Abstract
Fish-passable rock riffles are widely used to ease fish passage at road crossings and dams and to restore pool and riffle habitats in channelized streams. Pools, runs and riffles are created by breaking the uniform hydraulic gradient of channelized streams into relatively mild, shallow and steeply sloping segments. Riffle height is governed by the stage of the upstream pool required to pass the channel design flood or to backflood a fish passage structure. Riffles are mildly v-shaped to extend the flow across the channel or constrict the width to create a central torrent that will maintain a deeper downstream pool. The riffles may be closely spaced to create stepped pool channels or spread apart to divide the spacing into runs and pools typical of meandering channels. Four cases of riffle, pool and run dimensions are discussed with illustrative projects in walleye, salmon and trout streams. These projects, undertaken in the last three decades, illustrate their long-term stability and improvement in fish habitat. The projects are in four physiographic settings to emphasize the need for site-specific surveys peculiar to inherited post-glacial river channels and valleys in Canada.
Les radiers de roches sont largement utilisés pour faciliter le passage des poissons aux traversées routières et aux barrages et pour restaurer les habitats calmes et troubles dans les cours d’eau canalisés. Des fosses, des passages et des radiers sont crées en divisant le gradient hydraulique uniforme des cours d’eau canalisés en segments relativement peu inclinés, peu profonds et abrupts (fortement inclinés). La hauteur du radier est dictée par le niveau de la fosse en amont nécessaire au passage de la crue nominale ou nécessaire pour inonder par reflux un ouvrage de passe à poissons. Les radiers sont conçus légèrement en forme de V pour étendre le débit d’un bout à l’autre du chenal, ou encore leur largeur est réduite afin de créer un torrent central qui assurera le maintien d’une fosse plus profonde en aval. Les radiers peuvent être étroitement espacés pour créer des chenaux en escalier ou ils peuvent être distancés afin de diviser l’espacement en passages et en fosses propres aux chenaux à méandres. Quatre cas de radiers, de fosses et de passages de différentes dimensions sont abordés à l’aide de projets d’illustration dans des cours d’eau abritant des dorés jaunes, des saumons et des truites. Ces projets ont été entrepris au cours des trois dernières décennies de manière à illustrer leur stabilité à long terme et l’amélioration de l’habitat du poisson. Ils sont exécutés dans quatre milieux physiographiques afin de mettre en évidence le besoin d’études propres au site portant spécifiquement sur les vallées et les chenaux fluviaux hérités de la période postglaciaire au Canada.
Introduction
Fish-passable rock riffles may be used as drop structures to control bed erosion, reduce meander migration, form backwatering pools for stream crossings, restore aquatic habitats and create low flow continuity. Pool, riffle and meander dimensions have been observed in valley bottomlands formed by contemporary alluvial rivers under the present climatic regime (Leopold and Wolman 1957). Meandering occurs in the mid and lower reaches of a sequence of channel patterns in an idealized concave upward alluvial river profile (Leopold and Maddock 1953; Vannote et al. Citation1980). The thalweg undulates with shallow gradients in pools and steeper gradients in runs and riffles (Leopold and Wolman Citation1960; Jowett Citation1983). Average pool-riffle spacing measured in numerous studies falls between five and seven alluvial channel widths in alluvial streams (Leopold and Wolman Citation1960; Chang Citation1988; Gregory et al. Citation1994). In Canada, with the exception of a few elevated landforms, stream valley bottomlands have been created or altered by glaciation and late-glacial meltwater in a different climatic regime. There is seldom an orderly sequence of channel patterns that persists for more than a portion of the river profile. For example, Mollard and Janes (Citation1984) found segments of 20 types of gullies and river channels in 25 different catchment patterns in Canada. The dimensions and spacing of riffles, runs and pools varied widely in response to stable lag deposits, broken valley gradients and other remnants of pro-glacial drainage channels. This does not limit the use of riffles as grade control structures in stream restoration projects but they must be tailored to fit their post-glacial setting.
Riffle design geometry
The riffle design height is normally governed by a targeted upstream pool stage. The stage may be the breakout point to the floodplain or set to an elevation that will form a pool to backflood stream crossing works, drop structures and dam tailraces. Riffle spacing along a stream reach determines the allocation of potential energy between pools, runs and riffles. Closely spaced riffles form backflooding pools between the downstream riffle crest and the upstream riffle face. The largest part of the potential energy in the reach is dissipated in the drop on the riffle surface and the deceleration zone at the head of the next downstream pool. As the spacing of the same size of riffles increases, a larger part of the potential energy in the reach is dissipated in runs and pools (Leopold et al. Citation1964, Chapter 7). The riffle profile varies from simple rock chutes to shallow cobble bed bars depending on fish passage and spawning requirements, the maintenance of downstream pool habitats and the stable size of the bed paving materials. Fish swimming ability and endurance governs the downslope gradient and ruggedness of fish-passable riffles. Emergent boulders and cobbles break the flow into short torrents and pockets of refugia on the riffle face. A comprehensive review of rock grade control structures found that passage was successful if the average gradients were 0.063 or less for freshwater species (Harper et al. Citation1998; Thomas et al. 2011). Successful fish passage gradients observed in reference reaches in Canadian streams ranged from 0.05 for freshwater species to 0.10 for salmonid species (Newbury and Gaboury Citation1993; Newbury Citation2010). Design dimensions for riffles, runs and pools (Table ) are shown schematically in Figure . Four cases of pool, run and riffle forms are illustrated with examples from several Canadian projects undertaken between 1976 and 2010 (Newbury and Gaboury Citation1994; Newbury Citation2010).
Table 1. Summary of riffle, pool, and run dimensions (Figure ).
Figure 1 Riffle, run, and pool dimensions. Riffle spacing may be adjusted to create stepped pools or run and pool profiles typically found in meandering channels. The downstream slope SB is shallow (< 0.01) to allow the flow to decelerate in an undulating surface hydraulic jump in the run or pool below.
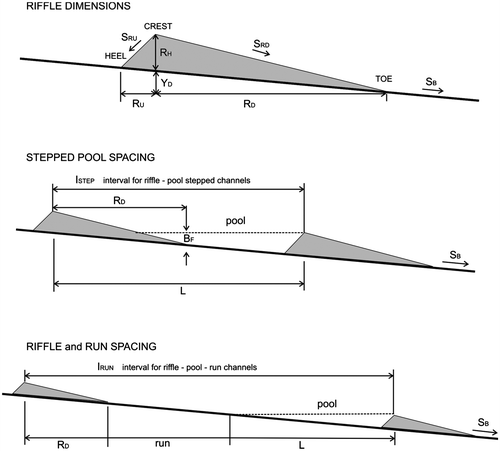
Case 1. Stepped pools with uniform width riffles
The crest and downstream face of uniform riffles extend across the full width of the channel. The surface slopes towards the centre of the channel to form a shallow v-shaped cross-section (Figure ). This allows low flows to collect in central fish-passable channels between emergent cobbles. During floods the flow exits the riffle with a strong central current allowing lower velocities and back-eddies to form along the downstream banks.
Figure 2 Uniform width riffles extend between the toes of the banks for the full length of the downstream face. They are shallowly v-shaped in cross-section to concentrate low discharges in fish-passable channels between cobbles and boulders.
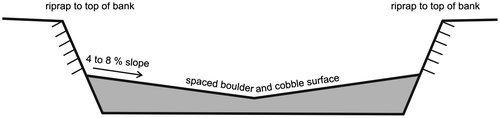
The surface of the riffle is finished with a range of emergent cobbles and boulder sizes that are aligned and spaced to break the flow into paths that can be followed up the riffle face by migrating fish (Figure ). Under moderate flood discharges, channels on the edges of the riffle replace channels in the central part of the riffle as the flow depth and velocity increase.
Lake Dauphin, MB, walleye restoration projects
Sediments deposited by Glacial Lake Agassiz at the end of the Pleistocene Epoch formed the flat prairie landscape east of the Canadian Shield and west of the Manitoba Escarpment in southern Manitoba. Streams meander in the fine-grained sediments with gradients as low as 0.0003. Beginning in the late 1800s, Federal and Provincial Drainage Acts were adopted to provide drainage networks that would remove snowmelt and storm water that was standing on the flat agricultural fields to extend the short summer growing season (Bower Citation2007). This led to a proliferation of drainage canals on public road allowances to replace existing streams. A typical network of drainage canals, locally called drains, leading from Riding Mountain National Park on the Manitoba Escarpment to Lake Dauphin is shown in Figure .
Figure 3 A uniform riffle constructed in the Okanagan River in Oliver, BC. The 1.2-m high riffle floods the stilling basin and tailrace below Vertical Drop Structure No. 12 to ease fish passage. The deeper central portion of the riffle is finished with rounded boulders to form a smooth chute for recreational swimmers and rafters. Eliminating the plunge pool and hydraulic jump reduces the drowning hazard at the vertical drop structure. Riprap extends to the top of the banks for the length of the riffle.
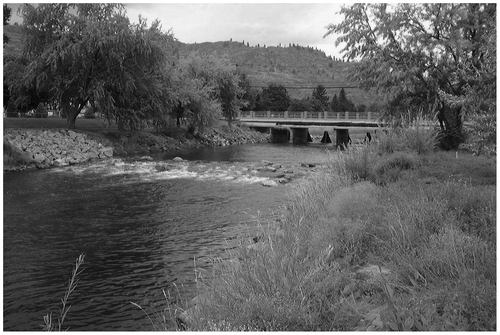
Figure 4 The network of drainage canals and channelized streams leading from Riding Mountain National Park to Lake Dauphin, MB. All but the largest streams have been straightened and relocated to follow a 30.2-m strip of public land separating square-mile agricultural sections.
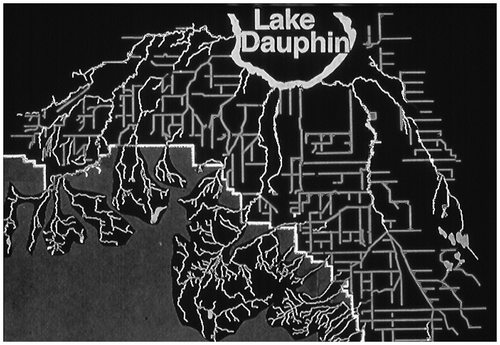
The headwater streams are unaltered until they reach the Park boundary. There the drainage network begins in deltas and alluvial fans of weathered shale particles deposited by glacial meltwater streams entering Glacial Lake Agassiz as recently as 7000 years BP (McGinn Citation1979). A typical fan at the foot of the escarpment at Wilson Creek is shown in Figure (McKay Citation1969; Schwartz Citation1970).
Figure 5 Alluvial fans of weathered Cretaceous shale are deposited on the abandoned beaches and bed of Glacial Lake Agassiz at the foot of the Manitoba Escarpment. The fans occur below meltwater valleys formed in the late Wisconsin period (Klassen Citation1978). In 1928 a small drainage canal was excavated between the toe of the Wilson Creek fan and an east-west drainage canal on the northern edge of the deposits (Committee on Headwater Flood and Erosion Control Citation1983).
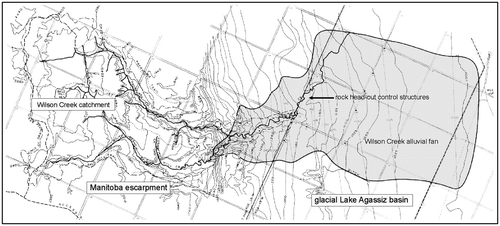
Before the drainage network extended to the foot of the escarpment, flood discharges would disperse and infiltrate into the fan deposits. After the drainage canals were extended across the alluvial fans to intercept the streams at the foot of the escarpment, the flow was concentrated into a single channel that rapidly downcut through the deposits (Figure ) (Committee on Headwater Flood and Erosion Control Citation1983).
Figure 6 The Wilson Creek canyon (1975) is typical of channels that eroded to the base of the alluvial fan deposits when drainage canals were connected to the foot of the Manitoba Escarpment. The original channel was dug with a horse and scoop in 1928.
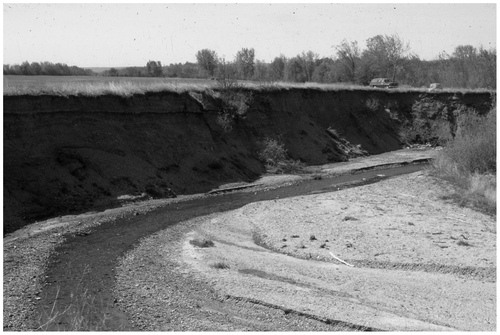
The eroded sediments were carried downstream and deposited in the lower gradient canal below the fan, causing local flooding and disrupting highway and rail crossings. Re-excavation of the Wilson Creek drain and similar alluvial fan drains was required on average every seven years (Figure ) (Committee on Headwater Flood and Erosion Control Citation1983).
Figure 7 Low gradient channelized streams in the farmland below infill and widen with shale gravels eroded from the alluvial fans. The channels are regularly re-excavated as they approach the boundaries of the 30.2-m wide public road allowances separating square-mile farm sections (Thomson Citation1967). Baseline stakes for the re-excavation of the Wilson Creek drain are shown in the foreground.
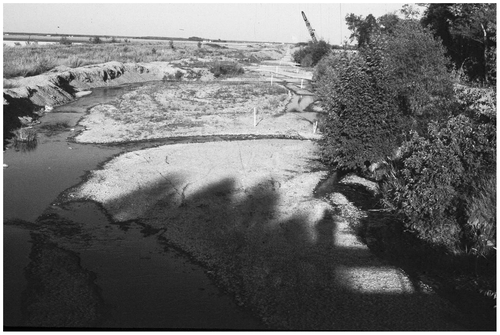
Beginning in 1976, a series of 2.0-m high rock riffles were constructed in the Wilson Creek canyon to break the steep profile into stepped pools and stop further head cutting into the fan (Figure ). The lower gradient in the pools stopped bank erosion and re-transporting of shale gravels. The stable bottom and sides of the canyons were rapidly overgrown with shrubs and trees (Figure ).
Figure 8 A typical rock riffle drop structure constructed in 1976 in the Wilson Creek alluvial fan canyon to create a stepped pool profile. Similar structures were constructed in all Riding Mountain alluvial fans connected to the drainage network.
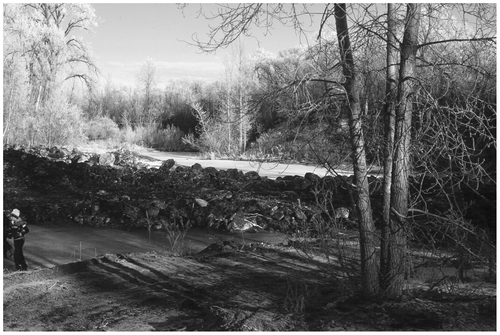
Figure 9 The pool and vegetation infilling the reach above the uppermost Wilson Creek drop structure 10 years after construction. A small shale gravel bar has deposited at the head of the pool. There is no coarse bed load transported through the reach.
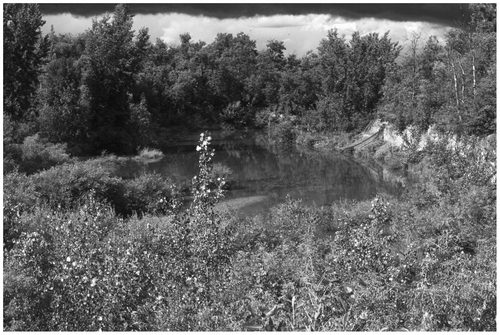
In the ensuing 10 years, similar rock riffles and stepped pools were added to all of the Riding Mountain escarpment streams with drainage canals running through alluvial fans. Attention was then turned to stabilizing and restoring canals that had replaced walleye spawning streams tributary to Lake Dauphin. Channelization destroyed spawning riffles and shallow pools that provided flow continuity for walleye fry drifting back to Lake Dauphin. The commercial walleye fishery declined from 100,000 kg in 1950 to 5000 kg in 1965 (Gaboury Citation1985). The design and construction of a typical project is summarized for Mink Creek, one of a dozen reaches restored between 1985 and 1993 (Newbury and Gaboury Citation1993; Gaboury et al. Citation1997).
Mink Creek restoration project
The meandering channel of Mink Creek was straightened and channelized in 1951 as part of the drainage improvement works for lands surrounding Lake Dauphin, MB (Figure ). In 1985 rock riffles and pools were added to steeper reaches (0.003 gradient) of the channel that crossed the foreshore of abandoned Lake Agassiz beaches (Klassen Citation1978). The riffles and pools were designed to restore walleye spawning habitats observed in natural streams (Figure ). The riffles were designed to mimic walleye spawning conditions observed in nearby unaltered streams (Gaboury Citation1985). A typical reference riffle on the Valley River, MB, is shown in Figure .
Figure 10 A ford crossing in the channelized reach of Mink Creek viewed from Provincial Trunk Highway 20, 3 km west of Lake Dauphin, MB, in 1984. The channel has been reconstructed three times since 1951 as the shale gravel bed eroded and the banks collapsed. The 17 m3/s design flood capacity at the floodplain level increased to 76 m3/s in the down-cutting channel.
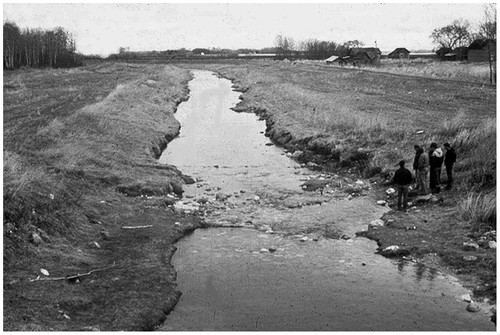
Figure 11 Fish passable rock riffles were constructed in the Mink Creek drain below Provincial Trunk Highway 20 in 1985. The reduced gradient between riffles stopped bed erosion and bank collapse and created a continuous low flow path to Lake Dauphin for walleye spawning and fry drifting back to the lake. The reach is shown in 1995, 10 years after construction. The thalweg of the flow has developed an alternating path between riffles but there is insufficient energy in the pools to initiate meandering.
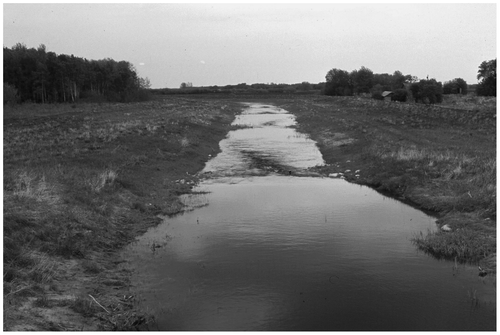
Figure 12 A walleye spawning riffle on the Valley River, MB, during the high discharge spring runoff period. Spawning walleye were observed in night surveys on and just above the crest of the riffle. Fish egg densities were sampled by suctioning and filtering water from the surface of the emergent rocks (Newbury and Gaboury Citation1994).
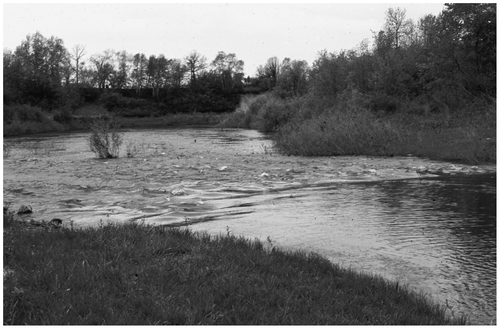
The natural riffle downslopes are mild, not exceeding 0.05 gradients. The exit flow from the riffle enters the channel at a shallow angle, forming an undular hydraulic jump near the surface of the downstream pool (Froude Numbers 0.8 to 1.2; Chow Citation1959). The surface of the riffles is hydraulically rough with Manning’s resistance factors of 0.07 under flood conditions and 0.20 under low mid-summer discharges. Large emergent cobbles and boulders trap walleye eggs dispersed by fish spawning in the flow immediately above the riffle crest. A smaller constructed riffle on Mink Creek under low flow conditions in the period of walleye fry return is shown in Figure .
Figure 13 Fish passable rock riffles were constructed in steeper reaches of Mink Creek, MB, to restore flow continuity and walleye spawning habitats. Emergent cobbles and boulders are arranged on the surface to trap walleye eggs dispersed in the pool above the crest of the riffle in the high discharge spring spawning period. The v-shaped face of the riffle concentrates the flow to ensure fish and fry passage in small channels between cobbles under low discharge conditions.
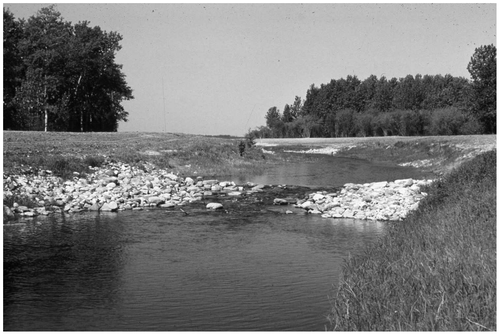
The specific energy in the pool above the riffle, as the 17 m3/s design flood stage just reaches the floodplain level, governs the allowable riffle height and spacing. In mildly sloping channels such as this, less specific energy is required to pass the same discharge at the critical velocity (Chow Citation1959). The maximum allowable riffle height is the difference in specific energy between the sub-critical flow in the approaching pool and the critical flow on the riffle crest shown schematically on the specific energy curve (Figure ).
Figure 14 The average riffle height in the Mink Creek reach is 0.54 m. The riffle crests are 0.56 m below the uniformly graded floodplain.
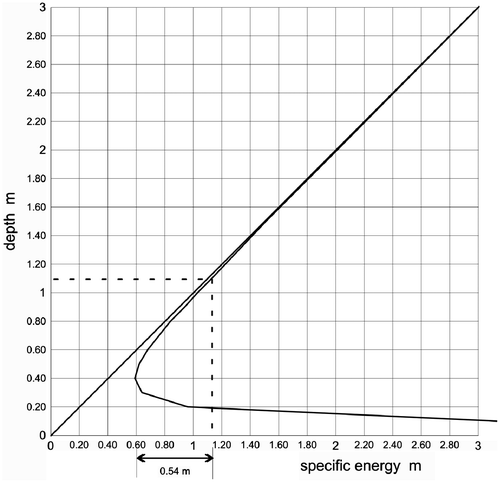
The riffle spacing is approximately 100 m and creates 0.27 m of backflooding depth on the upstream riffle toe (see dimensions Table ). Backflooding the downstream face of the upstream riffle ensures that there is a continuous watercourse for walleye fry to drift back to Lake Dauphin after the spring runoff period (Figure ). The Mink Creek riffles were constructed in mid-winter when nearby fields were frozen to allow access to fieldstone piles in the surrounding farmland. There was no surface discharge in the stream (Figure ).
Figure 15 Pools and riffles in the Mink Creek channel under low discharge conditions (June 1988). Flow continuity is ensured by backflooding the downstream face of the upstream riffle.
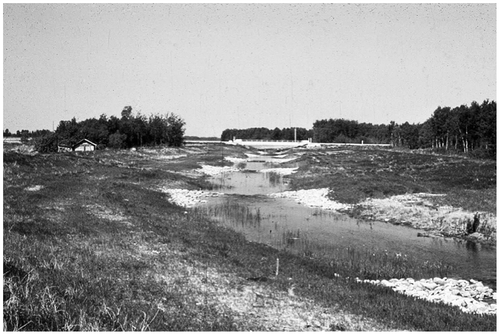
Figure 16 Mink Creek riffles constructed in the winter of 1985/86. Fieldstone was gathered from nearby farms that had been set aside as the land was prepared for cultivation. Sand and shale gravels from the bed of the stream were used to infill the interstitial spaces.
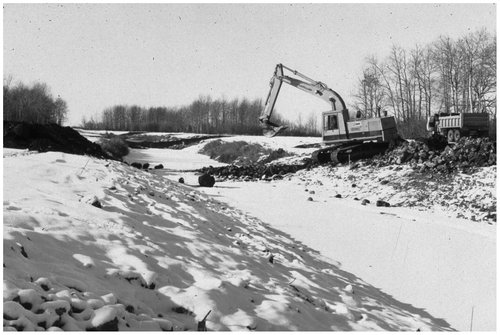
The rock was sorted to set aside river cobbles and boulders (200 to 400 mm) to finish the downstream face of the riffle. The remaining rock was spilled down the banks to a tracked backhoe. A shallow trench filled with larger rock (600 to 900 mm) was constructed across the channel at the riffle crest. The upstream and downstream grades were staked out on the bed and banks and filled with layers of randomly sized rock. Gaps between the rocks were infilled with sands and shale gravels excavated from the trench. The backhoe tracks ran back and forth on the riffle face to compact the fill in layers. Seepage cutoff walls in the core of the riffles were not found necessary as long as the materials were well infilled and compacted. Emergent rounded rocks were set in the surface of the last layer in a pattern that would create low discharge channels on the riffle surface. The minimum stable rock size on the riffle surface was calculated from the bed shear stress at the maximum design flood (Lane Citation1955). In the final stage of construction, the banks adjacent to the riffle were riprapped and the site was cleared of debris and seeded with rye grass.
After the first flood discharge, the pools were deepened for a short reach below the riffles. There has been no movement of the riffles. Degradation and bank slumping in the shale gravel channel that was occurring prior to the project halted. There is no riparian vegetation as the floodplains are regularly mowed for hay. Fisheries monitoring in the reach in the next 10 years found the same rate of walleye egg retention and fry passage as observed in nearby unaltered streams (Newbury and Gaboury Citation1993).
Case 2. Stepped pools with constricted width riffles
Riffle cross-sections may be narrowed to create central torrents that extend high-velocity exit flows further into the downstream pool (Figure ). In salmonid streams the gradient of the downstream face may be as high as 0.01 without restricting fish passage at low and moderate flood discharges. The torrent maintains a deeper central channel in the upper reach of the pool.
Figure 17 Uniform riffles may be constricted in width to form a central torrent into the downstream pool. The allowable constricted riffle height is lower to accommodate the constriction at the same floodplain access discharge.
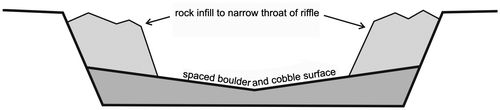
The difference between the specific energy at the riffle crest and the specific energy of the design flood in the pool approaching the riffle is divided between the effects of narrowing the channel and the riffle height. Maximum channel narrowing occurs when the riffle height is zero. The maximum riffle height occurs when there is no narrowing as in the uniform riffle case. A typical constricted width riffle is shown in Figure .
Figure 18 A constricted riffle in Oulette Creek, BC. A deep overwintering pool for Coho salmon is maintained by the flood torrent in the upper part of the pool. Logs and other woody debris are cabled to the banks of the stream in the pool area to provide cover from predators.
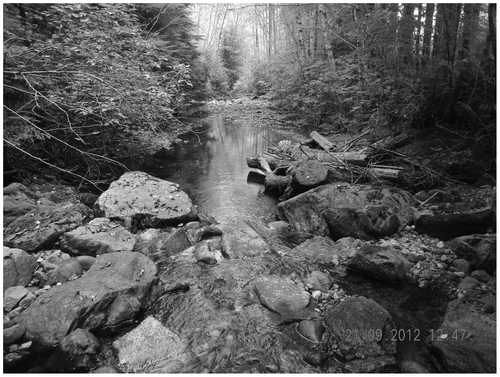
If the flood torrent is sufficiently strong, back-eddies are created on either side of the entering flow (Figure ). Observations in nearby streams suggest that the torrent flow separates in the pool to create back-eddies when the ratio of Froude numbers between the riffle and pool are 5:1 or more (Bates Citation2000).
Oulette Creek salmon restoration project
The eastern shore of Georgia Strait is predominantly bound by the steep bedrock cliffs of the Coastal Mountains. Alluvial fans are formed at the mouths of fjords and glaciated valleys draining the mountains and interior plateau. Towns, mills, ferry terminals, harbours and historic settlements occupy the fans. Branching streams on the fan surface are collected into a single channel and relocated to the edge of the developments (Gaboury and McCulloch 2002). In 1976, Oulette Creek was intercepted at the head of the alluvial fan and rerouted to follow the edge of a log sorting yard on Howe Sound (Figure ).
Figure 19 The central torrent formed in the Oulette Creek constricted riffle shown in Figure . The flood discharge is at the design floodplain stage in the approaching pool.
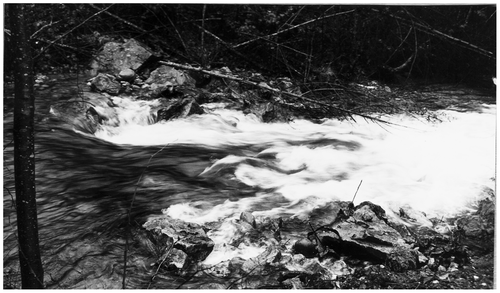
Figure 20 The Oulette Creek, BC, alluvial fan was cleared and infilled to provide a log sorting yard in 1978. The stream was collected in a single channel constructed on the eastern edge of the fan. Oulette Creek falls 385m in 3km from the peak of Mount Elphinstone. The stream gradient changes from 0.12 to 0.03 at the toe of the fan. There were no fish spawning in the rugged channel above the fan.
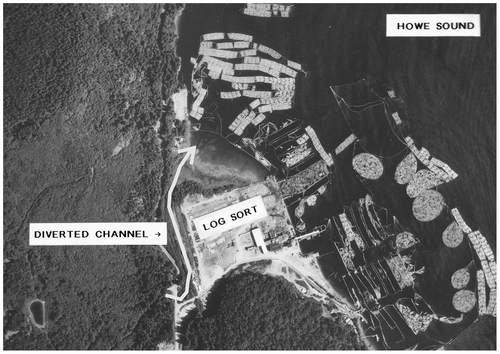
The diversion was constructed in 100 m reaches with alternating “digger” logs and cross-vane rock sills to promote gravel accumulation and overwintering pools (Figure ). In the first flood the logs and rock vanes were undermined or buried in eroded pools scoured by the sharply plunging overflow (Figure ). The channel bed eroded 1.1 m before it was paved with large cobbles and boulders. Although a small hatchery was built as compensation for fish habitat loss there were no spawning fish returns to the channel.
Figure 21 Construction of a 1.2-km long Oulette Creek diversion channel from the toe of the alluvial fan to Howe Sound, BC. Braided channels on the fan surface were infilled for the development and protected by the dyke shown on the left. The design discharge at the constructed floodplain level was 17 m3/s.
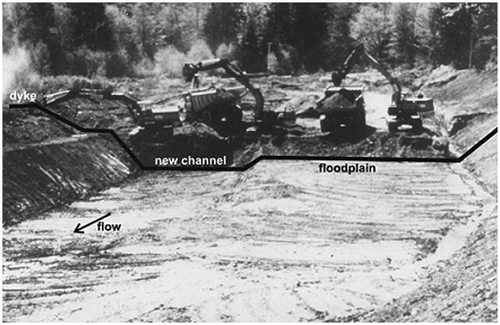
Figure 22 The Oulette Creek diversion channel four years after construction. The cross-vane rock sill in the foreground is buried in an infilled plunge pool. The 1.2-m diameter digger log in the background is undermined and suspended from the banks.
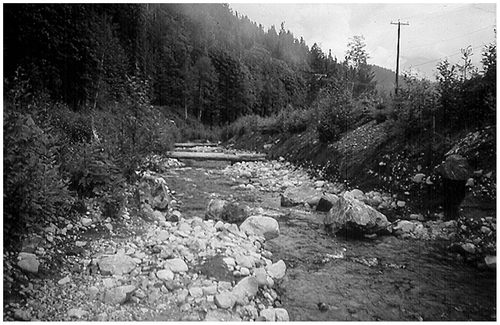
In 1994, riffles with a downslope gradient of 0.10 were constructed at the former log and cross-vane drop structures locations. The riffle heights in the entrenched channel were set to reactivate the constructed floodplain at the design discharge of 17 m3/s. (Figure ).
Figure 23 Rock riffles replaced the original logs and cross-vane drop structures in the entrenched channel in 1994. The riffle heights were raised to re-activate the constructed floodplain at the original 17 m3/s design flood.
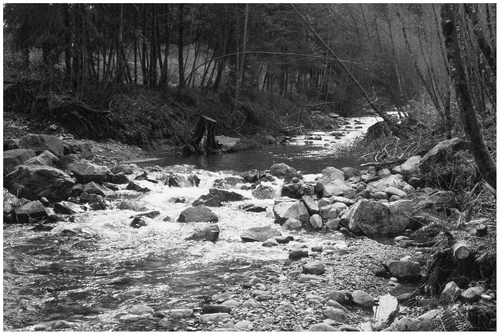
Two riffle configurations were used. The lower two riffles from mean tidal level were built with a 9-m wide uniform crest. Pink and chum salmon created redds in the flat bed of coarse gravels accumulated above the riffles in the first year after construction. The width of the upstream riffles was reduced to 6 m with large boulders projecting from the banks to form a torrent that would maintain a scoured segment of the downstream pool. Coho salmon spawn in the cobbles and coarse gravels accumulated above the riffles. Coho fry overwinter in the deep section of the pool below the riffle. The riffle spacing of 30.5 m backflooded the downstream face of the riffles by 0.2 m, providing continuous access to Howe Sound. The riffle height was reduced from 0.78 m to 0.46 m to accommodate the narrower crest with the same specific energy in the upstream pool (Figure ).
Figure 24 Specific energy curves for riffles added to the Oulette Creek diversion (9 m wide, solid line; 6 m wide, dashed line). The corresponding riffle heights are 0.78 and 0.46 m resp.
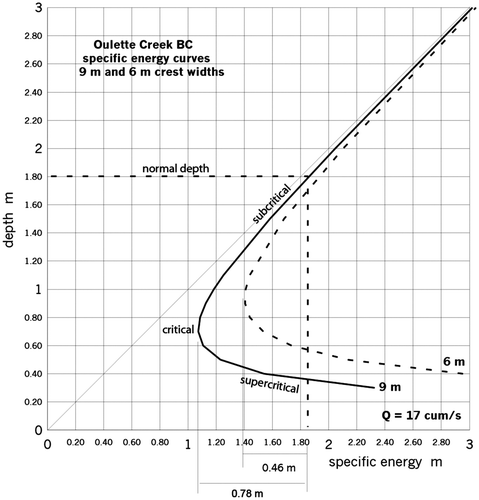
Monitoring for 12 years after construction found that approximately equal areas of pool and riffle habitats have been maintained by the narrowed riffles (Figure ). The biomass of fish increased dramatically following restoration (Figure ).
Figure 25 The distribution of riffles and pools (black bar) in the Oulette Creek diversion channel before and after the addition of riffles in 1994 (Newbury et al. 2011).
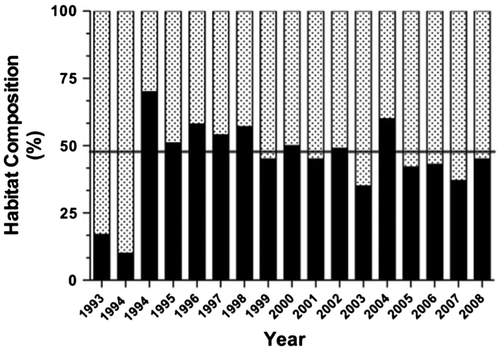
Figure 26 The response of fish biomass to the creation of a pool and riffle profile in Oulette Creek (Newbury et al. 2011).
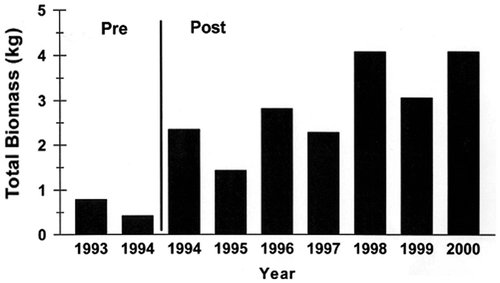
The riffles were constructed in compacted layers of rock and infill during periods of low discharge. A tracked backhoe created boulder ramps as it moved across the stream, allowing the flow to run under the tracks (Figure ). Periodic pool maintenance is required as coarse bed load sediment continues to be delivered to the head of the alluvial fan from the steeply sloping basin (Walker et al. Citation2004). Sediments in the upper pool are removed from an access point built on the floodplain at the time of construction. The upper pool filled with cobbles and coarse sediment in the first 10 years. Similar rates of infill have been observed at nearby projects in Twin, Langdale and Chapman Creeks.
Figure 27 Riffle construction was undertaken during low discharge periods by placing the largest emergent boulders on the riffle face to act as an elevated ramp for the tracked backhoe. This allowed the stream to flow between the boulders. The gaps were infilled and packed with cobbles and gravels from the downstream pool.
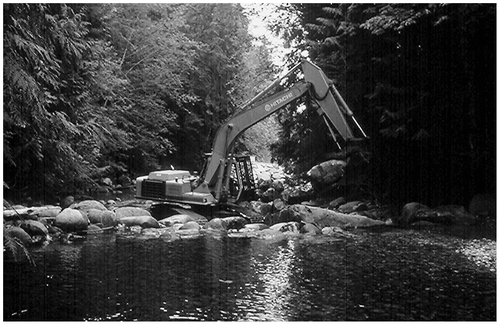
Dickson Brook project, Fundy National Park
In the previous two examples, the allowable riffle heights were set by the channelized stream design and the elevation of the uniformly constructed floodplains. Hydraulic analysis was simplified as all the riffles were built to the same height. In many projects the streams have been channelized and diverted with no alteration of the existing floodplain elevation. In these cases, flood discharges must be routed through the reach to determine the channel capacity and flood breakout points. For example, in the Dickson Brook restoration project through the Fundy National Park golf course (Figure ) riffle heights were adjusted to allow flood waters to follow paths though the course that avoided greens and long runs through fairways (Newbury Citation2010; Watts Citation2011). Riparian shrubs and log and boulder cover were added to out-of-play reaches to create brook trout and Atlantic salmon habitats. The stream was restocked with Atlantic salmon in 2010 as part of the Bay of Fundy salmon recovery strategy (Fisheries and Oceans Canada 2010).
Figure 28 A stepped pool profile was constructed in Dickson Brook, NB, through the Fundy National Park golf course, to stabilize the stream and provide salmon and sea run brook trout habitat. The channel is fed by four steeply sloping tributaries from the bedrock sides of the emerging coastal valley (Atlantic Geoscience Society 2001) (photo J. Watts).
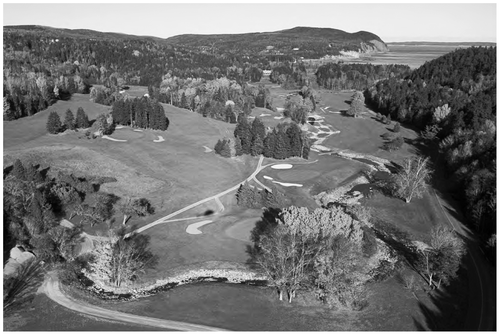
The Hydraulic Engineering Center River Analysis System (HEC-RAS; www.hec.usace.army.mil/software) modelled profiles for two pool and riffle segments between a fairway and green (Figure ) are shown in Figure at a floodplain breakout discharge of 20 m3/s. Floodplain access in less vulnerable lower reaches occurs at the median annual instantaneous flood peak of 11 m3/s.
Case 3. Riffles, pools, and runs
Riffles may be designed to create spawning bars and circulating pool habitats in stream meanders where there is sufficient gradient. The main difference from the previous stepped channel configuration is the distribution of energy between the meander pool and the downstream riffle. The gradient in the run and pool must be sufficient to overcome frictional losses and provide the momentum required to rotate the flow in a helical pattern with a small return current on the inside of the meander bend. Helical flow conditions are optimized when the radius of curvature of the upper third of the meander is between two and three times the channel width (Bagnold Citation1960). Shallower curvatures flow uniformly around the meander bends and sharper curvatures separate the flow from the inner bank in turbulent eddies. These are general observations from a wide range of relationships between discharge, stream width, bed material and meander geometry in alluvial streams (Leopold and Wolman 1957; Leopold and Langbein 1962). Reference reach stream surveys are required to provide the design details for bed material distribution, point bar dimensions, riffle rock spacing, inner and outer bank profiles, fish cover and riparian planting.
North Pine River trout restoration project
The North Pine River gathers water from shallow lakes on Duck Mountain, MB, and flows eastward down the Manitoba Escarpment to Lake Winnipegosis. The lower reaches of the stream cross horizontal beds of Cretaceous shale, bentonite, and limestone before reaching the foot of the escarpment (Klassen Citation1978). Intermittent reaches of meandering adult rainbow trout habitat occur in shallow cusps in the profile that were formed as the stream encountered more resistant bedrock layers. The bed and banks are formed in gravels, cobbles and boulders deposited in the lower section of the cusp. Groundwater seepage into the stream commonly occurs as the stream approaches the intersection with the underlying bedrock (Little and Sie Citation1976). Proven trout habitat meanders were fished, surveyed and sketched to obtain a typical trout habitat template (Figures). The dimensions agreed generally with published values for alluvial meanders (Leopold and Wolman Citation1960). The lower valley gradients in meander segments ranged from 0.015 to 0.02. Approximately one-third of the fall occurred in the riffle segments. A recirculating portion of the helical flow deposited sands and fine gravels on the toe of the point bar formed on the inside bend of the meander. A lag deposit of boulders and cobbles was scattered throughout the gravel bed reach.
Figure 31 Surveys and sketches of known rainbow trout habitats on the Duck and Porcupine segments of the Manitoba Escarpment were compiled to produce a meander template. The rate of meander migration was slow enough to allow trees on the outer bend to correct their vertical growth habit as the bank was slowly undercut.
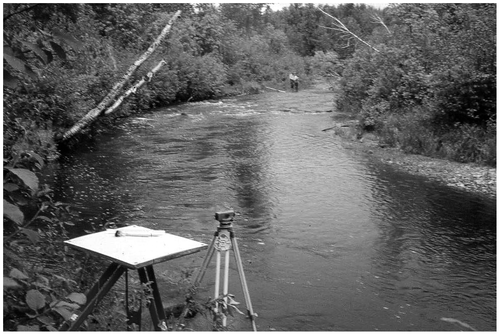
Figure 32 A typical plane table contour map of a trout habitat meander (Figure ). The average depth below the bankfull stage in the riffle segments is 0.5 m. The deepest point in the skewed meander cross-section near the outside bank is 1.5 m below bankfull.
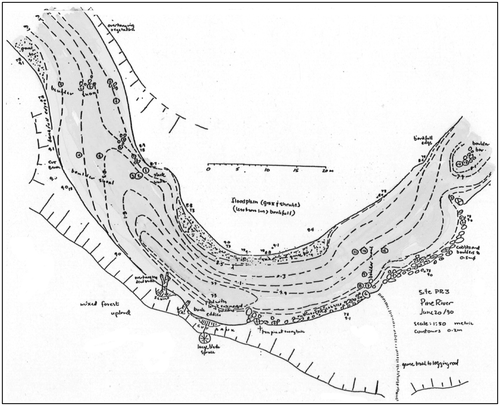
The reach selected for restoration was one of several crossings on Manitoba Provincial Trunk Highway (PTH) 360 where the channel had been straightened and aligned to run at an angle through a clear span bridge. A meander was added above and below the crossing to align the channel squarely with the bridge (Figure ).
Figure 33 New meanders in the North Pine River, MB, were designed to align the flow with the highway bridge and restore adult rainbow trout habitat.
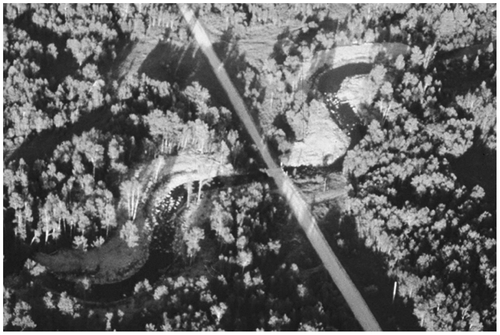
The riffle height was 0.32 m based on the specific energy of the bankfull discharge at the flood stage in the approaching pool. The riffles were spaced at 60 m, approximately six times the excavated bankfull width (Figure ).
Figure 34 The plan and profile of the North Pine River restoration reach is based on alluvial river dimensions and surveys in proven rainbow trout habitats in the Duck and Porcupine segments of the Manitoba Escarpment.
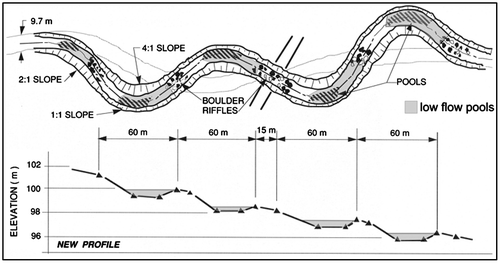
The path of the new channel was cleared through the floodplain and excavated in the winter of 1990/91. The elevations followed the undulating profile of run, pool, and riffle segments. The depth of the channel ranged from 0.5 m in riffles to 1.5 m in the pool, mimicking the general shape of the bed shown in Figure . The riffle segments were paved with boulders, cobbles, and gravels set aside as the excavation took place (Figure ).
Figure 35 The North Pine River meandering channel was excavated in the floodplain mimicking the cross-sections and undulating profile of runs, pools and riffles observed in natural sites. The riffles were paved with cobbles and boulders set aside as the excavation progressed.
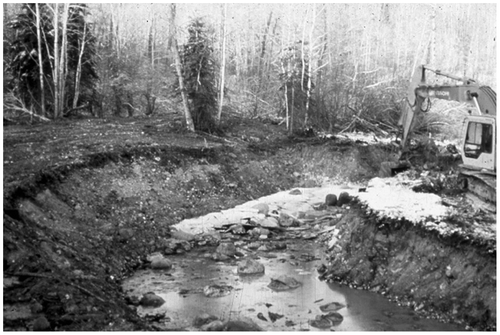
Spring flood discharges completed the channel configuration. Fines were flushed from the riffle surface and deposited in new point bars in the meander bends by the recirculating flow (Figure ). Creel surveys of the new meanders found rainbow trout in numbers similar to natural sites one year following construction. Images of the reach 10 years later in 2010 show a slight progression of the upstream meander.
Case 4. Structure-specific riffles for fish passage
Upstream pool stages may be set to overcome fish blockages and restore low flow continuity. There is widespread use of fish-passable rock riffles to backflood culverts. The FishXing program (United States Department of Agriculture 2012) provides a convenient method to reiterate tests of alternate discharges and crest elevations for a variety of fish species. A rating curve for the riffle below the obstruction is developed and various crest elevations are tested until fish passage criteria are met. Several riffles may be added downstream to enable fish to reach the highest pool in stages (Figure ). The channel in the stepped reach may require berms or dykes to contain the design flood discharges.
Figure 37 Two riffles and pools lead up to a riffle constructed in the stilling basin of the Dominion City dam on the Roseau River, MB, in 1992. A wide range of freshwater fish passes over the dam (Gaboury et al. Citation1995).
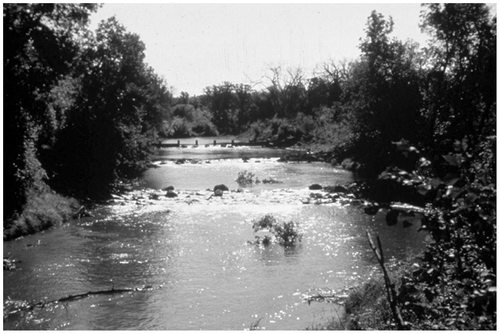
Similarly, fish passage through a drop structure may be achieved by backflooding the stilling basin to create a launching pool and to decrease the extent of non-swimmable air-entrained flow (Figures).
Figure 38 The shallow bedrock tailrace of the Sakinaw Lake, BC, dam was backflooded in three stages from the estuary to create a launching pool for sockeye and coho salmon in 1997. The riffles and infill materials were built with materials collected on the backshore of the marine beach. There is only barge access to the site.
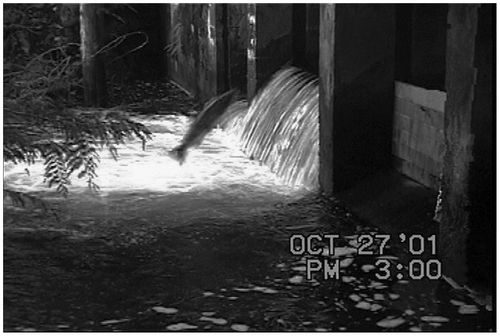
To avoid changing the dam rating curve the maximum stage of the backflooding pool is set by adjusting the riffle elevation and width so that the maximum design flood discharge remains critical on the dam crest (Figure ).
Figure 39 Flow over the upper riffle crest below the Sakinaw Lake dam in a peak flood event. The tailrace is backflooded but the dam rating curve has not changed. The smooth parabola of critical flow can be seen on the dam crest in the background.
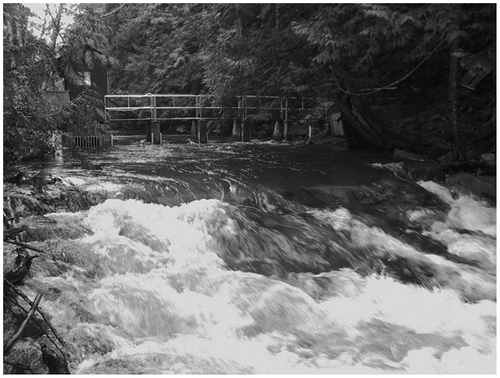
Dam backflooding may also ease salmonid fish passage over moderately high overshot gates. The depth of the pool below the gates is 20% or more than the height of the jump required to clear the gates (Stuart Citation1962). For example, a 2-m high riffle was constructed below the stilling basin of McIntyre Dam on the Okanagan River, BC, to create the launching pool for Columbia River salmon and steelhead (Figure ). The gates are set to limit the maximum jump to 1.8 m during the spawning run (Figure ) (Rivard-Sirois et al. Citation2013). Salmon and steelhead jump over the gates at moderate discharges, providing the flow is distributed thinly across all the gates to minimize aeration in the pool below and the impact on fish that launch through the nappe (Figure ).
Figure 40 The McIntyre Dam riffle was constructed in layers and compacted by the tracked backhoe. There is no cutoff wall. The height and width of the riffle is adjusted to allow the maximum flood capacity to pass through the dam with all the gates fully open without a backwater effect.
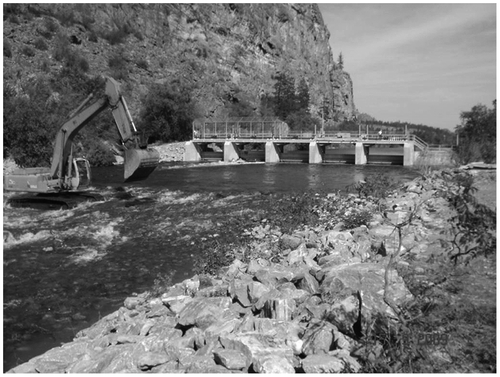
Figure 41 A schematic view of the riffle and pool backflooding the McIntyre Dam stilling basin to create a launching pool for Columbia River salmon and steelhead on the Okanagan River, BC. The maximum height for the overshot gates during the spawning run is 1.8 m.
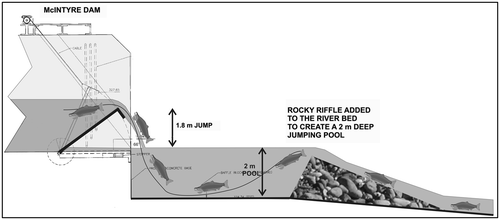
Figure 42 Columbia River salmon and Steelhead trout launch over the McIntyre Dam gates by accelerating upwards in the impounded pool as they approach the dam (photo R. McLean).
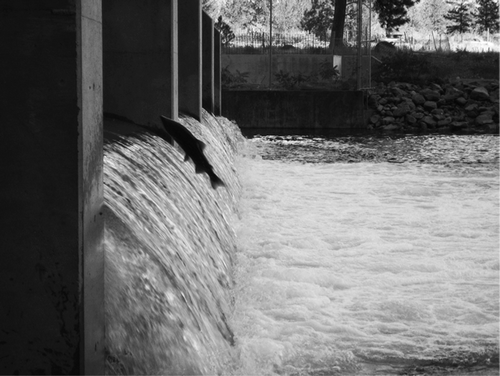
Pool and riffle fishways around dams are built with small-scale constricted width riffles. The length, width and drop in the riffle segment are adjusted to accommodate the burst speed of the fish species in transit. The riffles are narrowed to create resting areas in backwater eddies on either side of the pool segment. The fishway may be added to earthfill dams by running the fishway on additional fill compacted on the downstream face with an underlying seepage cutoff layer (Figure ) (Gaboury et al. Citation1995).
Figure 43 The pool and riffle fishway on the Rapid City, MB, water supply dam on the Little Saskatchewan River was constructed in 1992. A wide range of freshwater fish burst over the short riffles and pause in the deeper pools as they ascend the ladder (Gaboury et al. Citation1995). A horizontal pipe (right of figure) passes under the dam crest to the reservoir.
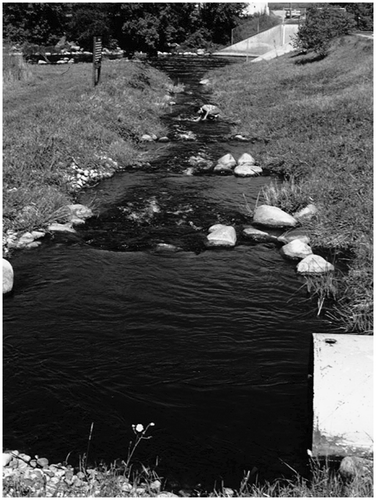
Discussion
The projects presented here illustrate the dimensions and construction of rock riffles designed with conventional open channel hydraulic methods commonly used in engineering practice. All of the relationships can be found in Chow (Citation1959) with particular reference to specific energy curves (Chapter 3) and the design of energy-dissipating structures (Chapter 15). More recent references include updated design and modelling techniques, for example Chang (Citation1988), Chanson (Citation1994) and Sturm (Citation2001). Significant research advances have been made in reproducing two- and three-dimensional flow patterns in bends and around obstructions (Miller Citation1995; Booker et al. Citation2001; Smith et al. 2011).
The stability of rock riffles is based on the relationship between the shear stress of the design flood and the minimum size of stable rock paving the surface. Smaller rock and gravels may be compacted to form the core of the riffle. If the downstream face of the riffle is ruggedly covered with larger emergent cobbles and boulders, the maximum shear stress occurs near the crest of the riffle because the downslope flow is broken into local chutes, pools and hydraulic jumps. If the riffle is smoothly paved, the shear stress occurs further down the face as the flow accelerates. Both cases can be modelled as backwater curves. Hydraulic roughness values observed in shallow rock-filled stream channels may be applied to riffles with emergent cobbles and boulders. In the rugged riffle projects described here, the maximum shear stress at the crest was conservatively estimated by assuming the critical flow depth had abruptly plunged to follow the slope of the riffle face. None of the riffles constructed over the last three decades have washed out. A few have had additional rock added to the riprapped banks if they were not fully covered initially.
The dimensions of riffles, pools and meanders are largely compiled in alluvial rivers flowing in valley bottoms that have been created by the present rivers and climate regime. In Canada, the designs must be tailored to fit a variety of channel dimensions and valley materials formed in the post-glacial drainage network. In all of the cases cited here, reference reach surveys were required to determine the size, spacing and form of the restoration works. There are obstacles to this approach:
(1) Limited restoration project boundaries.
Surveys and research beyond the reach to be restored are required to develop reference reaches for the distribution of bed materials and the downstream hydraulic geometry (van Duin and Garcia Citation2006). This requires a rare research and out-of-project survey and travel component in a restoration contract.
(2) Unknown post-glacial channel hydraulics.
The hydraulics of Canadian streams with boulder lag deposits and subdivided cross-sections are largely unknown. Under low discharge conditions, hydraulic roughness values may be four times greater than the flood stage values predicted in alluvial channels. Designing for low discharge conditions is critical to the persistence of fish habitats in ice-covered months and midsummer droughts (Davar et al. Citation1996; Jowett Citation1997). The valley bottomland characteristics vary widely in Canadian post-glacial landscapes. All of the riffle projects occurred in catchments affected by glaciation, for example in meltwater channels and deltas, alluvial fans and abandoned beaches of post-glacial lakes.
There are opportunities to compile hydraulic guides, stream characteristics and habitat features in a range of Canadian rivers adjacent to federal and provincial gauging stations (Kellerhals et al. 1972; Hicks and Mason Citation1991; Collins et al. Citation2012; MacCulloch and Whitfield Citation2012). However, there is presently no funding, nor is there a central repository for the data. Some of the benefits would be:
(1) The application of hydrometric records without additional gauging stations.
The hydraulic characteristics of a wide range of discharges using observed channel roughness values could be transferred to un-gauged streams and rivers with similar channel geometries and hydrologic characteristics to improve the prediction of flood levels and critical mid-winter and low-level drought conditions.
(2) Monitoring the effects of catchment and climate change on river regimes.
Reference reach data serve as a baseline for assessing land use and climate change effects on the stability, geometry and regime of smaller streams and rivers. In many cases, these will be the first to react to changes in land and runoff conditions in small catchments
(3) Integrating fisheries and recreation requirements with discharge records.
The reference reach data and reach photographs provide the real-time integration of discharge, fish habitat and recreational opportunities in a typical river reach rather than a single gauging station control section.
Acknowledgements
The projects selected in this paper were built from sketch designs accepted by many government and private agencies. Three decades ago, the first packed rock riffles without cutoff walls were built on faith with the Wilson Creek Headwater Control Committee (G.H. MacKay), Manitoba Habitat Heritage Corporation (D. McMaster) and Manitoba Fisheries Branch (M.N. Gaboury). Many of the later projects arose from graduate student research projects that linked hydraulics and fish habitats at the University of Manitoba, Simon Fraser University and the University of New Brunswick. Unpublished observations of fish response to the restoration and fish passage projects were generously given by M. Erickson and K. Kansas (Manitoba Fisheries Branch) and G. McBain (Canada Fisheries and Oceans). There is nothing unique in the “newbury riffles” cited in many publications. Everything is copied from nature.
References
- Booker, D. J., D. A. Sear, and A. J. Payne. 2001. “Modelling Three Dimensional Flow Structures and Patterns of Boundary Shear Stress in a Natural Pool-Riffle Sequence.” Earth Surface Processes and Landforms 26 (5): 533–576.
- Bower, S. S. 2007. “Watersheds: Conceptualizing Manitoba’s Drained Landscape, 1895–1950.” Environmental History 12 (4): 796–819.
- Chang, H. H. 1988. Fluvial Processes in River Engineering. New York: Wiley.
- Chanson, H. 1994. Hydraulic Design of Stepped Cascades, Channels, Weirs and Spillways. New York: Pergamon.
- Chow, V. T. 1959. Open Channel Hydraulics. New York: McGraw-Hill.
- Collins, M. J., J. R. Gray, M. C. Peppler, F. A. Fitzpatrick, and J. P. Shubauer-Bergian. 2012. “Developing a National Stream Morphology Data Exchange: Needs, Challenges and Opportunities.” EOS Magazine Forum 93 (20): 195.
- Davar, K. S., S. Beltaos, and B. Pratte. 1996. A Primer on Hydraulics of Ice Covered Rivers. Ottawa: Hydrology Section, Canadian Geophysical Union.
- Gaboury, M. N., R. A. Janusz, and K. E. Broughton. 1997. “Stream Channel and Riparian Rehabilitation in the Lake Dauphin Watershed, Manitoba.” Journal of Water Quality Research 32 (2): 257–272.
- Gaboury, M. N., and M. McCullough. 2002. Fish Habitat Designs for East Vancouver Island Watersheds. Sydney, BC: Conservation Foundation Report.
- Gaboury, M. N., R. W. Newbury, and C. M. Erickson. 1995. Pool and Riffle Fishways for Small Dams. Winnipeg: Manitoba Department of Natural Resources Fisheries Branch Report.
- Gregory, K. J., A. M. Gurnell, C. T. Hill, and S. Tooth. 1994. “Stability of the Riffle-Pool Sequence in Changing River Channels.” Regulated Rivers 9 : 35–43.
- Harper, D. E., E. Ebrahimnezhad, and F. Climente. 1998. “Artificial Riffles in River Rehabilitation: Setting Goals and Measuring Success.” Aquatic Conservation 8 (1): 5–16.
- Hicks, D. M., and P. D. Mason. 1991. Roughness Characteristics of New Zealand Rivers. Eaglewood, CO: Water Resources Publications LLC.
- Jowett, I. G. 1983. “A Method for Objectively Identifying Pool, Run and riffle Habitats from Physical Measurements.” New Zealand Journal of Marine and Freshwater Research 27 : 241–248.
- Lane, E. K. 1955. “Design of Stable Channels.” American Society of Civil Engineers Transactions 120 : 1234–1279.
- Leopold, L. B., and M. G. Wolman. 1960. “River Meanders.” Geological Society of America Bulletin 71 (6): 769–793.
- Leopold, L. B., M. G. Wolman, and J. P. Miller. 1964. Fluvial Processes in Geomorphology. San Francisco: Freeman.
- Little, J., and D. Sie. 1976. Groundwater Availability Map Series: Duck Mountain Area (62N). Winnipeg: Manitoba Department of Natural Resources.
- MacCulloch, G., and P. H. Whitfield. 2012. “Towards a Stream Classification System for the Canadian Prairie Provinces.” Canadian Water Resources Journal 37 (4): 311–332.
- Mollard, J. D., and J. R. Janes. 1984. Air Photo Interpretation of the Canadian Landscape. Ottawa: Queens Printer.
- Newbury, R. W. 2010. Stream Restoration Hydraulics: Project Casebook. Fredericton: Canadian Rivers Institute, University of New Brunswick.
- Newbury, R. W., and M. N. Gaboury. 1993. “Exploration and Rehabilitation of Hydraulic Habitats in Streams Using Principles of Fluvial Behaviour.” Freshwater Biology 29 : 195–210.
- Newbury, R. W., and M. N. Gaboury. 1994. Stream Analysis and Fish Habitat Design. Winnipeg: Manitoba Habitat Heritage Corporation.
- Rivard-Sirois, C., K. Alex, S. Folks, and N. Audy. 2013. Providing Fish Passage at McIntyre Dam – Okanagan Sockeye Adult Monitoring (2009–2012). Kelowna: Okanagan Nation Alliance Fisheries Report.
- Sturm, T. W. 2001. Open Channel Hydraulics. New York: McGraw-Hill.
- Thomson, D. W. 1967. Men and Meridians: The History of Surveying and Mapping in Canada. Ottawa: Queens Printer . 370 pp.
- van Duin, B., and J. Garcia. 2006. “Impacts of Urbanization on West Nose Creek – A Canadian Experience.” Water Science and Technology 53 (10): 237–245.
- Vannote, R. L., G. W. Minshall, K. W. Cummins, J. R. Sedell, and C. E. Cushing. 1980. “The River Continuum Concept.” Canadian Journal of Fisheries and Aquatic Science 37 : 130–137.
- Walker, D. R., R. G. Millar, and R. W. Newbury. 2004. “Energy Profiles Across Constructed and Natural Riffles.” Journal of Hydraulic Engineering 130 (3): 199–207.