Abstract
The sediment budget of the lower Fraser River provides the basis to understand the net sedimentary changes in the river and delta distributary channels and sediment delivery to the Strait of Georgia. Currently, it is not possible to construct the contemporary sediment budget because the input and output volumes of sediment to the sand-bedded reach of the river are unknown. In this study, the results of a sediment sampling program at Mission, British Columbia, designed to explore sediment delivery into the sand-bedded reach and delta over an annual hydrograph, are presented. The 2010 daily sediment load, in a year with a low freshet, varied between 2700 tonnes in April and 100,732 tonnes in late June. The annual sediment load was ~7.2 M t. About 70% of the 2010 sediment load transport was silt- and clay-sized material. Most of the sand transport occurred during the high-flow months of May, June and July. The threshold discharge for significant sand suspension lies between 5000 and 5700 m3/s. In 2010, 94% of the total load and 97% of the sand load were moved when the river discharge was above 1000 m3/s. A strong hysteresis for silt-clay, but a weaker effect for sand, was found. This pattern of transport is consistent with observations between 1966 and 1986 by the Water Survey of Canada. The 2010 sediment budget is on the low side of historically measured annual suspended sediment loads, raising the possibility that recently there may have been a secular shift in the suspended sediment influx into the estuary.
Le bilan des sédiments dans le cours inférieur du fleuve Fraser donne les bases pour comprendre les changements touchant le fleuve, ses défluents et le débit des sédiments jusqu’au Détroit de Géorgia. Nous n’allons pas à même construire ici le bilan contemporain des sédiments parce que nous ne connaissons ni le volume de sédiment arrivant dans la portion du fleuve comportant un lit sableux, ni celui le quittant. Nous présentons d’abord des résultats d’un programme de collecte d’échantillons de sédiment à Mission, British Columbia, conçu pour étudier le débit des sédiments dans la portion du fleuve au lit sableux et dans son delta réalisé sur une période d’un an. La charge quotidienne des sédiments en 2010, année avec de faibles crues, variait entre 2700 tonnes en Avril et 100 732 tonnes en fin-Juin. La charge annuelle de sédiment était d’environ 7,2 millions de tonnes. Environ 70% de la charge de sédiment transportée en 2010 était dans les classes de granulométrie limon et argile. La plupart du transport des sables a lieu durant les mois à haut débit, i.e., mai, juin et juillet. Le seuil du débit afin de rencontrer une proportion significative des sables en suspension se situe entre 5000 et 5700 m3/s. En 2010, 94% de la charge totale et 97% de la charge des sables ont été déplacé lorsque le débit du fleuve était supérieur à 1000 m3/s. Nous observons une forte hystérésis pour les limons-argiles mais un effet bien plus faible pour les sables. Ce modèle de transport est en accord avec les observations réalisées entre 1966 et 1986 par les Relevés hydrologiques du Canada. Le bilan des sédiments de 2010 se situe dans le bas de la fourchette des mesures historiques annuelles des charges de sédiments, soulevant ainsi la possibilité qu’il y ait eu récemment un important changement de l’afflux des sédiments en suspension dans l’estuaire.
Introduction
The Fraser River in British Columbia (BC) delivers significant amounts of sediment to the Fraser Estuary and Delta. In the distal, sand-bedded portion, these sediments range in size from clay and silt to coarse sand with a small quantity of granule gravel. The sand load is important because it forms the channel bed; sand exchange between the bed and the active sediment load sets the downstream reach sediment budget, and so is related to channel stability. The silt-clay load is wash load (material that passes directly through the reach), yet it contributes to both the physical and ecological development of the Fraser Delta and is an important water quality element. For many years, the main channel of the Fraser River has been dredged below Port Mann to maintain a deep-water shipping channel, drastically altering the sediment budget in the delta’s Main Arm. The sediment budget provides a long-term perspective of the net changes in the river and delta distributary channels and in sediment delivery to the Strait of Georgia.
The sediment budget of the river between Mission – near the head of the tidally affected, sand-bed reach – and Sand Heads, the mouth of the Main Arm of the river (Figure ), is defined as:(1)
Figure 1. Study site location just upstream of the Mission Railway Bridge, Mission, British Columbia (data source: Google Earth, accessed October, 2011).
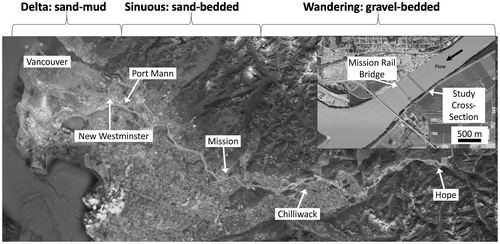
where is the net change in sediment stored within the reach, Vin is the volume of sediment inflow to the reach at Mission during the designated time period,
is the volume of sediment outflow from the reach at Sand Heads during the period,
is the volume of sediment dredged and removed from the reach during the time period and Vspoil is the volume of dredged material disposed of in the reach (i.e. dredged material that was not actually removed from the river). At present,
and
, almost all of which are taken and disposed of downstream from Port Mann, are known on an annual basis. However,
is unknown and current estimates of
(Northwest Hydraulic Consultants Citation2002) are based on historic gauging that may no longer represent accurate information. This prevents the confident determination of the current annual sediment budget for long-term management purposes.
The historic information consists of sediment rating curves built on the Water Survey of Canada’s (WSC) 1966–1986 measurements. According to the WSC’s sediment transport records, analyzed by McLean et al. (Citation1999a), the Fraser River at Mission (Station No. 08MH024) has an annual total suspended load, on average, of 17 million tonnes per year (Mt/a). The total sediment load is broken down by grain size in Table . About one third, 6.1 × 106 Mt/a, is suspended sand, and half of that (3.1 Mt/a) is sand finer than 0.177 mm (McLean et al. Citation1999a). This fine sediment is generally absent from the river bed, indicating that a portion of the sand load is wash load. Another way to consider the load is as total bed material load – bed load and suspended sand coarser than 0.177 mm that is carried in intermittent suspension. This bed material load is 3.2 Mt/a, accounting for 19% of the total. All but 5% of it is transported in intermittent suspension near the bed (McLean et al. Citation1999a).
Table 1. Mean grain size specific sediment load in the Fraser River at Mission (McLean et al. Citation1999a, based on Water Survey of Canada (WSC) data for 1966–1986).
Church (Citation2010) considered that the ~3.2 Mt/a of sand > 0.177 mm delivered to the Mission-Port Mann reach of the river is an appropriate estimate for a much longer period before measurements commenced. He argued that there was no significant change in storage in this reach, so that volume was delivered to the Fraser Estuary, where a small amount of aggradation has been recorded (~0.2 Mt/a). This means that ~3.0 Mt of sand was delivered to the delta front annually. Deepening the shipping channel to increase navigation drafts has led to an upstream migrating knickpoint in the river bed that is causing channel bed degradation and lower water levels that are progressing upstream into the Mission-Port Mann reach (McLean et al. Citation2006). The degradation is thought to be delivering an additional ~1.0 Mt/a of sand to the estuary. To maintain the shipping channel, 2.9 Mt/a needs to be dredged from the river, so that only about 1.3 Mt/a of sand > 0.177 mm is delivered to the delta front today, reducing the rate of delta front advance and limiting wildlife habitat (Church Citation2010). The speculative nature of these estimates highlights a need for the development of a securely founded, contemporary sediment budget for long-term sediment management purposes. The first step in implementing the sediment budget is to establish sediment inflow (Vin) to the estuary at Mission, BC.
As part of a project designed to investigate hydroacoustic methods of establishing Vin, an examination of suspended sediment transport at Mission during 2010, obtained by traditional sampling, is presented. Answers to the following questions are sought:
What is the current seasonal distribution of suspended sediment flux in the Fraser River at Mission?
What is the contemporary annual sediment load of the river at Mission and how does it compare to historical records?
Can available methods be applied to model suspended sediment flux in the reach?
Methods
Measurement site: Fraser River at Mission, British Columbia
The Fraser River drains parts of the humid Coast Range, the subhumid Interior Plateaux and the Columbia and Rocky Mountains of BC for a total basin area at Mission of 228,000 km2. The runoff pattern is dominated annually by the spring snowmelt in May–June. High flow occurs throughout late May, June and early July, and flow recedes in August and September (Figure a). The mean annual flow at Mission is 3410 m3/s, the mean annual flood is 9790 m3/s and the 1894 flood, in which flows are estimated to have reached 17,000 ± 1000 m3/s (Northwest Hydraulics Consultants Citation2008), is the historic flood of record. The largest flood during the period of sediment transport measurements occurred in 1972 and had a peak flow of 14,400 m3/s (McLean et al. Citation1999a).
Figure 2. (a) Hydrographs for 2010 for Fraser River at Mission (Water Survey of Canada [WSC] Station 08MH024) and Hope (WSC Station 08MF005), British Columbia, and the daily mean water level at Mission. Note: scales for discharge and water level are independent. The WSC rating curve discharge at Mission is calculated only when flow at Hope and the Harrison River, a tributary between Hope and Mission, exceeds 5000 m3/s due to the tidal influence on water levels. (b) Continuous water level record at Mission in 2010 showing the seasonally varying tidal influence. The dotted vertical lines highlight dates of the sampling campaigns (data source: Environment Canada Citation2011, accessed March 2011).
![Figure 2. (a) Hydrographs for 2010 for Fraser River at Mission (Water Survey of Canada [WSC] Station 08MH024) and Hope (WSC Station 08MF005), British Columbia, and the daily mean water level at Mission. Note: scales for discharge and water level are independent. The WSC rating curve discharge at Mission is calculated only when flow at Hope and the Harrison River, a tributary between Hope and Mission, exceeds 5000 m3/s due to the tidal influence on water levels. (b) Continuous water level record at Mission in 2010 showing the seasonally varying tidal influence. The dotted vertical lines highlight dates of the sampling campaigns (data source: Environment Canada Citation2011, accessed March 2011).](/cms/asset/7d55f4b7-d1df-4d2e-8bba-c5a06c19f409/tcwr_a_942105_f0002_b.gif)
At the eastern end of Sumas Mountain, 13 km upstream from Mission, the Fraser River changes from a wandering gravel-bedded river to a single-thread, sand-bedded channel. A 10-times reduction in flood water surface gradient occurs from the gravel-bedded to the sand-bedded reaches of the river (McLean et al. Citation1999a). An abrupt gravel-sand transition occurs in the channel, which also marks the upstream limit of tidal influence (Venditti et al. Citation2010). At Mission, just downstream from Sumas Mountain, the tidal range varies from a few centimetres during the freshet to over 1 m during the highest winter tides (Figure b). Although the effect on river stage is minimal at high flow, the effect on flow velocity remains substantial as the rising tide induces a strong backwater effect. However, salt water at low flow reaches only as far upstream as the head of the delta at New Westminster (Figure ).
This study focuses on Fraser River at Mission, the measurement section located 240 m upstream of the Mission Railway Bridge (Figure ), which is the site of WSC Mission gauge (Station No. 08MH024) and historic WSC sediment measurements. Here, the river has a mean annual flood width of approximately 540 m and mean depth of 12.6 m (McLean et al. Citation1999a). At mean annual flow level, the width is 518 m and the mean depth is 9.4 m. Discharge at Mission is measured several times per year by the WSC. Those measurements contribute to a rating curve that is deemed accurate when the combined discharge at the Hope, BC, hydrometric gaging station (Station No. 08MF005) and the Harrison River hydrometric gaging station (Station No. 08MG022) exceeds 5000 m3/s.
Field observations
The sampling program consisted of six campaigns to capture the rise, peak and fall of the 2010 hydrograph (Figure ). Observations of suspended sediment concentrations, depth and velocity, and bed material were obtained at five stations along a cross-section of the river (Figure ). Suspended sediment measurements were made via conventional bottle-sampling methods when water levels were approaching low tide to capture the maximum current and when flow was least affected by tides. A United States Geological Survey (USGS) P63 sampler was used to collect point and depth-integrated suspended sediment samples in five vertical profiles (Figure ). The sampler was deployed from a 6-m launch fitted with a davit, a motorized winch and a manual USGS B-reel that was used to measure depths and trigger the P63 sampler. The P63 is a cable-launched isokinetic sampler with a valve that opens and closes when triggered by a 48-volt battery. Samples were collected for approximately 30 to 120 seconds, depending on the flow, into a quart-sized bottle (0.95 L). At each of the verticals, point samples were collected at five relative depths: 0.1h (i.e. near-bed), 0.2h, 0.4h, 0.6h and 0.8h, where h is flow depth at the vertical, following standard WSC sampling procedure. A depth-integrated sample was also obtained at each vertical, with two samples collected at the centre of the channel (Profile 3; Figure ). All data presented herein are based on the point-integrated sampling unless otherwise indicated. This procedure was less strictly followed during low flow conditions in April, when sometimes only four points in the water column were sampled. During the 27–28 June campaign, only four vertical profiles (Profiles 1, 2, 3, 4) were collected because the winch for the electric motor broke.
Figure 3. Cross-section of Fraser River at Mission indicating sampling locations and panels for computing section totals. Bed elevation is relative to the Canadian geodetic datum. Transects were surveyed in 2008 by Public Works Canada immediately upstream and downstream of the vertical profiles. Peak flow for 2010 was 7620 m3/s at 22:50 Pacific Standard Time (PST) on 28 June.
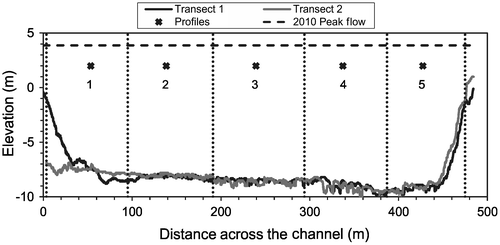
During each sampling campaign, river discharge was measured using a Teledyne RDI downward-looking 1200 kHz Workhorse Rio Grande Acoustic Doppler Current Profiler (ADCP), except on 28 June when peak flows had depths beyond the range of the 1200-kHz instrument and so a 600-kHz instrument was used. A supplementary measurement was also taken on 2 November 2010. For each measurement, four cross-stream transects were obtained as the tide was approaching low water, and then averaged to give the values reported here.
Additional velocity profiles and depth measurements were obtained using a 600-kHz ADCP at each profile location during each sampling campaign for the purpose of suspended sediment flux calculations. The ADCP was deployed from the 6-m launch, approximately 2 m away from the suspended sediment sampler (P63) on the opposite side of the vessel. Positioning of the ADCP was accomplished using a Trimble real-time kinematic (RTK) global positioning system (GPS).
Bed material samples were collected with a pipe dredge at the approximate location of each vertical profile during all sampling periods, except for 15–16 April. Samples were dried, weighed and sieved at ½ phi increments. Particle-size distribution statistics were calculated using GRADISTAT Version 4.0 (Blott and Pye Citation2001a, Citation2001b).
Sample processing
Samples that could not be processed promptly were treated with 1 mL of 0.4 g/L copper sulphate solution to inhibit growth of organic material. Each suspended sediment sample was processed using a Sequoia Scientific laser in-situ scattering transmissiometry (LISST-Portable) instrument that uses laser diffraction to calculate the grain-size distribution. Water-sediment samples were transferred to the instrument’s measurement chamber and agitated during the measurement process. The LISST-Portable calculates the grain-size distribution in 32 logarithmically spaced bins from a lower limit of 1.9 μm to the upper limit of 381 μm. The data are provided as a volumetric concentration (μL/L) for each bin, the sum of which is the total sediment concentration in the sample. A Random Particle Shape Model (Agrawal et al. Citation2008) was used to calculate grain-size. The grain-size distribution provided by the LISST was used to separate the total filtered concentration into two size classes, sand and silt + clay.
The LISST-Portable measurement chamber capacity is 180 mL, so the samples were allowed to settle for several days (minimum 5 days) and the clear water on the top was siphoned off to reduce the water-sediment sample volume to 180 mL. The volume of water removed was recorded and used in the calculation of the sample total volume. The smallest particle size sensed by the LISST (1.9 μm) has a settling velocity of 1.24 × 10−6 m/s (Dietrich Citation1982). A 5-day settling period allows these fines to travel a distance of 54 cm, which is much greater than the depth of the water within the sample bottle (maximum 16 cm), assuring the settling time was sufficient.
Sediment concentration was also measured by filtering the water sample through a 47-mm diameter Whatman® glass microfibre filter (pore size of 1.6 μm), oven-dried and weighed. The entire sample, including the portion siphoned prior to the LISST analysis and the water and sediment that passed through the LISST, was filtered to provide a mass concentration per volume. This provided a second measure of total sediment concentration.
In order to examine the organic content, samples from selected vertical profiles were combusted. The vertical profiles were chosen to represent low, increasing and peak flows and to capture variation through the water column. Twenty samples were ashed at 375°C for a minimum of 16 hours and reweighed to obtain the difference between pre and post combustion.
Data analysis
Velocity profiles obtained from the downward-looking ADCP were averaged over the sampling time of each P63 bottle sample. The averaged profiles derived for each of the five (four in April) samples taken in a profile were then themselves averaged to obtain a mean velocity profile for each profile location. The grand average represents a 3–5-minute sampling of the velocity profile, depending on the time to take each suspended sediment sample. The grand average plots were compared against the logarithmic velocity profile for hydraulically rough flow:(2)
where u is the water velocity, u* is the shear velocity, κ = 0.41 is the von Kármán constant, z is the height above the bed and zo= ks/30 is the height of the plane of zero velocity. Shear velocity was estimated from the slope of the logarithmic fit to the velocity profile, m, where u* = mκ. The bed roughness, ks, was back-calculated using a known velocity, u(z), at a known height above the bed, z.
The vertical profiles of suspended sediment were compared against profiles calculated using the Rouse Equation:(3)
where c is suspended sediment concentration at a height z above the bed, ca is the concentration at z = a, a is a reference height with respect to the bed and Ro is the Rouse Number defined as:
(4)
where ws is particle settling velocity and β is the ratio of the fluid diffusion to sediment diffusion. If β < 1, a lag between the motion of sediment particles and the fluid occurs (this is certainly true for larger particles). It has been argued that values of β > 1 indicate that particle diffusion is affected by additional factors (e.g. centrifugal forces on the particle) (van Rijn Citation1993). The form of the Rouse profile given in Equations (Equation3(2) ) and (Equation4
(4) ) assumes that the vertical variation of diffusivity is parabolic as a consequence of the log-linear increase in velocity above the bed and the inverse linear relation between height above the bed and shear stress (van Rijn Citation1993). Other forms of the Rouse Equation can be derived by assuming alternate forms of the vertical fluid diffusivity profile, and hence the velocity profile. Checking the soundness of these assumptions is the reason why the velocity profiles are compared against Equation (2).
The value of Ro changes the vertical gradient of suspended sediment concentration for a given grain size, such that a smaller Ro (associated with finer sediment) yields a curve with a steeper slope – that is, a smaller gradient in sediment concentration throughout the water column. The distribution of suspended sediment becomes more uniform throughout the flow depth as Ro decreases, that is, particle settling velocity (dependent on grain size) decreases relative to u*, as is typical of finer sediment.
Rouse profiles were calculated for each of the 32 grain size ranges analyzed by the LISST-Portable. The particle settling velocity was calculated using the method of Dietrich (Citation1982). The 32 profiles were then summed and divided by 32 to give a total relative concentration that ranged from 0 at the water surface to 1 at the height of the reference concentration at a = 0.1h.
Unit suspended sediment flux was obtained for the full grain-size distribution (referred to as total suspended sediment), suspended sand and suspended silt-clay. At-a-point suspended sediment flux qs(z) was calculated as the product of u and c measured at each height above the bed. Sediment flux in a vertical profile qs-vert was calculated by summing qs(z) over the entire water column using:(5)
where dz is the portion of the water column that each value of qs(z) represents. To obtain a measure of cross-channel suspended sediment flux, the flux for each vertical, qs-vert, was multiplied by dy, the width of the panels 1 through 5, which is the portion of the channel that each vertical represents:(6)
An initial estimate of the annual sediment load was made by block-averaging the results of the individual total flux measurements as: (7)
where Δt represents the length of the integration period (daily for our calculations). A further estimate was made by forming a rating curve for the season from the data of the six field campaigns:(8)
with R2 = 0.90 and standard error of estimate 15,718 tonnes/day. Mission flow was calculated as the sum of Hope flow (08MF005) and Harrison River near Harrison Hot Springs (08MG013), except when Mission flows were available from the WSC rating curve. Correlation between this sum and Mission flows suggests that a further 350 m3/s should be added to the sum to account for contributions from Vedder River and minor tributaries. This value was tapered toward the beginning and end of the period. Annual load was estimated as the sum of the daily totals inferred from this equation.
Results
Flow in the cross-section
Figure a shows the 2010 measured discharge at Mission and Hope as well as water levels at Mission. Discharge follows the commonly observed hydrograph shape for the Fraser River with low flows in April to mid-May, rising flows in late May and early June, peak discharge during the 27/28 June sampling campaign, and decreasing flows through July and August. The 2010 freshet peaked at approximately 7600 m3/s, fifth lowest in a 40-year record and, of course, lower than the mean annual flood of 9790 m3/s.
Figure shows velocity profiles collocated with the suspended sediment sampling for low, rising and peak flows obtained from the downward-looking ADCP. Comparison of the velocity profiles against Equation (2) indicates that most are log-linear over the full depth. Profiles 1 and 4 at high flow are slightly kinked, which has been related to the presence of bedforms (e.g. Smith and McLean Citation1977; McLean et al. Citation1999b). However, the reason for the present kinks is not clear. Bedforms occur in the reach upstream of the cross-section, but have not been observed at the cross-section due to some sediment supply or unknown hydraulic limitation. At Profile 1, the bed material is not sand. This suggests that the kinks in the profile are due to spatial patterns of flow (convective acceleration/deceleration) and not bed roughness.
Figure 4. Velocity profiles for low flow (16 April; black), rising flow (19 May; grey), and peak flow (28June; black). Note that the velocity profiles were measured on 16 April, but the closest discharge value available was for 15 April . There is no Profile 3 on 15 April and no Profile 5 on 28 June. The data points are the measured velocities and the solid lines are calculated from the Law of the Wall (Equation 2).
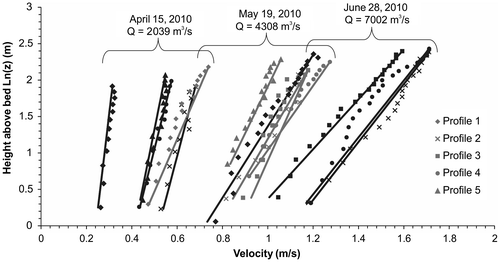
Changes in bed material
Bed material grain size is not uniform across the channel at Mission. Figure shows the median bed material grain size at each profile for sampling campaigns between May and August (no bed samples were obtained in the April campaign). Near the south bank, bed material is medium sand and there was virtually no grain size change through the freshet. Near the north bank, the bed material is significantly coarser and there are clear patterns of bed material grain size change. At Profile 2, the bed material is composed of coarse sand and there was coarsening, then fining through the freshet (Figure ). At Profile 1, where there were generally lower velocities, there was a shift in bed-material grain size from fine sand (~150 μm) early in the freshet to medium sand (~500 μm) prior to the peak flow and to a gravel bed after the peak. The fine sand present early in the season was carried away as washload in the channel at higher flows. This material appears to be deposited in the low-flow zone near the north bank at lower discharges and is entrained during high flows, revealing an underlying gravel bed.
Comparison of filtered and LISST-Portable sediment concentrations
To convert the LISST-Portable measures of volumetric concentration in μL/L to dry mass concentration, the instrument manufacturer recommends a conversion factor of 2.65, based on the characteristic density of clastic sediment (Sequoia Scientific 2010). However, linear regression between the filtered suspended sediment concentration (mg/L) and the volumetric LISST concentrations (μL/L), forced through the origin, suggests that the conversion factor is actually closer to 1.67 for this data set (Figure a). LISST-derived concentrations calculated with a conversion factor of 2.65 overestimate sediment concentrations observed during the freshet (Figure b). The 1.67 conversion factor overestimates sediment concentration during low flows (Figure a), but improves during rising flows in mid-May (Figure c). During peak flows in late June, the situation is the opposite, and the 1.67 conversion factor leads to an underestimation from the LISST (Figure e).
Figure 6. (a) Relation between filtered suspended sediment concentration (mg/L) and laser in-situ scattering transmissiometry (LISST)-Portable concentrations (μL/L). (b) Relation between filtered and LISST concentrations after conversion using densities 2.65 g/cm3 and 1.67 g/cm3; the relation derived from (a).
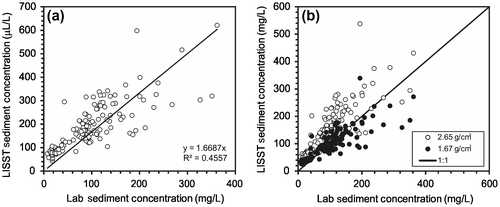
Figure 7. Profile 4 sediment concentration for (a, b) 15–16 April 2010, (c, d) 18–19 May 2010 and (e, f) 27–28 June 2010 sampling campaigns. Graphs a, d and g show filtered concentrations and laser in-situ scattering transmissiometry (LISST)-Portable concentrations calculated using conversion factors (CF) of 2.65 and 1.67. Graphs b, d and f are filtered silt/clay and sand concentrations discriminated using the LISST-Portable grain-size distributions. SSC, suspended sediment concentration.
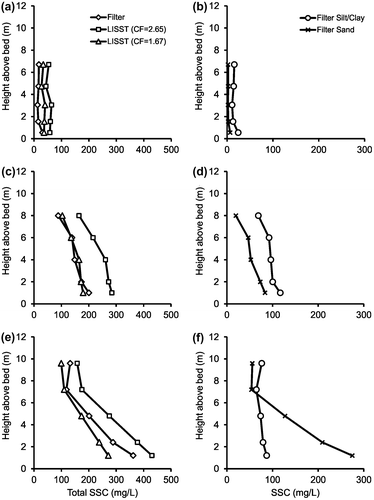
There are a number of possible reasons for the discrepancy between the conversion factors. The presence of particulate organic matter (POM) would reduce the effective density of the samples; however, combustion removed only approximately 5% of the mass, suggesting this is not the case. Another reason could be flocculation (Sequoia Scientific 2010), but flocculation is typically stronger in saline waters than in fresh water and is more prevalent for clay particles, whereas the fine fraction of sediment load in the Fraser is dominated by silt. Bias might be caused by sediment loss during processing through the LISST-Portable device, where particles may have been trapped and therefore not captured in the filter, but there was no obvious release of sediment during sample processing from the LISST, so this is unlikely. Elimination of these possible reasons suggests that the bias is real and that the 2.65 conversion factor suggested by the manufacturer is a calibration parameter that may deviate for different data sets. The fact that the 1.67 conversion factor over and underestimates concentrations at different flows may be a reflection of the dominant sediment source. At low flows, the load is dominantly silt. At higher flows, there is a significant sand fraction (McLean et al. Citation1999a). Due to the uncertainty surrounding the LISST mass concentrations, filter concentrations are used for the remainder of the calculations presented herein, but the grain-size distributions from the LISST are utilized.
Suspended sediment concentrations in the reach
Figure shows that total suspended sediment concentration (SSC) was dominated by silt and clay during low flows and that sand concentrations increased with higher flows. At low flow, total concentrations were low and there was little sand in suspension, except near the bed (Figure b). During rising (Figure d) and peak flows (Figure f), both silt-clay and sand concentrations increased substantially. Silt/clay concentrations were relatively uniform throughout the water column, whereas sand concentrations exhibited a distinct gradient, suggesting that bed material was suspended into the water column.
The median grain size (D50) of sediment in suspension varied over the freshet both across the channel and through the water column (Figure ). During the 15–16 April campaign, D50 ranged from 14–21 μm. During the rising flows sampled on 18–19 May and 27–28 May, median grain size was slightly larger, ranging from 15–47 μm, with the exception of a single sample on 28 May at Profile 3 taken at 0.2h, which had D50 of 101 μm. Samples from 7–8 June varied from about 21 to 162 μm, and during the peak flows recorded on 27–28 June ranged from approximately 21–201 μm. Suspended sediment during post-freshet flows on 3–4 August had D50 that ranged from 18–66 μm.
Figure 8. Suspended sediment grain size (D50) across the channel for each elevation above the bed. The dashed line signifies the sand-silt division (63 μm).
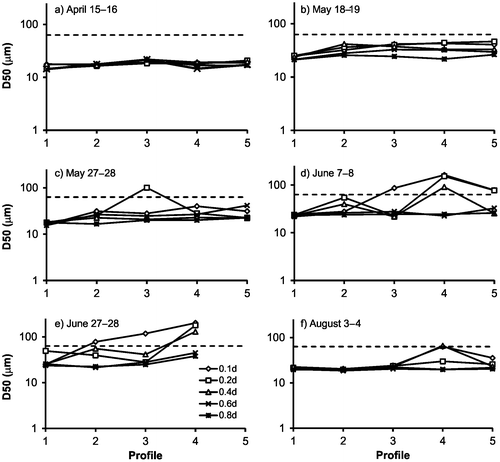
A cross-channel gradient in suspended sediment grain size became increasingly pronounced as flows increased (Figure ), the consequence of bed material suspension in part of the section. Median suspended grain size at Profiles 1 and 2 was always in the silt-clay range (< 63 μm), but at Profiles 3–5 it rose into the fine sand range near the bed during the high flows of June. This suggests that transport is washload dominated near the north bank, even at high flows, but that bed material mobilization occurs at higher flows on the south side of the channel.
Figure compares calculated Rouse profiles with the measured concentration profiles. Observed sediment concentration gradients are generally well duplicated by the Rouse calculations – granted that the sample data are subject to substantial short-term fluctuations – but there are often substantial differences in absolute concentration. Figure shows the ratio of the depth-averaged concentrations (z = a to z = h) between the Rouse profile and the measurements. Suspended sediment flux at Profile 2 is well predicted across all flows. Profiles 1 and 5 (the two near-bank profiles) overpredict concentrations at low flows and underpredict concentration at higher flows. Concentrations at Profiles 3 and 4 are overpredicted by the Rouse equation at all sampled flows. The ratio of fluid to sediment diffusivity in Equation (Equation4(4) ) (β) was varied in an attempt to achieve better fits to the data. However, the values of β required to achieve better fits did not vary systematically, insofar as the optimal β value was different for different profiles during the same sampling campaign. Furthermore, a grain-size dependent value of β calculated from van Rijn (Citation1984):
(9)
Figure 9. Suspended sediment concentration profiles. C/Ca is the ratio of suspended sediment concentration, C, at a height z above the bed to a reference concentration, Ca, at height a above the bed. h is flow depth. Dashed lines are filtered concentrations and solid lines are the Rouse Equation.
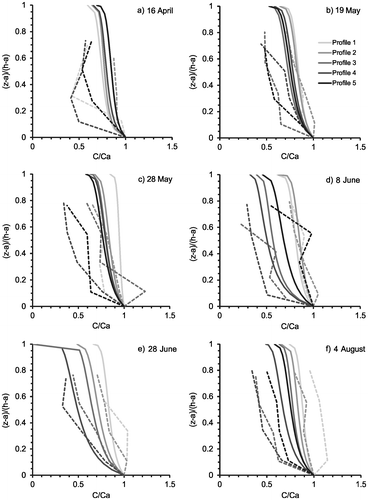
Figure 10. The ratio of the depth-averaged Rouse profile concentration estimates to the measured concentrations in the reach. Depth averaging is from the reference height z = a to the water surface. Profile 5 was not obtained on 28 June.
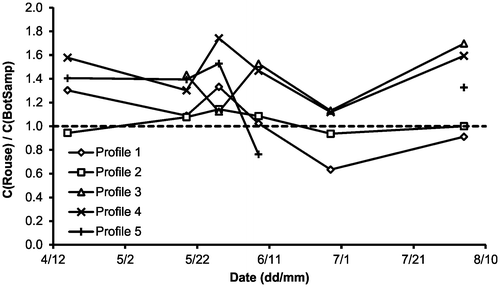
had little effect on the concentration profiles because β only deviates from 1 when 0.1 < ws/u* < 1, which happens for the coarsest grain sizes in the data set. There is no obvious reason for these outcomes: sampling variability on the temporal scale of bottle sampling might explain random variation, but not systematic bias. The appropriateness of the Rouse profile, at least for describing the profile of suspended sediment, is accordingly thrown into doubt.
Suspended sediment flux at Mission in 2010
The suspended sediment load at Mission is composed primarily of silt/clay-sized sediment with a seasonally significant portion of sand. At low flows, ~80% of the load was silt/clay sized material, declining to ~60% of the total load at peak flow. During the rising and falling limbs of the hydrograph, silt-clay made up ~70% of the total load. The pattern suggests that sand sourced from the local channel bed makes up an increasing, though modest, fraction of the total load as flows increase. Suspended sediment flux closely followed discharge throughout the freshet, although there were two peaks in suspended sediment flux, one minor peak in late May and the major one at the end of June (Figure a). The minor peak in late May was caused by an increase in the silt-clay concentrations, presumably due to increasing washload contributions from the watershed.
Figure 11. (a) Suspended sediment flux (total, silt/clay and sand) and measured discharge at Mission. The points are joined with lines to show the observed seasonal pattern. (b) Daily suspended sediment load over a range of discharge conditions. Points are joined to illustrate hysteresis. Arrows show direction of hysteresis loop.
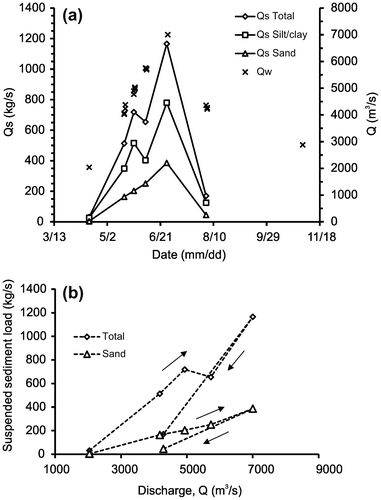
The sedigraph at Mission shows significant clockwise hysteresis in the total suspended sediment load with discharge (Figure b). Sediment concentrations are larger on the rising limb of the hydrograph than on the falling limb. Although this hysteresis is based on one data point, comparison to the historical record from the WSC for 1984, 1985 and 1986 shows this hysteresis occurs annually (Figure ). A weaker, yet still clear, clockwise hysteresis occurs for the sand fraction of the load in 2010 (Figure b). Clockwise hysteresis can be caused by a seasonal exhaustion effect in the delivery of wash material. It may also occur in sand-bedded rivers when bedforms are adjusted to long recessional hydrograph flows but are deformed by rapidly rising flow and are out of phase with the flow, resulting in increased suspension (e.g. Iseya Citation1982). However, this would not affect the washload-dominated hysteresis observed. Rather, the hysteresis pattern in the total load is consistent with an exhaustion effect, which occurs even for the fine sand (McLean and Church Citation1986). The overall hysteresis of the sand load is more muted, suggesting changes in the local sand supply, which may be related to deformation of the bedform field upstream of the study site or seasonal removal of sand from the gravel-bedded reach (e.g. McLean et al. Citation1999a).
Figure 12. Historic (1984, 1985, 1986) and 2010 seasonal hysteresis in daily average total suspended sediment concentration (SSC) with discharge (data source: Environment Canada Citation2011, accessed March, 2011).
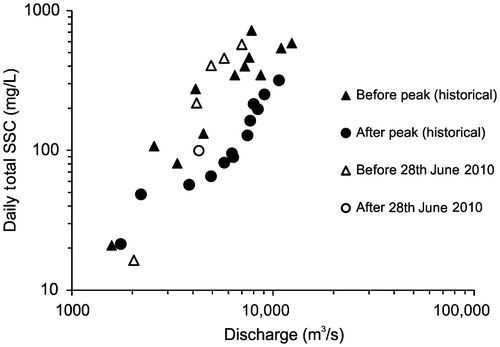
Because the seasonal pattern of sediment flux is relatively smooth, and tracks discharge in approximately linear fashion, the sedigraph in Figure a – even though based on only six measurements – may be integrated to obtain an estimate of the annual sediment flux in the river. In order to do so, it is assumed that the measured sediment flux on 15 April 2010 is representative of the low-flow condition between 15 November and 15 April, roughly corresponding to the period when the river discharge was just below 1000 m3/s (Figure ). The estimated annual load for the 2010 hydrologic year was, accordingly, 6.92 Mt. The annual sediment load was also calculated by developing a correlation between discharge and the daily sediment load, as described in the Methods section. The estimated annual load using this method was 7.21 Mt/a ± 0.59 Mt (p = 0.05). A more refined result could be obtained by developing separate correlations for the rising and falling limbs of the hysteresis loop and calculating daily sediment loads, but the shape of the loop is not sufficiently precise to warrant that exercise.
Discussion
Comparison of 2010 observations to historical records
The estimated load for 2010 was lower than any annual load measured during the WSC’s observations between 1966 and 1986. The annual peak flow, with which annual sediment load is highly correlated, was also lower than in all but 2 years of the WSC sampling period when, respectively 14.5 Mt (1977) and 11 Mt (1980) were estimated. Comparison of suspended sediment concentrations during the 2010 peak flow (Q = 7002 m3/s) and measurements taken at the closest discharge in the historical record (Q = 7150 m3/s) show that concentrations in 2010 were ~30% of those observed in 1986 at the same flow (Environment Canada Citation2011). However, it is difficult to make this direct comparison in light of the hysteresis and the effect that the sequence of previous peak flows may have on sediment storage in the drainage basin.
The year 1977 marked the start of a series of low-flood years extending through 1980 after relatively high freshets through the earlier 1970s and late 1960s. The 2010 season was the second low-flood year following 2 years (2007–2008) with notably high floods. It is known that fine sediment, in particular sand, accumulates in the upstream gravel-bed reach during years of moderate to low freshet, and is evacuated during a subsequent high freshet (McLean et al. Citation1999a). Following two high freshets, it is not surprising that the 2010 load was small; it is somewhat surprising that the 1977 load (Qmax = 7110 m3/s) was so large.
These comparisons aside, the low annual load for 2010 does not appear to be highly anomalous in comparison with the scatter of annual loads (Figure a), nor does the sand load (Figure b). The ratios of silt-clay and sand to the total flux were similar to those earlier observed. Silt-clay made up ~64% of the total load between 1966–1986, while it made up ~70% of the load in the low freshet year 2010. In 2010, ~94% of the total suspended sediment flux and 97% of the suspended sand flux occurred during the freshet hydrograph (15 April to 15 November). During the 3 months of highest flow (May, June, July), 84% of the annual sand load was moved. Despite the small total annual load, it is not inconsistent with the historical measurements. Its magnitude does, however, raise the question whether a secular change in sediment delivery from the drainage basin may have occurred.
Figure 13. (a) Correlation between maximum annual daily flow and annual suspended sediment load at Mission. (b) Correlation between maximum annual daily flow and annual suspended sand load at Mission. Data for 2010 are shown as a square symbol and are not included in the calculation of the displayed mean relation.
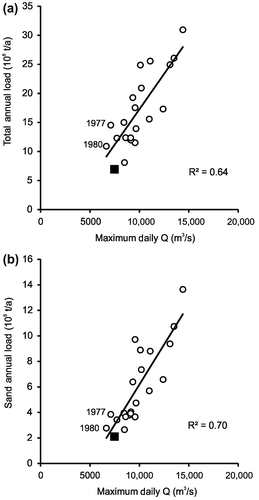
Bed material and wash load
There is no unambiguous way to divide washload from bed material load, although a rule of thumb is that the size division is the D10 of the bed material, which is the grain size corresponding to the 10th percentile (e.g. McLean and Church Citation1986). This means that washload-sized material makes up < 10% of the bed material. Figure compares bed and suspended material over the freshet at 0.1h (the deepest sampling point) at the centre Profile 3. The D10 of the bed material in the channel centre ranges from 184–264 μm. The grain-size class that is less than this is 177 μm, which is the nominal washload-bed material load division in the reach established by McLean and Church (Citation1986). The overlap in the grain-size distributions in Figure suggests that the coarser sand in suspension in the reach is sourced from the bed.
Figure 14. Bed material and laser in-situ scattering transmissiometry (LISST)-Portable suspended sediment grain-size distributions at 0.1h in the water column for Profile 3. The bed material distribution (short-dashed lines) is the fraction retained on a sieve. The suspended material fraction (solid lines) is the fraction in a particular grain-size bin. The horizontal long-dashed line marks the conventional definition of washload as being less than 10% of the bed material grain size. The vertical dotted line marks where the bed material grain-size distributions intersect at 190 μm with the 10% criterion. The nearest sieve size is 177 μm.
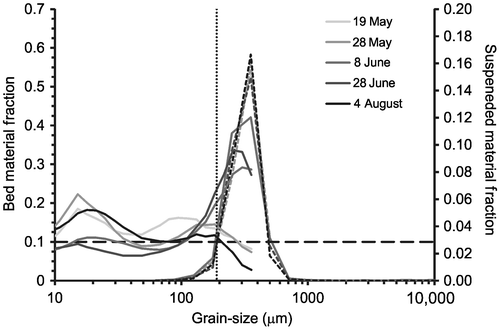
During low-moderate flows on 18–19 May, 27–28 May and 3–4 August, sand greater than 177 μm represented 15, 16 and 10%, respectively, of suspended material. During the high and peak flows of the 7–8 June and 27–28 June campaigns, 36 and 41%, respectively, of the sediment load was composed of sand > 177 μm at the lowest sampling point in Profile 3. This implies that there is significant bed material suspension at high flows in the reach. The threshold for significant bed material suspension lies between the discharges during the 27–28 May and 7–8 June campaigns (5000–5700 m3/s at Mission).
The K-factor
The variability in flow across the measurement section affects sediment flux. In its 1966–1986 measurement program, the WSC typically obtained a single depth-integrated profile of suspended sediment near channel centre each day throughout the spring, summer and autumn seasons. WSC obtained an empirical relation between depth-integrated sediment concentration, cK, at the centreline profile and the cross-sectional average concentration, cR, to define a “K-factor”, based on a few complete measurements in each year: (10)
(as reported by McLean and Church Citation1986) in order to estimate total sediment flux from the data of the single profile. The K-factor for the recent data was calculated using cR equal to the weighted mean of the depth-integrated samples taken at each of the five profile locations and cK equal to the second depth-integrated sample obtained at the centre of the channel at Profile 3.
Historic and 2010 K-factors as a function of discharge are shown in Figure . There were sufficient historical data available for only 1984, 1985 and 1986. McLean and Church (Citation1986) found that the K-factor at Mission varied systematically: higher at low flows, becoming noticeably lower at higher discharges, implying that a disproportionate share of the sediment load moves near the margins of the channel at low flows, but becomes more evenly distributed across the section, with slight central channel dominance, at high flows. The 2010 K-factor data change in a similar manner (Figure ). However, the average K-factors appear to be variable over time. K-factors have ranged from approximately 1.0 to almost 1.6 during low flows and from 0.65 to 0.85 for high-flow flows. The average K-factor varied between 1984 and 1986 from approximately 0.8 to 0.9. In 2010 the average K-factor was 0.93 because the low freshet effectively increased the weight of low-flow conditions. The consistent pattern between the historical record and 2010 suggests that a generalized K-factor remains an appropriate approach for estimating sediment flux in the channel.
Figure 15. Comparison of 2010 variation in K-factor with discharge to observations in 1984, 1985 and 1986. The dashed lines are the year’s average K-factor. The arrows highlight a hysteresis in the K-factor that occurs in all years. (Data source: Environment Canada Citation2011, accessed November, 2011).
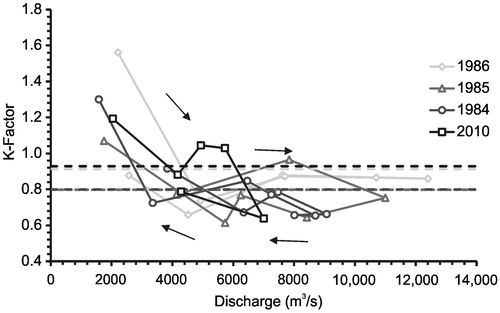
Comparison of current sediment yield to other Canadian rivers
It is difficult to determine if the remarkably low mean annual sediment yield – only 40% of the historical mean annual yield – during 2010 in the Fraser River occurs elsewhere in the region or across Canada. The sediment monitoring program undertaken by the WSC has been discontinued across the country. However, generally, Fraser River sediment yields are near average for Canadian rivers. Sediment yield scales with area, so comparisons must be restricted to equivalent areas (Church et al. Citation1999). The only similarly-sized river in the region for which there are comparable sediment yield data is the Peace River, the major basin to the north of Fraser Basin. The Peace Basin yields more than twice as much sediment at Peace Point (area 293,000 km2), on average, as Fraser Basin (area 228,000 km2). The principal reason for the difference is the relative erodibility of rocks in the two drainages.
Sediment yield from the Fraser can be compared to smaller drainages on an area-constrained basis. At 104 km2, the sediment yield of Fraser Basin is 0.5 Mt/km2/a, compared with 0.75 Mt/km2/a in the Peace-Mackenzie system. The relatively arid South Saskatchewan Basin yields 0.20 Mt/km2/a at 104 km2 while southern Ontario basins yield 0.15 Mt/km2/a. For smaller areas, the sediment yields are different, but the ratio of sediment yield from the Fraser to other drainages remains approximately the same at 102 km2 except for southern Ontario, where yields remain at 0.15 Mt/km2/a – that is, sediment yield in this heavily settled region is essentially scale-free (all data from Church et al. Citation1999). Nevertheless, this comparison is based on the historical records of sediment yield. Whether the remarkably low mean annual yield observed in the Fraser in 2010 is a more widespread phenomenon cannot be assessed.
Conclusions
Suspended sediment concentration, bed material, velocity and discharge were measured on the Fraser River at the site of WSC gauge 08MH024 at Mission, BC, over the 2010 freshet. Total suspended sediment concentrations were obtained, and flux was calculated from isokinetic, filtered, bottle samples. Flux was broken into sand and silt-clay fractions using grain-size distributions obtained from a LISST-Portable instrument. The estimates of concentration and flux were compared with historical records based on sediment rating curves from the Water Survey of Canada’s 1965–1986 sediment monitoring program. The results demonstrate that:
The annual sediment flux at Mission was the lowest on record, but falls within the envelope of data obtained by WSC during 1966–1986. The ratios of sand and silt-clay to the total annual sediment load remain relatively stable. Most of the transport is silt and clay, which are supply-limited.
There is seasonal clockwise hysteresis in the sediment flux. Grain-size discrimination reveals a strong hysteresis for silt-clay, but a weaker effect for sand.
Significant bed material suspension occurs at flows exceeding 5000 m3/s. Approximately 94% of the annual sediment flux and 97% of the sand flux occurs when the flow > 1000 m3/s. During the three highest flow months, 84% of the annual sand load is moved.
A discrepancy exists between sediment concentrations reported by the LISST-Portable and those found via traditional filtering methods. The manufacturer suggests that measured volumetric sediment concentrations can be converted to mass per volume concentrations using a conversion factor of 2.65. This work shows a conversion factor of 1.67 is more appropriate for the Fraser River at Mission, but is not unbiased.
Model calculations of sediment flux based on the Rouse profile exhibit significant variation in absolute concentration from observed results, although vertical gradients of suspended sediment concentration are reasonably approximated. Further work is required to thoroughly analyze model estimates which, in any case, still depend on an observed reference sediment concentration.
Acknowledgements
We thank Russ White and Bruno Tassone from the Water Survey of Canada, Environment Canada, for providing funding for the project. Emily Huxter and Curt Naumann, Water Survey of Canada staff, provided technical support, including the water discharge measurements. Megan Hendershot and Sally Haggerstone provided technical support for the sediment sampling operation. Maureen Attard was supported by a Simon Fraser University Graduate Fellowship, the Geography Department, and Natural Sciences and Engineering Research Council (NSERC) Discovery grants to Jeremy Venditti and Michael Church. Participation of Jeremy Venditti and Michael Church was supported by NSERC Discovery grants.
References
- Agrawal, Y. C., A. Whitmire, O. A. Mikkelsen, and H. C. Pottsmith. 2008. Light scattering by random shaped particles and consequences on measuring suspended sediments by laser diffraction. Journal of Geophysical Research 113: C04023. doi:10.1029/2007JC004403.
- Blott, S. J., and K. Pye. 2001a. GRADISTAT, Version 4.0. http://www.kpal.co.uk/gradistat.html (accessed September, 2011).
- Blott, S. J., and K. Pye. 2001b. GRADISTAT: A grain-size distribution and statistics package for the analysis of unconsolidated sediments. Earth Surface Processes and Landforms 26: 1237–1248.
- Church, M. 2010. Sustaining Fraser Estuary: Bases for a sediment management plan. Report prepared for Fraser Estuary Management Program (now defunct), Burnaby, British Columbia, Canada, August 26, 2010, 39 p. (available from author).
- Church, M., D. Ham, M. A. Hassan, and O. Slaymaker. 1999. Fluvial sediment yield in Canada: Scaled analysis. Canadian Journal of Earth Sciences 36: 1267–1280.
- Dietrich, W. E. 1982. Settling velocity of natural particles. Water Resources Research 18(6): 1615–1626.
- Environment Canada. 2011. Real-time hydrometric data. http://www.wateroffice.ec.gc.ca (accessed March, 2011).
- Iseya, F. 1982. A depositional process of reverse graded bedding in flood deposits of the Sakura River, Ibaraki Prefecture, Japan. Geographical Review of Japan 55: 597–613.
- McLean, D. G., and M. Church. 1986. A re-examination of sediment transport observations in the lower Fraser River. Report 1W-WRB-HQSS-86-5, 56 pp., Environment Canada, Water Resources Branch, Sediment Survey, Ottawa.
- McLean, D. G., M. Church, and B. Tassone. 1999a. Sediment transport along lower Fraser River. 1. Measurements and hydraulic computations. Water Resources Research 35: 2533–2548.
- McLean, S. R., S. R. Wolfe, and J. M. Nelson. 1999b. Spatially averaged flow over a wavy boundary revisited. Journal of Geophysical Research 104(C7): 15,743–15,753.
- McLean, D. G., Mannerström, M., and T. Lyle. 2006. Impacts of human interventions on the lower Fraser River. Canadian Water Resources Association, British Columbia Division, Annual Conference, Vancouver, October 24–25, 10 pp.
- Northwest Hydraulic Consultants. 2002. Review of lower Fraser River sediment budget: Final report. Report prepared for the Dredge Management Advisory Committee, Fraser River Estuary Management Program. www.bieapfremp.org/fremp/pdf_files/SedimentBudgetFinalReportFeb03.pdf (accessed May, 2014).
- Northwest Hydraulic Consultants. 2008. Comprehensive review of Fraser River at Hope, flood hydrology and flows – Scoping study. Report prepared for British Columbia Ministry of Environment. www.env.gov.bc.ca/wsd/public_safety/flood/pdfs_word/review_fraser_flood_flows_hope.pdf (accessed May, 2014).
- Sequoia Scientific, Inc. 2010. How do I convert the volume concentrations (VC) from the LISST to mass concentrations? http://sequoiasci.com/Articles/ArticlePage.aspx?pageid=197 (accessed February, 2012).
- Smith, J. D., and S. R. McLean. 1977. Spatially averaged flow over a wavy surface. Journal of Geophysical Research 83(12): 1735–1746.
- van Rijn, L. C. 1984. Sediment transport, part II: Suspended load transport. Journal of Hydraulic Engineering 110: 1613–1641.
- van Rijn, L. C. 1993. Principles of sediment transport in rivers, estuaries and coastal seas. Amsterdam, The Netherlands: Aqua Publications.
- Venditti, J. G., R. P. Humphries, M. A. Allison, J. A. Nittrouer, and M. Church. 2010. Morphology and dynamics of a gravel-sand transition. In Proceedings of the 9th Federal Interagency Sedimentation Conference, 11 p., June 27-July 1, 2010, Las Vegas, NV.