of the arid zone, Diamantina River, Australia. Journal of Hydrology, 323(1–4), 138–153. https://doi.org/https://doi.org/10.1016/j.jhydrol.2005.08.022
- Costelloe, J. F., Hudson, P. J., Pritchard, J. C., Puckridge, J. T., & Reid, J. R. W. (2004). ARIDFLO Scientific Report: Environmental flow requirements of arid zone rivers with particular reference to the Lake Eyre drainage basin. Final Report from the School of Earth and Environmental Sciences, University of Adelaide, to South Australian Department of Water, Land and Biodiversity Conservation; and Commonwealth Department of Environment and Heritage.
- Costelloe, J. F., Irvine, E. C., Western, A. W., & Herczeg, A. L. (2009). Groundwater recharge and discharge dynamics in an arid‐zone ephemeral lake system, Australia. Limnology and Oceanography, 54(1), 86–100. https://doi.org/https://doi.org/10.4319/lo.2009.54.1.0086
- Costelloe, J. F., Leeder, J., & Strang, M. (2016). Drivers of the distribution of a dominant riparian tree species (Eucalyptus coolabah) on a dryland river system, Diamantina River, Australia. In J. A. Webb, J. F. Costelloe, R. Casas-Mulet, J. P. Lyon, & M. J. Stewardson (Eds.). Proceedings of the 11th International Symposium on Ecohydraulics, Melbourne. Barton: Engineers Australia. www.researchgate.net/profile/Justin-Costelloe
- Costelloe, J. F., & Russell, K. L. (2014). Identifying conservation priorities for aquatic refugia in an arid zone, ephemeral catchment: A hydrological approach. Ecohydrology, 7(6), 1534–1544. https://doi.org/https://doi.org/10.1002/eco.1476
- Costelloe, J. F., Shields, A., Grayson, R. B., & McMahon, T. A. (2007). Determining loss characteristics of arid zone river waterbodies. River Research and Applications, 23(7), 715–731. https://doi.org/https://doi.org/10.1002/rra.991
- Craddock, R. A., Hutchinson, M. F., & Stein, J. A. (2010). Topographic data reveal a buried fluvial landscape in the Simpson Desert, Australia. Australian Journal of Earth Sciences, 57(1), 141–149. https://doi.org/https://doi.org/10.1080/08120090903416278
- Croke, J., Fryirs, K., & Thompson, C. (2016). Defining the floodplain in hydrologically‐variable settings: Implications for flood risk management. Earth Surface Processes and Landforms, 41(14), 2153–2164. https://doi.org/https://doi.org/10.1002/esp.4014
- Croke, J., Magee, J., & Price, D. (1996). Major episodes of Quaternary activity in the lower Neales River, northwest of Lake Eyre, central Australia. Palaeogeography, Palaeoclimatology, Palaeoecology, 124(1–2), 1–15. https://doi.org/https://doi.org/10.1016/0031-0182(96)00016-8
- Croke, J., Thompson, C., & Fryirs, K. (2017). Prioritising the placement of riparian vegetation to reduce flood risk and end-of-catchment sediment yields: Important considerations in hydrologically-variable regions. Journal of Environmental Management, 190, 9–19. https://doi.org/https://doi.org/10.1016/j.jenvman.2016.12.046
- Crowley, G. M., & Preece, N. D. (2019). Does extreme flooding drive vegetation and faunal composition across the Gulf Plains of north-eastern Australia? Austral Ecology, 44(7), 1256–1270. https://doi.org/https://doi.org/10.1111/aec.12803
- de Broekert, P., & Sandiford, M. (2005). Buried inset-valleys in the Eastern Yilgarn Craton, Western Australia: Geomorphology, age, and allogenic control. The Journal of Geology, 113(4), 471–493. https://doi.org/https://doi.org/10.1086/430244
- Deblauwe, V., Couteron, P., Bogaert, J., & Barbier, N. (2012). Determinants and dynamics of banded vegetation pattern migration in arid climates. Ecological Monographs, 82(1), 3–21. https://doi.org/https://doi.org/10.1890/11-0362.1
- DeVogel, S. B., Magee, J. W., Manley, W. F., & Miller, G. H. (2004). A GIS-based reconstruction of late Quaternary paleohydrology: Lake Eyre, arid central Australia. Palaeogeography, Palaeoclimatology, Palaeoecology, 204(1–2), 1–13. https://doi.org/https://doi.org/10.1016/S0031-0182(03)00690-4
- Drexel, J. F., & Preiss, W. V. (Eds.). (1995). The Geology of South Australia: Vol. 2, The Phanerozoic; Bulletin 54. Geological Survey of South Australia.
- Driessen, P., Deckers, J., Spaargaren, O., & Nachtergaele, F. (2001). Lecture notes on the major soils of the world. World Soil Resources Reports No. 94. United Nations: Food and Agriculture Organisation on the United Nations ISBN 925-104637-9.
- Duguid, A., Barnetson, J., Clifford, B., Pavey, C., Albrecht, D., Risler, J., & McNellie, M. (2005). Wetlands in the arid Northern Territory: Inventory and significance. Report from the Northern Territory Department of Natural Resources, Environment and the Arts to the Australian Department of the Environment and Heritage.
- Dulhunty, J. (1986). Holocene sediments of Lake Eyre playa. In R. T. Wells & R. A. Callen (Eds.), The Lake Eyre Basin - Cainozoic sediments, fossil vertebrates and plants, landforms, silcretes and climatic implications. Australasian Sedimentologists Group Field Guide Series (No. 4) (pp. 73–78). Geological Society of Australia.
- Dunkerley, D. L., & Brown, K. J. (1995). Runoff and runon areas in a patterned chenopod shrubland, arid western New South Wales, Australia: Characteristics and origin. Journal of Arid Environments, 30(1), 41–55. https://doi.org/https://doi.org/10.1016/S0140-1963(95)80037-9
- Dunkerley, D. L. (2011). Desert Soils. In D. S. Thomas, Ed. Arid zone geomorphology: Process, form and change in drylands. 3rd (pp. 101–129). John Wiley & Sons. https://doi.org/https://doi.org/10.1002/9780470710777
- Dunkerley, D. L., & Brown, K. J. (1999). Banded vegetation near Broken Hill, Australia: Significance of surface roughness and soil physical properties. Catena, 37(1–2), 75–88. https://doi.org/https://doi.org/10.1016/S0341-8162(98)00056-3
- Edgoose, C. J. 2005. Barkly Tableland Region, Northern Territory. In R. R. Anand & P. de Broekert. (Eds.), Regolith landscape evolution across Australia: A compilation of regolith landscape case studies with regolith landscape evolution models (pp. 148–150). Cooperative Research Centre for Landscape Environment and Mineral Exploration (CRC LEME). http://crcleme.org.au/Pubs/monographs.html
- Environment Australia 2000. Revision of the Interim Biogeographic Regionalisation of Australia (IBRA) and the Development of Version 5.1. - Summary Report. Department of Environment and Heritage. www.environment.gov.au/land/nrs/publications/revision-ibra-development-of-version-5-1-summary-report
- Fagan, S. D., & Nanson, G. C. (2004). The morphology and formation of floodplain surface channels, Cooper Creek, Australia. Geomorphology, 60(1–2), 107–126. https://doi.org/https://doi.org/10.1016/j.geomorph.2003.07.009
- Fanning, P. C. (1999). Recent landscape history in arid western New South Wales, Australia; a model for regional change. Geomorphology, 29(3–4), 191–209. https://doi.org/https://doi.org/10.1016/S0169-555X(99)00014-8
- Fensham, R. J., Silcock, J. L., Kerezsy, A., & Ponder, W. (2011). Four desert waters: Setting arid zone wetland conservation priorities through understanding patterns of endemism. Biological Conservation, 144(10), 2459–2467. https://doi.org/https://doi.org/10.1016/j.biocon.2011.06.024
- Finlayson, B. L., & McMahon, T. A. (1988). Australia v. the world: A comparative analysis of streamflow characteristics. In R. F. Warner (Ed.). Fluvial geomorphology of Australia. (pp. 17–40). Academic Press.
- Fisher, J. A., Krapf, C. B. E., Lang, S. C., Nichols, G. J., & Payenberg, T. H. D. (2008). Sedimentology and architecture of the Douglas Creek terminal splay, Lake Eyre, central Australia. Sedimentology, 55(6), 1915–1930. https://doi.org/https://doi.org/10.1111/j.1365-3091.2008.00974.x
- Fitzsimmons, K. E. (2007). Morphological variability in the linear dunefields of the Strzelecki and Tirari Deserts, Australia. Geomorphology, 91(1–2), 146–160. https://doi.org/https://doi.org/10.1016/j.geomorph.2007.02.004
- Fitzsimmons, K. E., Bowler, J., Rhodes, E., & Magee, J. (2007). Relationships between desert dunes during the late Quaternary in the Lake Frome region, Strzelecki Desert, Australia. Journal of Quaternary Science, 22(5), 549–558. https://doi.org/https://doi.org/10.1002/jqs.1120
- Fitzsimmons, K. E., Magee, J. W., & Amos, K. J. (2009). Characterisation of aeolian sediments from the Strzelecki and Tirari Deserts, Australia: Implications for reconstructing palaeoenvironmental conditions. Sedimentary Geology, 218(1–4), 61–73. https://doi.org/https://doi.org/10.1016/j.sedgeo.2009.04.004
- Fitzsimmons, K. E., Rhodes, E. J., Magee, J. W., & Barrows, T. T. (2007). The timing of linear dune activity in the Strzelecki and Tirari Deserts, Australia. Quaternary Science Reviews, 26(19–21), 2598–2616. https://doi.org/https://doi.org/10.1016/j.quascirev.2007.06.010
- Freudenberger, D. O., & Hiernaux, P. (2001). Productivity of patterned vegetation. In D. J. Tongway, C. Valentin, & J. Seghieri (Eds.), Banded vegetation patterning in arid and semiarid environments: Ecological processes and consequences for management (pp. 198–209). Springer. https://doi.org/https://doi.org/10.1007/978-1–4613-0207-0
- Fryirs, K. A., & Brierley, G. J. (2013). Geomorphic analysis of river systems: An approach to reading the landscape. Chichester: Wiley-Blackwell. ISBN 978-1-4051-9274-3.
- Fryirs, K., & Brierley, G. (1998). The character and age structure of valley fills in upper Wolumla Creek catchment, south coast, New South Wales, Australia. Earth Surface Processes and Landforms, 23(3), 271–287. https://doi.org/https://doi.org/10.1002/(SICI)1096-9837(199803)23:3<271::AID-ESP867>3.0.CO;2-5
- Fryirs, K., Brierley, G. J., & Erskine, W. D. (2012). Use of ergodic reasoning to reconstruct the historical range of variability and evolutionary trajectory of rivers. Earth Surface Processes and Landforms, 37(7), 763–773. https://doi.org/https://doi.org/10.1002/esp.3210
- Fujioka, T., Chappell, J., Fifield, L. K., & Rhodes, E. J. (2009). Australian desert dune field initiated with Pliocene–Pleistocene global climate shift. Geology, 37(1), 51–54. https://doi.org/https://doi.org/10.1130/G25042A.1
- Fujioka, T., Chappell, J., Honda, M., Yatsevich, I., Fifield, K., & Fabel, D. (2005). Global cooling initiated stony deserts in central Australia 2–4 Ma, dated by cosmogenic 21Ne–10Be. Geology, 33(12), 993–996. https://doi.org/https://doi.org/10.1130/G21746.1
- George, R., Clarke, J., and English, P. (2006). Modern and palaeogeographic trends in the salinisation of the Western Australian Wheatbelt. ASEG Extended Abstracts, 2006(1), 1–22. https://doi.org/10.1071/ASEG2006ab049
- Gibling, M. R., Nanson, G. C., & Maroulis, J. C. (1998). Anastomosing river sedimentation in the Channel Country of central Australia. Sedimentology, 45(3), 595–619. https://doi.org/https://doi.org/10.1046/j.1365-3091.1998.00163.x
- Gillen, J. S. (2017). Coolibah (Eucalyptus coolabah Blakely & Jacobs) of the Diamantina and Warburton River systems in North Eastern South Australia. Report by Australian National University to the South Australian Arid Lands Natural Resources Management Board, Pt Augusta. www.landscape.sa.gov.au/saal/water/managing-water-resources/Lake_Eyre_Basin_catchment_projects
- Graeme, D., & Dunkerley, D. L. (1993). Hydraulic resistance by the river red gum, Eucalyptus camaldulensis, in ephemeral desert streams. Australian Geographical Studies, 31(2), 141–154. https://doi.org/https://doi.org/10.1111/j.1467-8470.1993.tb00411.x
- Habeck-Fardy, A., & Nanson, G. C. (2014). Environmental character and history of the Lake Eyre Basin, one seventh of the Australian continent. Earth-Science Reviews, 132, 39–66. https://doi.org/https://doi.org/10.1016/j.earscirev.2014.02.003
- Habermehl, M. A. (1982). Springs in the Great Artesian Basin – Their origin and nature. Report 235. Canberra: Bureau of Mineral Resources, Geology and Geophysics.
- Hesse, P. P. (2010). The Australian desert dunefields: Formation and evolution in an old, flat, dry continent. Geological Society, London, Special Publications, 346(1), 141–164. https://doi.org/https://doi.org/10.1144/SP346.9
- Hesse, P. (2011). Sticky dunes in a wet desert: Formation, stabilisation and modification of the Australian desert dunefields. Geomorphology, 134(3–4), 309–325. https://doi.org/https://doi.org/10.1016/j.geomorph.2011.07.008
- Hollands, C. B., Nanson, G. C., Jones, B. G., Bristow, C. S., Price, D. M., & Pietsch, T. J. (2006). Aeolian-fluvial interaction; evidence for late Quaternary channel change and wind-rift linear dune formation in the northwestern Simpson Desert, Australia. Quaternary Science Reviews, 25(1–2), 142–162. https://doi.org/https://doi.org/10.1016/j.quascirev.2005.02.007
- Huang, H. Q., Nanson, G. C., & Fagan, S. D. (2002). Hydraulic geometry of straight alluvial channels and the principle of least action. Journal of Hydraulic Research, 40(2), 153–160. https://doi.org/https://doi.org/10.1080/00221680209499858
- Hubble, G. D. (1984). The cracking clay soils: Definition, distribution, nature, genesis and use. In J. W. McGarity, E. H. Hoult, and H. B. So (Eds.), The Properties and Utilisation of Cracking Clay Soils (pp. 3–13). ISBN : 0858345099 Reviews in Rural Science. Armidale: University of New England.
- Jansen, J. D., & Brierley, G. J. (2004). Pool-fills: A window to palaeoflood history and response in bedrock-confined rivers. Sedimentology, 51(5), 901–925. https://doi.org/https://doi.org/10.1111/j.1365-3091.2004.00643.x
- Jansen, J. D., Nanson, G. C., Cohen, T. J., Fujioka, T., Fabel, D., Larsen, J. R., Codilean, A. T., Price, D. M., Bowman, H. H., May, J.-H., & Gliganic, L. A. (2013). Lowland river responses to intraplate tectonism and climate forcing quantified with luminescence and cosmogenic 10 Be. Earth and Planetary Science Letters, 366, 49–58. https://doi.org/https://doi.org/10.1016/j.epsl.2013.02.007
- Jell, P. A. (Ed.). (2013). Geology of Queensland. Geological Survey of Queensland.
- Kemp, J., Pietsch, T., Gontz, A., & Olley, J. (2017). Lacustrine-fluvial interactions in Australia’s Riverine Plains. Quaternary Science Reviews, 166, 352–362. https://doi.org/https://doi.org/10.1016/j.quascirev.2017.02.015
- Kingsford, R. (2018). The Lake Eyre Basin - one of the world’s great desert river systems. R. Kingsford Ed., Lake Eyre Basin rivers: Environmental, social and economic importance. (3–18). CSIRO Publishing.
- Kingsford, R. T., Costelloe, J., & Sheldon, F. (2014). Lake Eyre Basin – Challenges for managing the world’s most variable river system. V. R. Squires, H. M. Milner, & K. A. Daniell Eds., River basin management in the twenty-first century.(346–367). Taylor & Francis Group.
- Knighton, A. D., & Nanson, G. C. (1993). Anastomosis and the continuum of channel pattern. Earth Surface Processes and Landforms, 18(7), 613–625. https://doi.org/https://doi.org/10.1002/esp.3290180705
- Knighton, A. D., & Nanson, G. C. (1994). Waterholes and their significance in the anastomosing channel system of Cooper Creek, Australia. Geomorphology, 9(4), 311–324. https://doi.org/https://doi.org/10.1016/0169-555X(94)90052-3
- Knighton, A. D., & Nanson, G. C. (2000). Waterhole form and process in the anastomosing channel system of Cooper Creek, Australia. Geomorphology, 35(1–2), 101–117. https://doi.org/https://doi.org/10.1016/S0169-555X(00)00026-X
- Knighton, A. D., & Nanson, G. C. (2001). An event-based approach to the hydrology of arid zone rivers in the Channel Country of Australia. Journal of Hydrology, 254(1–4), 102–123. https://doi.org/https://doi.org/10.1016/S0022-1694(01)00498-X
- Knighton, A. D., & Nanson, G. C. (2002). Inbank and overbank velocity conditions in an arid zone anastomosing river. Hydrological Processes, 16(9), 1771–1791. https://doi.org/https://doi.org/10.1002/hyp.1076
- Krapf, C., Costar, A., Stoian, L., Keppel, M., Gordon, G., Inverarity, L., and Munday, T. (2019). A sniff of the ocean in the Miocene at the foothills of the Musgrave Ranges – Unravelling the evolution of the Lindsay East Palaeovalley. MESA Journal, 90(2), 4–22. https://energymining.sa.gov.au/minerals/knowledge_centre/mesa_journal
- Laity, J. E. (2011). Pavements and stone mantles. In D. S. Thomas, Ed. Arid zone geomorphology: Process, form and change in drylands. 3rd (pp. 181–207). John Wiley & Sons. https://doi.org/https://doi.org/10.1002/9780470710777
- Larsen, A., May, J. H., Moss, P., & Hacker, J. (2016). Could alluvial knickpoint retreat rather than fire drive the loss of alluvial wet monsoon forest, tropical northern Australia? Earth Surface Processes and Landforms, 41(11), 1583–1594. https://doi.org/https://doi.org/10.1002/esp.3933
- LEBSAP (2008). State of the Basin 2008: Rivers Assessment. Report by the Lake Eyre Basin Scientific Advisory Panel, to the Environmental Water & Natural Resources Branch, Australian Department of the Environment, Water, Heritage and the Arts.
- Leigh, C., Sheldon, F., Kingsford, R. T., & Arthington, A. H. (2010). Sequential floods drive ‘booms’ and wetland persistence in dryland rivers: A synthesis. Marine & Freshwater Research, 61(8), 896–908. https://doi.org/https://doi.org/10.1071/MF10106
- Leon, J. X., & Cohen, T. J. (2012). An improved bathymetric model for the modern and palaeo Lake Eyre. Geomorphology, 173, 69–79. https://doi.org/https://doi.org/10.1016/j.geomorph.2012.05.029
- Ludwig, J., Tongway, D., Hodgkinson, K., Freudenberger, D., & Noble, J. 1996. Landscape ecology, function and management: Principles from Australia’s rangelands CSIRO Publishing.
- Mabbutt, J. A. (1967). Denudation chronology in central Australia; structure, climate, and landform inheritance in the Alice Springs area. In J.N. Jennings & J.A. Mabbutt (Eds.). Landform studies from Australia and New Guinea (pp. 144–181). Australian National University Press.
- Mabbutt, J. A. (1977). Desert Landforms (An Introduction to Systematic Geomorphology: 2). Australian National University Press.
- Mabbutt, J. A., & Fanning, P. C. (1987). Vegetation banding in arid Western Australia. Journal of Arid Environments, 12(1), 41–59 www.sciencedirect.com/journal/journal-of-arid-environments .
- Magee, J. W. (2009). Palaeovalley groundwater resources in arid and semi-arid Australia – a literature review. Record 2009/03. Canberra: Geoscience Australia.
- Makaske, B. (2001). Anastomosing rivers: A review of their classification, origin and sedimentary products. Earth-Science Reviews, 53(3–4), 149–196. https://doi.org/https://doi.org/10.1016/S0012-8252(00)00038-6
- Maroulis, J. C., & Nanson, G. C. (1996). Bedload transport of aggregated muddy alluvium from Cooper Creek, central Australia: A flume study. Sedimentology, 43(5), 771–790. https://doi.org/https://doi.org/10.1111/j.1365-3091.1996.tb01501.x
- Maroulis, J. C., Nanson, G. C., Price, D. M., & Pietsch, T. (2007). Aeolian-fluvial interaction and climate change: Source-bordering dune development over the past c100 ka on Cooper Creek, central Australia. Quaternary Science Reviews, 26(3–4), 386–404. https://doi.org/https://doi.org/10.1016/j.quascirev.2006.08.010
- Marriott, S. B., & Wright, V. P. (2004). Mudrock deposition in an ancient dryland system: Moor Cliffs Formation, Lower Old Red Sandstone, southwest Wales, UK. Geological Journal, 39(3–434), 277–298. https://doi.org/https://doi.org/10.1002/gj.990
- McKenzie-Smith, F. (2004). Review, evaluation and collation (metadata) of relevant natural resource information and datasets for river systems and catchments in the Lake Eyre Basin agreement area. (Project Background Document 3; Lake Eyre Basin Rivers Assessment Methodology Development). Report from the Centre for Riverine Landscapes, Griffith University, to Land & Water Australia.
- McKeon, G., Hall, W., Henry, B., Stone, G., & Watson, I. 2004. Pasture Degradation and Recovery in Australia’s Rangelands: Learning from history Queensland Department of Natural Resources, Mines, and Energy.
- McMahon, T. A., & Finlayson, B. L. (2003). Droughts and anti‐droughts: The low flow hydrology of Australian rivers. Freshwater Biology, 48(7), 1147–1160. https://doi.org/https://doi.org/10.1046/j.1365-2427.2003.01098.x
- McMahon, T. A., Murphy, R. E., Peel, M. C., Costelloe, J. F., & Chiew, F. H. (2008a). Understanding the surface hydrology of the Lake Eyre Basin: Part 1—rainfall. Journal of Arid Environments, 72(10), 1853–1868. https://doi.org/https://doi.org/10.1016/j.jaridenv.2008.06.004
- McMahon, T. A., Murphy, R. E., Peel, M. C., Costelloe, J. F., & Chiew, F. H. (2008b). Understanding the surface hydrology of the Lake Eyre Basin: Part 2—streamflow. Journal of Arid Environments, 72(10), 1869–1886. https://doi.org/https://doi.org/10.1016/j.jaridenv.2008.06.001
- McNeil, D. G., Cheshire, D. L. M., Schmarr, D. W., & Mathwin, R. (2015). A conceptual review of aquatic ecosystem function and fish dynamics in the Lake Eyre Basin, central Australia. (Technical Report Series No. 15/36). Goyder Institute for Water Research. www.pir.sa.gov.au/research/publications/research_reports/research_reports_2015
- McNeil, D. G., Schmarr, D. W., & Rosenberger, A. E. (2011). Climatic variability, fish and the role of refuge waterholes in the Neales River Catchment: Lake Eyre Basin, South Australia. Report by South Australian Research and Development Institute (Aquatic Sciences) to the South Australian Arid Lands Natural Resources Management Board. www.landscape.sa.gov.au/saal/water/managing-water-resources/Lake_Eyre_Basin_catchment_projects .
- Mernagh, T. P. (Ed.). (2013). A review of Australian salt lakes and assessment of their potential for strategic resources. Record 2013/39. Geoscience Australia.
- Morón, S., & Amos, K. J. (2018). Downstream grain-size changes associated with a transition from single channel to anabranching. Sedimentology, 65(5), 1590–1610. doi:https://doi.org/10.1111/sed.12439.
- Morón, S., Edmonds, D. A., & Amos, K. (2017). The role of floodplain width and alluvial bar growth as a precursor for the formation of anabranching rivers. Geomorphology, 278, 78–90. https://doi.org/https://doi.org/10.1016/j.geomorph.2016.10.026
- Moussavi Harami, S. R., & Alexander, E. (1998). Tertiary stratigraphy and tectonics, Eromanga Basin region. MESA Journal, 8, 32–36 https://energymining.sa.gov.au/minerals/knowledge_centre/mesa_journal.
- Mudd, G. M. (2000). Mound springs of the Great Artesian Basin in South Australia: A case study from Olympic Dam. Environmental Geology, 39(5), 463–476. https://doi.org/https://doi.org/10.1007/s002540050452
- Nanson, G. C. (2013). Anabranching and anastomosing rivers. In E. Wohl (Ed.), Treatise on Geomorphology: Volume 9: Fluvial Geomorphology (pp. 330–345). Elsevier.
- Nanson, G. C., Callen, R. A., & Price, D. M. (1998). Hydroclimatic interpretation of Quaternary shorelines on South Australian playas. Palaeogeography, Palaeoclimatology, Palaeoecology, 144(3–4), 281–305. https://doi.org/https://doi.org/10.1016/S00310182(98)00123-0
- Nanson, G. C., & Knighton A. D.(1996). Anabranching rivers: their cause, character and classification. Earth Surface Processes and Landforms, 21(3), 217–239. https://doi.org/10.1002/(SICI)1096-9837(199603)21:3<217::AID-ESP611>3.0.CO;2-U
- Nanson, G. C., Price, D. M., Jones, B. G., Maroulis, J. C., Coleman, M., Bowman, H., Cohen, T. J., Pietsch, T. J., & Larsen, J. R. (2008). Alluvial evidence for major climate and flow regime changes during the middle and late Quaternary in eastern central Australia. Geomorphology, 101(1–2), 109–129. https://doi.org/https://doi.org/10.1016/j.geomorph.2008.05.032
- Nanson, G. C., Rust, B. R., & Taylor, G. (1986). Coexistent mud braids and anastomosing channels in an arid-zone river: Cooper Creek, central Australia. Geology, 14(2), 175–178. https://doi.org/https://doi.org/10.1130/0091-7613(1986)14<175:CMBAAC>2.0.CO;2
- Nanson, G. C., Tooth, S., & Knighton, A. D. (2002). A global perspective on dryland rivers: Perceptions, misconceptions, and distinctions. L. J. Bull & M. J. Kirkby Eds., Dryland Rivers: Hydrology and Geomorphology of Semi-Arid Channels. (17–54). John Wiley & Sons.
- Nawaz, M., & Wasson, R. (2019). Sediment transfer in an extremely low gradient, low-relief and highly buffered system: Darwin Harbour catchment, northern Australia. Australian Journal of Earth Sciences, 67(3), 351–359. https://doi.org/https://doi.org/10.1080/08120099.2019.1659415
- Osterkamp, W. R., & Friedman, J. M. (2000). The disparity between extreme rainfall events and rare floods - with emphasis on the semi‐arid American West. Hydrological Processes, 14(16–17), 2817–2829. https://doi.org/https://doi.org/10.1002/1099-1085(200011/12)14:16/17<2817::AID-HYP121>3.0.CO;2-B
- Pain, C., Gregory, L., Wilson, P., & McKenzie, N. (2011). The physiographic regions of Australia: Explanatory notes. Geoscience Australia and CSIRO. https://publications.csiro.au/publications/publication/PIcsiro:EP113843
- Parsons, M., Thoms, M. C., & Norris, R. H. (2004). Development of a standardised approach to river habitat assessment in Australia. Environmental Monitoring and Assessment, 98(11–3), 109–130. https://doi.org/https://doi.org/10.1023/B:EMAS.0000038182.03176.97
- Pearson, M. R., Reid, M. A., Miller, C., & Ryder, D. (2020). Comparison of historical and modern river surveys reveal changes to waterhole characteristics in an Australian dryland river. Geomorphology, 356, 107089. https://doi.org/https://doi.org/10.1016/j.geomorph.2020.107089
- Pell, S. D., Chivas, A. R., & Williams, I. S. (2000). The Simpson, Strzelecki and Tirari Deserts: Development and sand provenance. Sedimentary Geology, 130(1–2), 107–130. https://doi.org/https://doi.org/10.1016/S0037-0738(99)00108-6
- Phelps, D. G., Lynes, B., Connelly, P. T., Horrocks, D. J., Fraser, G. W., & Jeffery, M. R. (2007). Sustainable Grazing in the Channel Country Floodplains; Final Report, project NBP 329. Meat and Livestock Australia. www.mla.com.au/research-and-development/reports/2007/sustainable-grazing-in-channel-country-floodplains-phase-2
- Phelps, D., Lynes, B., Forrest, K., Connelly, P., & Horrocks, D., 2006a. Cooper Creek Catchment Flood Rules of Thumb. Queensland Department of Agriculture and Forestry. https://futurebeef.com.au/document-library
- Phelps, D., Lynes, B., Forrest, K., Connelly, P., & Horrocks, D., 2006b. Diamantina River Catchment Flood Rules of Thumb. Queensland Department of Agriculture and Forestry. https://futurebeef.com.au/document-library
- Pickup, G. (1985). The erosion cell - a geomorphic approach to landscape classification in range assessment. The Rangeland Journal, 7(2), 114–121. https://doi.org/https://doi.org/10.1071/RJ9850114
- Pickup, G. (1991). Event frequency and landscape stability on the floodplain systems of arid central Australia. Quaternary Science Reviews, 10(5), 463–473. https://doi.org/https://doi.org/10.1016/0277-3791(91)90007-H
- Pickup, G., Allan, G., & Baker, V. R. (1988). History, palaeochannels and palaeofloods of the Finke River, central Australia. In R. F. Warner (Ed.). Fluvial geomorphology of Australia (pp. 177–200). Academic Press.
- Pietsch, T. J., & Nanson, G. C. (2011). Bankfull hydraulic geometry; the role of in-channel vegetation and downstream declining discharges in the anabranching and distributary channels of the Gwydir distributive fluvial system, southeastern Australia. Geomorphology, 129(1–2), 152–165. https://doi.org/https://doi.org/10.1016/j.geomorph.2011.01.021
- Pillans, B. (2005). Geochronology of the Australian regolith. In R. R. Anand & P. de Broekert (Eds.), Regolith landscape evolution across Australia: A compilation of regolith landscape case studies with regolith landscape evolution models (pp. 41–52). Cooperative Research Centre for Landscape Environment and Mineral Exploration (CRC LEME). http://crcleme.org.au/Pubs/monographs.html
- Polvi, L. E., Lind, L., Persson, H., Miranda-Melo, A., Pilotto, F., Su, X., & Nilsson, C. (2020). Facets and scales in river restoration: Nestedness and interdependence of hydrological, geomorphic, ecological, and biogeochemical processes. Journal of Environmental Management, 265, 110288. https://doi.org/https://doi.org/10.1016/j.jenvman.2020.110288
- Pringle, H., & Tinley, K. (2003). Are we overlooking critical geomorphic determinants of landscape change in Australian rangelands? Ecological Management & Restoration, 4(3), 180–186. https://doi.org/https://doi.org/10.1046/j.1442-8903.2003.00154.x
- Prosser, I. P., Chappell, J., & Gillespie, R. (1994). Holocene valley aggradation and gully erosion in headwater catchments, south-eastern highlands of Australia. Earth Surface Processes and Landforms, 19(5), 465–480. https://doi.org/https://doi.org/10.1002/esp.3290190507
- Quigley, M. C., Sandiford, M., & Cupper, M. L. (2007a). Distinguishing tectonic from climatic controls on range‐front sedimentation. Basin Research, 19(4), 491–505. https://doi.org/https://doi.org/10.1111/j.1365-2117.2007.00336.x
- Quigley, M., Sandiford, M., Fifield, K., & Alimanovic, A. (2007b). Bedrock erosion and relief production in the northern Flinders Ranges, Australia. Earth Surface Processes and Landforms, 32(6), 929–944. https://doi.org/https://doi.org/10.1002/esp.1459
- Radke, B., & Ransley, T. (2020). Connectivity between Australia’s Great Artesian Basin, underlying basins, and the Cenozoic cover. Hydrogeology Journal, 28(1), 43–56. https://doi.org/https://doi.org/10.1007/s10040-019-02075-z
- Ransley, T. R., Radke, B. M., Feitz, A. J., Kellett, J. R., Owens, R., Bell, J., Stewart, G., & Carey, H. (2015). Hydrogeological Atlas of the Great Artesian Basin. Geoscience Australia. https://doi.org/https://doi.org/10.11636/9781925124668
- Ransley, T. R., & Smerdon, B. D. (2012). Hydrostratigraphy, hydrogeology and system conceptualisation of the Great Artesian Basin: A technical report to the Australian Government from the CSIRO Great Artesian Basin Water Resource Assessment. Canberra: CSIRO, Water for a Healthy Country Flagship. https://doi.org/https://doi.org/10.4225/08/584c457b1676f
- Rey, P. F. (2013). Opalisation of the Great Artesian Basin (central Australia): An Australian story with a Martian twist. Australian Journal of Earth Sciences, 60(3), 291–314. https://doi.org/https://doi.org/10.1080/08120099.2013.784219
- Risbey, J. S., Pook, M. J., McIntosh, P. C., Wheeler, M. C., & Hendon, H. H. (2009). On the remote drivers of rainfall variability in Australia. Monthly Weather Review, 137(10), 3233–3253. https://doi.org/https://doi.org/10.1175/2009MWR2861.1
- Roberts, J., & Marston, F. (2011). Water regime for wetland and floodplain plants: A source book for the Murray-Darling Basin. National Water Commission.
- Rust, B. R. (1981). Sedimentation in an arid-zone anastomosing fluvial system; Cooper’s Creek, central Australia. Journal of Sedimentary Research, 51(3), 745–755 doi:https://doi.org/10.1306/212F7D97-2B24-11D7-8648000102C1865D.
- Rust, B. R., & Nanson, G. C. (1989). Bedload transport of mud as pedogenic aggregates in modern and ancient rivers. Sedimentology, 36(2), 291–306. https://doi.org/https://doi.org/10.1111/j.1365-3091.1989.tb00608.x
- Saco, P. M., Willgoose, G. R., & Hancock, G. R. (2007). Eco-geomorphology of banded vegetation patterns in arid and semi-arid regions. Hydrology and Earth System Sciences Discussions, European Geosciences Union, 11(6), 1717–1730. https://doi.org/https://doi.org/10.5194/hess-11-1717-2007
- Sandercock, P. J., Hooke, J. M., & Mant, J. M. (2007). Vegetation in dryland river channels and its interaction with fluvial processes. Progress in Physical Geography, 31(2), 107–129. https://doi.org/https://doi.org/10.1177/0309133307076106
- Sandiford, M., Lawrie, K., & Brodie, R. S. (2020). Hydrogeological implications of active tectonics in the Great Artesian Basin, Australia. Hydrogeology Journal, 28(1), 57–73. https://doi.org/https://doi.org/10.1007/s10040-019-02046-4
- Schellart, W. P., & Spakman, W. (2015). Australian plate motion and topography linked to fossil New Guinea slab below Lake Eyre. Earth and Planetary Science Letters, 421, 107–116. https://doi.org/https://doi.org/10.1016/j.epsl.2015.03.036
- Schmarr, D. W., Mathwin, R., & Cheshire, D. L. (2017). Aquatic Ecology Assessment and Analysis of the Diamantina River Catchment: Lake Eyre Basin, South Australia. Report by the South Australian Research and Development Institute to the South Australian Arid Lands Natural Resources Management Board. www.landscape.sa.gov.au/saal/water/managing-water-resources/Lake_Eyre_Basin_catchment_projects .
- Schmarr, D. W., Mathwin, R., Cheshire, D. L., & McNeil, D. G. (2013). Aquatic ecology assessment and analysis of the Cooper Creek: Lake Eyre Basin, South Australia. Report by the South Australian Research and Development Institute (Aquatic Sciences) to the South Australian Arid Lands Natural Resources Management Board. www.landscape.sa.gov.au/saal/water/managing-water-resources/Lake_Eyre_Basin_catchment_projects ; SAAL-NRM
- Senior, D. (1968). Durham Downs, Queensland. 1: 250,000 Map Sheet SG/54-15, Geological Map and Explanatory Notes. Bureau of Mineral Resources. Geology and Geophysics, Canberra.
- Shannon, J., Richardson, R., & Thornes, J. (2002). Modelling event-based fluxes in ephemeral streams. In L. J. Bull & M. J. Kirkby (Eds.). Dryland Rivers: Hydrology and Geomorphology of Semi-Arid Channels, (pp. 128–172). John Wiley & Sons.
- Sheldon, F., Brunner, P., & Hoggett, A. (2004). Classification of the Lake Eyre Basin Rivers. (Project Background Document 2; Lake Eyre Basin Rivers Assessment Methodology Development). Report from Centre for Riverine Landscapes, Griffith University, to Land & Water Australia.
- Sheldon, F., McKenzie-Smith, F., Brunner, P., Hoggett, A., Shephard, J., Bunn, S., … Phelps, D. (2005). Project Methods for Assessing the Health of Lake Eyre Basin Rivers. (Final Report, Lake Eyre Basin Rivers Assessment Methods Development). Report by the Centre for Riverine Landscapes, Griffith University, to Land & Water Australia.
- Shilton, P., Norman, P., Stone, B., & Carey, B. (2015). Soil conservation guidelines for Queensland. Department of Science, Information Technology and Innovation. https://www.publications.qld.gov.au/dataset/soil-conservation-guidelines
- Silcock, J. (2009). Identification of Permanent Refuge Waterbodies in the Cooper Creek and Georgina-Diamantina River Catchments for Queensland and South Australia. South Australian Arid Lands Natural Resource Management Board. www.landscape.sa.gov.au/saal/water/managing-water-resources/surface-water
- Stevens, B. P. J. (1991). Some aligned claypans in the Strzelecki dunefield, Central Australia. Australian Journal of Earth Sciences, 38(4), 485–495. https://doi.org/https://doi.org/10.1080/08120099108727985
- Taylor, C. F. H. (1999). The role of overbank flow in governing the form of an anabranching river: The Fitzroy River, northwestern Australia. In N. D. Smith, and J. Rogers (Eds.), Fluvial Sedimentology VI (pp. 77–92) ISBN13 9780632053544; Blackwell Science.
- Tedford, R. H., Williams, D. L. G., & Wells, R. T. (1986). Late Cainozoic sediments and fossil vertebrates. In R. T. Wells, and R. A. Callen Eds., The Lake Eyre Basin - Cainozoic sediments, fossil vertebrates and plants, landforms, silcretes and climatic implications. Australasian Sedimentologists Group Field Guide Series (No. 4) (pp. 42-72). Geological Society of Australia. ISSN 0815-1172
- Teller, J. T., Bowler, J. M., & Macumber, P. G. (1982). Modern sedimentation and hydrology in Lake Tyrrell, Victoria. Journal of the Geological Society of Australia, 29(1–2), 159–175. https://doi.org/https://doi.org/10.1080/00167618208729202
- The Long Paddock (2020a). Queensland’s extended wet and dry periods: Australian rainfall periods (April to March) relative to historical records 1889-2020. Queensland Government Ecosciences precinct. https://www.longpaddock.qld.gov.au/rainfall-poster
- The Long Paddock (2020b). Australia’s variable rainfall. Australian rainfall periods (April to March) relative to historical records 1889-2020. Queensland Government Ecosciences precinct. https://www.longpaddock.qld.gov.au/rainfall-poster
- Thiry, M., Milnes, A. R., Rayot, V., & Simon-coinçon, R. (2006). Interpretation of palaeoweathering features and successive silicifications in the Tertiary regolith of inland Australia. Journal of the Geological Society, 163(4), 723–736. https://doi.org/https://doi.org/10.1144/0014-764905-020
- Thoms, M., Capon, S., Price, R., & Watkins, D., 2009. Lake Eyre Basin Rivers Assessment Implementation Plan Project: Proposed LEB Rivers Assessment Methodology (Milestone 2 Report). Report by Kir-ganai Research to the Australian Department of the Environment, Water, Heritage and the Arts.
- Thoms, M., Parsons, M., & Southwell, M. (2016). The physical template of Australia’s rivers. In S. Capon, C. James, & M. Reid (Eds.). Vegetation of Australian riverine landscapes: Biology, ecology and management. (pp. 5–26). CSIRO Publishing.
- Tongway, D. J., & Ludwig, J. A. (1994). Small-scale resource heterogeneity in semi-arid landscapes. Pacific Conservation Biology, 1(3), 201–208. https://doi.org/https://doi.org/10.1071/PC940201
- Tongway, D. J., & Ludwig, J. A. (2001). Theories on the origins, maintenance, dynamics, and functioning of banded landscapes. In D. J. Tongway, C. Valentin, & J. Seghieri (Eds.), Banded vegetation patterning in arid and semiarid environments: Ecological processes and consequences for management (pp. 20–31). Springer. https://doi.org/https://doi.org/10.1007/978-114613-0207-0
- Tooth, S. (1999). Floodouts in central Australia. In A. J. Miller, and A. Gupta (Eds.). Varieties of Fluvial Form (pp. 219–247). New York: John Wiley & Sons. ISBN 10 0471973513
- Tooth, S. (2000a). Process, form and change in dryland rivers: A review of recent research. Earth-Science Reviews, 51(1–4), 67–107 doi:https://doi.org/10.1016/S0012-8252(00)00014-3.
- Tooth, S. (2000b). Downstream changes in dryland river channels: The Northern Plains of arid central Australia. Geomorphology, 34(1–2), 33–54. https://doi.org/https://doi.org/10.1016/S0169-555X(99)00130-0
- Tooth, S. (2005). Splay formation along the lower reaches of ephemeral rivers on the Northern Plains of arid central Australia. Journal of Sedimentary Research, 75(4), 636–649. https://doi.org/https://doi.org/10.2110/jsr.2005.052
- Tooth, S., & Nanson, G. C. (1995). The geomorphology of Australia’s fluvial systems: Retrospect, perspect and prospect. Progress in Physical Geography, 19(1), 35–60. https://doi.org/https://doi.org/10.1177/030913339501900103
- Tooth, S., & Nanson, G. C. (2004). Forms and processes of two highly contrasting rivers in arid central Australia, and the implications for channel-pattern discrimination and prediction. Geological Society of America Bulletin, 116(7–87), 802–816. https://doi.org/https://doi.org/10.1130/B25308.1
- Tooth, S., & Nanson, G. C. (2011). Distinctiveness and diversity of arid zone river systems. In D. S. Thomas, Ed. Arid zone geomorphology: Process, form and change in drylands. 3rd ed., (pp. 270–300). John Wiley & Sons. https://doi.org/https://doi.org/10.1002/9780470710777
- Trewin, D. (2006). Climatic aspects of Australia’s deserts. In D. Trewin (Ed.), Year Book Australia; Number 88. Australian Bureau of Statistics. ISSN 0312–4746.
- Ullyott, J. S., & Nash, D. J. (2016). Distinguishing pedogenic and non-pedogenic silcretes in the landscape and geological record. Proceedings of the Geologists’ Association, 127(3), 311–319. https://doi.org/https://doi.org/10.1016/j.pgeola.2016.03.001
- Unmack, P. J. (2001). Fish persistence and fluvial geomorphology in central Australia. Journal of Arid Environments, 49(4), 653–669. https://doi.org/https://doi.org/10.1006/jare.2001.0813
- Valentin, C., d’Herbès, J. M., & Poesen, J. (1999). Soil and water components of banded vegetation patterns. Catena, 37(1–2), 1–24. https://doi.org/https://doi.org/10.1016/S0341-8162(99)00053-3
- Valentin, C., & d’Herbès, J. M. (1999). Niger tiger bush as a natural water harvesting system. Catena, 37(1–2), 231–256. https://doi.org/https://doi.org/10.1016/S0341-8162(98)00061-7
- Van Etten, E. J. B. (2009). Inter-annual rainfall variability of arid Australia: Greater than elsewhere? Australian Geographer, 40(1), 109–120. https://doi.org/https://doi.org/10.1080/00049180802657075
- Vanderstaay, L. (2000a). Geology and geomorphology of Western Queensland ( Western Queensland Best Practice Guidelines, Technical Notes WQ31). Queensland Government Department of Main Roads. https://tmr.qld.gov.au
- Vanderstaay, L. (2000b). Soils of Western Queensland ( Western Queensland Best Practice Guidelines, Technical Notes WQ32). Queensland Government Department of Main Roads. https://tmr.qld.gov.au
- Waclawik, V. G., Lang, S. C., & Krapf, C. B. E. (2008). Fluvial response to tectonic activity in an intra-continental dryland setting: The Neales River, Lake Eyre, Central Australia. Geomorphology, 102(1), 179–188. https://doi.org/https://doi.org/10.1016/j.geomorph.2007.06.021
- Wakelin-King, G. A. (1999). Banded mosaic (“tiger bush”) and sheetflow plains: A regional mapping approach. Australian Journal of Earth Sciences, 46(1), 53–60 doi:https://doi.org/10.1046/j.1440-0952.1999.00685.x.
- Wakelin-King, G. A. (2011). Geomorphological assessment and analysis of the Neales Catchment. Port Augusta: South Australian Arid Lands Natural Resources Management Board. www.landscape.sa.gov.au/saal/water/managing-water-resources/Lake_Eyre_Basin_catchment_projects; Report by Wakelin Associates to the SAAL-NRM.
- Wakelin-King, G. A. (2013). Geomorphological assessment and analysis of the Cooper Creek catchment (SA section). Port Augusta: South Australian Arid Lands Natural Resources Management Board. www.landscape.sa.gov.au/saal/water/managing-water-resources/Lake_Eyre_Basin_catchment_projects; Report by Wakelin Associates to the SAAL-NRM.
- Wakelin-King, G. A. (2015a). Geomorphology of Finke River and Arckaringa Creek: the bedload rivers. Report by Wakelin Associates to the South Australian Department of Environment, Water and Natural Resources; Lake Eyre Basin River Monitoring Project. DEWNR Technical report 2015/51. Adelaide: Department of Environment, Water and Natural Resources. https://www.researchgate.net/profile/Gresley-Wakelin-King
- Wakelin-King, G. A. (2015b). Geomorphology of the Thomson River, Queensland: overview and comparison with Cooper Creek; a report to the Department of Environment, Water and Natural Resources, South Australia; Lake Eyre Basin River Monitoring Project. DEWNR Technical report 2015/52. Adelaide: Department of Environment, Water and Natural Resources. https://www.researchgate.net/profile/Gresley-Wakelin-King
- Wakelin-King, G. A. (2017). Geomorphology of the Diamantina River Catchment (SA). Port Augusta: South Australian Arid Lands Natural Resources Management Board. www.landscape.sa.gov.au/saal/water/managing-water-resources/Lake_Eyre_Basin_catchment_projects; Report by Wakelin Associates to the SAAL-NRM
- Wakelin-King, G. A., & Amos, K. J. (2016). A time-slice of the Lake Eyre Basin: Sand/mud depositional geometries in a diverse lowstand endorheic drylands setting. W. Lodwick Ed., Eastern Australasian Basins Symposium, A Powerhouse Emerges: Energy for the Next Fifty Years. (97–113). Petroleum Exploration Society of Australia, Special Publication.
- Wakelin-King, G. A., & Webb, J. A. (2007a). Threshold-dominated fluvial styles in an arid-zone mud-aggregate river: Fowlers Creek, Australia. Geomorphology, 85(1–2), 114–127. https://doi.org/https://doi.org/10.1016/j.geomorph.2006.03.011
- Wakelin-King, G. A., & Webb, J. A. (2007b). Upper-flow-regime mud floodplains, lower-flow-regime sand channels: Sediment transport and deposition in a drylands mud-aggregate river. Journal of Sedimentary Research, 77(9), 702–712. https://doi.org/https://doi.org/10.2110/jsr.2007.074
- Wasson, R. J., Fitchett, K., Mackey, B., & Hyde, R. (1988). Large-scale patterns of dune type, spacing and orientation in the Australian continental dunefield. Australian Geographer, 19(1), 89–104. https://doi.org/https://doi.org/10.1080/00049188808702952
- Watson, P. 1983. This precious foliage: A study of the Aboriginal psycho-active drug pituri. Oceania Monograph 26. Sydney: University of Sydney. ISBN 0867581190.
- Watson, P. 1998. Frontier lands and pioneer legends: How pastoralists gained Karuwali land. Sydney: Allen & Unwin. ISBN: 1-86448-495-0.
- Wells, R. T., & Callen, R. A. (1986). Regional structural setting; Lake Eyre and Birdsville Basins. In R. T. Wells & R. A. Callen (Eds.). The Lake Eyre Basin — Cainozoic sediments, fossil vertebrates and plants, landforms, silcretes and climatic implications (pp. 6-21). Australasian Sedimentologists Group Field Guide Series No. 4. Geological Society of Australia, Sydney. ISSN 0815-1172
- Willett, S. D., McCoy, S. W., Perron, J. T., Goren, L., & Chen, C. Y. (2014). Dynamic reorganization of river basins. Science, 343(6175), 1248765. https://doi.org/https://doi.org/10.1126/science.1248765
- Williams, G. E. (1970). The central Australian stream floods of February-March 1967. Journal of Hydrology, 11(2), 185–200. https://doi.org/https://doi.org/10.1016/0022-1694(70)90103–4
- Williams, G. E. (1971). Flood deposits of the sand-bed ephemeral streams of Central Australia. Sedimentology, 17(1–2), 1–40. https://doi.org/https://doi.org/10.1111/j.1365-3091.1971.tb01128.x
- Wopfner, H., & Twidale, C. R. (1967). Geomorphological history of the Lake Eyre basin. In J. N. Jennings & J. A. Mabbutt (Eds.). Landform Studies from Australia and New Guinea (pp. 118–143). Australian National University Press
- Wray, R. A. L. (2009). Palaeochannels of the Namoi River Floodplain, New South Wales, Australia: The use of multispectral Landsat imagery to highlight a Late Quaternary change in fluvial regime. Australian Geographer, 40(1), 29–49. https://doi.org/https://doi.org/10.1080/00049180802656952