ABSTRACT
A suite of soft-sediment deformation structures occurs within the basal sands and gravels in the non-marine Pleistocene succession in the Noarlunga Embayment of the Cenozoic St Vincent Basin south of Adelaide, South Australia, the significance of which has hitherto gone unrecognised. The inclusion of large, isolated blocks of well-bedded sands randomly encased within fluidised, structureless clayey sands indicates that the soft-sediment deformation was intense, occurring soon after deposition by liquefaction of a still saturated sediment. Seismic activity has been, and continues to be, a regular occurrence along the adjacent Adelaide fold belt. The intensity of the deformation is comparable to the thixotropic response to earthquake activity observed during the Christchurch (New Zealand) earthquakes of 2010 and 2011, the earthquakes and aftershocks near Quorn, South Australia, in January 1887, in the southeast of South Australia in May 1899, and west of Adelaide in April 1954. This, however, is the first record of an interpreted seismite in the St Vincent Basin, although its full extent is yet to be mapped.
Introduction
Unusual soft-sediment deformation structures occur in a sand unit near the base of a sequence of non-marine Pleistocene sediments exposed in coastal cliffs at Witton Bluff, south of Adelaide, South Australia (). The cliffs hem the eastern side of Gulf St Vincent, where the Pleistocene terrestrial sediments unconformably overlie older Cenozoic marine limestones and clays that together fill in the Noarlunga Embayment of the former St Vincent Basin (). The structures were first described as intraformational slumping by May (Citation1992) when mapping and sampling the Pleistocene succession in the coastal cliffs between Sellicks Beach and Hallett Cove (). However, recent lithostratigraphic and regolith studies in the area (May & Milnes, Citation2024) led to a re-examination of the deformation structures and a re-interpretation of their likely origin. The study site, Witton Bluff, lies close to the northern edge of the Noarlunga Embayment.
Figure 1. a: Epicentres of earthquakes of magnitude 2 or greater recorded in eastern South Australia until 2016 (from Preiss, Citation2019). b: locality map of the Noarlunga Embayment on the eastern side of Gulf St Vincent south of Adelaide (modified from Smith et al., Citation2016). c: satellite view showing the en echelon splay faults at the southern end of the Adelaide fold belt and the Noarlunga and Willunga Embayments (modified from Sandiford, Citation2003). The Witton Bluff coastal cliffs lie towards the northern limit of the sedimentary fill of the Noarlunga Embayment.
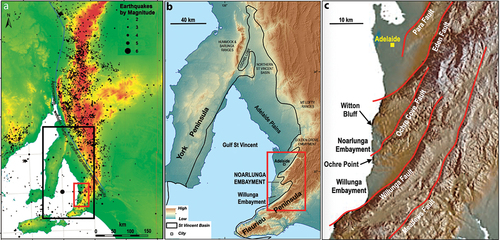
Figure 2. a: N–S coastal transect across the Noarlunga and Willunga Embayments showing locations of the cliff sections in relation to the geology, including the main faults defining the southern margins of the embayments (May & Milnes, Citation2024). Trend-lines represent bedding in Cenozoic sediments; vertical lines represent Neoproterozoic bedrock (modified from McGowran et al., Citation2016). b: view of the cliff section from Witton Bluff southwards. Late Eocene Blanche Point Formation (white) crops out just above beach level at the base of the cliff. The unconformably overlying Pleistocene non-marine sequence is brown-coloured. (Google earth image).
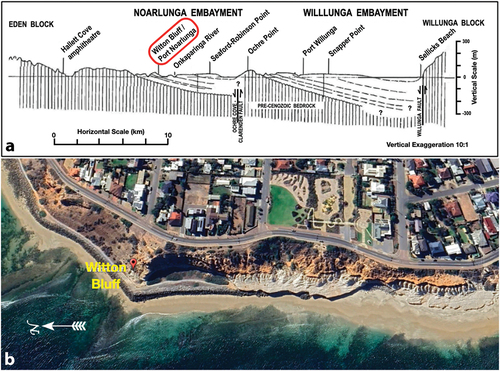
Geological setting and general stratigraphy
The generally north-south trending range of hills that extends over 600 km from the Fleurieu Peninsula in the south to the northern Flinders Ranges constitutes the Adelaide fold belt (deformed Neoproterozoic and early Palaeozoic metasediments forming Mount Lofty and Flinders Ranges) and is one of the most seismically active zones in Australia (). Although small earthquakes are frequent, earthquakes large enough to cause liquefaction (~5 magnitude, Richter scale) occur, on average, about once every 5–10 years (Dix, Citation2013; McCue, Citation2013).
The modern sea cliffs bounding the Gulf St Vincent display excellent sections through the Cenozoic marine and overlying non-marine sedimentary fill in the St Vincent Basin (McGowran & Alley, Citation2008; Stuart, Citation1969, Citation1970). The basin is ca 15000 km2 in area and is defined by a series of roughly N–S arcuate early Palaeozoic faults that were reactivated in the Late Palaeogene as the separation of Australia and Antarctica progressed. A compressional tectonic regime that formed the St Vincent Basin in Proterozoic, Cambrian and Carboniferous-Permian bedrock and generated sub-basins or embayments (asymmetrical tectonic valleys including the Willunga and Noarlunga Embayments and Adelaide Plains Sub-basin), was responsible for periodic disruption of sedimentation and later uplift of the adjacent highlands of Fleurieu Peninsula and Yorke Peninsula (; McGowran & Alley, Citation2008; McGowran et al., Citation2016; Preiss, Citation2019). The earliest deposits are essentially non-marine Middle Eocene sediments overlain by marginal marine and marine limestones ranging in age up to the Late Pliocene (Cooper, Citation1985; McGowran et al., Citation2016; Stuart, Citation1969, Citation1970). The seas then regressed and a swathe of terrestrial fluvial and alluvial sediments progressively filled the basin.
The study site lies in the northern portion of the sedimentary fill in the Noarlunga Embayment () that trends northeastward beyond the eastern margin of the St Vincent Basin. The embayment is bounded on its southeastern margin by the Ochre Cove–Clarendon Fault, one of the suite of en echelon splay faults developed towards the southern extremity of the Adelaide fold belt. The lithostratigraphy of the Quaternary sequence exposed in the cliffs is complicated by groundwater-induced secondary alterations. The original stratigraphic subdivisions of Ward (Citation1966) were subsequently revised by Phillips (Citation1988) and May (Citation1992) and it is this latter stratigraphy (summarised by May & Milnes, Citation2024) that is utilised herein ().
Figure 3. Stratigraphic sequence in the cliff sections in the immediate vicinity of Witton Bluff. a: just south of the Bluff: Robinson Point Formation gravels and sands unconformably overlie late Eocene Blanche Point Formation limestones and siliceous marls. A concretionary carbonate horizon separates the Robinson Point Formation from the overlying reddish Neva Clay Member of the Ngaltinga Formation and a carbonate blanket and surface soil. b: north of Witton Bluff, Robinson Point Formation gravels and sands with an interbed of Burnham Limestone below a pink haematitic zone of alunite-halloysite alteration. Thickly bedded sands, Fe-mottled, extend almost to the carbonate blanket at the top of the section. c: thinly bedded Robinson Point Formation sands at the bottom of the section; thick sand unit with significant soft-sediment deformation above. Note disrupted blocks of thinly bedded sand scattered throughout an unstructured sand matrix. Carbonate blanket caps the section.
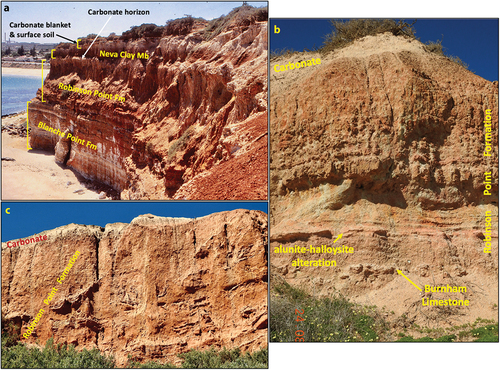
Stratigraphy exposed in the cliff section
Distinctive white sediments at the base of the cliff section between Witton Bluff and Port Noarlunga () are fossiliferous limestones and silica-rich marls of the Late Eocene Blanche Point Formation. They are unconformably overlain by gravels, sands and sandy clays of the Pleistocene Robinson Point Formation (; May & Milnes, Citation2024). In the cliff section, the Robinson Point Formation essentially comprises:
Figure 4. Composite sketch of the Pleistocene succession in the coastal cliffs between Witton Bluff and Port Noarlunga (after May, Citation1992) with photographs of representative lithologies.
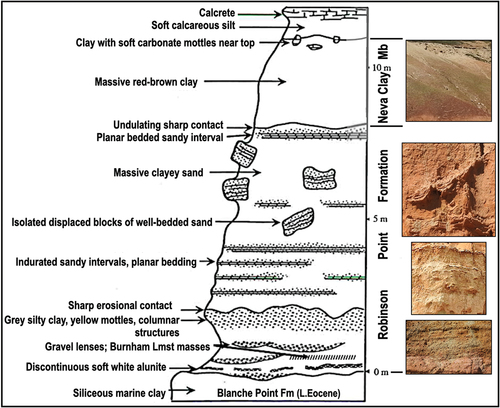
a basal layer of parallel-bedded to shallowly channelled sandstones with layers of rounded granules and is characterised by cavernous weathering;
a light orange–brown clay, generally less than 1 m thick, conformable at the base but with an irregular upper boundary; then, overlying an erosional surface,
a sequence, averaging 1 m thick, of parallel-bedded to shallowly channelled, clays and sands, often with rounded granules, that grades into
essentially structureless clayey sands, 5–7 m thick, within which occur isolated blocks of indurated, parallel-bedded sands at high angles, some of which display ball-and-pillow-like structures suggestive of early post-sedimentation loading.
Capping these clayey sands of the Robinson Point Formation is an interval of red-brown clays, up to 4 m thick, assigned to the Neva Clay Member of the Ngaltinga Formation (May & Milnes, Citation2024). Locally sandy or marly and displaying a characteristic self-mulching surface (composed of easily disturbed, small aggregates), these clays can be interpreted as floodplain/overbank deposits distal to alluvial fans (Phillips & Milnes, Citation1988).
On the southern side of Witton Bluff, a massive to platy carbonate bed of variable thickness (up to 60 cm) occurs between the Robinson Point Formation and the Neva Clay (); this is not present on the northern side where there is a complication due to significant disruption of the sequence. Carbonate mottles and blotches occur in the upper parts of the Neva Clay Member immediately below a calcareous silt capped by calcrete and a surface soil mantle. The latter are part of a widespread blanket of carbonate silts and calcretes in the region (Milnes & Hutton, Citation1983; Phillips & Milnes, Citation1988).
On the northern side of Witton Bluff, near the base of the Robinson Point Formation, is a thin horizon comprising gravel lenses up to 30 cm thick within which occur sporadic masses of shelly Late Pliocene Burnham Limestone (). The sandy units are, generally, horizontally bedded and often indurated although rare tabular cross-beds are observed. Thin horizontal seams of alunite in the gritty sands above are associated with a distinctive pink-coloured haematitic impregnation, which everywhere characterises this zone of secondary alteration (May & Milnes, Citation2024). The limestone is not easily located in the vertical cliff section on the southern side of the bluff, but an unusually thick (20 cm) seam of chalky, white alunite in association with pink haematitic alteration is conspicuous near the unconformity at the base of the Robinson Point Formation. The alunite is laterally continuous for several hundred metres and typically displays, on its underside, rounded, bulbous masses that protrude into the underlying clays. The alunite seam grades into isolated small nodules as it peters out towards the south.
Sandy units near the base of the Robinson Point Formation both north and south of Witton Bluff are generally thin, planar-bedded and somewhat indurated, although rare tabular cross-beds occur locally (). Above this interval, there is a ~5 m-thick zone in which the sands have been considerably disrupted. Blocks of thinly bedded sand up to 1.5 m across are often steeply tilted and scattered randomly within otherwise massive, unstructured clayey sands. Bedding within the blocks cannot be traced laterally, and adjacent blocks are unrelated. Underlying sediments of similar lithology and overlying clays remain undisturbed. May (Citation1992) produced a composite sketch of the stratigraphy in the general locality () and suggested that the soft-sediment deformation was a result of intraformational slumping of well-bedded and cohesive sandy sediments.
Characteristics of the deformation structures
A close examination of the Witton Bluff cliff face, however, has identified a variety of soft-sediment structures, including:
Pillow structures or thixotropic bowls/wedges: well-bedded sands deformed into shallow synclines, occasionally showing increasing deformation with depth . One persistent section displays cavernous weathering within which such pillows, akin to “pear-drop” structures (Scott & Price, Citation1988), deepen into wedges and pillars reminiscent of liquefaction-induced wedges and fissures of the Pleistocene age described by Montenat et al. (Citation2007) and Thorson et al. (Citation1986), respectively, ;
Figure 5. a: Well-bedded sands (wb) deformed into a thixotropic bowl isolated within fluidised clayey sand (fs); near Port Noarlunga. b, c: depictions of the lower unit of disturbed well-bedded sands in the Robinson Point formation displaying cavernous weathering; near Port Noarlunga. Thixotropic wedges (tw) are indicated. d: thixotropic wedge (for comparison with b, c) in Pleistocene littoral calcarenite seismite from Calabria, Italy (from Montenat et al., Citation2007).
Dish Structures: several detached, bowl-shaped remnants of deformed well-bedded sands () up to 1 m in width are isolated at high angles within a matrix of structureless clayey sands. Incipient intrusive flame structures separate the bowls;
Figure 6. Isolated blocks of well-bedded sands floating within a surrounding matrix of structureless clayey sand injected along fluidisation conduits (dashed yellow arrows). Note step-faulting of indurated sands in a (dashed blue lines). White dotted lines in b & c indicate bedding trends. White dashed lines in b indicate faults. a, b: from near Port Noarlunga. c: from Witton Bluff. d: Fluidisation conduit in sandy late Messinian seismite from Alicante, Spain (from Montenat et al., Citation2007).
Liquefaction dykes: a suite of essentially vertical dyke-like structures ranging from pipes to pillars and conduits up which fluidised clayey sand flowed ();
Step faulting: brittle deformation of partially indurated well-bedded sands (): a form of “fault grading” as defined by Seilacher (Citation1969).
Interpretation
Soft-sediment deformation in unconsolidated to partially consolidated sediments results from their dewatering, which can be a consequence of sedimentary loading, fluctuating groundwater tables, gravity- or storm-induced slope failure (turbidites), tsunamis and tidal activity or seismic/earthquake activity. From a review of the literature on soft-sediment deformation resulting from earthquakes (Alfaro et al., Citation2010; Boukhedimi et al., Citation2017; Gladkov et al., Citation2016; Guessoum et al., Citation2000; Hilbert-Wolf et al., Citation2016; Hoffmann & Reicherter, Citation2012; Menegoni et al., Citation2023; Mohindra & Thakur, Citation1998; Moretti, Citation2000; Moretti & Sabato, Citation2007; Pisarska-Jamrozy et al., Citation2018; Rana et al., Citation2013; Scott & Price, Citation1988; Thorson et al., Citation1986), the intensity and pervasiveness of soft-sediment deformation in the alluvial/fluvial sediments in the Witton Bluff cliff section strongly suggests liquefaction by a seismic event.
Recognition of seismites
Sedimentary sequences that have been disrupted by seismic activity (“seismites”) are typified by sedimentary structures that principally involve fluidisation and liquefaction. Moretti and Sabato (Citation2007) and Moretti and van Loon (Citation2014) attempted to define the characteristics of seismites that distinguish them from other causes of fluidisation and liquefaction and concluded that the soft-sediment deformation seemingly “diagnostic” of a “seismite” should:
be laterally continuous within specific sedimentary horizons, often at different levels separated by undeformed horizons;
display an intensity that varies laterally, depending on the distance from the epicentre;
occur in a sedimentary basin typified by tectonic activity at the time of deformation; and
be typical of modern seismically induced soft-sediment deformation structures.
Montenat et al. (Citation2007) and Boukhedimi et al. (Citation2017) attempted to classify the various soft-sediment deformation structures present in a typical seismite, finding that they fall into five main categories. These include:
Seismo-slumping, involving the disruption of horizontally bedded sands resulting from liquefaction;
Thixotropic Bowls and Dykes, which include bowl- to pillar-shaped depressions of well-bedded sands (akin to load-induced ball-and-pillow/dish structures);
Liquefaction/Injection Dykes creating pillars to broad conduits of fluidised sediment;
Foundered blocks of bedded sediments detached from their depositional position and isolated within a structureless liquefaction sand; and, additionally,
Step Faults, a brittle form of “fault grading” (Hoffmann & Reicherter, Citation2012; Moretti & Sabato, Citation2007; Seilacher, Citation1969), can be induced by earthquake activity within bedded indurated sands.
The Witton Bluff sequence as a seismite
Several lines of evidence indicate that the massive sand units that constitute Witton Bluff and its adjacent cliffs represent a thick and extensive seismite deposit. The soft-sediment deformation structures evident include ball-and-pillow loads, isolated pillows (pseudonodules) and dish structures, thixotropic bowls and wedges (), numerous liquefaction/injection conduits (), foundered blocks isolated within structureless clayey sand slurries (), and step faulting (). All these structures are indicative of seismically induced soft-sediment deformation.
Other than the observation made by May (Citation1992), there is no prior record of liquefaction and soft-sediment deformation in the Pleistocene succession in the St Vincent Basin. Nevertheless, our reconnaissance of the cliff sections south of Witton Bluff in the Noarlunga Embayment indicates that the zone of inferred liquefaction extends for some distance. We recognise lithostratigraphic features peculiar to the deformation in the cliffs southwards towards Port Noarlunga () and further south again beyond the mouth of the Onkaparinga River ().
Figure 8. General view of the cliff face near Port Noarlunga showing intense liquefaction-induced soft-sediment deformation disrupting horizon X throughout the sequence above horizon Y, including listric slump faulting (white dashed lines). The cavernous weathering within the indurated unit capped by horizon Z is indicative of distorted bedding while the sequence between horizons Y and Z displays thin layers of soft-sediment deformation between well-bedded layers.
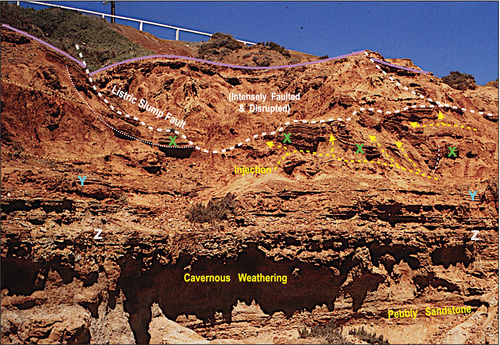
Figure 9. Views of the liquefaction deformation interval (X) in the cliff section south of the Onkaparinga River mouth, southern Noarlunga Embayment. The unconformity marking the top of the Late Eocene Blanche Point Formation and the base of the Robinson Point Formation is indicated by the lower arrow, while the upper arrow indicates the base of the Neva Clay Member of the Ngaltinga Formation. A white carbonate blanket is at the top of the section.
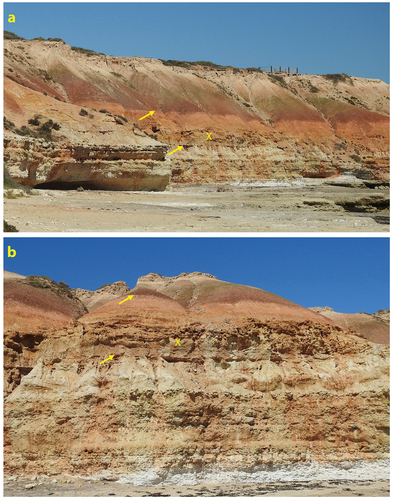
The peculiar weathering characteristics of the sediments in the deformed interval are distinctive, as is the haphazard arrangement of blocks of laminated sand and associated fluid injection features within it. It is noteworthy that in his study of the geology, geomorphology and soils of the Noarlunga and Willunga Embayments, Ward (Citation1966) identified a particular formation in the basal part of the Pleistocene succession on the basis of its conspicuous appearance in the cliff sections, namely coarse red reticulated ferruginous mottling that has hardened on exposure such that outcrops commonly have a rough cavernous appearance, and called it Ochre Cove Formation. He identified a thick section of the unit at Witton Bluff but nominated the Ochre Cove locality in the Willunga Basin as its Type Section. Cavernous weathering is well developed in the liquefaction-deformed interval (), as are Fe mega-mottles, and we speculate that Ward’s mapping of his Ochre Cove Formation could represent the lateral extent of the liquefaction.
The liquefaction of the stratigraphic interval for which we describe the deformation features would have required it to be water-saturated at the time of the causative seismic event. The severe bending, breakage and disorientation of masses of thinly bedded and partly indurated sands, and the thixotropic upwards “escape” of sand slurries, which later dehydrated to form unstructured sand masses, are testament to this. Our working hypothesis is that a causative seismic event occurred soon after deposition of the fluvial-alluvial sediments. We have not recognised soft-sediment deformation features in the underlying sands and gravels or the overlying clays, suggesting that these sediments were not water-saturated at the time, or in the case of the overlying clays, they were deposited after the seismic event.
Evidence of earthquake activity
The intensity of the soft-sediment deformation observed over a thickness of several metres in the Witton Bluff/Port Noarlunga cliff face is comparable to the thixotropic response to earthquake activity that occurred in the saturated layers following the Christchurch, New Zealand, earthquakes of 2010–2011 (Bastin et al., Citation2016; Quigley et al., Citation2013) when more than 200,000 tonnes of liquefied silt upwelled within the city, accompanied by considerable ground movement.
Similar extensive expulsion of liquefied sand from fissures and rabbit holes followed a large (magnitude 6.5) earthquake (same size as the main 2010–2011 Christchurch earthquakes) in the southeast of South Australia in May 1897 (Dix, Citation2013; McCue, Citation2013). Also, landslides were reported east of Adelaide following a magnitude 6 earthquake and aftershocks as recently as February–March 1954 (Dix, Citation2013; McCue, Citation2013) involving the flowage of a significant volume of liquefied grey mud.
In addition to the soft-sediment deformation, listric slump faults are locally evident in the southern (Port Noarlunga) portion of the cliff (). These slumps, however, post-date the deformation caused by liquefaction (although by how long it is uncertain), as isolated blocks and fluidisation conduits are present as inclusions within the fault debris. A similar slump faulting of the Pleistocene succession has also been reported from Sellicks Beach (May & Bourman, Citation1984). While excessive rainfall was an assumed prerequisite to facilitate flowage, the evidence of tectonic activity occurring nearby intermittently during the Miocene, Early and Middle Pleistocene and recent times suggests earthquake activity as a likely trigger.
Interpretation of timing
The Robinson Point Formation containing the liquefaction-deformed stratigraphic interval was contemporaneous with the Late Pliocene Burnham Limestone, at least in the lower parts where the limestone is interbedded with gravels and sands (). Palaeomagnetic data for Fe mottles collected from Hallett Cove (to the north of Witton Bluff) by Pillans and Bourman (Citation1996) from part of this formation (equated by them to Ward’s Ochre Cove Formation) indicated that the haematite crystallised more than 780 ka ago in the Matuyama reverse polarity chron. Deposition of the host sediment significantly pre-dates this (May & Milnes, Citation2024) and so it is likely that the seismic event responsible for the liquefaction deformation occurred during the Early to Middle Pleistocene.
Other direct indicators of palaeoseismicity in the region are rare. McGowran et al. (Citation2016), Preiss (Citation2019) and others described a faulted contact between Cambrian shales, sediments assigned to Ward’s Ochre Cove Formation and younger Pleistocene sediments near Sellicks Beach, adjacent to the Willunga Fault (). However, indirect evidence of seismicity and uplift of the southern Mt Lofty Ranges is considered by some to be the development of substantial alluvial fans adjacent to some major fault traces (Preiss, Citation2019).
Possible tie to Pleistocene glaciations
Several, but by no means all, of the pillar-like structures in the lower deformed layer at the Port Noarlunga end of the cliff outcrop bear a resemblance to ice wedges (see Worsley, Citation2014). To that end, Ward (Citation1965) correlated the Quaternary succession in the Noarlunga and Willunga Embayments of the St Vincent Basin to sea-level movements related to Pleistocene glacial advances and retreats, while Twidale (Citation1988) discussed the interplay of tectonic activity along the various bounding faults and Pleistocene glacial events. Although the Robinson Point Formation is Plio–Pleistocene in age and the principal glacial advances of the Northern Hemisphere occurred in the Middle to Late Pleistocene, Head et al. (Citation2008) suggested that the first significant glacial advance occurred at about 1.2 Ma in the Early Pleistocene, essentially coincident with the Cob Mountain palaeomagnetic event within the Matuyama reverse polarity chron. Furthermore, according to Colhoun and Barrows (Citation2011), the Adelaide region did not experience Pleistocene glacial activity, its extent within Australia being limited to Tasmania and the uplands of the Snowy Mountains, whilst the formation of ice wedges requires temperatures of between −4° and −8°C (Karte, Citation1983). Thus, although a glacial influence for these pillar-like structures cannot be ruled out altogether, their pervasiveness within that singular horizon over a considerable distance with only a few resembling ice wedges strongly suggests that, as with the liquefaction fissures of Thorson et al. (Citation1986) and gravelly “pseudo-ice-wedges” of Black (Citation1983), their formation was the result of a non-glacial seismic mechanism, namely an earthquake.
Implications & conclusions
The seismicity of the broader region of South Australia has been studied extensively and the epicentres of all detected earthquakes mapped (). McCue (Citation2013) noted that the Modified Mercalli Intensity dataset for Adelaide produced an earthquake hazard assessment similar to that for Christchurch, New Zealand, with a return period for a destructive earthquake in the order of a thousand years. However, the measured recurrence rate of earthquakes of magnitude 5 and greater, which have the potential to cause liquefaction in unconsolidated soils and sediments, is 5–10 years. There is every possibility, therefore, that seismicity during the Pleistocene was similar and it is postulated herein that the studied interval with deformation features in the upper part of the Robinson Point Formation in the Witton Bluff/Port Noarlunga cliff exposures is a seismite resultant from just such tectonic activity. We suggest that the seismite be recognised as the Witton Bluff Seismite Member, a distinct and unique facies within the Robinson Point Formation.
Acknowledgments
Our thanks to Professor Kevin McCue for information and comments on a draft of the paper. We also thank two anonymous reviewers for their encouragement to publish and positive comments that helped improve the focus of the paper.
Disclosure statement
No potential conflict of interest was reported by the author(s).
References
- Alfaro, P., Gilbert, L. M., Garcia-Tortosa, M., J, F., De Galdeano, C. S., Galindo-Zaldivar, J.&. L.-G., & C, A. (2010). The significance of giant seismites in the plio-Pleistocene baza palaeo-lake (S Spain). Terra Nova, 22(3), 172–179. https://doi.org/10.1111/j.1365-3121.2010.00930.x
- Bastin, S. H., Bassett, K., Quigley, M. C., Maurer, B., Green, R. A., Bradley, B., & Jacobson, D. (2016). Late Holocene liquefaction at sites of contemporary liquefaction during the 2010–2011 Canterbury earthquake sequence, New Zealand. Seismological Society of America Bulletin, 106(3), 881–903. https://doi.org/10.1785/0120150166
- Black, R. F. (1983). Pseudo-ice-wedge casts of Connecticut, northwestern United States. Quaternary Research, 20(1), 74–89. https://doi.org/10.1016/0033-5894(83)90066-2
- Boukhedimi, M. A., Louni-Hacini, A., Bouhadad, Y., Ritz, J.-F., Machane, D., Benhamouche, A., & Bourenane. (2017). Evidence of seismites in coastal Quaternary deposits of western Oranie (northwestern Algeria). Journal of Seismology, 21(3), 539–549. https://doi.org/10.1007/s10950-016-9616-2
- Colhoun, E. A., & Barrows, T. T. (2011). The glaciation of Australia. Developments in Quaternary Sciences, 15, 1037–1045. https://doi.org/10.1016/B978-0-444-53447-7.00074-X
- Cooper, B. J. (1985). The Cainozoic St Vincent Basin - Tectonics, structure, stratigraphy. Special Publication, South Australian Department of Mines and Energy, 5, 35–49.
- Dix, K. L. (2013). South Australian historical earthquakes in the pre-instrumental period 1837–1963: A comprehensive chronicle and analysis of available intensity data [ MPhil thesis]. School of Physics, University of Adelaide. https://digital.library.adelaide.edu.au/dspace/handle/2440/84130
- Gladkov, A. S., Lobova, E. U., Deev, E. V., Korzhenkov, A. M., Mazeika, J. V., Abdieva, S. V., Rogozhin, E. A., Rodkin, M. V., Fortuna, A. B., Charimov, T. A., & Yudakhin, A. S. (2016). Earthquake-induced soft-sediment deformation structures in Late Pleistocene lacustrine deposits of Issyk-Kul Lake (Kyrgyzstan). Sedimentary Geology, 344, 112–122. https://doi.org/10.1016/j.sedgeo.2016.06.019
- Guessoum, N., Benhamouche, A. A., Bouhadad, Y., Bourenane, H., & Abbouda, M. (2000). Field evidence of Quaternary seismites in the Mostaganem-Relizane (western Algeria) region: Seismotectonic implication. Arabian Journal of Geosciences, 11(641), 13. https://doi.org/10.1007/s12517-018-4009-1
- Head, M. J., Pillans, B., & Farquhar, S. A. (2008). The early-middle Pleistocene transition: Characterization and proposed guide for the defining boundary. Episodes, 31(2), 255–259. https://doi.org/10.18814/epiiugs/2008/v31i2/014
- Hilbert-Wolf, H. L., Roberts, E. M., & Simpson, E. L. (2016). New sedimentary structures in seismites from SW Tanzania: Evaluating gas- vs. water-escape mechanisms of soft-sediment deformation. Sedimentary Geology, 344, 253–262. https://doi.org/10.1016/j.sedgeo.2016.03.011
- Hoffmann, G., & Reicherter, K. (2012). Soft-sediment deformation of Late Pleistocene sediments along the southwestern coast of the Baltic Sea (NE Germany). International Journal of Earth Sciences, 101(1), 351–363. https://doi.org/10.1007/s00531-010-0633-z
- Karte, J. (1983). Periglacial phenomena and their significance as climatic and edaphic indicators. GeoJournal, 7(4), 329–340. https://doi.org/10.1007/BF00241455
- May, R. I. (1992). Origin, mineralogy and diagenesis of sediments from the Noarlunga and Willunga Embayments, South Australia [ PhD thesis]. University of Adelaide. https://digital.library.adelaide.edu.au/dspace/handle/2440/19746
- May, R. I., & Bourman, R. P. (1984). Coastal landslumping in Pleistocene sediments at Sellicks Beach, South Australia. Transactions of the Royal Society of South Australia, 108(2), 85–94. https://www.biodiversitylibrary.org/pdf4/1688090i00128035.pdf
- May, R. I., & Milnes, A. R. (2024). Characteristics of sediments and regolith alterations in the Pleistocene succession, coastal cliff sections, St Vincent Basin, South Australia. Sedimentologika, 2(1), 1–27. https://doi.org/10.57035/journals/sdk.2024.e21.1260
- McCue, K. (2013). Historical earthquakes in South Australia. Australian Earthquake Engineering Society. https://aees.org.au/wp-content/uploads/2013/11/McCue_SA_EQs.pdf
- McGowran, B., & Alley, N. F. (2008). History of the Cenozoic St Vincent Basin in South Australia. In S. A. Shepherd, S. Bryars, I. R. Kirkegaard, P. Harbison, & J. T. Jennings (Eds.), Natural history of Gulf of St Vincent (pp. 13–28). Royal Society of South Australia Inc. https://hdl.handle.net/2440/60541
- McGowran, B., Lemon, N., Preiss, W., & Olliver, J. (2016). Cenozoic Willunga Embayment: From Australo-Antarctic Gulf to Sprigg Orogeny. In Geological field excursion guide, Australian earth sciences convention (pp. 48). Department of State Development.
- Menegoni, N., Maino, M., Toscani, G., Mordeglia, L. I., Valle, G., & Perotti, C. (2023). Holocene deformations at the Po Plain–Southern Alps transition (Lake Maggiore, Italy): Inferences on glacially vs. tectonic-induced origin. Geosciences, 13(286), 24. https://doi.org/10.3390/geosciences13090286
- Milnes, A. R., & Hutton, J. T. (1983). Calcretes in Australia – A review. In K. A. Handreck (Ed.),Soils: An Australian Viewpoint, chapter (Vol. 10, pp. 119–162). CSIRO, Melbourne/Academic Press.
- Mohindra, R., & Thakur, V. C. (1998). Historic large earthquake-Induced soft sediment deformation features in the Sub-Himalayan Doon Valley. Geological Magazine, 135(2), 269–281. https://doi.org/10.1017/S0016756898008413
- Montenat, C., Barrier, P., D′estevou, P. O., & Hibsch, C. (2007). Seismites: An attempt at critical analysis and classification. Sedimentary Geology, 186(1–4), 5–30. https://doi.org/10.1016/j.sedgeo.2006.08.004
- Moretti, M. (2000). Soft-sediment deformation structures interpreted as seismites in middle-Late Pleistocene aeolian deposits (Apulian foreland, southern Italy). Sedimentary Geology, 135(1–4), 167–179. https://doi.org/10.1016/S0037-0738(00)00070-1
- Moretti, M., & Sabato, L. (2007). Recognition of trigger mechanisms for soft-sediment deformation in the Pleistocene lacustrine deposits of the SantʻArcangelo Basin (Southern Italy): Seismic shock vs. overloading. Sedimentary Geology, 196(1–4), 31–45. https://doi.org/10.1016/j.sedgeo.2006.05.012
- Moretti, M., & van Loon, A. J. (2014). Restrictions to the application of ‘diagnostic’ criteria for recognizing ancient seismites. Journal of Palaeogeography, 3(2), 162–173. https://doi.org/10.3724/SP.J.1261.2014.00050
- Phillips, S. E. (1988). The interaction of geological, geomorphic and pedogenic processes in the genesis of calcrete [ PhD thesis]. University of Adelaide. https://digital.library.adelaide.edu.au/dspace/handle/2440/19052
- Phillips, S. E., & Milnes, A. R. (1988). The Pleistocene terrestrial carbonate mantle on the southeastern margin of the St Vincent Basin, South Australia. Australian Journal of Earth Sciences, 35(4), 463–481. https://doi.org/10.1080/08120098808729463
- Pillans, B., & Bourman, R. (1996). The Brunhes/Matuyama polarity transition (0.78 ma) as a chronostratigraphic marker in Australian regolith studies. AGSO Journal of Australian Geology & Geophysics, 16, 289–294.
- Pisarska-Jamrozy, M., Beloit, S., Börner, A., Hoffmann, G., Hüneke, H., Kanzler, M., Obst, K., Rother, H., & van Loon, A. J. (2018). Evidence from seismites for glacio-isostatically induced crustal faulting in front of an advancing land-ice mass (Rügen Island, SW Baltic Sea). Tectonophysics, 745, 338–348. https://doi.org/10.1016/j.tecto.2018.08.004
- Preiss, W. V. (2019). The tectonic history of Adelaide’s scarp-forming faults. Australian Journal of Earth Sciences, 66(3), 305–365. https://doi.org/10.1080/08120099.2018.1546228
- Quigley, M. C., Bastin, S., & Bradley, B. A. (2013). Recurrent liquefaction in Christchurch, New Zealand, during the Canterbury earthquake sequence. Geology, 41(4), 419–422. https://doi.org/10.1130/G33944.1
- Rana, N., Bhattacharya, F., Basavaiah, N., Pant, R. K., & Juyal, N. (2013). Soft sediment deformation structures and their implications for late Quaternary seismicity on the South Tibetan detachment system, central Himalaya (Uttarakhand), India. Tectonophysics, 592, 165–174. https://doi.org/10.1016/j.tecto.2013.02.020
- Sandiford, M. (2003). Neotectonics of southeastern Australia: Linking the Quaternary faulting record with seismicity and in situ stress. In R. R. Hillis & R. D. Müller (Eds.), Evolution and dynamics of the Australian plate (Vol. 22, pp. 107–119). Geological Society of Australia, Special Publication. https://doi.org/10.1130/0-8137-2372-8.107
- Scott, B., & Price, S. (1988). Earthquake-induced structures in young sediments. Tectonophysics, 147(1–2), 165–170. https://doi.org/10.1016/0040-1951(88)90154-0
- Seilacher, A. (1969). Fault-graded beds interpreted as seismites. Sedimentology, 13(1–2), 155–159. https://doi.org/10.1111/j.1365-3091.1969.tb01125.x
- Smith, M. L., Fontaine, K., & Lewis, S. J. (2016). Regional hydrogeological characterisation of the St Vincent Basin, South Australia. Technical report for the national collaboration framework. Regional Hydrogeology Project. Geoscience Australia Record 2015/16. https://ecat.ga.gov.au/geonetwork/srv/eng/catalog.search#/metadata/78884
- Stuart, W. J. (1969). Stratigraphic and structural development of the St Vincent Tertiary Basin, South Australia [ PhD Thesis]. University of Adelaide. https://digital.library.adelaide.edu.au/dspace/handle/2440/21275
- Stuart, W. J. (1970). The Cainozoic stratigraphy of the eastern coastal area of Yorke Peninsula, South Australia. Transactions of the Royal Society of South Australia, 94, 151–178. https://www.biodiversitylibrary.org/pdf4/1689630i00127752.pdf
- Thorson, R. M., Clayton, W. S., & Seeber, L. (1986). Geologic evidence for a large prehistoric earthquake in eastern Connecticut. Geology, 14, 463–467. https://doi.org/10.1130/0091-7613(1986)14<463:GEFALP>2.0.CO;2
- Twidale, C. R. (1988). Chapter 2: Geomorphological evolution. In C. R. Twidale, M. J. Tyler, & B. R. Webb (Eds.), Natural history of the Adelaide region. Royal Society of South Australia (Inc.). https://www.rssa.org.au/wp-content/uploads/2022/07/natural_history_of_the_adelaide_region.pdf
- Ward, W. T. (1965). Eustatic and climatic history of the Adelaide area South Australia. The Journal of Geology, 73(4), 592–602. https://doi.org/10.1086/627095
- Ward, W. T. (1966). Geology, geomorphology and soils of the South-Western part of County Adelaide, south Australia. Soil Publication, CSlRO, Australia, 23, 115.
- Worsley, P. (2014). Ice-wedge growth and casting in a Late Pleistocene, periglacial, fluvial succession at Baston, Lincolnshire. Mercian Geologist, 18(3), 159–170.