Abstract
Birdsong often plays a crucial role in sexual behavior, including territorial defense and mate attraction. This behavior is particularly well studied in species in which only males sing and respond to gonadal testosterone depending on the breeding season. Although hummingbirds are long known to sing in a breeding context, the sensitivity of these vocalizations to testosterone in both sexes remains unknown. Therefore, we investigated (i) which vocalizations are emitted during their breeding season, (ii) the testosterone levels in free-ranging birds, and (iii) the effect of testosterone on these vocalizations in a semi-natural environment in both sexes of the black jacobin (Florisuga fusca), an early-branched hummingbird. Females and males of black jacobin produced the same set of vocalizations (high-pitched song and chirp call) at a similar rate during the breeding season, although testosterone levels in males were significantly higher. Testosterone treatment did not affect the vocal activity or acoustic parameters of their song in both sexes. However, testosterone maintained high levels of aggression in treated birds of both sexes and hyper-developed the oviduct of females. In contrast to almost all well-studied bird species studied so far, the vocal behavior of the black jacobins in their breeding season seems to be insensitive to high testosterone levels.
Introduction
Sexual male-typical traits, such as song in Northern Hemisphere songbirds are known to be activated during the reproductive periods with high levels of testosterone (reviewed in Wingfield & Farner Citation1978; Gahr Citation2014). These traits are important for courtship (mate attraction) and agonistic encounters (territorial defense) (Catchpole & Slater Citation1995). Evidence of testosterone-dependent vocal performance is nevertheless not exclusive to songbirds (oscine passerines) since it has also been found in other taxa, as in suboscine passerines (Kroodsma Citation1984) and parrots (Brockway Citation1968; Nespor et al. Citation1996). Crowing of roosters in domestic chicken (Gallus gallus) as well as of other galliform species, such as the Japanese quail (Coturnix japonica) and the grey partridge (Perdix perdix), is another typical vocal trait known to be testosterone dependent (Hamilton Citation1938; Marler et al. Citation1962; Andrew Citation1963; Beani et al. Citation2000). A particular vocalization type, the nest-coo used in the courtship of male ring doves (Streptopelia risoria) was induced by brain implantation of testosterone (Cohen & Cheng Citation1982).
In all well-studied bird species, changes in vocalization were found in association with naturally elevated testosterone plasma levels of the breeding season or after testosterone implantation (Gahr Citation2020), for example, increase of vocal output (song or/and call rate), acoustic features such as frequency range or stereotypy (Arnold Citation1975; Heid et al. Citation1985; Fusani et al. Citation1994), and utterance of testosterone-specific vocalizations such as trills (Pröve Citation1974; Fusani et al. Citation1994; Voigt & Leitner Citation2013). Further, a masculinized song can be induced in females (that do not sing otherwise) by testosterone implants, not only in songbirds such as canaries, chaffinches, white-crowned sparrows and white-browed sparrow weavers (Kern & King Citation1972; Nottebohm Citation1980; Voigt & Leitner Citation2013; Chiver & Schlinger Citation2019; Vellema et al. Citation2019) but also in non-songbirds such as golden-collared manakins (Manacus vitellinus) (Chiver & Schlinger Citation2019), budgerigars (Melopsittacus undulatus) (Lahaye et al. Citation2012) and Japanese quail (Balthazart et al. Citation1983).
High levels of testosterone appear to be necessary to activate sexually relevant vocal behaviors in Northern Hemisphere songbirds during a short breeding season (Wingfield & Farner Citation1978; Gahr Citation2020), whereas, in tropical songbirds such as the silver-beaked tanager (Ramphocelus carbo), during a long breeding season (between 6 and 7 months), the testosterone levels are only high at the end of the season coinciding with higher rates of dawn-song, but this species show a higher brain sensitivity to testosterone in the beginning of the season which indicates testosterone sensitivity of their dawn-song (Quispe et al. Citation2016). However, testosterone sensitivity of these vocal behaviors are less clear in tropical and sub-tropical non-songbird species with protracted breeding periods or year-round territoriality (Goymann et al. Citation2004). Such a bird family are the hummingbirds in which males and in some species females are known to sing (Baptista & Schuchmann Citation1990; Ficken et al. Citation2000; da Silva & Vielliard Citation2006; Ferreira et al. Citation2006).
Hummingbirds have neural song control areas analog to songbirds which are active during song production (Jarvis et al. Citation2000) and express androgen receptors (binding site of testosterone and its converted product 5α-dihydrotestosterone, DHT) (Gahr Citation2000). Androgen receptors were also found in hummingbird syrinx (A. Monte unpublished data), the sound-producing organ of birds. Nevertheless, it is unknown whether song is a male-typical trait or whether any vocalization is testosterone sensitive in hummingbirds, hence our motivation to study these aspects in the black jacobin (Florisuga fusca). It is a species of the Topazes clade (tribe Topazini) which is a sister taxon of all other extant hummingbirds according to the most recent evolutionary tree (McGuire et al. Citation2009, Citation2014). Therefore, the study of the black jacobin may give insights into the ancestral condition of testosterone-sensitive vocalizations in the hummingbird family.
The black jacobin occurs in the Atlantic forest on the extent of the Brazilian coast until Uruguay and Argentina (Sick Citation1997; Schuchmann & Bonnan Citation2019). Black jacobins produce a high-pitched song composed of very fast frequency oscillations (Olson et al. Citation2018; Monte et al. Citation2020) and uttered in the breeding season (from September until March; Ruschi Citation1964); however, it is unknown whether this high-pitched or other vocalizations produced in the breeding season are the exclusivities of males and whether they are testosterone sensitive. To investigate the sensitivity of their vocalizations to testosterone, firstly, we measured testosterone plasma levels and body weight of free-living black jacobins from both sexes in the middle of the breeding season (December). Secondly, we carried out an experiment with wild-caught birds placed in semi-natural aviaries. We recorded the vocalizations and associated behaviors before and after testosterone implants. We established their vocal repertoire and quantified their vocal output (song and call rate), as well as the acoustic parameters of the song. Additionally, we investigated the number of attacks as a proxy for aggressiveness and their feeding behavior. We found higher circulating testosterone levels and body weight in males than females, but no sex differences in their vocalizations. The testosterone treatment induced the hypertrophy of the oviduct but did not affect the testes weight. Both sexes maintained their aggressive behavior before and after testosterone implantation whereas the control group implanted with placebos reduced aggressiveness during the study period. Unexpectedly, we could not show any changes in their vocal behaviors following testosterone implantation. These results indicate that in contrast to songbirds and most other birds studied to date, the vocalizations of hummingbirds in their breeding season seem insensitive to testosterone.
Methods
Animals
This study was carried out in the park of the Museum of Biology Prof. Mello Leitão in Santa Teresa, Espírito Santo, Brazil in the middle of the breeding season that lasts from September until March (Ruschi Citation1964). Free-living black jacobins (Florisuga fusca) were captured between 6:00–8:00 and 17:00–18:00 during their regular visit to feeder bottles filled with sugary water (solution of water with 20% sugar), hanging inside a trap called “Ruschi net trap” developed and optimized to capture hummingbirds (Ruschi Citation2009).
Black jacobin’s plumage is not sexually dimorphic (Ruschi Citation1973), the birds captured for the testosterone experiment (n = 32) were individually marked with plastic-colored tags attached to their back (Kapoor Citation2012) and kept randomly in outdoor aviaries until molecular sexing. Upon sexing results, we kept 14 males and 10 females that were distributed in four adjacent outdoor aviaries (2.35 m long × 2.70 m high × 3.20 m deep; A1 to A4) under natural conditions of daylight, rain, temperature, and humidity. We placed six birds per aviary. Three males and three females in aviaries A1 and A4 and four males and two females in aviaries A2 and A3. The unequal number of females and males was due to two mistakes in the molecular sexing results that were only noticed at the end of the experiment when we checked the gonads. Additionally, we caught and released 206 wild birds for blood sampling (50 females and 153 males, 3 died) and captured and sacrificed further 14 birds (seven females and seven males) for future tissue analyses.
The aviaries contained vegetation to offer shadow, branches of different thicknesses to be used as perches, two hanging pots with flowers and thin perches, three feeder bottles with watery sugar, a wooden box containing fermented fruits to attract fruit flies (part of the diet of the black jacobins), and an irrigation sprinkler activated only between observations when the temperature was above 35 ºC to spread water in half of the aviary to be used for bathing. The feeder bottles were refilled once a day before the morning observations. At the end of the aviary experiment, the birds were killed by decapitation, as for the 14 extra caught birds, organs were immediately frozen in dry ice and stored at − 80 ºC until use.
All procedures of this study were conducted in conformity with the instruction no. 03/2014 of the Chico Mendes Institute for Biodiversity Conservation (ICMBio) and with the permission of the Brazilian Institute of Environment and Renewable Natural (IBAMA) under the Biodiversity Information and Authorization System (SISBio) licenses numbers 30319–1, 41794–2 and 49097–1. The bird handling and housing procedures were conducted in accordance with Law no. 11.794/2008, as well as with the rules issued by the National Council for Control of Animal Experimentation (CONCEA) and was approved by the Ethics Committee on Animal Use of the Federal University of Para (CEUA/UFPA) under the protocol number 5937130718 (ID 000953).
Experimental procedure and testosterone implants
The first 6 days of captivity were used as a time for the housed birds (n = 24) to acclimate to the aviaries (no recorded observations), and then we started monitoring observations for 1 week (days − 6 to − 1) before the testosterone (T) or empty implant treatment for baseline activity (“before treatment”). On the 7th day (day 0), a total of seven females (aviaries A1, A3 and A4 with two each; A2 with one) and five males (aviaries A1, A3 and A4 with one each; A2 with two) were implanted with testosterone (“T-treated group”) and the others with placebo empty implants (“control group”) and monitored for the next 2 weeks (days 1 to 12, “after treatment”, Fig. S1 in Supplemental Data). Each bird was observed for 20 min twice a day, in the morning (between 5:30, after sunrise, and 12:00) and the afternoon (between 12:00 and 18:30, before sunset; except days 8 and 12, in which birds were monitored only in the morning due to logistic problems). All the birds within an aviary were observed consecutively in a randomized order. Each of the two observation sessions per bird was conducted by a different observer (A. Monte or R. da Silva Matos) to minimize the observer bias. In total, we sampled 96 hr of observations before and 176 hr after T-treatment.
The implants were self-made with a 3 mm silastic tube (Dow Corning, USA; 1.47 mm inner diameter, and 1.96 mm outer diameter) filled with ca 0.9 mg of testosterone (Sigma T1500, Sigma-Aldrich GmbH, Germany) (T implant) or left empty (placebo implant) and the endings sealed with liquid silicone sealant (ASC 40064, USA). The implant size was estimated in proportion to the body size as it was reported in other species studied (Quispe et al. Citation2015; Prior et al. Citation2016). The implants were subcutaneously inserted at the lower back of the birds after local anesthesia with lidocaine hydrochloride (Lidogel 2%, Neoquimica).
Sound recording and analyses of vocalizations
In each session we monitored the vocal behavior, feeding behavior and aggressiveness in the four aviaries following a focal-bird sampling technique (Altmann Citation1974; Martin & Bateson Citation2007).
For sound recording, we used a digital recorder Marantz PMD 671 (USA) mounted with a shotgun directional microphone (Sennheiser MKH 70 P48, Germany; Rode NTG2, Australia). The recordings were obtained in the mono channel, with frequency sample of 48 kHz and 32 bits. The audio recordings were screened manually in the software Audacity (version 2.0.3, Audacity Team Citation2013), and the descriptions were transcribed into audio labels that were exported as text files. The labels were quantified by a customized algorithm written on R version 3.0.3 (R Core Team Citation2014).
We identified three vocal types based on spectral parameters as previously described for the species (Olson et al. Citation2018; Monte et al. Citation2020): high-pitched (most common, 68.1% of emission in the entire monitoring), low-pitched (0.6%) and chirps (31.3%) (Fig. S2 in Supplemental Data). The high-pitched vocalization has harmonics up to 80 kHz with a fundamental frequency between 10 and 14 kHz. The fundamental frequency was fast-modulated and had periodic oscillation called vibrato (Monte et al. Citation2020). It is the most spectrally complex of black jacobin’s vocalizations and is suggested as the song of the species (Olson et al. Citation2018). The song is composed of one or several units (or syllable) emitted in sequence with each unit composed of three (triplet), two (double) or one (single) subunit (or notes) (Fig. S3 in Supplemental Data). Chirps can be emitted alone or followed by a few repetitions with variable silent interval (single chirp or s-chirp) or followed by many repetitions with progressively longer silence intervals (multiple chirps or m-chirp) (Fig. S2 in Supplemental Data) as previously described for rufous-breasted hermit (Glaucis hirsutus) (Ferreira et al. Citation2006). Given that m-chirp was rare, we quantified the number of chirps in the m- and s-chirps and presented them together in the results. The low pitch was also a very rare event and was not analyzed.
We quantified the number of songs and chirps emitted per bird in each 20 min observation session (songs or chirps/20 min). We subsampled the longest song bouts with a good signal-to-noise ratio and selected ~ 200 songs per bird (range: 5–1000 song bouts per bird, per session). We labeled the triplets, doubles and singles manually in the software Audacity (version 2.0.3, Audacity Team Citation2013) and saved the labels as text files. We quantified their repeatability (R) which represents how much of the total variance in the number of triplets, doubles and singles is attributed among birds and was calculated with the function “rpt” implemented by the package “rptR” (Stoffel et al. Citation2017) and using a customized algorithm written in R version 3.0.3 (R Core Team Citation2014). For the analysis of the spectral and temporal acoustic parameters (in total 26, Table S1 in Supplemental Data), we used the function “specam” and package “warbleR” (Araya-Salas & Smith-Vidaurre Citation2017). We analyzed in total 3660 songs. We calculated the averages of each parameter per bird before, 1 and 2 weeks after T-treatment followed by a Principal Component Analysis (PCA) to reduce the number of parameters and maintain those with higher variance (see Statistical analyses).
Quantification of other associate behavioral observations
We quantified the number of attacks per focal bird per session (a proxy for aggressiveness), that is when the focal bird chased another bird away or pecked the bird with the bill (attacks/20 min). We also quantified the feeding events per session as the number of times the focal bird drank sugary water from the feeder or caught a fruit fly (a proxy for metabolism). These feeding counts were also a proxy for the well-being of the birds as lethargy and anorexia are signs of sickness (Hart Citation1988). Those two behaviors have been previously documented to be influenced by testosterone in other birds, especially in males (Wingfield et al. Citation2001; Soma Citation2006).
Blood sampling
For sex determination, a droplet of blood was collected from each bird with a heparinized capillary tube after the section of the hallux’s nail as described by Ruschi (Citation1950) diluted in Queens Lysis Buffer (0.01 M EDTA; 0.01 M Tris; 0.01 M NaCl; 1% n-Lauroylsarcosine and pH 8.0) and stored in the fridge (~ 5 ºC). For the measurement of the testosterone plasma levels, blood (mean µL, 30–80 µL) was collected from the jugular vein as described by Hoysak and Weatherhead (Citation1991) otherwise from the trunk after decapitation. A droplet of blood was diluted in Queens Buffer for later sexing and for the rest of the blood, the plasma was separated by centrifugation (10 min at 6000 rpm) and stored in dry ice (~ − 80 ºC).
Sexing protocol
The blood diluted in Queens Lysis Buffer was used for genomic DNA extraction with the NucleoSpin Blood QuickPure DNA Extraction Kit (Macherey-Nagel 740569.250, Germany). We specifically designed primers pair for the black jacobins: floFus3-gold-fw (5’-TGCTCATGGCTAGAGGGACT-3’) and floFus3-gold-rv (5’-TAATCCCCCTCTCCTCCCTA-3’) that allowed successful sex determination with 92% of efficiency (calculated as the percentage of correct sexing result for those birds which gonads could be inspected, n = 24). The primers were designed based on the sequence information of a female Florisuga fusca genome assembly available at the Department for Behavioral Neurobiology of the Max Planck Institute for Biological Intelligence (former Max Planck Institute for Ornithology) in Seewiesen, Germany (manuscript in preparation). For the PCR reaction, we used 2 uL primer mix (10 pmol/µL each primer), 2 µL 10× Buffer B1 (Hot FirePol, Solis BioDyne, 01-02-00500, Estonia), 1.6 µL MgCl2 25 mM (Solis BioDyne, 01-02-00500, Estonia), 0.4 µL dNTPs 10 mM each nucleotide (Solis BioDyne, 02-31-00020, Estonia), 0.5 µL Taq Polymerase 5 u/µL (Hot FirePol, Solis BioDyne, 01-02-00500, Estonia) and 1 µL DNA template. All were diluted in 12.5 µL nuclease-free distilled water for a total volume of 20 µL. The PCR protocol for all samples was run in a T 3000 Thermocycler 48 (050–723, Biometra, Germany) and consisted of a first 15 min at 95 °C, followed by 35 cycles of 94 °C for 30 sec, 55 °C for 45 sec and 72 °C for 50 sec with the last extension step at 72 °C for 2 min. The PCR products were separated by electrophoresis at 100 V between 1.5 and 2 hr in a 2% agarose gel stained with ethidium bromide.
Testosterone level measurements
In total, we measured the plasma testosterone levels of 241 black jacobins. The samples for this profile were obtained within 10 consecutive days in December, during the middle of the breeding season (Ruschi Citation1964) for 57 females (52 adults and five juveniles) and 160 males (145 adults and 15 juveniles). First-year juveniles could be identified by differential plumage (Ruschi Citation1973). Since there was no significant difference between 1st-year juveniles and adults (Table S2 in Supplemental Data), they were pooled together.
The testosterone plasma levels were measured by radioimmunoassay as described by Goymann et al. (Citation2006). In total, three assays were realized. The samples from the testosterone-treated birds were assayed 3 times, and the final testosterone value was obtained by the simple mean. These triplicates were conducted in different batches with consistent outcomes; thus, it is unlikely that the values obtained for testosterone-treated birds are an artifact. The lower detection limits were between 0.33 and 0.4 pg/mL with all samples above the detection limit. The extraction recovery of testosterone per assay was respectively 89, 90 and 87%, and the intra-assay coefficients of variation 7, 5 and 12.2%. The inter-assay coefficient of variation was 3.2%.
Statistical analyses
The statistical analyses were performed using R version 3.0.3 (R Core Team Citation2014). All data and code are available in the online repository and accessible in this link (https://osf.io/9fs5w/).
The testosterone plasma levels (T-levels) were log10 transformed. For wild black jacobins (57 females and 160 males), to explore the effect of sex, body weight, age and day on T-levels, and effect of sex, T-levels, age and day on body weight, we used linear models. First, we fitted models including age (1st-year juveniles × adults) which had no effect both on T-levels (estimate = 0.22 ± 0.20, t = 1.13, P = 0.262, Table S2 in Supplemental Data) and body weight (estimate = 0.18 ± 0.11, t = 1.60, P = 0.112, Table S2 in Supplemental Data). Then we fitted models without age, and we plotted 1st-year juveniles and adults together. For captive black jacobins (10 females and 14 males), to compare T-levels among sex and experimental groups (control and T-treated), we used Wilcoxon rank sum exact test (non-parametric). To compare control to wild birds, we randomly subsampled 10 T-levels values of each sex from the wild birds.
To compare gonadal and brain weight, we used Kruskal–Wallis rank sum test (non-parametric) to compare wild, control and T-treated. We used non-parametric statistics in all cases in which at least one of the groups (T-treated females) had only three data points. In these cases, we reported the median interquartile range (IQR) [25%, 75% quartiles]. Following a significant Kruskal–Wallis test, we performed Dunn’s Kruskal–Wallis multiple comparisons as post hoc analysis with the function “dunnTest” implemented by the package “FSA” (Ogle et al. Citation2020). To compare body weight, we weighed the aviaries birds every time they were handled: when they were housed (before T), on the day of the implantation (T) and at the end of the experiment (after T). Then, we used repeated measures one-way ANOVA (parametric because all the assumptions were met). In the results, we reported the mean (± SD). Following a significant ANOVA, we performed Tukey multiple comparisons of means as post hoc analysis. In both post hoc analyses, we adjusted the P-values with the Bonferroni method. Given that the body weight of males had a wide variance, we further performed the F test to compare two variances (control and T-treated) with the “var.test” function. The significance level for all tests was α = 0.01.
To test whether the testosterone treatment (T-treatment) affected the number of songs and chirps emitted by the black jacobins, their feeding behavior and aggressiveness in the aviaries, we used generalized linear mixed models (GLMMs). In all models, to account for the non-independence of the samples, we included “bird id” as a random effect. To determine the best fit model for each response variable (song, chirp, feeds or attack counts), we fitted models with all possible combinations of the predictors that were biologically meaningful for the respective response variable. Then, we compared the models using the differential Akaike’s information criterion (dAIC) obtained by the function “AICtab” implemented by the “bbmle” package (Bolker & R Development Core Team Citation2020) and selected the most parsimonious models based on the lowest AIC (Burnham & Anderson Citation2016). We accessed the statistical significance of the best-fitting models’ predictors via asymptotic Wald’s z‐tests (P < 0.01). In the results, we reported the estimated mean 95% CI [lower, upper confidence interval] for song and chirp counts models and the means of the simulated values from the posterior distributions and the predicted 95% credible intervals (CrI) [lower, upper credible interval] for feeding and attack counts models. Details about the fitting of the GLMMs the assessment of their assumptions and post-hoc analysis are provided in the Supplemental Data.
We performed a Principal Component Analysis to reduce song parameters using the function “prcomp” implemented by the package “car” (Fox & Weisberg Citation2018). We plotted the first two Principal Components (PC1 and PC2) using a customized version of the function “ggbiplot” implemented by the package “ggbiplot” (Vu Citation2011). We inspected visually the PC1 and PC2 plot to identify whether the birds were grouped by treatment and/or sex at the different time points (before, 1 and 2 weeks after T-treatment). We further investigated one of the variables that were heavily loaded within the PC1 vector (mean dominant frequency of the song) and one within the PC2 vector (song duration). We compared the averages of the dominant frequency and song duration for each of these four groups: control females, T-treated females, control males and T-treated males at the time points: before, 1 and 2 weeks after T-treatment using the Friedman Rank Sum Test which is an ideal statistic to use for a repeated measures type of experiment to determine if a particular factor has an effect (Scheff Citation2016). We also calculated the repeatability (R) using the function “rpt” implemented by the package “rptR” (Stoffel et al. Citation2017).
Results
Testosterone plasma levels and body weight differ between sexes in free-living birds during the breeding season as well as in captivity
Adult males had higher testosterone levels [estimated mean 95% CrI (lower, upper credible interval); n = 160 birds, 102.19 pg/mL (90.24, 117.18)] than adult females [n = 57, 54.39 pg/mL (44.12; 6838)] (estimate = 0.52 ± 0.14, t213 = 3.82, P < 0.001; , left panel) and had a higher body weight [7.85 g (7.74, 7.96)] (estimate = 0.26 ± 0.08, t213 = 3.33, P = 0.001; , right panel) than adult females [7.47 g (7.33, 7.60)] (). The model for T-levels indicated a strong effect of body weight (estimate = 0.34 ± 0.12, t213 = 2.90, P = 0.004; ). Thus, to verify whether the sex difference found in T-levels is not a side effect of the differences in body weight, we selected males (n = 115), which fall into the weight range of the females (female weight mean ± SD) and compared T-values again. The difference remained and females still had lower T-levels than males (t = − 3.40, df = 142.27, P = 0.001). The model for T-levels also indicated a strong effect of sampling time (estimate = − 0.29 ± 0.02, t213 = − 13.79, P < 0.001; ). This temporal correlation may reflect a decline in the baseline testosterone in the second half of their breeding season. Furthermore, nearly 7% of the males (n = 11) showed T-levels above 1000 pg/mL and 30% of the females (n = 17) showed T-levels above 100 pg/mL which is expected for birds in breeding condition in the tropics (e.g. Goymann et al. Citation2004; Goymann & Wingfield Citation2014).
Fig. 1. Circulating levels of plasma testosterone (T plasma levels) and body weight of wild and captive black jacobins. All T plasma levels are represented on a logarithmic scale (Log10). (A) Wild black jacobin is sexually dimorphic in the T plasma levels (left panel) and body weight (right panel). The outlines of the violin plot represent the proportion of the data located in each value given by kernel probability density. The data points represent individual measurements of females (n = 57, lighter points) and males (n = 160, darker points). The error bars in gray represent the fitted mean and the 95% credible interval given by the linear models. (B) Captive black jacobins under T implantation had T plasma levels increased 2000-fold for females and 300-fold for males in relation to those of the same sex that received placebo implants (controls). The error bars on the left indicate the median and interquartile range (25 to 75%) of the wild black jacobins as a reference to the physiological range. The box plots indicate the median and interquartile range (25 to 75%) of the captive black jacobins. The data points represent individual measurements of females (control, n = 3 and T-treated, n = 7; lighter points) and of males (control, n = 9 and T-treated, n = 5; darker points). (C) Females lost weight in captivity independently of T-treatment. The body weight of the captive black jacobins was obtained at three time points: on the day there were placed in the aviaries (before T), on the day of the T implantation (T), and at the end of the experiment (after T). Although T-treated black jacobins presented T levels above the physiological range, neither females nor males had their body weight affected by the treatment, instead, females were affected by the captive condition.
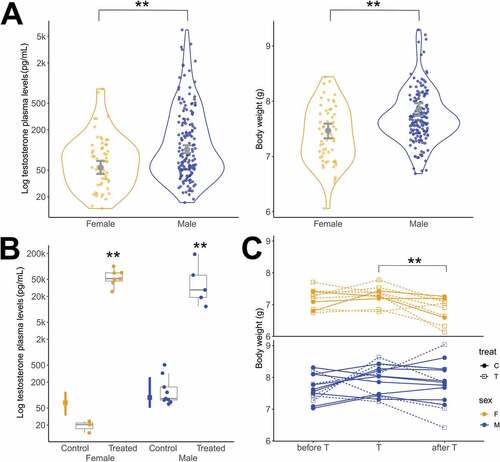
Table 1. Results of linear models explaining variation in the circulating testosterone plasma levels (T plasma levels) and body weight of wild black jacobins. In the wild, T plasma levels were affected by sex, body weight, and time, whereas body weight was affected by sex and T plasma levels. In bold are the significant predictors (P < 0.01).
T-levels of captive (aviaries) control birds were inside the range of the free-living birds in both females (W = 26, P = 0.077) and males (W = 44, P = 0.968); therefore, captivity did not affect T-levels. T-levels of T-treated birds were higher than controls in both females (W = 0, P < 0.017) and males (W = 0, P = 0.001): 2.5k-fold above wild average for females and 300-fold for males (, ).
Table 2. Median and quartiles of the testosterone plasma levels for wild and captive black jacobins grouped by sex and treatment: birds treated with placebo (control) and birds treated with testosterone implants (T-treated).
Captivity, but not testosterone treatment decreased body weight only in females
Captivity decreased the weight of females, but not that of males. The body weight of females changed with time [F (2,18) = 6.47, P = 0.008], but not between treatment groups (W = 16, P = 0.267). Before T application, females’ weight did not differ between the control (mean ± SD, 7.1 g ± 0.3) and T-treated (7.2 g ± 0.3) groups. At the T-treatment day, weight of all females increased slightly, but not significantly compared to before (P = 0.723) (control, 7.3 g ± 0.1; T-treated, 7.3 g ± 0.4). Two weeks after the testosterone treatment (after T) weight decreased significantly compared to baseline (P = 0.041) and to T day (P = 0.008) (control, 7 g ± 0.4; T-treated 6.8 g ± 0.4) (, upper panel). This effect was not observed in males, as their weight did not change in time [F (2,26) = 1.36, P = 0.276] and not between groups [F (8,4) = 0.21, P = 0.058]: Before T: control group 7.7 ± 0.4 g and T-treated group 7.6 ± 0.4 g. On the T-treatment day: control group 7.9 ± 0.4 g and T-treated group 8 g ± 0.6; after T: control group 7.9 ± 0.5 g and T-treated group 7.7 ± 1 g (, lower panel).
Testosterone treatment increased oviduct weight but did not affect testes weight
The oviduct weight differed between groups (H = 6.56, df = 2, P = 0.038): wild (n = 6), captivity control (n = 3), and captivity T-treated (n = 7) (see for medians). Ovary weight was lower in control captive birds than in the wild (P = 0.021) and T-treated females (P = 0.017), but this was not significant after Bonferroni correction (P adj. = 0.063 and 0.051, respectively) (, left panel). However, the oviduct weight differed between groups (H = 11.21, df = 2, P = 0.004) with heavier oviducts in T-treated (by five and six-fold, respectively) compared to the wild (P adj. = 0.011) and to control (P adj. = 0.025) (, right panel; ). The testis weight was similar between groups [F (2,18) = 2.14, P = 0.147] (, ).
Table 3. Median and quartiles of testes, ovaries, and oviduct weights for wild and captive black jacobins grouped by treatment: birds treated with placebo (control) and birds treated with testosterone implants (T-treated).
Fig. 2. Although both sexes of black jacobin have androgen receptors in their gonads, the testosterone treatment (T-treatment) affected only the gonads of the females. (A) The ovary weight (left panel) of wild and T-treated females does not differ, although the ovary of control females is slighter lighter. The oviduct weight (right panel) of T-treated females is significantly heavier than wild and control females. The data points represent individual measurements of females (wild, n = 6; control, n = 3 and T-treated, n = 7). (B) The brain weight of both sexes wild, control and T-treated black jacobins did not differ. The data points represent individual measurements of females (wild, n = 7; control, n = 3 and T-treated, n = 7; lighter dots) and males (wild, n = 7; control, n = 9 and T-treated, n = 5; darker dots). (C) The testes weight of wild, control and T-treated males also did not differ (wild, n = 7; control, n = 9 and T-treated, n = 5). All box plots indicate the median and interquartile range (25 to 75%).
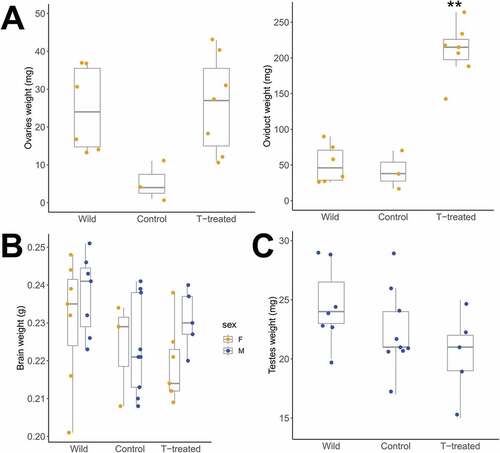
No difference in brain weight was found between groups in either females and males [females, median (25, 75) quartiles: wild 0.235 g (0.224, 0.242), control 0.229 g (0.219, 0.232) and T-treated 0.214 g (0.212, 0.223), (H = 1.79, df = 2, P = 0.409); males, wild 0.241 g (0.229, 0.245), control 0.221 g (0.213, 0.238) and T-treated 0.230 g (0.227, 0.237); F (2,18) = 2.98, P = 0.076; ].
Testosterone treatment did not affect song or chirp emissions of males and females
Males tended to sing more than females (% of male plus female songs emitted in a time window) before T-treatment (males: 79%, females: 21%), 1 week after T-treatment (males: 76%, females: 24%) and 2 weeks after T-treatment (males: 57%, females: 43%); however, this difference was not significant (fixed effects estimate = 0.46 ± 0.54, z = 0.84, P = 0.398, ). The likelihood ratio test showed that T-treatment did not affect the emission of song in males and females (χ2 = 6.12, df = 4, P = 0.191). Disregarding T-treatment or sex, black jacobins sang less in the afternoon than in the morning in the aviaries (linear: fixed effects estimate = − 20.46 ± 2.81, z = − 7.29, P < 0.001 and quadratic term: fixed effects estimate = − 8.13 ± 1.73, z = − 4.71, P < 0.001, , Fig. S4 in Supplemental Data). This singing pattern is similar to the daily variation in singing usually found in birds in the wild (McNamara et al. Citation1987; A. Monte personal observation). Disregarding T-treatment, 2 weeks after T-treatment, males sang less than before (fixed effects estimate = − 0.91 ± 0.27, z = − 3.39, P = 0.001, ). Males emitted fewer songs [predicted means 95% CI (lower, upper confidence interval)] the longer they were in the aviaries [before T-treatment: control 26.2 songs/20 min (14.8, 46.7) and T-treated 14.8 (6.3, 35), 1 week after T-treatment: control 17.5 songs/20 min (9.2, 33.4) and T-treated 10 (4.5, 22) and 2 weeks after T-treatment: control 15.8 songs/20 min (8.2, 30.2) and T-treated 6.1 (2.6, 14)] and females, in special those from the control group, sang more [before T-treatment: control 16.6 (6.5, 42.5) and T-treated 12.8 (6.4, 25.4), 1 week after T: control 18.7 (7.1, 49.5) and T-treated 14.5 (7.4, 28.5) and 2 weeks after T: control 24.8 songs/20 min (9.9, 62) and T-treated 13 (6.8, 24.9), , Table S4 in Supplemental Data]. Dispersion of the data tended to be greater in males in comparison to female (dispersion effects, estimate = 0.54 ± 0.22, z = 2.48, P = 0.013, ).
Table 4. Results of the generalized linear mixed model explaining variation in the number of songs emitted per observation session (20 min) of captive black jacobins. For a better fit, the hour of the day was transformed into linear, quadric and cubic terms. The birds tend to emit fewer songs throughout the day and males sing less than females 2 weeks after the testosterone treatment. In bold are the significant predictors (P < 0.01).
Fig. 3. Testosterone treatment (T-treatment) did not affect the number of songs and chirps emitted by both sexes in black jacobin. (A) Spectrogram (frequency with time) and oscillogram (relative amplitude with time) of the black jacobin’s song and (C) chirp. The song is composed of a single high-pitched syllable (vocal unit) that can be repeated multiple times in a song bout. The chirp is characterized by a wide frequency bandwidth in a fast duration that can also be repeated consecutively in a chirp bout. (B) The number of songs produced by both sexes in control and T-treated groups did not differ before, 1 or 2 weeks after T-treatment. (D) Although T-treated females tended to consistently produce more chirps, the number of chirps produced by both sexes in control and T-treated groups also did not differ significantly before, 1 or 2 weeks after T-treatment. The data points represent song or chirp counts obtained per bird in 20 min of observation. The error bars represent the fitted mean and the 95% confidence interval given by the generalized linear mixed models.
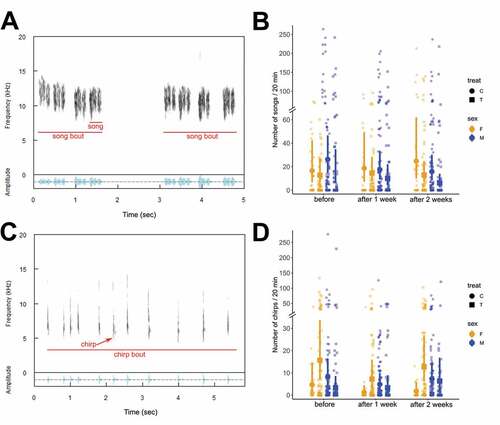
Males and females emitted similar number of chirps () (% of total male plus female chirps per time window; before T-treatment: males: 56% and females 44%; 1 week after T: males 58%, females 42%; 2 weeks after T: males 49%; females 51%, fixed effects estimate = 0.57 ± 0.67, z = 0.85, P = 0.395, ). The likelihood ratio test indicated that T-treatment also did not affect the emission of chirps in both sexes (χ2 = 12.75, df = 8, P = 0.121). Disregarding T-treatment or sex, black jacobins emitted fewer chirps one (fixed effects, estimate = − 1.30 ± 0.38, z = − 3.39, P < 0.01) and 2 weeks after T-treatment (fixed effects, estimate = − 0.96 ± 0.35, z = − 2.77, P < 0.01) (, ). When the birds were not emitting chirps, they were feeding in the feeders (zero-inflated effects, estimate = − 1.14 ± 0.34, z = − 3.34, P < 0.01). T-treatment and sex jointly had an effect closer to a significance for T-treated males in relation to controls and females (fixed effects interaction between T-treatment and sex, estimate = − 2.12 ± 0.87, z = − 2.44, P = 0.015). The means estimated from the best-fitting model for chirp counts show that T-treated females produced 15.67 chirps (7.26, 33.81) [estimated mean (lower, upper 95% CI)] before, 7.19 chirps (3.25, 15.9) 1 week after T and 12.73 chirps (6.02, 26.93) 2 weeks after T (, Table S5 in Supplemental Data). The dispersion of the data explained by the model was greater in aviary A1 in relation to aviary A2 (dispersion effects, estimate = − 1.48 ± 0.25, z = − 5.84, P < 0.01), A3 (dispersion effects, estimate = − 0.68 ± 0.25, z = − 2.69, P < 0.01) and A4 (dispersion effects, estimate = − 1.29 ± 0.25, z = − 5.13, P < 0.01).
Table 5. Results of the generalized linear mixed model explaining variation in the number of chirps emitted per observation session (20 min) of captive black jacobins. The birds tend to emit fewer chirps across time. In bold are the significant predictors (P < 0.01).
Testosterone treatment did not affect the acoustic parameters of the song of males and females
Black jacobin song can be composed of one (single), two (double) or three (triplets) subunits (Fig. S3A in Supplemental Data). The proportion of singles, doubles and triplets was stable before, 1 week and 2 weeks after T-treatment, because these proportions showed high repeatability within birds (R = 0.46 ± 0.11, P < 0.01). Singles were rare, most of the birds emitted triplets and three males emitted mostly only doubles (Fig. S3B in Supplemental Data).
Concerning the acoustic parameters of the song, a Principal Components Analysis (PCA) reduction revealed the first three eigenvalues to contain ~ 75% of the overall variation. PC1 (~ 36% of the variation) described mainly spectral parameters (such as frequency mean, frequency standard deviation, median, lower (25%) and upper (75%) frequency quantiles, spectral entropy, spectral flatness, dominant frequency, minimum dominant frequency, dominant frequency range, start dominant frequency, as well as peak frequency mean); PC2 (~ 23% of the variation) described temporal parameters [duration, median time, upper (75%) quantile and time interquartile range as well as time entropy] and PC3 (~ 16% of the variation) described skewness and kurtosis (Tables S6-S7 in Supplemental Data). PC1 and PC2 did not separate control from T-treated birds neither in females nor in males, although showing a slight separation between sexes ().
Fig. 4. Testosterone treatment (T-treatment) did not affect the acoustic parameters of the song in both sexes of black jacobin. (A) Biplot including the first two components (PC1 versus PC2) of a Principal Components Analysis including 26 acoustic parameters of black jacobin’s song (see Table S1 in Supplemental Data for a list of the parameters). The first component PC1 explained 36% of the overall variation and described mainly spectral parameters such as dominant frequency. The second component PC2 explained 23% of the variation and described temporal parameters such as duration. Each data point represents the mean of the PCA loads per bird in three time points: before, 1, and 2 weeks after T-treatment. If T-treatment had affected and differentiated the acoustic parameters of the song, we expected to see a clear separation of the PCA points of T-treated birds (dashed circle) after the T-treatment in comparison to the controls (solid circle). Instead, the biplot shows a complete overlap between T-treated and controls in both sexes indicating that there are no clear sex or treatment differences in the acoustic parameters of the song before, 1 or 2 weeks after the T-treatment. Nevertheless, a small separation between sexes can be observed, because some males had consistently more positive loadings along PC1 axis and some females more negative reflecting a trend of male’s song toward higher pitch independent of treatment or time. (B) The dominant frequency (left panel) and the duration (right panel) of black jacobin’s song in both sexes of control and T-treated groups did not differ before, 1 or 2 weeks after T-treatment. Males consistently tended to sing higher-pitched songs than females, whereas females consistently tended to sing longer songs than males, but the differences were not significant. All box plots indicate the median and interquartile range (25 to 75%). Each data point indicates an individual song measurement (see for details on sample size). FC, female control; FT, female T-treated; MC male control and MT, male T-treated.
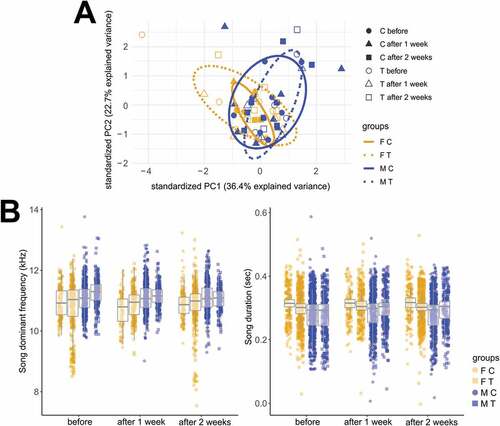
We further investigated the parameters with the highest eigenvalue in PC1, the mean dominant frequency of the song, and in PC2, the song duration. The mean dominant frequency of the male and female songs was similar in control and T-treated groups across time (Fr = 9, df = 3, P = 0.029). The mean dominant frequencies [data are: median and (25, 75) quartiles] of control females were 10.9 kHz (10.5, 11.3) before T (n = 178 songs), 10.8 kHz (10.3, 11.1) (n = 107 songs) 1 week after T and 10.9 kHz (10.6, 11.1) (n = 146) 2 weeks after T-treatment. For the T-treated females, the mean dominant frequencies were 11 kHz (10.5, 11.3) before T (n = 342 songs), 10.9 kHz (10.5, 11.2) 1 week after T (n = 201 songs) and 11 kHz (10.7, 11.2) 2 weeks after T (n = 370 songs). For control males, mean dominant frequencies were 11.1 kHz (10.8, 11.4) before T (n = 624 songs), 11.1 kHz (10.8, 11.4) 1 week after T (n = 545 songs) and again 11.1 kHz (10.8, 11.4) 2 weeks after T (n = 466 songs). For T-treated males, the mean dominant frequencies were 11.3 kHz (11, 11.5) before T (n = 268 songs), 11.2 kHz (10.9, 11.4) 1 week after T (n = 228 songs) and 11.1 kHz ± (10.8, 11.3) 2 weeks after T treatment (n = 185 songs) (, left panel).
The same consistency was observed in the medians of the song duration. Control females had nearly the same median before, 1 and 2 weeks after T-treatment [0.32 sec median interquartile range (0.30, 0.33)]. T-treated females had little variation in their medians before [0.30 sec (0.28, 0.31)], 1 [0.30 sec (0.29, 0.32)] and 2 weeks after T-treatment [0.30 sec (0.29, 0.31)]. Control males had also nearly the same median before, 1 and 2 weeks after T-treatment [0.29 sec (0.25, 0.31), except for the median 1 week after T-treatment which was 0.30 sec]. T-treated males tended to sing slighter shorter songs before [0.29 sec (0.24, 0.31)] in relation to 1 [0.30 sec (0.28, 0.32)] and 2 weeks after T-treatment [0.31 sec (0.27, 0.32)]. Although male’s songs were around 10 msec shorter than those of the females, the averages of the control females, control males, T-treated females and T-treated males were similar before, 1 and 2 weeks after the T-treatment (Fr = 7, df = 3, P = 0.072) (, right panel). Both the dominant frequency and duration of the song were highly repeatable within birds (dominant frequency: R = 0.40 ± 0.07, P < 0.001; duration: R = 0.37 ± 0.07, P < 0.001).
Testosterone treatment maintained high aggressiveness in males in comparison with a decrease in placebo birds
We counted the number of attacks (chasing other birds or beak pecking on another bird) as a proxy for aggressiveness. In all observations and regardless of sexes, the observed birds attacked another bird twice or more in 55% of the observations, once in 37% and did not attack in 8%, which indicates the intrinsic high aggressiveness of the black jacobins. The number of attacks was weakly associated with song counts (r = 0.16, P < 0.001).
The number of attacks before T compared to 2 weeks after T was influenced by time (estimate = − 0.67 ± 0.19, z = − 3.58, P < 0.001), by temperature (estimate = − 0.20 ± 0.05, z = − 3.76, P < 0.001) and by the feeding counts to the feeders (estimate = 0.25 ± 0.03, z = 7.21, P < 0.001) (). A post-hoc analysis of interactions indicated that the control birds of both sexes attacked less as longer they spent in captivity (females χ2 = 12.79, df = 2, P = 0.007 and males χ2 = 23.37, df = 2, P < 0.001), whereas T-treated birds of both sexes kept the number of attacks similar to baseline values (females χ2 = 3.56, df = 2, P = 0.674 and males χ2 = 3.68, df = 2, P = 0.635). The number of attacks of the T-treated females was relatively low before T-treatment by chance [1.84 attacks 95% CrI (1.36, 2.46)], but these values remained similar 1 [1.59 attacks (1.18, 2.13)] and 2 weeks after the T-treatment [1.37 attacks (1.03, 1.89)]. The number of attacks of the T-treated males attacked was similar before [2.65 attacks (1.93, 3.70)], 1 week [2.68 attacks (1.97, 3.76)] and 2 weeks after the T-treatment [2.03 attacks (1.46, 2.87)] ().
Table 6. Results of the generalized linear mixed model explaining variation in the number of attacks per observation session (20 min) of captive black jacobins. For a better fit, all continuous predictors were mean-centered (z-scored). The number of attacks was affected by time 2 weeks in relation to before treatment, temperature and number of feeds on the feeder. In bold are the significant predictors (P < 0.01).
Fig. 5. Testosterone treatment (T-treatment) maintained aggressiveness of T-treated birds towards initial values (post hoc analysis of the mixed-effect model; female χ22 = 3.56, P = 0.67; male, χ22 = 3.68, P = 0.63), whereas controls become less aggressive with time (female, χ22 = 12.79, P = 0.007; male, χ22 = 23.37, P < 0.001). The data points represent attack counts obtained per bird in 20 min of observation as a proxy for aggressiveness. The error bars represent the estimated mean and the 95% credible interval given by the generalized linear mixed model.
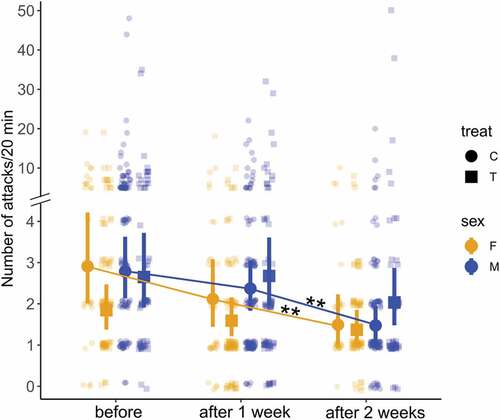
The number of times the birds feed on the feeders in the aviary was not affected by T-treatment (estimate = − 0.06 ± 0.20, z = − 0.30, P = 0.761), nor by the time they spent in captivity 1 (estimate = − 0.27 ± 0.14, z = − 1.93, P = 0.054) or 2 weeks after T-treatment (estimate = 0.14 ± 0.14, z = 1.06, P = 0.290) in relation to the baseline before T (Fig. S5, Table S3 in Supplemental Data). However, T-treated males and females tended to catch more fruit flies 2 weeks after T-treatment. The best-fitting model for fruit-fly captures indicated that the number of captures was affected by the interaction of T-treatment and the time they spent in captivity after 2 weeks (estimate = 1.57 ± 0.43, z = 3.68, P < 0.001) and also by the number of feeding from the feeders (estimate = 0.27 ± 0.05, z = 5.39, P < 0.001, Table S3 in Supplemental Data). A post hoc analysis of interactions indicated that the control birds of both sexes captured the same number of fruit flies across time (females χ2 = 7.34, df = 2, P = 0.102 and males χ2 = 4.86, df = 2, P = 0.353), whereas T-treated birds tended to capture more fruit-flies as longer they spent in captivity, but this difference was non-significative (females χ2 = 9.86, df = 2, P = 0.029 and males χ2 = 9.71, df = 2, P = 0.031) (Fig. S5 in Supplemental Data).
Discussion
This study presents the first profile of testosterone plasma levels (T-levels) and the effects of testosterone treatment (T-treatment) on the vocal behavior in females and males of a hummingbird species, the black jacobin. Although T-levels of black jacobins in the wild were higher in males, vocal behaviors of control and testosterone treated males and females did not differ. Therefore, the vocal behavior of these hummingbirds seems insensitive to testosterone in contrast to species of other avian families in which testosterone either affects vocal output or vocal pattern or both (Gahr Citation2014, Citation2020). Note that T-levels tended to get lower in both sexes towards the end of the breeding season (March compared to December), indicating that they can change during the breeding season or/and throughout the year. In relation, 7% of the males had T-levels comparable to reproductively active males of other species in the tropics (Goymann et al. Citation2004), which, too, indicates that T-levels vary during the breeding season. The large individual differences of plasma T-levels are likely due to the long breeding period of tropical species, which leads to de-synchronized reproductive activities within populations.
The T-levels of wild populations of hummingbirds were so far documented in only a few species given the methodological constraints to obtaining blood samples of such small birds. Previously, T-levels of hummingbirds were estimated from measurements of testosterone concentrations in the cloacal fluid of three species, all of the clade Bees, during the breeding season. In the black-chinned hummingbird (Archilochus alexandri), males had higher T-levels than females, whereas in Anna’s hummingbird (Calypte anna) and the rufous hummingbird (Selasphorus rufus) males and females had similar T-levels (González-Gómez et al. Citation2014). Surprisingly, although these species weigh about half of black jacobin’s body weight, their average T-levels were about 90-fold higher than that of black jacobins, their T-levels ranged between ~ 8 and ~ 23 ng/mL (González-Gómez et al. Citation2014), whereas the T-levels of wild black jacobins ranged (25 to 75% quantiles) from ~ 0.03 to 0.1 in females and ~ 0.05 to 0.2 ng/mL in males (). However, a fraction of males and females had testosterone levels typical of reproductively active male and female birds of the temperate zones with T-levels above 1 ng/mL plasma for males and 100 pg/mL plasma for females. This difference may reflect (i) the distant relationship of black jacobins (clade Topazes) and the Bees in the hummingbird family; (ii) a sampling methodology difference as T-levels quantified from the cloacal fluid can be distorted due to individual differences caused by the metabolism of hormones by gut bacteria and by matrix effects (Goymann Citation2012) or, more likely, (iii) methodological problems in measuring testosterone levels from cloacal fluid; such high T-levels are only found in birds after testosterone treatment (Goymann & Wingfield Citation2014; this study).
The T-levels of the wild black jacobin were collected in the middle of their 7 months long breeding season (Ruschi Citation1964), although there was no information about the exact life stage such as incubating or having off-springs of the sampled birds, the high T-levels of 7% of wild males indicate their reproductive status. Interestingly, if we calculate the median of the plasma T-levels by considering only wild males with values above 1 ng/mL (n = 11), the outcome is 1.8 ng/mL (1.4, 3.3) which is rather low when compared to average plasma T-levels of northern temperate birds in the wild during the breeding season (2.8 ± 0.4 ng/mL), but on the range of tropical birds (1.3 ± 0.2 ng/mL) (Goymann et al. Citation2004). For tropical birds, it is suggested that their maxima T-levels is mainly determined by the length of the breeding season and the altitude they occur: species with longer breeding seasons (above 4 months) tends to show relatively lower T-levels and species inhabiting higher altitudes, higher values (Goymann et al. Citation2004). Therefore, the black jacobin as a species with long breeding season that occupy relatively lower altitudes is expected to have the observed T maxima.
The T-values of the T-treated black jacobins at the end of the experiment was about 300 times higher than control in males but 33 times higher than wild males with highest T-levels (> 1 ng/mL, n = 11) and 2,500 times higher than control in females but 350 times higher than wild females with highest T-levels (> 0.1 ng/mL, n = 17). In relation, T-values of intact breeding males of zebra finches after T implantation that are ~ 4 times higher than controls has been shown to induce singing and reduce time spent in close proximity to their partner (Prior et al. Citation2016). In castrated males of canaries, T-values 2 days after implantation can be ~ 10 times higher than controls and induce higher singing rates (Shevchouk et al. Citation2019). In intact and photostimulated males of house sparrow (Passer domestics), a dose-dependent effect was observed: after low doses, T-values were up to 2 times higher than control and induced testes atrophy, whereas after high doses, T-values ranged from 2 to 10 times higher while spermatogenic activity was maintained (Turek et al. Citation1976). Despite the much higher circulating T-levels in both sexes of T-treated black jacobins, neither gonadal, body and brain weights nor vocal behavior were affected; testosterone only affected oviduct growth in females and the maintenance of high aggressiveness in both sexes.
It is important to keep in mind that, in some species, T-treatment induce singing in castrated or intact birds in non-breeding condition but has no effect when administered in intact birds in breeding condition because T-levels reached a certain physiological threshold (reviewed in Fusani Citation2008; Goymann & Wingfield Citation2014). In ring doves (Streptopelia risoria), for example, castrated males vocalize more after T-treatment (Cohen & Cheng Citation1982); however, in intact males in breeding conditions both low and high dosage T-implants did not affect any element of their courtship activity including the sexually relevant vocalizations (Fusani & Hutchison Citation2003). Although T-treatment was conducted in intact and potentially breeding birds, this is unlikely the case for the black jacobin because T-treatment modulated aggressiveness and induced oviduct atrophy indicating that there was not a generalized lack of effect due to the supraphysiological T-levels in treated birds. Furthermore, a sex difference was observed in the T-levels of wild birds but not on vocal rates which reinforce the hypothesis that none of these vocalizations are a T-sensitive trait. T-implantation in castrated black jacobins would require developing surgical procedures and is, therefore, difficult to perform. Nevertheless, implants with anti-androgens in birds whose life-stages are known may help to further confirm our findings. The quantification of testosterone metabolites DHT (binds to androgen receptors) and estradiol (binds to estrogen receptors) also remains to be seen. In chickens, testosterone and estrogen act synergically on the preparation of the magnum – part of the oviduct – for normal secretion of albumen into the egg; when administered alone, testosterone increased magnum weight, but did not induce the formation of albumin secreting cells (Yu & Marquardt Citation1973). In black jacobin females, the presence of androgen receptors in the part of the oviduct, that is histologically similar to the magnum, may explain the testosterone-induced oviduct growth (A. Monte unpublished data). Testosterone treatment did not affect the weight of the testis of male black jacobins. This result is in line with some other species in which high T-levels did not decrease testis weight (Turek et al. Citation1976; Desjardins & Turek Citation1977) while in other species elevated T-levels regressed the testis (Chu Citation1940).
Hummingbirds are aggressive year-round, especially while foraging (Greenewalt Citation1990; Schuchmann Citation1999). Aggressiveness levels (number of attacks) of T-treated black jacobins were maintained during the whole experiment compared to a reduction in control birds over time in captivity. In seasonal species, aggressiveness is correlated with T-levels (Wingfield et al. Citation1987; reviewed in Soma Citation2006). To cope with the costs of high T-levels, these birds raise T-levels, particularly in periods of social instability during territorial challenges. Conversely, when their status or territory boundaries are maintained (social stability), T-levels are kept low (Wingfield et al. Citation1990). In the aviaries, the reduction in the number of attacks of control black jacobins across time may be explained by social stability; there was one feeder for every two birds, thus some birds might have alternated instead of disputed the same feeder. A similar use of the feeders was also observed in wild black jacobins, they awaited a free feeder instead of competing for feeders (A. Leitão personal observation).
We observed no effects of the T-treatment on the vocal rates and the acoustic parameters of the song, in contrast with other bird species investigated to date (reviewed in Gahr Citation2014, Citation2020). In the brain, testosterone can act on the medial preoptic nucleus (POM) or directly in the vocal control system (reviewed in Gahr Citation2014; Shevchouk et al. Citation2019). For example, direct testosterone implantation in POM of castrated male canaries induced high vocal rates but increased either vocal rate and song stereotypy when administered concomitantly in POM and HVC, an important nucleus of the vocal control system (reviewed in Alward et al. Citation2017). In the POM and song control regions of songbirds, there are androgen receptors (AR) and estrogen receptors that mediate the activity of testosterone and its androgenic and estrogenic metabolites on the cellular level (reviewed in Ball & Balthazart Citation2007). Similar, AR is expressed in the putative song control region of two hummingbird species, Anna’s hummingbird (Calypte anna) and amazilia hummingbird (Amazilia amazilia) (Gahr Citation2000). AR and estrogen receptors in the POM are a general feature of all birds (reviewed in Gahr Citation2020). Although the reason for life-stage dependent effects of testosterone is unknown, we can speculate that the number of AR (Fusani et al. Citation2000; Quispe et al. Citation2016) or of testosterone metabolizing enzymes such as 5ß-reductase varies seasonally; birds reduce testosterone to 5ß-dhihydrotestosterone that cannot activate the AR (Floch et al. Citation1985). Likewise, we could assume that AR is expressed at low levels or 5ß-reductase is expressed at high levels in the putative song control brain regions of the black jacobin so that testosterone cannot affect song rate and pattern. Further, although we quantified AR in the syrinx of the black jacobin, these AR were not in muscles but in skeletal parts of the syrinx (A. Monte unpublished data), which makes an activating effect of testosterone on the syrinx and song unlikely. The only finding that indicates hormonal regulation of the song of the black jacobin is a previous report suggesting a seasonal change in the entropy of the song (Olson et al. Citation2018). However, these birds were not ringed and were recorded in the wild at the beginning (September) and end of the breeding season (March) (Olson et al. Citation2018). Thus, this seasonal change could be due to the fact that more juvenile birds were recorded at the end of the breeding season rather than an effect of testosterone. Entropy measurements of the song of our birds were not affected by testosterone (Fr = 5, df = 3, P = 0.172, Fig. S6 in Supplemental Data).
The insensitivity of vocalization rates and song features to testosterone in both sexes combined with our observations of the behavior of the birds indicates that none of these vocalizations are used exclusively in a sexual context. Chirps were mainly produced in flight shortly before feeding, whereas song was mainly produced when perched or sometimes in flight when chasing another bird (Fig. S7 in Supplemental Data). Black jacobins were observed to use their songs outside of the breeding season for defending food resources (Olson et al. Citation2018; A. Monte personal observation). Hence, it could be conceivably hypothesized that year-round song types that are also used in non-sexual contexts are not sensitive to testosterone. Thus, the American crow (Corvus brachyrhynchos), for example, which uses its song all year long in multiple contexts to facilitate affiliative behaviors among group members (Brown Citation1985) is not expected to show a correlation between T-levels in contrast with species that use their song as a secondary sexual trait during breeding season in which song is modulated by testosterone as in the common chaffinch (Fringilla coelebs) (Thorpe Citation1958) or canaries (Fusani et al. Citation2000). Further studies on the seasonality of black jacobin song and the behavioral context of their emission are, nevertheless, needed to support this hypothesis.
Hummingbirds have the highest metabolic rates amongst homeotherm vertebrates (Suarez Citation1992), which may constrain their daily activities towards finding and defending nectar-rich food sources. Thus, we speculate that, in black jacobin, the song is used by both sexes primarily to defend food sources. Although it remains to be seen whether the testosterone-insensitivity of black jacobin’s vocalizations is life-stage dependent, it is possible that the non-reproductive use of song implied the loss of the activation effect of testosterone in their vocal control system. The courtship display of the black jacobin is a joint flight of both sexes in which males chase the female around 100 m upward in a stepwise fashion followed by a synchronously straight dive (Ruschi Citation1949). Thus, we speculate that, in black jacobin, mate choice is primarily based on visual displays and visual sensory processing. Thus, it needs to be seen if the non-reproductive use of song implied the loss of the activation effect of testosterone in their vocal control system, and in turn, the evolution of testosterone-sensitive visual displays and visual processing.
Supplemental Material
Download MS Word (1.5 MB)Acknowledgments
We thank all the staff of the Professor Mello Leitão Biology Museum (Museu de Biologia Professor Mello Leitão) and the National Institute of the Atlantic Forest for their support with the challenging field logistics, the former director Hélio de Queiroz Boudet Fernandes and the actual director Sergio Lucena Mendes for the administrative support. We thank Antje Bakker and Marcella Bader-Blukott for molecular sexing of the animals, Renan Betzel for helping with behavioral data collection of captive birds, Dr Wolfgang Goymann for valuable advice in the initial phase of the project, Dr Mariana Diales Rocha for assisting the elaboration of the testosterone implants, Dr Lucía Mentesana and Dr Nicolás Adreani for helpful advice on the statistical analysis, Dr Michaela Hau for insightful comments on the manuscript and Dr Silene Maria Araujo de Lima for assisting the project execution and application to the ethics committee.
Disclosure statement
No potential conflict of interest was reported by the authors.
Supplemental data
Supplemental data for this article can be accessed at https://doi.org/10.1080/03949370.2023.2181873
Additional information
Funding
References
- Altmann J. 1974. Observational study of behavior: sampling methods. Behaviour. 49(3):227–267. doi:10.1163/156853974X00534
- Alward BA, Rouse ML, Balthazart J, Ball GF. 2017. Testosterone regulates birdsong in an anatomically specific manner. Anim Behav. 124:291–298. doi:10.1016/j.anbehav.2016.09.013
- Andrew RJ. 1963. Effects of testosterone on the behavior of the domestic chick. J Prof Nurs. 56(5):933–940. doi:10.1037/h0045105
- Araya-Salas M, Smith-Vidaurre G. 2017. WarbleR: an r package to streamline analysis of animal acoustic signals. Methods Ecol Evol. 8(2):184–191. doi:10.1111/2041-210X.12624
- Arnold AP. 1975. The effects of castration and androgen replacement on song, courtship, and aggression in zebra finches (Poephila guttata). J Exp Zool. 191(3):309–325. doi:10.1002/jez.1401910302
- Audacity Team. 2013. Audacity (2.0.3) [Computer software]. Available from: http://www.audacityteam.org/home/ [Accessed 25 May 2018].
- Ball GF, Balthazart J. 2007. The neuroendocrinology and neurochemistry of birdsong. In: Lajtha A Blaustein J, editors. Handbook of neurochemistry and molecular neurobiology: behavioral neurochemistry, neuroendocrinology and molecular neurobiology. Boston (MA): Springer US; p. 419–457. doi:10.1007/978-0-387-30405-2_10
- Balthazart J, Schumacher M, Ottinger MA. 1983. Sexual differences in the Japanese quail: behavior, morphology, and intracellular metabolism of testosterone. Gen Comp Endocrinol. 51(2):191–207. doi:10.1016/0016-6480(83)90072-2
- Baptista LF, Schuchmann K-L. 1990. Song learning in the Anna hummingbird (Calypte anna). Ethology. 84(1):15–26. doi:10.1111/j.1439-0310.1990.tb00781.x
- Beani L, Briganti F, Campanella G, Lupo C, Dessì-Fulgheri F. 2000. Effect of androgens on structure and rate of crowing in the Japanese quail (Coturnix japonica). Behaviour. 137(4):417–435. doi:10.1163/156853900502150
- Bolker B, R Development Core Team. 2020. Bbmle: tools for general maximum likelihood estimation (1.0.23.1). [R package]. Available from: https://CRAN.R-project.org/package=bbmle [Accessed 17 Aug 2020].
- Brockway BF. 1968. Influences of sex hormones on the loud and soft warbles of male budgerigars. Anim Behav. 16(1):5–12. doi:10.1016/0003-3472(68)90099-7
- Brown ED. 1985. the role of song and vocal imitation among common crows (Corvus brachyrhynchos). Z Tierpsychol. 68(2):115–136. doi:10.1111/j.1439-0310.1985.tb00119.x
- Burnham KP, Anderson DR. 2016. Multimodel inference: understanding AIC and BIC in model selection. Sociol Methods Res. doi:10.1177/0049124104268644
- Catchpole CK, Slater PJB. 1995. Bird song: biological themes and variations. 2nd ed. Cambridge (UK): Cambridge University Press.
- Chiver I, Schlinger BA. 2019. Sex-specific effects of testosterone on vocal output in a tropical suboscine bird. Anim Behav. 148:105–112. doi:10.1016/j.anbehav.2018.12.011
- Chu JP. 1940. The effects of oestrone and testosterone and of pituitary extracts on the gonads of hypophysectomized pigeons. J Endocrinol. 2(2):21–37. doi:10.1677/joe.0.0020021
- Cohen J, Cheng M-F. 1982. Effects of testosterone metabolites and estrogen in the midbrain control of courtship behavior in the male Ring dove (Streptopelia risoria). Neuroendocrinology. 34(1):64–74. doi:10.1159/000123279
- da Silva ML, Vielliard J. 2006. Entropy calculations for measuring bird song diversity: the case of the White-vented violet-ear (Colibri serrirostris) (Aves, Trochilidae). Razprave IV. Razreda SAZU. 47:37–49.
- Desjardins C, Turek FW. 1977. Effects of testosterone on spermatogenesis and luteinizing hormone release in Japanese quail. Gen Comp Endocrinol. 33(2):293–303. doi:10.1016/0016-6480(77)90253-2
- Ferreira ARJ, Smulders TV, Sameshima K, Mello CV, Jarvis ED. 2006. Vocalizations and associated behaviors of the Sombre hummingbird (Aphantochroa cirrhochloris) and the Rufous-breasted hermit (Glaucis hirsutus). Auk. 123(4):1129–1148. doi:10.2307/25150225
- Ficken MS, Rusch KM, Taylor SJ, Powers DR. 2000. Blue-throated hummingbird song: a pinnacle of nonoscine vocalizations. Auk. 117(1):120–128. doi:10.1093/auk/117.1.120
- Floch JY, Morfin R, Picart D, Daniel JY, Floch HH. 1985. Testosterone metabolism in the uropygial gland of the quail. Steroids. 45(5):391–401. doi:10.1016/0039-128X(85)90004-2
- Fox J, Weisberg S. 2018. An R companion to applied regression. Newbury Park (CA): SAGE Publications.
- Fusani L. 2008. Endocrinology in field studies: problems and solutions for the experimental design. Gen Comp Endocrinol. 157(3):249–253. doi:10.1016/j.ygcen.2008.04.016
- Fusani L, Beani L, Dessì-Fulgheri F. 1994. Testosterone affects the acoustic structure of the male call in the Grey partridge (Perdix perdix). Behaviour. 128(3/4):301–310. doi:10.1163/156853994X00307
- Fusani L, Hof TV, Hutchison JB, Gahr M. 2000. Seasonal expression of androgen receptors, estrogen receptors, and aromatase in the canary brain in relation to circulating androgens and estrogens. J Neurobiol. 43(3):254–268. doi:10.1002/_SICI_1097-4695_20000605_43:3<254:AID-NEU4>3.0.CO;2-W
- Fusani L, Hutchison JB. 2003. Lack of changes in the courtship behaviour of male ring doves after testosterone treatment. Ethol Ecol Evol. 15(2):143–157. doi:10.1080/08927014.2003.9522679
- Gahr M. 2000. Neural song control system of hummingbirds: comparison to swifts, vocal learning (songbirds) and nonlearning (suboscines) passerines, and vocal learning (budgerigars) and nonlearning (dove, owl, gull, quail, chicken) nonpasserines. J Comp Neurol. 426(2):182–196. doi:10.1002/1096-9861(20001016)426:2<182:AID-CNE2>3.0.CO;2-M
- Gahr M. 2014. How hormone-sensitive are bird songs and what are the underlying mechanisms? Acta Acust United Acust. 100(4):705–718. doi:10.3813/AAA.918749
- Gahr M. 2020. Seasonal and life-history stage dependent vocal communication of birds. In: Fritzsch B, editor. The senses: a comprehensive reference, 2nd ed. Amsterdam (Netherlands): Elsevier; p. 163–186. doi:10.1016/B978-0-12-809324-5.24266-1
- González-Gómez PL, Blakeslee WS, Razeto-Barry P, Borthwell RM, Hiebert SM, Wingfield JC. 2014. Aggression, body condition, and seasonal changes in sex-steroids in four hummingbird species. J Ornithol. 155(4):1017–1025. doi:10.1007/s10336-014-1088-y
- Goymann W. 2012. On the use of non-invasive hormone research in uncontrolled, natural environments: the problem with sex, diet, metabolic rate and the individual. Methods Ecol Evol. 3(4):757–765. doi:10.1111/j.2041-210X.2012.00203.x
- Goymann W, Moore IT, Scheuerlein A, Hirschenhauser K, Grafen A, Wingfield JC. 2004. Testosterone in tropical birds: effects of environmental and social factors. Am Nat. 164(3):327–334. doi:10.1086/422856
- Goymann W, Trappschuh M, Jensen W, Schwabl I. 2006. Low ambient temperature increases food intake and dropping production, leading to incorrect estimates of hormone metabolite concentrations in European stonechats. Horm Behav. 49(5):644–653. doi:10.1016/j.yhbeh.2005.12.006
- Goymann W, Wingfield JC. 2014. Male-to-female testosterone ratios, dimorphism, and life history—what does it really tell us? Behav Ecol. 25(4):685–699. doi:10.1093/beheco/aru019
- Greenewalt CH. 1990. Hummingbirds. New York (NY): Dover Publications.
- Hamilton JB. 1938. Precocious masculine behavior following administration of synthetic male hormone substance. Endocrinology. 23(1):53–57. doi:10.1210/endo-23-1-53
- Hart BL. 1988. Biological basis of the behavior of sick animals. Neurosci Biobehav Rev. 12(2):123–137. doi:10.1016/S0149-7634(88)80004-6
- Heid P, Güttinger HR, Pröve E. 1985. The influence of castration and testosterone replacement on the song architecture of canaries (Serinus canaria). Z Tierpsychol. 69(3):224–236. doi:10.1111/j.1439-0310.1985.tb00148.x
- Hoysak DJ, Weatherhead PJ. 1991. Sampling blood from birds: a technique and an assessment of its effect. Condor. 93(3):746–752. doi:10.2307/1368207
- Jarvis ED, Ribeiro S, da Silva ML, Ventura D, Vielliard J, Mello CV. 2000. Behaviourally driven gene expression reveals song nuclei in hummingbird brain. Nature. 406(6796):628–632. doi:10.1038/35020570
- Kapoor JA. 2012. Improved methods for color-marking hummingbirds. J Field Ornithol. 83(2):186–191. doi:10.1111/j.1557-9263.2012.00368.x
- Kern MD, King JR. 1972. Testosterone-induced singing in female white-crowned sparrows. Condor. 74(2):204–209. doi:10.2307/1366289
- Kroodsma DE. 1984. Songs of the Alder flycatcher (Empidonax alnorum) and Willow flycatcher (Empidonax traillii) are innate. Auk. 101(1):13–24. doi:10.1093/auk/101.1.13
- Lahaye SEP, Eens M, Darras VM, Pinxten R. 2012. Testosterone stimulates the expression of male-typical socio-sexual and song behaviors in female budgerigars (Melopsittacus undulatus): an experimental study. Gen Comp Endocrinol. 178(1):82–88. doi:10.1016/j.ygcen.2012.04.021
- Marler P, Kreith M, Willis E. 1962. An analysis of testosterone-induced crowing in young domestic cockerels. Anim Behav. 10(1–2):48–54. doi:10.1016/0003-3472(62)90130-6
- Martin P, Bateson P. 2007. Measuring behaviour: An introductory guide, 3rd ed. Cambridge (UK): Cambridge University Press. doi:10.1017/CBO9780511810893
- McGuire JA, Witt CC, Remsen JV Jr, Corl A, Rabosky DL, Altshuler DL, Dudley R. 2014. Molecular phylogenetics and the diversification of hummingbirds. Curr Biol. 24(8):1–7. doi:10.1016/j.cub.2014.03.016
- McGuire JA, Witt CC, Remsen JV Jr, Dudley R, Altshuler DL. 2009. A higher-level taxonomy for hummingbirds. J Ornithol. 150(1):155–165. doi:10.1007/s10336-008-0330-x
- McNamara JM, Mace RH, Houston AI. 1987. Optimal daily routines of singing and foraging in a bird singing to attract a mate. Behav Ecol Sociobiol. 20(6):399–405. doi:10.1007/BF00302982
- Monte A, Cerwenka AF, Ruthensteiner B, Gahr M, Düring DN. 2020. The hummingbird syrinx morphome: a detailed three-dimensional description of the black jacobin’s vocal organ. BMC Zool. 5(1):7. doi:10.1186/s40850-020-00057-3
- Nespor AA, Lukazewicz MJ, Dooling RJ, Ball GF. 1996. Testosterone induction of male-like vocalizations in female budgerigars (Melopsittacus undulatus). Horm Behav. 30(2):162–169. doi:10.1006/hbeh.1996.0020
- Nottebohm F. 1980. Testosterone triggers growth of brain vocal control nuclei in adult female canaries. Brain Res. 189(2):429–436. doi:10.1016/0006-8993(80)90102-X
- Ogle D, Wheeler P, Dinno A 2020. FSA: fisheries stock analysis (0.8.30) [R package]. Available from: https://github.com/droglenc/FSA [Accessed 5 Dec 2020].
- Olson CR, Fernández-Vargas M, Portfors CV, Mello CV. 2018. Black Jacobin hummingbirds vocalize above the known hearing range of birds. Curr Biol. 28(5):R204–205. doi:10.1016/j.cub.2018.01.041
- Prior NH, Yap KN, Liu TQD, Vignal C, Soma KK. 2016. Context-dependent effects of testosterone treatment to males on pair maintenance behaviour in zebra finches. Anim Behav. 114:155–164. doi:10.1016/j.anbehav.2016.01.023
- Pröve E. 1974. Der Einfluß von Kastration und Testosteronsubstitution auf das Sexualverhalten männlicher Zebrafinken (Taeniopygia guttata castanotis Gould). [The influence of castration and testosterone substitution on the sexual behavior of male zebra finches (Taeniopygia guttata castanotis Gould)]. J Ornithol. 115(3):338–347. German. doi:10.1007/BF01644328
- Quispe R, Sèbe F, da Silva ML, Gahr M. 2016. Dawn-song onset coincides with increased HVC androgen receptor expression but is decoupled from high circulating testosterone in an equatorial songbird. Physiol Behav. 156:1–7. doi:10.1016/j.physbeh.2015.12.027
- Quispe R, Trappschuh M, Gahr M, Goymann W. 2015. Towards more physiological manipulations of hormones in field studies: comparing the release dynamics of three kinds of testosterone implants, silastic tubing, time-release pellets and beeswax. Gen Comp Endocrinol. 212:100–105. doi:10.1016/j.ygcen.2015.01.007
- R Core Team. 2014. R: A language and environment for statistical computing. Vienna (Austria): R Foundation for Statistical Computing. Available from: http://www.R-project.org/ [Accessed 20 Nov 2018].
- Ruschi A. 1949. Observações sobre os Trochilideos. [Observations on the Trochilids]. Bol Mus Biol Prof. Mello Leitão. 7. Portuguese. Available from: http://boletim.sambio.org.br/pdf/bi_07.pdf
- Ruschi A. 1950. A técnica para obtenção de sangue dos troquilídeos em laboratório, sem sacrificá-los e alguns dados biológicos. [The technique for obtaining blood from trochilids in the laboratory, without sacrificing them, and some biological data]. Bol Mus Biol Prof Mello Leitão. 9:1–4. Portuguese.
- Ruschi A. 1964. A estação ou o período de reprodução nos beija-flores. [The breading season of hummingbirds]. Bol Mus Biol Prof. Mello Leitão. 42. Portuguese.
- Ruschi A. 1973. Algumas observações sobre Melanotrochilus fuscus (Vieillot, 1817). [Some Observations on Melanotrochilus fuscus (Vieillot, 1817)]. Bol Mus Biol Prof. Mello Leitão. 51. Portuguese. Available from: http://www.boletimmbml.net/pdf/zo_051.pdf
- Ruschi PA. 2009. A new hummingbird trap. Bol Mus Biol Prof Mello Leitão. 25:67–75.
- Scheff SW. 2016. Chapter 8 - Nonparametric statistics. In: Scheff S, editor. Fundamental statistical principles for the neurobiologist. Cambridge (MA): Academic Press; p. 157–182. doi:10.1016/B978-0-12-804753-8.00008-7
- Schuchmann KL. 1999. Family Trochilidae (Hummingbirds). In: del Hoyo J, et al., editors. Handbook of the birds of the world. Vol. 5. Barn, barnowls to hummingbirds. Barcelona (Spain): Lynx Edicions; p. 34–75.
- Schuchmann KL, Bonnan A. 2019. Family Trochilidae (Hummingbirds). Handbook of the birds of the world alive. Ithaca (NY): Cornell Lab of Ornithology. Available from: https://www.hbw.com/node/52268 [Accessed 7 Jul 2019].
- Shevchouk OT, Ball GF, Cornil CA, Balthazart J. 2019. Rapid testosterone-induced growth of the medial preoptic nucleus in male canaries. Physiol Behav. 204:20–26. doi:10.1016/j.physbeh.2019.02.007
- Sick H. 1997. Ornitologia Brasileira [Brazilian ornithology]. Rio de Janeiro (Brazil): Editora Nova Fronteira. Portuguese.
- Soma KK. 2006. Testosterone and aggression: berthold, birds and beyond. J Neuroendocrinol. 18(7):543–551. doi:10.1111/j.1365-2826.2006.01440.x
- Stoffel MA, Nakagawa S, Schielzeth H. 2017. rptR: repeatability estimation and variance decomposition by generalized linear mixed-effects models. Methods Ecol Evol. 8(11):1639–1644. doi:10.1111/2041-210X.12797
- Suarez RK. 1992. Hummingbird flight: sustaining the highest mass-specific metabolic rates among vertebrates. Experientia. 48(6):565–570. doi:10.1007/BF01920240
- Thorpe WH. 1958. The learning of song patterns by birds, with especial reference to the song of the Chaffinch Fringilla coelebs. Ibis. 100(4):535–570. doi:10.1111/j.1474-919X.1958.tb07960.x
- Turek FW, Desjardins C, Menaker M. 1976. Antigonadal and progonadal effects of testosterone in male House sparrows. Gen Comp Endocrinol. 28(4):395–402. doi:10.1016/0016-6480(76)90147-7
- Vellema M, Diales Rocha M, Bascones S, Zsebők S, Dreier J, Leitner S, Van der Linden A, Brewer J, Gahr M. 2019. Accelerated redevelopment of vocal skills is preceded by lasting reorganization of the song motor circuitry. eLife. 8:e43194. doi:10.7554/eLife.43194
- Voigt C, Leitner S. 2013. Testosterone-dependency of male solo song in a duetting songbird — evidence from females. Horm Behav. 63(1):122–127. doi:10.1016/j.yhbeh.2012.10.006
- Vu VQ. 2011. Ggbiplot: a ggplot2 based biplot (0.55) [R package]. Available from: http://github.com/vqv/ggbiplot [Accessed 21 Sep 2020].
- Wingfield JC, Ball GF, Dufty AM, Hegner RE, Ramenofsky M. 1987. Testosterone and aggression in birds. Am Sci. 75(6):602–608.
- Wingfield JC, Farner DS. 1978. The annual cycle of plasma irLH and steroid hormones in feral populations of the White-crowned sparrow, Zonotrichia leucophrys gambelii. Biol Reprod. 19(5):1046–1056. doi:10.1095/biolreprod19.5.1046
- Wingfield JC, Hegner RE, Dufty AM, Ball GF. 1990. The “Challenge hypothesis”: theoretical implications for patterns of testosterone secretion, mating systems, and breeding strategies. Am Nat. 136(6):829–846. doi:10.1086/285134
- Wingfield JC, Lynn SE, Soma KK. 2001. Avoiding the “costs” of testosterone: ecological bases of hormone-behavior interactions. Brain Behav Evol. 57(5):239–251. doi:10.1159/000047243
- Yu JY-L, Marquardt RR. 1973. Synergism of testosterone and estradiol in the development and function of the magnum from the immature chicken (Gallus domesticus) oviduct. Endocrinology. 92(2):563–572. doi:10.1210/endo-92-2-563