Abstract
Our aim was to develop protective physical and biological thresholds that identify the upper limit of the least disturbed (reference) condition for total suspended solids (TSS). The study focuses on streams in agricultural regions across Canada from which we collected contemporary TSS and benthic invertebrate data, and compiled long-term TSS data sets. Reference conditions for TSS were analyzed by five approaches previously applied in Canada, Australia and New Zealand, and the USA. In addition, ecological reference conditions for TSS were developed using regression-tree analysis to determine change-points along TSS gradients for three invertebrate metrics (percentage Ephemeroptera-Plecoptera-Trichoptera, total richness, and modified family biotic index). Increased agricultural land-cover was linked to higher TSS in most agricultural regions which, in turn, was associated with degraded environmental conditions and a reduction in the relative abundance of pollution-intolerant taxa. The five physical approaches produced a narrow range of regional TSS reference condition values (2.7 to 6.2 mg L–1). Ecological reference conditions for TSS ranged from 3.5 to 11.1 mg L–1 across Canada. We conclude that long-term TSS databases may serve to generate interim sediment criteria because ecological reference conditions were similar to those calculated from physical data alone. These interim sediment criteria may be appropriate targets for use in evaluation of restoration programs designed to improve environmental quality of aquatic ecosystems (e.g., beneficial or best management practice applications).
Notre objectif était d’établir des seuils de protection physiques et biologiques correspondant à la limite supérieure de l’état de perturbation minimale (référence) pour le TSS (total suspended solids). Nous avons donc axé notre étude sur les cours d’eau des régions agricoles du Canada, où nous avons recueilli des données contemporaines sur le TSS et les invertébrés benthiques, et compilé des ensembles de données sur l’évolution du TSS à long terme. Pour analyser les conditions de référence, nous avons eu recours à cinq méthodes ayant déjà été utilisées au Canada, en Australie et en Nouvelle-Zélande, et aux états-Unis. Les conditions écologiques de référence pour le TSS ont été établies à l’aide d’une analyse par arbre de régression qui a permis de déterminer les points de modification des gradients de TSS pour trois paramètres liés aux invertébrés (proportion éphéméroptère-plécoptère-trichoptère, richesse totale et indice biotique de Hilsenhoff modifié). Une occupation accrue des terres agricoles a été associée à un TSS plus élevé dans la plupart des zones agricoles, lequel est corrélé avec une dégradation des conditions environnementales et une réduction de l’abondance relative des taxons intolérants à la pollution. Les valeurs des conditions de référence pour le TSS obtenues dans les différentes régions à l’aide des cinq méthodes physiques se situent toutes à l’intérieur d’un intervalle étroit (2,7 à 6,2 mg L–1). Les conditions écologiques de référence pour le TSS dans l’ensemble du Canada varient de 3,5 à 11,1 mg L–1. Nous en avons conclu que les bases de données sur l’évolution du TSS à long terme pourraient être utilisées pour établir des critères provisoires en matière de sédiments étant donné que les conditions écologiques de référence étaient similaires à celles calculées à partir des seules données physiques. Ces critères de sédiments provisoires pourraient constituer des cibles appropriées pour l’évaluation des programmes de remise en état destinés à améliorer la qualité de l’environnement des écosystèmes aquatiques (p. ex. adoption de pratiques de gestion bénéfiques ou exemplaires).
Introduction
Sediment inputs derived from anthropogenic land-disturbing activities are a pervasive threat to stream ecosystems in North America and elsewhere (Canadian Council of Ministers of the Environment (CCME) 2002; United States Environmental Protection Agency (US EPA) Citation2006; Larsen and Ormerod Citation2010). Of the many land-disturbing activities contributing to increased sediment inputs, agriculture is a common source of sediments in the USA and Canada (Waters Citation1995; US EPA Citation2002; Culp et al. Citation2009). Agricultural activities can cause soil exposure and compaction resulting in elevated rates of erosion from cultivated fields, riparian zones, and stream banks. When deposited, the effects of excess sediment include smothering of periphyton, mosses, invertebrates (notably mussels), vertebrate (fish and amphibian) eggs and larvae, and fouling of respiratory surfaces of invertebrates and vertebrates (see reviews by Waters Citation1995; Henley et al. Citation2000). Suspended sediments are also thought to affect biological structure and function in rivers: increased suspended sediment concentration can impair water quality, leading to increased mortality (Newcombe and MacDonald Citation1991) and reduced growth of fishes (Shaw and Richardson Citation2001). Exposure to elevated levels of suspended sediments can also increase invertebrate drift rates (Culp et al. Citation1986; Fairchild et al. Citation1987) and decrease density and biomass of benthic macroinvertebrates (Waters Citation1995). Despite these known effects of excessive sedimentation, there is little consensus on an approach to identify reference conditions that, if adopted into policy or regulation, would protect surface waters from impairment associated with increased suspended sediment concentrations (Cormier et al. Citation2008; Culp et al. Citation2009). Here we focus on an approach for setting reference conditions that are intended to protect stream communities from environmental degradation as measured by increased TSS (total suspended solids).
A particular challenge in deriving reference conditions is that suspended particles are a natural feature of lotic ecosystems with TSS concentration associated with hydrological, geological, topographical, and soil related conditions of a catchment. Although suspended sediments can be contaminated (e.g., nutrients, pesticides, metals), we focus exclusively on the development of reference conditions that identify the upper limit of the least disturbed condition (LDC, Stoddard et al. Citation2006) for TSS that protects aquatic life from the destructive physical consequences of inorganic sediments. The LDC describes sites subjected to the least amount of ambient human disturbance in the region. Numerous approaches have been used for deriving reference conditions to protect environmental health (see Cormier et al. Citation2008 for overview); however, in the case of non-toxic stressors, such as TSS and turbidity, numeric water quality criteria are most often based on natural background values (Brua et al. Citation2006). Because reference sites are currently few and historical data from sites that would have been considered reference at the time are typically lacking, various statistical methods have been applied to demark the threshold signifying a change from least disturbed to impaired conditions. A common approach in determining the reference condition is to identify a particular percentile of the data as the threshold between background and impaired conditions; these include the United States Environmental Protection Agency percentile approaches (US EPA Citation2000) and the Australian and New Zealand Environment and Conservation Council and Agriculture and Resource Management Council approach (ANZECC and ARMCANZ Citation2000a; Citation2000b; Citation2000c). Other statistical approaches for reference condition identification include the Y-intercept method (Dodds and Oakes Citation2004), CCME (Citation2002) approach, and regression-tree analysis methodology (Breiman et al. Citation1984). As there is no consensus on methods for reference condition development, Cormier et al. (Citation2008) applied several methods and used the mean of various thresholds as the criteria.
Our primary aim was to develop a widely applicable approach to establish reference conditions for TSS that, if adopted, would protect aquatic life from the adverse effects of elevated TSS, with particular emphasis on agricultural catchments. Key objectives included: (1) evaluating specific approaches for setting sediment reference conditions that have been applied in Canada, Australia and New Zealand, and the USA; (2) applying a subset of these approaches to develop TSS reference conditions for agricultural regions in Canada; (3) examining relationships between benthic macroinvertebrate indicators and TSS, and (4) comparing TSS reference conditions developed from physical or ecological data. By comparing our physically-based and biologically-derived reference conditions, we then recommend an approach for setting interim TSS criteria for the major agricultural regions of Canada. Development of these TSS reference conditions occurred under Canada’s National Agri-Environmental Standards Initiative (NAESI), which required new sediment thresholds as Canada’s existing sediment guidelines applied largely to assessment of episodic, point-source sediment disturbances (CCME Citation2002). While the developed approach is useful for identifying reference conditions for TSS in agricultural watersheds and has important application for the protection of aquatic life, the approach is not intended to provide detailed information on sediment delivery and transport processes. Finally, we note that companion studies address the effects of deposited sediments (Benoy et al. Citation2012) and nutrients (Chambers et al. Citation2012) on reference and ecological conditions of these agricultural catchments.
Methods
Study areas
Study areas were located in most major agricultural regions across Canada (Figure and Table ). Sampling stations in British Columbia were located throughout the Okanagan Basin in the Montane Cordillera ecozone. Livestock production, grain and oilseed crops were the dominant agriculture within this geographic region. Stations in the Western Prairies were located primarily in central and southern Alberta in the Prairies ecozone, with the predominant agricultural systems being grain, oilseed crops and livestock production. Stations in the Mixedwood Plains ecozone of southwestern Ontario were dominated by corn and soybean production. Dairy farming was a dominant form of agriculture in the Chaudière-Appalaches region of the Quebec portion of the Mixedwood Plains ecozone. In the Grand Falls region of northwestern New Brunswick and central Prince Edward Island, both part of the Atlantic Maritime ecozone, potato production was the dominant form of agriculture. Agricultural land use for each of the study regions was extracted from the most recent Canadian Census of Agriculture (Eilers et al. 2010). Ecozones were identified as outlined by the Ecological Stratification Working Group (Citation1995).
Figure 1 Locations of long-term and contemporary monitoring stations used to derive total suspended solids reference conditions across (A) Canada, with the focal geographical regions indicated as (1) Okanagan Basin and Western Prairies, (2) southwestern Ontario, and (3) Chaudière-Appalaches, Grand Falls and Prince Edward Island. In addition, panels B, C, and D provide map enlargements of the three focal geographical regions. Agricultural land cover is indicated in gray.
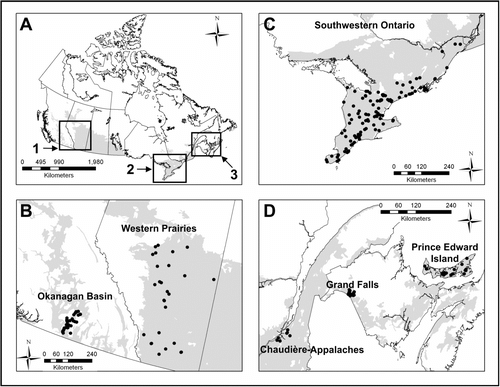
Table 1. Descriptive characteristics of monitoring stations (long-term, L, and contemporary, C) used in analyses to derive total suspended solid thresholds.
Data sources
Two sources of data were used for the development of physical TSS reference conditions: long-term monitoring data from numerous jurisdictional sources and contemporary TSS and biological data collected from five study areas. All TSS samples (long-term and contemporary) were analyzed as described using the methods below. For both data sets, stations were screened and only included if they had: (1) continuous flow when samples were collected; (2) 2 yr of data collected during the open water period and with
1 sample per month; (3) catchment size ⩽ 165 km2 for stations in southwestern Ontario, Chaudière-Appalaches, the Grand Falls region and Prince Edward Island, or < 500 km2 and < 700 km2 in the Western Prairies and Okanagan Basin, respectively, with all catchments being
3rd order; and (4) < 10% urban land cover within the catchment. Differences in
3rd order catchment area among regions were the result of more arid western regions having larger catchments due to lower annual precipitation than eastern catchments. To assess relationships between TSS and land cover or biotic impairment, a gradient in agricultural land cover was established in each geographic region (Table ).
Land cover analysis
Because this national study required a minimum standard for spatial land use and topographical data, we used the National Topographic Database (NTDB), which is a complete, uniform product comprised of 1:50000 digital vector data for all of Canada (Natural Resources Canada Citation2006). The delineation of catchment boundaries (i.e., the upstream contributing area) for each sampling location was accomplished through a combination of automated and manual boundary interpolation. Automated delineation was used to establish rough boundaries where Digital Elevation Model (DEM) data of sufficient detail were available. Once approximate boundaries were established, NTDB contour data were used to refine the boundary based on visual inspection and interpolation. In areas where DEM data of sufficient detail were not available, boundaries were developed using manual interpolation from NTDB contours alone. Where there was more than one station on a stream, landscape data were calculated for each catchment unit separately.
Agricultural land cover was defined as any area within the catchment upstream of the sampling location that was considered cropland or pasture/rangeland (Culp et al. Citation2008 Appendix A). We could not further compartmentalize agricultural land cover because detailed information on cropland versus rangeland was not available for all locations. Land cover data from NTDB (GeoGratis – Collection metadata) was then clipped using the delineated catchment boundaries. Because few true reference catchments exist within the agricultural region of Canada, catchments with < 25% agricultural land cover within the catchment were classified as LDC (Stoddard et al. Citation2006). These LDC catchments were considered to have limited agricultural impact as TSS values were similar at lower amounts of agriculture within the catchments.
Field and laboratory methods
For our contemporary sampling program, each station was monitored monthly for TSS from fall 2005 through all of 2007, except in the Grand Falls region and Prince Edward Island where sampling ceased at the end of 2006. This sampling frequency represents the common collection regime used in Canada and elsewhere. On each visit, water samples from wadeable streams were collected from the middle of the channel at mid-water depth for subsequent analysis of TSS. For all sampling stations (contemporary and long-term), TSS was determined by filtering a known volume of a water sample through a GF/C filter with nominal pore size of 1.2 μm, drying the residue retained on the filter to constant mass, and then weighing the mass (1 mg L–1 minimum detection limit) of sediments on the filter (Environment Canada Citation2010).
Benthic macroinvertebrates were collected for determination of taxonomic composition in late summer or fall (August–November) at each station in the Okanagan Basin, southwestern Ontario and in the Grand Falls region in 2005 and 2006. Following the protocol of the Canadian Aquatic Biomonitoring Network (CABIN) (Reynoldson et al. Citation1997), samples were collected with a kick net (400 μm mesh) by disturbing the streambed substrate for 3 min with the collector moving upstream in a zigzag pattern for approximately 10–15 m. Samples were emptied into a bucket and the organic material was separated from gravel and cobble by swirling the sample in water and pouring the suspended material into a 400-μm mesh sieve. The material retained on the sieve was preserved in 10% formalin. Samples were subdivided in the laboratory using a Marchant Box (Marchant Citation1989; Reynoldson et al. Citation2006) and a minimum of 300 individuals were removed for counting and identification to the lowest practical taxonomic unit (normally genus). These data were used to determine three, a priori selected, benthic macroinvertebrate indices or metrics commonly applied in river bioassessment: Ephemeroptera-Plecoptera-Trichoptera (EPT) relative abundance, total taxonomic richness and the Modified Family Biotic Index (MFBI; Hilsenhoff Citation1988).
Data analysis
Physical reference condition
Y-intercept
Dodds and Oakes (Citation2004) developed the y-intercept method to calculate reference conditions of catchments when few or no reference streams exist. To perform this technique, a mean of all TSS data for each sampling station is calculated. The log-transformed TSS mean for each station is then regressed against land use data within the catchment for each stream. The y-intercept from the regression model is the calculated TSS reference condition in this procedure.
US EPA percentiles
This US EPA method calculates a median value from the seasonal 25th percentile values derived from all streams in a geographical region (US EPA 2000). Seasons for southwestern Ontario, Chaudière-Appalaches, Grand Falls region and Prince Edward Island were classified as: Spring – April through May, Summer – June through August, Fall – September through October, and Winter – November through March. Seasons for the Okanagan Basin and Western Prairies were classified as: Spring – March through May, Summer – June through August, Fall – September through November, and Winter – December through February. The 25th percentile of TSS for all rivers in the geographic region was determined for each season. The median value from the four seasonal 25th percentile values (see Fig 4b in US EPA 2000) is established as the physical reference condition.
ANZECC and ARMCANZ percentile
To calculate the reference condition following the ANZECC and ARMCANZ method (Citation2000a; Citation2000b; Citation2000c), a minimum of 2 yr of data from LDC catchments is required to determine the 80th percentile of TSS. However, in our study, available data from LDC catchments used to calculate the 80th percentile covered a range of 2 to 14 yr (Table ). This reference condition value was determined for high flow periods, which were defined as Spring and Summer seasons for each geographical region as this is the period of peak flow resulting from snow melt and summer precipitation (Peters et al. Citation2012).
CCME guideline
The CCME approach differs from that of the other percentile approaches in that it is a narrative standard without a clear statistical method provided for determining the physical TSS reference condition. However, the most recent guideline narrative indicates that the value is based on background levels during clear flow periods (CCME Citation2002). To establish a physical TSS reference condition value for our data sets, all TSS data from LDC catchments were plotted for all years and the CCME reference condition was defined as the average low flow concentration. Clear flow periods were defined as any period of time when TSS concentration returned to normal background levels.
Regression-tree analysis
To determine physical TSS reference conditions, we compared log-transformed mean station TSS values with percent total agricultural land cover within the catchment. We then applied regression-tree analysis to determine change-points (P ⩽ 0.05) in agricultural land cover corresponding with the greatest response in TSS (Breiman et al. Citation1984). This approach maximizes the between-group deviance partitioning of the independent variable into two groups that have significant differences in means above and below the change-point. Regression-tree analysis calculates a fit value, which is a measure of goodness of fit, and a proportional reduction in error (PRE) value, which is similar to an R2 value. The median value of TSS of the stations below the percent total agricultural change-point is the physical TSS reference condition. The use of the median value below the change-point, rather than the change-point value, produces a more conservative reference condition because the change-point identifies the state where a significant change in sediment concentration has already occurred (Chambers et al. Citation2012).
Ecological reference condition
Ecological reference conditions for TSS were determined using regression-tree analysis to compare specific biotic metrics and indices (i.e., EPT relative abundance, total taxonomic richness, and MFBI) with mean station TSS values. Using the same procedure as outlined above for physical TSS thresholds, we applied regression-tree analysis to determine change-points in TSS values at which biotic metrics showed the greatest response (US EPA Citation2010). The change-point in this instance is the value calculated for the TSS reference condition. Only one dependent variable (biotic metric or index score) was tested at a time versus the independent variable TSS. In addition, we assessed the association between agricultural land cover and invertebrate metrics using Spearman rank correlation with P < 0.10 to reduce the likelihood of committing a Type I error.
Results
Long-term and contemporary data for TSS were obtained from a total of 280 stations across Canada. TSS values showed considerable regional variability, with mean station TSS exceeding 40 mg L–1 in the Western Prairies compared to less than 15 mg L–1 in the remaining regions (Table ). Similarly, median TSS concentration was highest in the Western Prairies at 10 mg L–1, while median TSS values ranged from 3 to 7 mg L–1 in the remaining geographical regions (Table ).
Table 2. Descriptive statistics of total suspended solids (TSS, mg L–1) for all data used to establish physical and ecological reference conditions. Statistics were calculated from all samples.
Comparison of slopes and intercepts of TSS data relative to percent total agricultural land cover within catchments revealed that the regional data sets could not be combined into a single analysis to derive a single, multi-regional TSS reference condition. Analysis of covariance showed a positive relationship between TSS and percent agricultural land cover within the catchment, but the slopes of TSS versus percent total agriculture were significantly different among regions (F = 8.51 df = 6,248; P < 0.0001; Figure ).
Physical TSS reference conditions
Reference condition for TSS calculated for six geographical regions using five different methodologies resulted in values that were relatively similar within and among techniques (Table ). Relative to other methods, the CCME approach tended to produce the lowest values while the highest TSS values were usually generated by the ANZECC and ARMCANZ and regression-tree methods. Furthermore, reference condition values from the y-intercept and US EPA 25th percentile approaches tended to be either more conservative or between those of the other three techniques. Considering all of the calculated values, the highest physical values of 11.0 and 11.5 mg L–1 TSS, determined using the ANZECC and ARMCANZ approach, were found in the Okanagan Basin and Western Prairies, while intermediate values were found in the Grand Falls region and the lowest values were determined for southwestern Ontario, Chaudière-Appalaches and Prince Edward Island (Table ). Because the y-intercept and regression-tree analyses assume an association between TSS and percent agriculture, we did not use these approaches to calculate reference conditions for the Western Prairies and Prince Edward Island
Figure 2 Comparison of the relationship between mean total suspended solids (log10 TSS mg L–1) and percent total agricultural land cover within a catchment for long-term and contemporary monitoring data combined. OB and medium dashed line represent Okanagan Basin, SO and solid line for southwestern Ontario, CA and long dashed line for Chaudière-Appalaches, and GF and short dashed line for Grand Falls region.
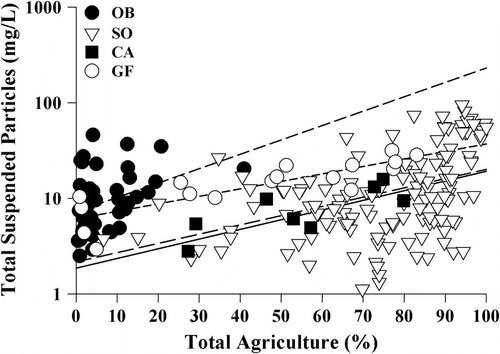
Table 3. Total suspended solids (TSS, mg L–1) reference conditions used to delimit change from least disturbed conditions. TSS reference conditions listed by geographical region as determined by five different methods of calculating reference condition values.
Ecological TSS reference conditions
In general, EPT relative abundance and total taxonomic richness were negatively correlated with increasing agricultural land cover, whereas the MFBI (which increases with greater number and proportion of tolerant taxa) was positively correlated with percent agricultural land cover within the catchment (Table a). All invertebrate endpoints were correlated with percent total agriculture in the Okanagan Basin and Grand Falls region, whereas MFBI was the only metric correlated with percentage total agriculture in southwestern Ontario and only in 2006.
Table 4. Spearman rank correlations (r) between benthic macroinvertebrate metrics by geographical region and year for (a) percent agricultural land cover within a catchment, and (b) mean station total suspended solids. Values in bold are significant at P ⩽ 0.10.
Correlations of EPT relative abundance, total taxonomic richness and the MFBI with TSS concentrations varied among regions (Table b). For example, EPT relative abundance and total taxonomic richness were negatively correlated with TSS in two of three geographic regions in 2005 and one of three regions in 2006. Total taxonomic richness and TSS were only significantly correlated in the Grand Falls regions. MFBI showed a significant, positive correlation with TSS in one of three regions in 2005 and two of three geographic regions in 2006.
Ecological reference conditions for TSS identified by regression-tree analysis resulted in values that were relatively similar amongst the three biotic metrics and between years for both the Okanagan Basin and southwestern Ontario. In the Okanagan Basin, ecological reference condition values ranged from 4.3 to 7.9 mg L–1 TSS for all three metrics in both years (Table , Figure ). Similarly, values for southwestern Ontario ranged from 2.6 mg L–1 for % EPT and MFBI to 5.5 mg L–1 for total taxa richness (Table , Figure ). In contrast, values for the Grand Falls region differed between years; values of 3.0 mg L–1 TSS were found in 2005 for all metrics, but in 2006 ranged from 13 to 31.7 mg L–1 TSS as a result of higher flow and frequent flood events (Table , Figure –I). Comparison amongst regions showed that ecological reference conditions were, on average, highest for the Grand Falls region. The Okanagan Basin had intermediate ecological reference condition values, while southwestern Ontario had the lowest values (Table ).
Table 5. Results of regression-tree analyses for benthic macroinvertebrate metrics in relation to total suspended solids (TSS, mg L–1) by geographical region for 2005 and 2006. The change-point value is the anti-log of the TSS concentration and represents the ecological reference condition for TSS. The mean below (± 1 SD) is the value for the benthic macroinvertebrate endpoint below the change-point.
Figure 3 Comparison of the relationship among Ephemeroptera, Plecoptera and Trichoptera (EPT) relative abundance (%), total taxonomic richness and the Modified Family Biotic Index (MFBI) versus total suspended solids (log10 TSS mg L–1) within a geographical region: (A–C) Okanagan Basin, BC, (D–F) southwestern ON, and (H–J) Grand Falls region, NB. Data for 2005 and 2006 are represented by solid and open symbols, respectively. The solid (2005) and dashed (2006) lines represents the lines of best fit, while the vertical dashed line represents the change-point value in total suspended solids as determined from regression-tree analyses.
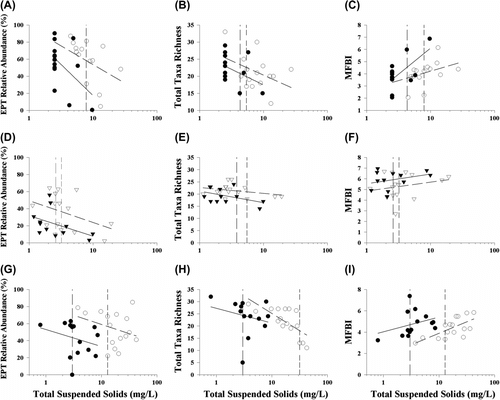
Regional physical and ecological TSS reference conditions
The five different methods used to develop physical TSS reference conditions produced relatively similar values within a region; thus, we derived a single physical TSS reference condition value for each region by averaging values from all five approaches (Table ). Use of the arithmetic mean as a method for integrating candidate values follows the approach of Cormier et al. (Citation2008). These averaged TSS values ranged from 2.7 to 6.2 mg L–1 across Canada (Table ). For the agricultural areas we examined, exceedance by individual samples of physical TSS reference condition values was lowest in the Okanagan Basin (∼20%) and greatest in Chaudière-Appalaches (∼68%), and averaged approximately 54% exceedance for all regions.
Table 6. Comparison of the physical and ecological total suspended solids (TSS, mg L–1) reference conditions for each geographic region with corresponding percent exceedance for individual samples collected. For total sample size please see Table 2.
A single ecological TSS reference condition value was produced for each of the Okanagan Basin, southwestern Ontario and Grand Falls regions by averaging values for the three metrics: EPT relative abundance, total taxa richness, and the MFBI (Table ). Ecological TSS reference conditions were greater than physically-derived values in all but one instance, and ranged from 3.5 to 11.1 mg L–1 (Table ). Exceedance of ecological TSS reference condition values was lowest in the Okanagan Basin (∼20%) and greatest in southwestern Ontario (69%; Table ). Ecological TSS reference conditions appeared to be slightly less conservative than physically-derived values, as the average exceedance for ecological values across all regions was approximately 41%.
Discussion
Analysis of data from 280 stations that were distributed across 209 streams and six agricultural regions of Canada showed that TSS in streams was positively correlated with increasing land disturbance from agriculture in four of the six regions. These changes in TSS were associated with decreased environmental conditions as shown by an increase in the relative importance of pollution tolerant taxa. Other studies have also found a positive association between increased agricultural land cover and higher TSS concentrations (Johnson et al. Citation1997; Perkins et al. Citation1998; Dodds and Whiles Citation2004; Kyriakeas and Watzin Citation2006). Often these agriculturally-related increases in TSS were associated with a decrease in environmental condition, as measured through benthic macroinvertebrate indicators (Lenat Citation1984; Kyriakeas and Watzin Citation2006; Larsen et al. Citation2009). Despite these general trends, a single, uniform TSS reference condition for all areas could not be developed because the relationship between TSS and agricultural land cover differed among geographical regions. Others have made similar observations of regional variability in TSS export (e.g., Dodds and Whiles Citation2004; Sheeder and Evans Citation2004), concluding that sediment reference conditions are likely more meaningful when developed for ecozones or smaller geographical units.
Developing physical TSS reference conditions
In our study of six Canadian geographical regions, mean TSS concentrations ranged from above 40 mg L–1 in the prairies to ⩽ 15 mg L–1 elsewhere. To produce regional TSS reference conditions associated with least disturbed conditions, we applied five different methodologies to each of these six regional data sets. The y-intercept approach was expected to produce the lowest values because it is assumed to extrapolate reference conditions without anthropogenic influence (Dodds and Oakes Citation2004; Dodds Citation2007); however, lower reference condition values were often created by the CCME approach. In contrast, the highest reference condition values tended to be produced using regression-tree and ANZECC and ARMCANZ 80th percentile analyses. In our case, the US EPA 25th percentile approach tended to produce values that were intermediate relative to the other methods. Each of these approaches has its own weaknesses; for example, ANZECC and ARMCANZ and CCME methods recommend the use of least disturbed catchments for reference condition development, yet such catchments are rare in agricultural landscapes. The CCME approach uses only periods between storm hydrographs to determine clear water conditions. In contrast, ANZECC and ARMCANZ restrict analysis to high flow periods (spring and summer), whereas other methods include information from high and low flow seasons.
Given these limitations and the fact that the five methods produced relatively similar physical reference condition values, the most robust approach to identify regional reference conditions was to average values from all approaches (c.f. Cormier et al. Citation2008). The resultant TSS reference condition values for our six agricultural regions ranged from 2.7 to 6.2 mg L–1 and are below the most stringent regulatory limits set for some USA states, i.e., 10–19 mg L–1 TSS (Dodds and Whiles Citation2004). However, for their sites with greater than 75% forest cover, a value which is roughly equivalent to our LDC catchments, Dodds and Whiles (Citation2004) demonstrated TSS concentrations of ⩽ 10 mg L–1, thus providing an explanation for our lower values. Our calculated physical reference condition values for TSS were exceeded by approximately 50% of the individual TSS samples. Dodds and Whiles (Citation2004) also found that mean stream TSS concentrations often exceeded (> 66%) standards in the United States. Although we cannot make a causal linkage between increased TSS concentration above LDC to ecological effect, we note that Graham (Citation1990) found that TSS concentrations as low as 2–5 mg L–1 had negative effects on river periphyton that resulted in poor food quality for benthic macroinvertebrates.
Developing ecological TSS reference conditions
Physical TSS reference condition values have been developed in many countries, in part because of the availability of large sets of longer-term TSS monitoring data. A more robust approach for setting TSS criteria is to associate environmental change in TSS and related factors with biotic condition. We observed that one or more of three benthic macroinvertebrate metrics (namely diversity [total taxa richness], abundance [EPT relative abundance] and relative importance of pollution intolerant taxa) were negatively related to elevated agricultural land cover. Furthermore, TSS concentration increased with higher percentage of agricultural land cover in these regions. Previous field studies revealed that increases in TSS concentration can reduce abundance of sensitive groups such as mayflies and caddisflies (Hogg and Norris Citation1991; Waters Citation1995); these effects are likely related to a combination of several mechanisms including high drift rates (Quinn et al. Citation1992; Molinos and Donohue Citation2009), sediment-related interference with and abrasion of the respiratory apparatus (Waters Citation1995), and impediments to filter (Aldridge et al. Citation1987) or visual feeding (Kefford et al. Citation2010). Although we cannot attribute cause and effect from our correlative findings, our results are consistent with the interpretation that increases in TSS concentration from least disturbed conditions may aid in identification of streams that are at risk from sedimentation (sensu Sutherland et al. Citation2002).
The sensitivity of benthic macroinvertebrate communities to TSS concentration, as reported herein and noted in the established literature, led us to hypothesize that ecological TSS reference condition values could be determined by assessing biotic change across the sedimentation gradient associated with increasing agricultural land cover. Ecological TSS reference condition values were determined using regression-tree analysis to distinguish a significant change in the relationship between biotic metrics and TSS concentration. Although between-year differences in values were small for two regions (Okanagan Basin and southwestern Ontario), the mean value for the Grand Falls region increased during a year of higher flow and frequent floods (i.e., 2006), suggesting that data for several years may be required for the development of ecological reference conditions. Nevertheless, TSS reference condition values generated from physical data alone or from relationships between biological metrics and physical data yielded similar TSS values, although in most cases the ecological values were larger. Previous research on nutrient guidelines in Wisconsin also produced greater values through assessment of change in ecological variables compared to chemical variables (Wang et al. Citation2007; Weigel and Robertson Citation2007). Comparison of physical and ecologically-derived TSS reference condition values had not been made previously, to our knowledge, although there have been several recent attempts to produce biologically-based deposited sediment values (Bryce et al. Citation2008; Cormier et al. Citation2008; Benoy et al. Citation2012).
Conclusions and recommendations
Excessive fine sediment inputs are a primary cause of ecological impairment in rivers with increased TSS concentration related to reduction of streambed particle size and heterogeneity (Bryce et al. Citation2008; Larsen and Ormerod Citation2010; Benoy et al. Citation2012). Our results suggest that TSS reference conditions can be developed for specific geographical regions, and that these derived reference condition values have the potential to identify change from least disturbed conditions. Moreover, our results suggest that these reference conditions can be derived from monthly TSS means collected over an annual period. Although sediment reference conditions are best developed by linking sediment variables, such as turbidity or TSS, to biological information, physical TSS reference conditions derived from large, long-term TSS databases, such as those we examined, may serve as interim sediment criteria, demarcating change from least disturbed condition and streams at risk to adverse sedimentation effects. Furthermore, these physical TSS reference condition values may serve as appropriate targets for evaluating restoration programs designed to improve environmental quality of agricultural catchments (e.g., beneficial or best management practice applications). To advance TSS reference condition development and improve environmental stewardship, future research aims need to focus on several key objectives. Effort must be made to broaden the geographical extent of TSS data for agricultural catchments linked to biological information through long-term biomonitoring programs along land cover disturbance gradients. Finally, there must also be ecoregional representation of smaller agricultural catchments where changes in land use have the most direct influence on changes in sediment input.
Acknowledgements
Project design benefited from ideas generated at the workshop Creating Nutrient, Sediment and Instream Flow Standards for Agricultural Watersheds, held during March 2005 in Cambridge, ON, Canada. In particular, we acknowledge the advice of L. Chow, M. Conly, A. Curry, K. Haralampides, K. Krishnappan, M. Gray, E. Luiker, C. Newcombe, C. Rabeni, D. Sauchyn and M. Stephenson. Several jurisdictions provided TSS data, including the: British Columbia Ministry of Environment (D. Einarson, V. Jensen); Alberta Agriculture, Food, and Rural Development and Alberta Environment (D. LeClair); Ontario Ministry of the Environment (S. Clerk), and Environment Canada’s Atlantic Region (C. Murphy, D. Bourgeois, D. Parent). We received logistical support from National Agri-Environmental Sciences Initiative coordinators, namely L. Maclean, C. Murphy, E. Roberts, S. Villeneuve, R. Wenting and M. Williamson. Technical assistance was provided by A. Alexander, R. Allaby, D. Bowerman, J. Erker, M. Fulton, N. Glozier, L. Grace, L. Graye, D. Halliwell, K. Heard, N. Horrigan, D. Hryn, L. Levesque, C. Logan, O. Logan, D. McGoldrick, J. McPhee, J. Mutch, T. Pascoe, K. Roach, C. Siefker, R. Smedley, S. Sylvestre, B. Upsdell and E. Wallace. Financial support to GAB, PAC and JMC was provided by Environment Canada and Agriculture and Agri-Foods Canada. JMC received funding from the National Sciences and Engineering Research Council (NSERC) Discovery Grant for Highly Qualified Personnel (HQP) support. Constructive comments from several reviewers improved earlier manuscript drafts.
References
- Aldridge , D. W. , Payne , B. S. and Miller , A. C. 1987 . The Effects of Intermittent Exposure to Suspended Solids and Turbulence on Three Species of Freshwater Mussel . Environmental Pollution , 45 : 17 – 28 .
- Australian and New Zealand Environment and Conservation Council (ANZECC) and Agricultural and Resource Management Council of Australia and New Zealand (ARMCANZ), 2000a. Australian and New Zealand Guidelines for Fresh and Marine Water Quality. National Water Quality Management Strategy Paper No. 4. Artarmon, NSW: Australian and New Zealand Environment and Conservation Council and Agriculture and Resource Management Council of Australia and New Zealand.
- Australian and New Zealand Environment and Conservation Council (ANZECC) and Agricultural and Resource Management Council of Australia and New Zealand (ARMCANZ), 2000b. Australian and New Zealand Guidelines for Fresh and Marine Water Quality: Volume 2, Aquatic Ecosystems — Rationale and Background Information (Chapter 8). National Water Quality Management Strategy Paper No. 4, 8.2-1–8.2-39. Artarmon, NSW: Australian and New Zealand Environment and Conservation Council and Agriculture and Resource Management Council of Australia and New Zealand.
- Australian and New Zealand Environment and Conservation Council (ANZECC) and Agricultural and Resource Management Council of Australia and New Zealand (ARMCANZ), 2000c. Australian and New Zealand Guidelines for Fresh and Marine Water Quality: Volume 2, Aquatic Ecosystems Aquatic Ecosystems — Rationale and Background Information (Chapter 8), Appendix 7, Comparing Test Data for Physical and Chemical Stressors with Guideline Trigger Values. National Water Quality Management Strategy Paper No. 4 A7–1-A712. Artarmon, NSW: Australian and New Zealand Environment and Conservation Council and Agriculture and Resource Management Council of Australia and New Zealand.
- Benoy , G. A. , Sutherland , A. B. , Culp , J. M. and Brua , R. B. 2012 . Physical and Ecological Thresholds for Deposited Sediments in Streams in Agricultural Landscapes . Journal of Environmental Quality , 41 : 31 – 40 .
- Breiman , L. , Freidman , J. H. , Olshen , R. A. and Stone , C. J. 1984 . Classification and Regression Trees , Belmont , CA : Chapman and Hall .
- Brua, R. B., J. M. Culp, G. A. Benoy, and P. A. Chambers. 2006. Development of Standards for Suspended Sediments and Turbidity to Reduce Sedimentation in Agricultural Watersheds. National Agri-Environmental Standards Initiative Technical Series Report No.2–51. Gatineau, QC: Environment Canada.
- Bryce , S. A. , Lomnicky , G. A. , Kaufmann , P. R. , McAllister , L. S. and Ernst , T. L. 2008 . Development of Biologically Based Sediment Criteria in Mountain Streams of the Western United States . North American Journal of Fisheries Management , 28 : 1714 – 1724 .
- Canadian Council of Ministers of the Environment (CCME). 2002. “Canadian Water Quality Guidelines for the Protection of Aquatic Life: Total Particulate Matter”. In Canadian Environmental Quality Guidelines, 1999, Updated 2002, 1–18. Winnipeg, MB: Canadian Council of Ministers of the Environment.
- Chambers , P. A. , McGoldrick , D. , Brua , R. B. , Vis , C. , Culp , J. M. and Benoy , G.A. 2012 . Development of Environmental Thresholds for Nitrogen and Phosphorus in Streams . Journal of Environmental Quality , 41 : 7 – 20 .
- Cormier , S. M. , Paul , J. F. , Spehar , R. L. , Shaw-Allen , P. , Berry , W.J. and Suter , G. W. II . 2008 . Using Field Data and Weight of Evidence to Develop Water Quality Criteria . Integrated Environmental Assessment and Management , 4 : 490 – 504 .
- Culp, J. M., G. A. Benoy, R. B. Brua, and P. A. Chambers. 2008. Total Suspended Sediment and Turbidity Standards to Prevent Excessive Sedimentation in Canadian Agricultural Streams. National Agri-Environmental Standards Initiative (NAESI) Technical Series Report No. 4–57. Gatineau, QC: Environment Canada.
- Culp, J. M., G. A. Benoy, R. B. Brua, A. B. Sutherland, and P. A. Chambers. 2009. Total Suspended Sediment, Turbidity and Deposited Sediment Standards to Prevent Excessive Sediment Effects in Canadian Streams. National Agri-Environmental Standards Initiative Synthesis Report No. 13. Gatineau, QC: Environment Canada.
- Culp , J. M. , Wrona , F. J. and Davies , R. W. 1986 . Response of Stream Benthos and Drift to Fine Sediment Deposition Versus Transport . Canadian Journal of Zoology , 64 : 1345 – 1351 .
- Dodds , W. K. 2007 . Trophic State, Eutrophication and Nutrient Criteria in Streams . Trends in Ecology and Evolution , 22 : 669 – 676 .
- Dodds , W. K. and Oakes , R. M. 2004 . A Technique for Establishing Reference Nutrient Concentrations across Watersheds Affected by Humans . Limnology and Oceanographic Methods , 2 : 333 – 341 .
- Dodds , W. K. and Whiles , M. R. 2004 . Quality and Quantity of Suspended Particles in Rivers: Continent-Scale Patterns in the United States . Environmental Management , 33 : 355 – 367 .
- Ecological Stratification Working Group. 1995. A National Ecological Framework for Canada. Report and National Map at 1:7,500,000 Scale. Ottawa/Hull, ON: Agriculture and Agri-Food Canada, Research Branch, Centre for Land and Biological Resource Research, Ottawa; and Environment Canada, State of the Environment Directorate, Ecozone Analysis Branch.
- Eilers, W., R. MacKay, L. Graham, and A. Lefebvre. 2010. Environmental Sustainability of Canadian Agriculture. Agri-Environmental Indicator Report Series – Report #3. Ottawa, ON: Agriculture and Agri-Food Canada.
- Environment Canada. 2010. Total Suspended Solids, SOP 4030, Modified from Standard Method 2540D, Revision 3.4. Saskatoon, SK: National Laboratory for Environmental Testing.
- Fairchild , J. F. , Boyle , T. , English , W. R. and Rabeni , C. 1987 . Effects of Sediment and Contaminated Sediment on Structural and Functional Components of Experimental Stream Ecosystems . Water Air Soil Pollution , 6 : 271 – 293 .
- Graham , A. A. 1990 . Siltation of Stone-Surface Periphyton in Rivers by Clay-Sized Particles from Low Concentrations in Suspension . Hydrobiologia , 199 : 107 – 115 .
- Henley , W. F. , Patterson , M. A. , Neves , R. J. and Lemly , D. 2000 . Effects of Sedimentation and Turbidity on Lotic Food Webs: A Concise Review for Natural Resource Managers . Selected Reviews in Fisheries Science , 8 : 125 – 139 .
- Hilsenhoff , W. L. 1988 . Rapid Field Assessment of Organic Pollution with a Family-Level Biotic Index . Journal of the North American Benthological Society , 7 : 65 – 68 .
- Hogg , I. D. and Norris , R. H. 1991 . Effects of Runoff from Land Clearing and Urban Development on the Distribution and Abundance of Macroinvertebrates in Pool Areas of a River . Australian Journal of Marine and Freshwater Research , 42 : 507 – 518 .
- Johnson , L. B. , Richards , C. , Host , G. E. and Arthur , J. W. 1997 . Landscape Influences on Water Chemistry in Midwestern Stream Ecosystems . Freshwater Biology , 37 : 193 – 208 .
- Kefford , B. J. , Zalizniak , L. , Dunlop , J. E. , Nugegoda , D. and Choy , S. C. 2010 . How are Macroinvertebrates of Slow Flowing Lotic Systems Directly Affected by Suspended and Deposited Sediment? . Environmental Pollution , 158 : 543 – 550 .
- Kyriakeas , S. A. and Watzin , M. C. 2006 . Effects of Adjacent Agricultural Activities and Watershed Characteristics on Stream Macroinvertebrate Communities . Journal of the American Water Resources Association , 42 : 425 – 441 .
- Larsen , S. and Ormerod , S. J. 2010 . Low-Level Effects of Inert Sediments on Temperate Stream Invertebrates . Freshwater Biology , 55 : 476 – 486 .
- Larsen , S. , Vaughan , I. P. and Ormerod , S. J. 2009 . Scale-Dependent Effects of Fine Sediments on Temperate Headwater Invertebrates . Freshwater Biology , 54 : 203 – 219 .
- Lenat , D. R. 1984 . Agriculture and Stream Water Quality: A Biological Evaluation of Erosion Control Practices . Environmental Management , 8 : 333 – 344 .
- Marchant , R. 1989 . A Subsampler for Samples of Benthic Invertebrates . Bulletin of the Australian Society of Limnology , 12 : 49 – 52 .
- Molinos , J. G. and Donohue , I. 2009 . Differential Contribution of Concentration and Exposure Time to Sediment Dose Effects on Stream Biota . Journal of the North American Benthological Society , 28 : 110 – 121 .
- Natural Resources Canada . 2006 . National Topographic Data Base, Machine Readable Data Files , Ottawa , ON : Natural Resources Canada, Centre for Topographic Information .
- Newcombe , C. P. and MacDonald , D. D. 1991 . Effects of Suspended Sediments on Aquatic Ecosystems . North American Journal of Fisheries Management , 11 : 72 – 82 .
- Perkins , B. D. , Lohman , K. , Van Nieuwenhuyse , E. and Jones , J. R. 1998 . An Examination of Land Cover and Stream Water Quality among Physiographic Provinces of Missouri, USA . Verhandlungen der Internationalen Vereinigung für Theoretische und Angewandte Limnologie , 26 : 940 – 947 .
- Peters , D. L. , Baird , D. J. , Monk , W. A. and Armanini , D. G. 2012 . Establishing Standards and Assessment Criteria for Ecological Instream Flow Needs in Agricultural Regions of Canada . Journal of Environmental Quality , 41 : 41 – 51 .
- Quinn , J. M. , Davies-Colley , R. J. , Hickey , C. W. , Vickers , M. L. and Ryan , P. A. 1992 . Effects of Clay Discharges on Streams. 2. Benthic Invertebrates . Hydrobiologia , 248 : 235 – 247 .
- Reynoldson , T. B. , Logan , C. , Pascoe , T. and Thompson , S. P. 2006 . CABIN (Canadian Aquatic Biomonitoring Network) Invertebrate Biomonitoring Field and Laboratory Manual for Running Water Habitats , Vancouver , BC : Environment Canada .
- Reynoldson , T. B. , Norris , R. H. , Resh , V. H. and Rosenberg , D. M. 1997 . The Reference Condition: A Comparison of Multimetric and Multivariate Approaches to Assess Water-Quality Impairment Using Benthic Macroinvertebrates . Journal of the North American Benthological Society , 16 : 833 – 852 .
- Shaw , E. A. and Richardson , J. S. 2001 . Direct and Indirect Effects of Sediment Pulse Duration on Stream Invertebrate Assemblages and Rainbow Trout (Oncorhynchu mykiss) Growth and Survival . Canadian Journal of Fisheries and Aquatic Sciences , 58 : 2213 – 2221 .
- Sheeder , S. A. and Evans , B. M. 2004 . Estimating Nutrient and Sediment Threshold Criteria for Biological Impairment in Pennsylvania Watersheds . Journal of the American Water Resources Association , 40 : 881 – 888 .
- Stoddard , J. L. , Larsen , D. P. , Hawkins , C. P. , Johnson , R. K. and Norris , R. H. 2006 . Setting Expectations for the Ecological Condition of Streams: The Concept of Reference Condition . Ecological Applications , 16 : 1267 – 1276 .
- Sutherland , A. B. , Meyer , J. L. and Gardiner , E. P. 2002 . Effects of Land Cover on Sediment Regime and Fish Assemblage Structure in Four Southern Appalachian Streams . Freshwater Biology , 47 : 1791 – 1805 .
- United States Environmental Protection (US EPA Agency) . 2000 . Ambient Water Quality Criteria Recommendations: Information Supporting the Development of State and Tribal Nutrient Criteria for Rivers and Streams in Nutrient Ecoregion I-XIV , Washington , DC : US Environmental Protection Agency, Office of Water, Office of Science and Technology, Health and Ecological Criteria Division .
- United States Environmental Protection Agency (US EPA). 2002. National Water Quality Inventory 2000 Report. EPA/841-R-02-001. Washington, DC: US Environmental Protection Agency, Office of Water.
- United States Environmental Protection Agency (US EPA). 2006. Framework for Developing Suspended and Bedded Sediment (SABS) Water Quality Criteria. EPA-822-R-06-001. Washington, DC: US Environmental Protection Agency, Office of Research and Development, Office of Water.
- United States Environmental Protection Agency (US EPA). 2010. Using Stressor-Response Relationships to Derive Numeric Nutrient Criteria. EPA-820-S-10-001. Washington, DC: US Environmental Protection Agency, Office of Science and Technology, Office of Water.
- Wang , L. , Robertson , D. M. and Garrison , P. J. 2007 . Linkages between Nutrients and Assemblages of Macroinvertebrates and Fish in Wadeable Streams: Implication to Nutrient Criteria Development . Environmental Management , 39 : 194 – 212 .
- Waters, T. F. 1995. Sediment in Streams: Sources, Biological Effects and Control. Bethesda, MD: American Fisheries Society Monograph 7.
- Weigel , B. and Robertson , D. 2007 . Identifying Biotic Integrity and Water Chemistry Relations in Nonwadeable Rivers of Wisconsin: Toward the Development of Nutrient Criteria . Environmental Management , 40 : 691 – 670 .