Abstract
Systems methodologies are employed to investigate water quantity and quality problems in Canada’s oil sands from a multiple-objective-decision-making viewpoint. Because water is one of the most important elements for human survival, many countries consider water issues to be of vital concern with respect to national security. Likewise, Canada is not an exception in terms of addressing its water resources problems as being of great import. In particular, water issues, such as large-scale water usage and troublesome polluted water disposal concerns connected to Canada’s oil sands industries, must be resolved. In this paper, Canada’s oil sands are described with respect to their characteristics, scale, and location. Then, technologies for recovering bitumen from oil sands and processes for upgrading the bitumen are discussed in terms of water consumption and water disposal. In addition, the environmental impacts and challenges with regards to water quantity and quality in Canada’s oil sands are examined in order to understand conflicts that have arisen in recent years. Multiple-criteria decision analyses based on the ProGrid methodology are carried out in order to grasp the structure of the conflict over alternatives for using and treating the water resources in oil sands development in Canada. An evaluation matrix, comparing the multiple criteria, is built, and the Language Ladders with different weights are established to allow the various groups of experts to evaluate the alternatives. Based on their evaluations, alternative solutions for the utilization of the water resources in Canada’s oil sands are prioritized with respect to the critical criteria using the ProGrid methodology. In conclusion, the strategic issues in water resources are addressed and priorities are determined to enhance decision-making.
Des méthodologies sont systématiquement utilisées pour analyser les éventuels problèmes tant de qualité que de quantité relatifs aux eaux provenant de l’exploitation des sables bitumineux du Canada et ce, afin de pouvoir prendre les décisions concernant les multiples objectifs visés. Parce que l’eau constitue un des éléments les plus importants pour la survie de l’être humain, plusieurs pays considèrent d’ailleurs les questions relatives à l’eau comme vitales pour leur sécurité nationale. Dans ce même esprit, le Canada ne fait pas exception non plus en ce qui concerne l’importance qu’il attache à tous problèmes relatifs à ses ressources hydriques. En particulier, des questions telles que l’utilisation de l’eau à grande échelle ou encore la disposition préoccupante des eaux usées provenant de l’exploitation des sables bitumineux, se doivent d’être résolues. Les sables bitumineux du Canada sont décrits ici avec leurs caractéristiques, localisation et étendue. Puis, les technologies requises pour traiter le bitume sont présentées en ce qui concerne la quantité d’eau requise et celle dont il faut ensuite disposer. De plus, les impacts sur l’environnement et ces défis concernant les volumes d’eau requis et leurs qualités sont examinés de façon à bien saisir les raisons et la nature des conflits rencontrés au cours des dernières années. Des grilles d’analyses faisant intervenir de multiples critères, basées sur la technologie ProGrid, sont effectuées de façon à saisir la structure des conflits pour ces alternatives d’utilisation et de traitement des eaux utilisés dans l’exploitation des sables bitumineux du Canada. Une matrice d’évaluation comparant ces divers critères a été mise au point et différents modes ou langages sont élaborés en fonction des besoins des différents groupes d’experts appelés à évaluer les alternatives en présence. Basé sur ces évaluations, ces alternatives de solutions pour l’utilisation de l’eau dans l’exploitation des sables bitumineux du Canada, sont priorisées en fonction des critères de la méthode ProGrid. En guise de conclusion, ces questions stratégiques concernant l’utilisation de l’eau sont dûment considérées et les priorités en sont déterminées afin d’en optimiser toute prise de décision.
Introduction
In general, the human body is composed of about 60 percent water, which means water is one of the most significant elements for human survival. Hence, water is directly connected to maintaining human existence, and water security must be considered vital to national security. Currently, Canada is standing at the crossroads of solving its key water resources problems. To achieve this, Canada must search for reasonable and strategic resolutions to the various water challenges. This paper describes characteristics, emerging water issues, and alternatives, with regard to Canada’s oil sands in order to address strategic water issues and to prioritize alternative solutions involving multiple-criteria using the ProGrid methodology. This paper is an expanded version of a paper originally presented at the WATER 2010 conference (Kim and Hipel 2010).
In the past century, river flows in the Prairie Provinces have declined. In particular, summer flows between May and August in the Athabasca River at Fort McMurray decreased by 29 percent from 1970 to 2005, as displayed in Figure (Schindler, Donahue, and Thompson Citation2007). Additionally, while winter flows in the Athabasca River fall to seasonal lows due to being frozen, which is a natural phenomenon, water from the river is extracted constantly to operate surface mining of oil sands. Hence, there is a very high possibility of water shortages during winter in the future (Mannix, A. E., C. Dridi, and W. L. Adamowicz, Citation2010). According to the Alberta Government’s 2006 report, the Athabasca River may not have enough capacity over the long term to sustain all the demands of planned mining operations and simultaneously maintain sufficient stream flows (Radke Citation2006). The report also mentions that Alberta Environment has not been successful in providing timely advice and direction with regard to water use. Moreover, the World Wildlife Fund (Citation2005) warns that warming temperature will have a negative impact on both water quantity and quality in the area.
Figure 1 The decline in average summer flow in the Athabasca River (Source: Schindler, Donahue, and Thompson Citation2007, with permission).
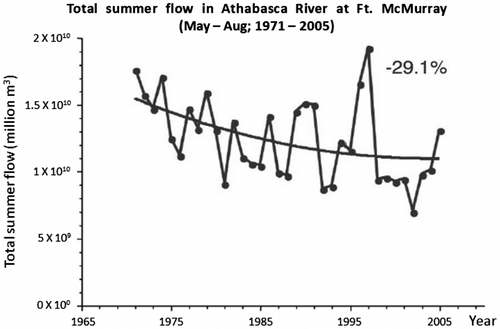
Water challenges in oil sands development
The oil sands (also called tar sands) are viscous mixtures of inorganic materials, bitumen, silt, clay, and water, along with a small portion of other materials (National Energy Board (NEB) 2004). Among the constituents, bitumen is the material used for producing crude oil. Bitumen is a viscous hydrocarbon-based material with a high density, various metal concentrations, and a high carbon-to-hydrogen ratio in comparison to conventional crude oils. Bitumen does not flow to a well in its natural state (Energy Type Citation2009). Therefore, the oil sands industry uses hot water and steam to recover bitumen from the oil sands.
Bitumen in oil sands is recovered in two main ways: surface mining technology and in-situ technology. Surface mining technology can be utilized on oil sands located within 75 metres of the surface. For a barrel of bitumen obtained through surface mining, about two to three barrels of water are withdrawn from the Athabasca River (Humphries Citation2008) and around 85 percent of the water used in the mining process is recycled (Canadian Association of Petroleum Producers (CAPP) 2008). On the other hand, in-situ methods work best for the deposits where the oil sands are deeply buried. For in-situ recovery, steam is injected into drilled wells to heat the bitumen. With reduced viscosity, it moves like conventional oil and can be pumped to the surface, along with recovered water. In-situ technology has greatly reduced water consumption, resulting in only 0.9 barrels of water being used per barrel (Prebble et al. 2009). Furthermore, about 90 to 95 percent of the water consumed in Steam-Assisted Gravity Drainage (SAGD) is recycled (Humphries Citation2008). In order to minimize freshwater consumption, some in-situ projects use water obtained from saline groundwater (Prebble et al. 2009), which reduces the impact on ground and surface water (Woynillowicz, D., C. Severson-Baker, and M. Raynolds, Citation2005). Fortunately, various types of water treatment technologies have been introduced to address water problems in the oil sands business (Allen Citation2008). Nonetheless, the water consumption in the oil sands industry is very high in comparison with the water used for conventional oil production where each barrel of conventional oil requires about 0.1 to 0.3 barrels of water (Prebble et al. 2009).
Heavy use of water in the oil sands industry is one of Canada’s most important environmental concerns, as insufficient water supply and inappropriate water disposal can have serious effects on the nation’s water security. As of 2007, eight percent of all the surface water in Alberta is licensed for withdrawals for all purposes (Nikiforuk Citation2008). The oil sands are consuming around one percent of the Athabasca’s average flow, enough water to sustain a city of two million people every year (Woynillowicz and Severson-Baker 2006), and its consumption from the river is forecasted to be about two percent in the future. The largest use – two thirds of all water consumption from the Athabasca River – is consumed for the oil sands industry (CAPP 2008). Also, less than 10 percent of the water used for the oil sands industry is returned to the Athabasca River (Richardson Citation2007). Hence, reducing the water consumption and increasing the water return volume is a major challenge that must be resolved for oil sands development.
Canada’s oil sands, located almost entirely in the province of Alberta, enable Canada to claim to have the second largest oil reserves in the world with the potential for over 100 years of production. According to statistics from BP (Citation2009), the oil reserves in Canada are estimated to be about 179.3 billion barrels, most of which are contained in Canada’s oil sands. In particular, most of Canada’s oil sands are located in three major areas in Alberta: the Athabasca, Cold Lake, and Peace River regions, as depicted in Figure . The total size of Canada’s oil sands is calculated to be about 140,200 square kilometres, which is an area three times larger than the size of Holland (CAPP 2008). It is estimated that these regions of Alberta contain approximately 1.7 trillion barrels of bitumen; proven resources consist of 173.2 billion barrels of potentially recoverable oil from oil sands (US Energy Information Administration 2010). As of 2008, Canada’s oil sands’ production is 1.31 million barrels per day (Government of Alberta Citation2009) and is forecasted to increase to 3.0 million barrels per day by 2015 (NEB 2006) and 4.3 million barrels per day by 2030 (Prebble et al. 2009). As the production from oil sands in Canada is predicted to increase rapidly, water consumption is also expected to grow at an increasing rate. The current water management framework estimates that potential water supply shortages to the oil sands industry will exist in the future (Mannix, A. E., C. Dridi, and W. L. Adamowicz, Citation2010). From an economic point of view, about 30% of the raw bitumen is being shipped outside of Canada for upgrading and this could increase to 50% by 2019. Hence, Canada is losing much of the “value-added” component of this key resource, such as its associated jobs, technology, and prosperity (Hipel and Bowman Citation2011)
Water quality is affected not only by changes to water quantity, but also by point source or non-point source inputs (Alberta Environment Citation2007). Since the mid-1990s, it has not been known whether increases in oil sands mining and activity have caused any changes in polycyclic aromatic hydrocarbon (PAH) loading (Schindler, D. W., W. F. Donahue, and J. P. Thompson, Citation2007). Moreover, Alberta has very few groundwater monitoring wells for long-term inspections (Prebble et al. 2009), and there is no regional hydrogeological framework to assess the cumulative impacts on groundwater quality (Gosselin et al. 2010). According to a report to the Inter American National Academies of Science (IANAS), there are not enough research programs to investigate potential groundwater pollution caused by oil sands development (Hipel, K. W., A. D. Miall, and D. W. Smith 2011). The Federal Environment Minister, John Baird, announced that Canada does not have a world-class monitoring system to protect against environmental impacts of oil sands development (The Globe and Mail 2010). However, according to a recent study, concentrations of most of the 13 priority pollutants (PPE) listed in the US Environmental Protection Agency’s Clean Water Act were greater near oil sands developments than at remote sites (Kelly et al. Citation2010). Other environmental water problems connected to the mining of oil sands include the creation of large open pits, the disruption of surface and ground water hydrology, and the accumulation of liquid wastes in large tailing ponds (Elshorbagy et al. Citation2005).
Introduction of the ProGrid methodology
The ProGrid methodology (Bowman Citation2005) is a logical decision-assisting tool for multiple-criteria decision analyses, and it has the capacity to help decision makers convert qualitative concepts into quantifiable measures that can be compiled and compared. Hence, the methodology is very useful for evaluating intangible assets such as the innovation capacity of a nation, the effectiveness of governance practices, and the monitoring of long-range societal goals. For example, the Canadian Academy of Engineering has used the ProGrid methodology to evaluate Canada’s energy pathways (Bowman and Griesbach 2007). Evaluating intangibles is similar to estimating an art work’s price at an auction. In other words, a subjective point of view could influence the evaluation, and inaccurate judgment could depreciate the true value, resulting in financial loss in terms of the owner’s investment. Therefore, the application of the ProGrid method attempts to decrease the errors and increase the accuracy of the evaluation process. In reality, ProGrid is a specialized technique from the field of Multiple Criteria Decision Analysis (MCDA) within which there is a wide range of methods (see, for example, the books by Saaty (Citation1980), Hipel (Citation1992), Roy (Citation1996), Keeney (Citation1992), Hobbs and Meier (Citation2000), Belton and Steward (2002), and Figueira, J., S. Greco, and M. Ehrgott. (Eds.), (Citation2005)). Within this paper, the ProGrid technique is utilized for the first time to address water security problems in Canada’s oil sands.
Five steps of the ProGrid methodology
Over the last decade, the ProGrid methodology has been developed to evaluate through modeling what cannot be measured by other means. Generally, the methodology is comprised of the following five steps: 1) establishing the Overarching Objectives, 2) constructing an Evaluation Matrix of multiple criteria, 3) building metrics through Language Ladders, 4) evaluating the Intangibles, and 5) marking the results on an Evaluation Grid. The first three steps are used to model intangibles; the other steps are concerned with the decision process. This section provides an explanation of the process carried out in each step.
1 Establishing the overarching objectives
In general, there are many conflicts in the world, which are often the result of two mindsets in apparent opposition and expressed through heightened tension and competition. Lowy and Hood (Citation2004) explain that tension from conflicts between two opposite objectives can give rise to higher levels of success through resolution. The processes of choosing and justifying the two key Overarching Objectives are needed as the first step in order for stakeholders to make logical decisions, and this step plays an important role in suitably evaluating performance.
2 Constructing an evaluation matrix of multiple criteria
The Evaluation Matrix is a cornerstone for evaluating intangible assets and includes important criteria for the evaluation. Creating the right criteria is one of the most critical steps in the ProGrid methodology. The methodology organizes the criteria into categories that show the real potential of objectively evaluating the intangible assets under consideration. Some criteria describe ‘inputs’ such as goals and resources that will be utilized; others illustrate ‘outputs’ such as the anticipated results. Other criteria represent ‘enablers’ such as processes and infrastructure that will allow inputs to be converted into outputs. Allocating the criteria to the appropriate columns, such as inputs, enablers, and outputs, in the Evaluation Matrix makes it easier to identify the importance of each criterion and serves as a framework for judging whether weak criteria can be modified or if they are fatally flawed. With this arrangement, the allocating process can be a practicable means to check performance as a guide for evaluation.
3 Building metrics through language ladders
The third step of the ProGrid methodology is the process that builds Language Ladders. The concept of the Language Ladder has been in use for a long time for describing intangibles. The Beaufort Scale, developed in 1806, is a good example of measuring an intangible – the wind itself. A ProGrid Language Ladder provides all evaluators with the means to describe the same thing: intensities of numerical ratings are equated with short descriptive statements. Each entry of the ladder has either a letter or number paired with a verbal definition. The entries in the scale are weighted and arranged hierarchically from weakest to strongest. The four-step ladder starts from ‘A’ and advances to ‘D’, and each step includes short sentences made up of key words in order to help users make sound evaluations. The ladder is conceptualized as a journey, with ‘A’ as the starting point and ‘D’ as the furthest point – the ultimate distance possible from ‘A’. In other words, it is an effective concept in terms of extending the scope of the ladder for achieving its goal, using the opposite of the terminology employed academically for grading. The words applied in each step of the Language Ladder must represent meaningful distinctions and be readily comprehensible by evaluators, thereby defining A, B, C, and D as in a dictionary.
Meaning of the R-values
The R-value is a percentage representing a measure of the distance of the grid point of a proposed alternative from the maximum rating possible. The R-value can be calculated mathematically to convert the qualitative assets into quantifiable values, using the criteria in the Evaluation Matrix and the Language Ladder steps (A, B, C, and D). The symbolic values such as A, B, C, and D can be defined as 0%, 33.3%, 66.7%, and 100%, respectively, although ProGrid methodology is not restricted to these values. The ratings for the criteria in the first column (input column) of the Evaluation Matrix can be assigned to the Y-axis of the grid chart, and for the third column (output column) to the X-axis, and finally for the middle column (enabler column) proportionally to both the X and Y-axes, in some cases equally. Figure presents an algorithm that shows how the ProGrid methodology works with an Evaluation Matrix and Language Ladders, and how the R-value is calculated.
The authors derived the following equations for Positions X, Y, and R-value from the ProGrid AdvisorSL software. The X and Y-axis positions of the evaluation on the grid are described as follows:
The equation for R-value (%) can be defined as:
4 Evaluating the intangibles
The next step of the ProGrid methodology is to allow the various groups of experts to evaluate the Intangibles with regard to the specific objectives and requirements of the organization. This process is performed by evaluators filling in the corresponding Evaluation Form made up of an Evaluation Matrix and Language Ladder based on an MS Excel file. Evaluators or reviewers generally assess the intangibles based on justifications and their expertise and fill in blanks on the form with their own ratings, such as A, B, C, and D, with reference to the Language Ladders. The evaluators can also fill in the reasons for their selections on short comment boxes provided. The results of ratings from all evaluators are gathered for discussion by a decision-making committee that consists of experts chosen for their wide knowledge in the corresponding field.
5 Marking the results on an evaluation Grid – output reports
The final step of the ProGrid methodology is to establish the grid with the results from the evaluation process, employing the overarching objectives as its axes. The output reports are mainly comprised of three charts: the Evaluation Grid, Evaluation Profile, and Opportunity Comparison, and one table: the Advisor Assessments, including comments.
Evaluation Grid
The Evaluation Grid provides the current grid position as evaluated by each expert and shows the evaluation average, with respect to the Overarching Objectives in the Evaluation Matrix. On this Evaluation Grid, the position Xs, the position Ys, and the R-value (%) from the results of experts’ evaluations are simply expressed, and make it easy for users to comprehend the evaluation results.
Evaluation Profile
The Evaluation Profile chart shows each rating of the performance criteria and the average rating of the evaluators with respect to all the criteria in the Evaluation Matrix. Hence, it is helpful in demonstrating strengths and weaknesses of the opportunity (alternative) and for tracking progress, using the bars to reflect the relative strength.
Opportunity Comparison
The Opportunity Comparison chart provides the X, Y grid positions of all the alternatives evaluated in a project, serving as a database. The chart shows the average rating of the evaluation results from the experts and compares the ranking of the current application (alternative) with other applications, with the overarching objectives as the axes. Having a position lower on the chart demonstrates that there are weaknesses either in both overarching objectives or in one of them that would need to be overcome. In other words, it can prioritize alternatives according to their magnitude (R-values).
Comparison of multiple criteria decision analysis techniques
The basic concept of the ProGrid methodology falls within the realm of MCDA. However, the detailed modelling and analytical techniques incorporated into specific MCDA methods can vary quite significantly. For instance, the Analytical Hierarchy Process (AHP) of Saaty (Citation1980) uses specified pair-wise comparisons of criteria and alternatives to sort and rank alternatives. Within the Electre method of Roy (Citation1996), fuzzy sets are employed to reflect uncertainty embedded in MCDA studies. Keeney (Citation1992) provides as insightful approach called value-focussed thinking for determining criteria and alternatives in as MCDA investigation. A number of insightful books and papers have been written to explain, compare, and apply a range of MCDA techniques (e.g., MacCrimmon Citation1968, Hipel Citation1992, Guitouni and Martel Citation1998, Hobbs and Meier Citation2000; and Belton and Steward 2002). More recent papers comparing MCDA techniques and deciding upon which method should be selected for applications to a particular problem include contributions by Figueira, J., S. Greco, and M. Ehrgott. (Eds.), (Citation2005), Polatidis et al. (Citation2006), and Zavadskas and Turskis (Citation2010).
The specific design of the ProGrid methodology differs from other MCDA techniques. For example, the ProGrid methodology is constructed to consider two opposite overarching objectives that are in conflict. The process of resolving the conflict between these objectives plays a critical role in achieving a higher level of success for drawing a rational solution. This concept of considering conflicting overarching objectives reflects the realities of societal-environmental problems. Therefore, the ProGrid methodology is the appropriate method for evaluating intangible assets such as water security problems in Canada’s oil sands, and this method will contribute to the making of strategic decisions. In addition, conventional evaluation methodologies such as opinion surveys collect massive amounts of information and weed out the unnecessary data progressively. As the amounts of information increase, the work of evaluation can be very difficult and may lose any meaningful focus. Compared with conventional evaluation methods, ProGrid methodology has the following advantages: 1) Comprehensive – confirms that all critical aspects have been covered, 2) Achieves consensus – encourages participation from all stakeholders, 3) Fast and reproducible – busy experts readily accept the evaluation task, 4) Graphical and easily comprehended, 5) Prioritizes values according to their magnitude, 6) Can be used as a corporate database, and 7) Concentrates on key strategic issues and decisions. In addition, the ProGrid methodology and software dramatically clarifies information by reducing it to understandable charts that emphasize the issues to be addressed for each decision. Moreover, the methodology provides an improved process for a decision environment with its openness, fairness, transparency, and accountability.
On the other hand, ProGrid itself does not make decisions. Rather, the methodology organizes various information to help make sound decisions by assisting stakeholders to carry out suitable evaluations, reviewers to take advantage of their own expertise, and decision makers to make more informed decisions. Another limitation is that the ProGrid methodology requires reviewing and updating over time through the active participation of all stakeholders.
Alternatives to water issues in Canada’s oil sands
This section describes various approaches for water resource allocation as alternatives to water problems in Canada’s oil sands. One objective of these alternatives is to allocate water reasonably to achieve optimization of the water resource, balancing environmental, social and economic aspects – but the evaluation of alternatives is difficult. In short, we need to find the best alternative that satisfies environmental and social goals with the least costs and negative impacts on economic goals. Adamowicz (Citation2007) introduced five alternatives (options) with respect to water issues in Canada’s oil sands development. As explained next, these alternatives (options) can be evaluated to assist stakeholders in making sound decisions.
Alternative 1: the “status quo”
The current water framework includes both permanent and temporary licenses related to water consumption in Alberta. A “green, yellow, red” scheme has been implemented to restrict water withdrawals according to the river flow conditions as part of the water management framework for the Athabasca River. However, the mechanisms for response to water scarcity make it difficult to apply a reasonable approach under the current policy framework: “first in time, first in right” system (the older license priority). In other words, historical property rights by date of license have priority over the value of water use. For example, if the state of the flow is judged to be in the yellow management zone (the cautionary threshold), a company that wants to obtain a license should accept the provisions for reduced water use, even though the company uses water for a higher value. In the red management zone situation, maximum withdrawal limitations, such as a restriction to an annual allocation percentage over all licensed users, will be implemented. In addition, users have little incentive for water use reduction under the current “command and control” approach unless there is a case of “yellow” or “red.” This approach does not encourage private companies to develop and employ new technologies related to water reduction. This system has the advantage of avoiding worst-case scenarios in terms of ecology, but it does not help in reducing costs related to economic activity. Hence, this approach is unfavorable for individual firms or water users and requires more costs in terms of water management compared to market-based mechanisms.
Alternative 2: tradable water rights
Tradable water rights have been implemented by various countries such as Australia, the Western United States, and Chile over the past 25 years. In recent times, southern Alberta has partially applied this approach to address water issues. Tradable water rights are a form of “cap and trade” system or market-based mechanism to protect the water environment. This system provides a strict legal and administrative framework to transfer water from low-value users to high-value users. Maximum total withdrawals are limited, and trading is only possible within the limits and without negative impacts on other users or the environment. In general, this system is approved by third parties. Tradable water rights encourage stakeholders to save water through an improved technology, so the stakeholders can have benefits through selling the rights to that amount of water. Rights trading includes both temporary and permanent trades, and it has resulted in an increase of flexibility in the trading system, leading to increased trade frequency and decreased environmental costs. Water rights trading requires the capability of enforcing and monitoring trades, so it cannot take place without administrative systems and approved basin management plans. This approach has the potential to accomplish water quantity goals with the least cost, and it may provide incentives with respect to implementing water storage. Tradable water rights can be implemented with relatively low transaction costs, with approaches to address third party effects, and with transfer flexibility for both permanent old and temporary new licenses. The key issue of the Athabasca case is establishing the maximum amount of water withdrawal, considering the seasonal water scarcities, long-term water flow variations, and environmental issues. Water rights trading is becoming more popular in various jurisdictions. However, allocating the initial rights is difficult in the oil sands area because of its rapid economic evolvement.
Alternative 3: water charges
Water pricing policy involves setting charges based on environmental and user costs with regard to water. This system can provide a standard of water resource allocation in terms of efficient water use. In addition, this approach can charge according to the type of water, such as surface water, groundwater, and saline water. Water pricing policy does not directly control water consumption the way tradable water rights do, but prices encourage water demand management such as by reducing water use and adopting technology that decreases water consumption. This methodology can apply the approach of supply management based on storage structure and storage markets. Metering and reporting of water use is important to the implementation of water pricing policy. Issues related to a water pricing approach include the responsiveness of water consumption to water charges, the cost implications for companies, and the use of water revenues. Increases in water costs bring about reduced water and substituted technologies such as recycling and recirculation. However, in general, Canada’s water costs for industry occupy a small portion of overall costs, and these modest water prices have little impact on overall costs. Hence, achieving a successful pricing approach requires information such as the impacts of pricing strategies and the potential for technical change. The establishment of the price levels and the use of the water revenues are also very critical issues in determining prices. The possibility for recycling, water substitution (among surface water, groundwater, and saline water), substitution of other materials, and process innovations are significant factors in evaluating the water pricing. The Refunded Emissions Payments (REP) scheme can be useful to resolve the issue of the use of the revenues from water charges. This scheme charges industry an excessive price per unit of emissions, but refunds a large portion of the revenue to the industry based on the output of the industry. The large charges can cause the industry to reduce emissions and to develop technology. However, if the amount of the charges is relatively low, the impacts are minor. This scheme is similar to a tradable permits scheme in terms of making reference to historical output levels, but it does not require many of the transaction costs compared to tradable permits schemes. The REP scheme has the potential to be an effective means of regulating the water charges in the oil sands area if it is employed suitably. The costs of monitoring and enforcements can be relatively low because water consumption and output are monitored in the oil sands region at present.
Alternative 4: performance standards and tradable performance standards
Performance standards or targets can be used to encourage firms to decrease water use. For instance, a target for the number of barrels of water used to produce a barrel of oil can be developed for the industrial sector at a level lower than the current industry average with disclosure on progress towards this target. The oil sands industry can voluntarily make an effort to accomplish these targets by setting technology-based standards, by supporting technology-based subsidies, and by employing differential incentive-based mechanisms. In the case of tradable performance, the desired emissions per unit output are established as a target. When a firm achieves a lower emissions intensity than this objective, the firm can sell some permits up to the target point. On the other hand, if a firm does not achieve the objective, the firm must buy permits to decrease their intensity to the target. This scheme is very similar to the tradable water rights and the REP scheme. Tradable water rights are a function of the “cap” and maximum water allocation, and the approach maximizes water use efficiency within the cap. The REP scheme is dependent upon water charges, and the procedure shows the possibility of efficient water use. However, in the tradable performance standards, the key characteristic is the target water use per unit output, and supporting regulations are required to limit water consumption to be within the cap with water charges.
Alternative 5: water storage
In order to deal with insufficient water during winter in the Athabasca Basin, a water storage approach can be one mechanism to satisfy winter flow needs with the construction of off-stream storage. This method is a feasible alternative and a practical solution to address low winter flows in the Athabasca River. However, the implementation cost has a potential to be significant. Different water charges between high-flow periods and low-flow periods can create an incentive to conserve water and to shift water withdrawals as a reasonable water strategy.
Application of the ProGrid methodology to Canada’s oil sands’ water problems
Modeling: water security problems in Canada’s oil sands
In order to address the water quantity and quality problems in Canada’s oil sands, the ProGrid methodology can be utilized as a reasonable decision-assisting tool because these issues are qualitative and require an in-depth analysis based on multiple-criteria.
Overarching objectives for water issues in Canada’s oil sands
The process of choosing the two key overarching objectives is the first step in ProGrid methodology. Through many considerations, the following two overarching objectives are selected:
• | Water Security | ||||
• | Balancing Environmental, Social, and Economic Impacts |
Evaluation Matrix of multiple criteria for the oil sands’ water issues
The second step in the method is to establish multiple-criteria for the evaluation through an Evaluation Matrix, as displayed in Table . This matrix was created to include all of the key criteria that would be important in addressing water security problems in Canada’s oil sands.
Table 1. Evaluation matrix for water issues.
Language ladders for the oil sands’ water issues
The next step is to build the Language Ladders for all the criteria. Every Language Ladder for the criteria includes short sentences composed of key words to help users make rational evaluations. The Ladders are readily comprehensible by evaluators, defining A, B, C, and D as in a dictionary. An example of the Language Ladder for Technology, one of the criteria, is illustrated in Table .
Table 2. Language Ladder for technology.
Evaluating water security problems in Canada’s oil sands
Five members of the Conflict Analysis Group at the University of Waterloo participated in the evaluation process to assess the alternatives in terms of the two Overarching Objectives. One of authors of this paper also completed an evaluation.
Output reports of Canada’s oil sands’ water security problems
All the output reports for each alternative are created in the form of the Evaluation Grid, the Evaluation Profile, and the Opportunity Comparison, presenting the results as the evaluators’ opinions and their averages. In particular, the Opportunity Comparison report summarizes the evaluation of all the alternatives.
Evaluation grid of the “status quo”
According to five alternatives, five Evaluation Grids are generated with each evaluator’s grid position and the average of the evaluations, with regard to the overarching objectives. In addition, the positions X and Y, and the R-value (%) from the average of the evaluation results are expressed as numbers. An Evaluation Grid of Alternative 1 (the “Status Quo”), one of the five Alternatives, is presented in Figure .
Through this Evaluation Grid chart, one can interpret the evaluation results about the “Status Quo” alternative as follows:
• | The average of the evaluators’ opinions is positioned in the “Consider” area. | ||||
• | The position (4.0, 4.9) is the average of the evaluation results. | ||||
• | The R-value is given as 44%, describing the progress on the X (Balancing Impacts) and Y (Water Security) axes. |
In summary, the “Status Quo” is positioned in the “Consider” area and, thus, the option has many issues that must be overcome in order to be the optimum alternative for water issues in Canada’s oil sands. The following report shows stakeholders the way the “Status Quo” can be appropriately improved for reaching the best alternative.
Evaluation profile of the “status quo”
In the same manner as the Evaluation Grid, five Evaluation Profiles are created. They describe the average rating of the evaluators with regard to all the criteria in the Evaluation Matrix, as displayed in Figure .
Figure shows the criteria’s relative strength and weakness with respect to the “Status Quo” alternative. The chart can be explained as follows:
• | The relative strength is: Water Quantity > Environmental Impact > Social Impact > Water Quality > Policy > Technology > Economic Impact | ||||
• | The “Status Quo” alternative is strong in terms of water quantity. | ||||
• | The economic impact related to water use efficiency and effectiveness must be considered as the first priority for improving the alternative. | ||||
• | Technology and policy are also carefully considered for enhancing the alternative. |
Opportunity comparison – database results
Figure provides the X, Y grid positions of the five alternatives evaluated in this study. This grid shows the results with respect to alternatives through the evaluation process, employing the overarching objectives as its axes.
With this representative result, the stakeholders can compare the evaluation results of all the alternatives and interpret them as follows:
• | Two alternatives (Tradable Water Rights and Water Charges) are located in the “Target” district, and three alternatives (Performance Standards, the “Status Quo”, Water Storage) are located in the “Consider” area. | ||||
• | The priority is as follows: Tradable Water Rights (R-value: 79%) > Water Charges (69%) >> Performance Standards and Tradable Performance Standards (56%) > the “Status Quo” (44%) > Water Storage (43%) | ||||
• | Each alternative has its R-value and the R-values are employed to rank the alternatives. |
Conclusions
The authors analyze water security problems in Canada’s oil sands for the first time using the ProGrid methodology with respect to five key alternatives considered for resolving the problems of water scarcity and associated impacts. The ProGrid methodology dramatically clarifies outstanding issues and information regarding water quantity and quality problems in Canada’s oil sands by reducing them to understandable charts that highlight the matters to be addressed. Furthermore, the methodology takes advantage of graphical presentations, thus, stakeholders can understand the comprehensive output reports quickly and easily.
The application of the ProGrid methodology to water security problems in Canada’s oil sands illustrates how alternatives can be evaluated strategically. In addition, the implementation of the ProGrid software prioritizes all the alternatives by using the R-value, and this approach interconnects with the goal of the methodology, thereby converting qualitative intangible assets such as the effectiveness of government policy into quantifiable measures. Evaluating the options objectively is a great contribution to assisting decision makers in making informed decisions.
Through the application of the ProGrid methodology and evaluations, two alternatives are recommended: Tradable Water Rights (R-value: 79%) and Water Charges (R-value: 69%). These two alternatives provide an integrated approach to achieving water security as well as the environmental, economic, and social goals in terms of water issues in Canada’s oil sands. The authors have concentrated more on water quantity. However, in terms of water quality, one can compare which alternative is better than others using the Evaluation Profile outcomes, for which detailed results are provided by Kim (Citation2010). Therefore, the findings of this paper should be useful for addressing water security problems, considering balancing environmental, economic, and social impacts.
Acknowledgements
The authors wish to express their sincere appreciation to Michele Heng, Motahareh Armin, Abul Bashar, and Qian Wang for completing the evaluation forms. They are also grateful to three anonymous referees for providing suggestions that enhanced the quality of their paper.
References
- Adamowicz , V. 2007 . “ Section 2: Water use and Alberta oil sands development – science and solutions: An analysis of options ” . In Running out of steam?: Oil sands development and water use in the Athabasca River-Watershed: Science and market based solutions , Edited by: Davison , D. J. and Hurley , A. M. 40 – 58 . Edmonton, Alberta : University of Alberta .
- Alberta Environment . 2007 . The water management framework: For the industrial heartland and capital region , Edmonton, Alberta : Alberta Environment .
- Allen , E. W. 2008 . Process water treatment in Canada’s oil sands industry: II. A review of emerging technologies . Journal of Environmental Engineering and Science , 7 : 499 – 524 .
- Belton , V. and Stewart , T. 2002 . Multiple criteria decision analysis: An integrated approach , Massachusetts : Kluwer Academic Publishers .
- Bowman , C. W. 2005 . Intangibles: Exploring the full depth of issues , Sarnia , ON : Grafiks Marketing & Communications .
- Bowman, C. W., and B. Griesbach. 2007. Energy Pathways Task Force Phase 1 – Final Report. Ottawa, ON: Canadian Academy of Engineering (CAE).
- BP. 2009. BP Statistical review of world energy: June 2009. London.
- Canadian Association of Petroleum Producers (CAPP) . 2008 . Environmental challenges and progress in Canada’s oil sands , Calgary, Alberta : CAPP .
- Elshorbagy , A. , Jutla , A. , Barbour , L. and Kells , J. 2005 . System dynamics approach to assess the sustainability of reclamation of disturbed watersheds . Canadian Journal of Civil Engineering , 32 : 144 – 158 .
- Energy Type. 2009. API gravity: Definition, conversion, calculation and table. Accessed July 2010. http://www.energytype.com/2009/11/api-gravity-definition-conversion.html
- Energy Resources Conservation Board (ERCB) . 2009 . ST98-2009: Alberta’s energy eeserves 2008 and supply/demand outlook 2008–2018 , Calgary, Alberta : ERCB .
- Figueira, J., S. Greco, and M. Ehrgott. (Eds.). 2005. Multiple criteria decision analysis: State of the art surveys. Boston: Springer Science + Business Media.
- Gosselin, P., S. E. Hrudey, M. A. Naeth, A. Plourde, R. Therrien, G. V. Kraak, and Z. Xu. 2010. Environmental and health impacts of Canada’s oil sands industry. The Royal Society of Canada Expert Panel. Ottawa, ON: The Royal Society of Canada.
- Government of Alberta . 2009 . Energy economics: Understanding royalties , Edmonton, Alberta : Government of Alberta .
- Guitouni , A. and Martel , J. M. 1998 . Tentative guidelines to help choosing an appropriate MCDA method . European Journal of Operational Research , 109 : 501 – 521 .
- Hipel , K. W. 1992 . Multiple objective decision making in water resources . Journal of the American Water Resources Association , 28 ( 1 ) : 3 – 12 .
- Hipel, K. W., and C. W. Bowman. 2011. “Is our energy superpower vision slipping away?” Toronto Star, March 16.
- Hipel, K. W., A. D. Miall, and D. W. Smith. 2011. Water resources in Canada: A strategic viewpoint. Report to the Inter American National Academies of Science (IANAS), unpublished manuscript, 21–53.
- Hobbs , B. and Meier , P. 2000 . Energy decisions and the environment: A guide to the use of multicriteria methods , Dordrecht, The Netherlands : Kluwer Academic .
- Humphries , M. 2008 . North American oil sands: History of development, prospects for the future , Washington , DC : Congressional Research Service .
- Keeney , R. L. 1992 . Value-focused thinking: A path to creative decisionmaking , Cambridge : Harvard University Press .
- Kelly , E. N. , Schindler , D. W. , Hodson , P. V. , Short , J. W. , Radmanovich , R. and Nielsen , C. C. 2010 . Oil sands development contributes elements toxic at low concentrations to the Athabasca River and its tributaries . Proceeding of the National Academy of Sciences (PNAS) , 107 ( 37 ) : 16178 – 16183 .
- Kim , Y. J. 2010 . Canada’s oil sands: Strategic decisions to make Canada an energy superpower , Waterloo , ON : University of Waterloo .
- Kim, Y. J. and K. W. Hipel. 2010. “Water quantity and quality problems in Canada's oil sands.” In Proceedings of Water 2010: Hydrology, Hydraulics and Water Resources in an Uncertain Environment. Quebec City, Quebec, July 5 -7.
- Lowy , A. and Hood , P. 2004 . The power of the 2 × 2 matrix , New York : Jossess-Bass .
- MacCrimmon , K. R. 1968 . Decision making among multiple-attribute alternatives: A survey and consolidated approach, RAND memorandum, RM-4823-ARPA , Santa Monica, California : The Rand Corporation . 72
- Mannix , A. E. , Dridi , C. and Adamowicz , W. L. 2010 . Water availability in the oil sands under projections of increasing demands and a changing climate: An assessment of the Lower Athabasca water management framework (Phase 1) . Canadian Water Resources Journal , 35 ( 1 ) : 29 – 52 .
- National Energy Board (NEB) . 2004 . Canada’s oil sands: Opportunities and challenges to 2015. An energy market assessment , Calgary Alberta : NEB .
- National Energy Board (NEB) . 2006 . Canada’s oil sands: Opportunities and challenges to 2015. An energy market assessment , Calgary, Alberta : NEB .
- Nikiforuk , A. 2008 . Tar sands: Dirty oil and the future of a continent , Vancouver , BC : Greystone Books .
- Polatidis , H. , Haralambopoulos , D. A. , Munda , G. and Vreeker , R. 2006 . Selecting an appropriate multi-criteria decision analysis technique for renewable energy planning . Energy Sources, Part B , 1 : 181 – 193 .
- Prebble, P., A. Coxworth, T. Simieritsch, S. Dyer, M. Huot, and H. Walsh. 2009. Carbon copy: Preventing oil sands fever in Saskatchewan. Oil Sands Fever Series. 14–20. Drayton Valley, Alberta: Pembina Institute, Saskatchewan Environmental Society, Canadian Parks and Wilderness Society.
- Radke , D. 2006 . Investing in our future: Responding to the rapid growth of oil sands development , Edmonton, Alberta : Government of Alberta .
- Richardson, L. 2007. “The oil sands: Toward sustainable development”. In Report of the Standing Committee on Natural Resources, 39th Parliament, 1st session. 34–47. Ottawa, ON: House of Commons.
- Roy , B. 1996 . Multicriteria methodology for decision aiding , Dordrecht, The Netherlands : Kluwer Academic Publishers .
- Saaty , T. 1980 . The analytic hierarchy process: Planning, priority setting, resource allocation , New York : McGraw-Hill International Book Co .
- Schindler , D. W. , Donahue , W. F. and Thompson , J. P. 2007 . “ Section 1: Future water flows and human withdrawals in the Athabasca River ” . In Running out of steam?: Oil sands development and water use in the Athabasca River-Watershed: Science and market based solutions , Edited by: Davison , D. J. and Hurley , A. M. 1 – 39 . Edmonton, Alberta : University of Alberta .
- The Globe and Mail. 2010. Ottawa vows oilsands monitoring. Accessed February 2011. http://www.theglobeandmail.com/report-on-business/video/ottawa-vows-oilsands-monitoring/article1846789/
- US Energy Information Administration. 2010. International petroleum (oil) reserves and resources tables and reports: Most recent estimates, based on information from the Oil & Gas Journal – January 2009. Accessed July 2010. http://www.eia.doe.gov/international/oilreserves.html
- World Wildlife Fund . 2005 . Implications of a 2 degrees global temperature rise on World Wildlife Canada’s water resource , Gland, Switzerland : World Wildlife Fund .
- Woynillowicz , D. , Severson-Baker , C. and Raynolds , M. 2005 . Oil sands fever: The environmental implications of Canada’s oil sands rush , Alberta : Pembina Institute .
- Woynillowicz, D., and C. Severson-Baker. 2006. Down to the last drop: The Athabasca River and the oil sands. Oil Sands Issue Paper No. 1. Calgary, Alberta: Pembina Institute.
- Zavadskas , E. K. and Turskis , Z. 2010 . A new additive ratio assessment (ARAS) method in multicriteria decision-making . Technological and Economic Development of Economy , 16 ( 2 ) : 159 – 172 .