Abstract
In May 1997, the Red River Valley of Manitoba, North Dakota and Minnesota experienced a record flood which came to be known as the “Flood of the Century”. Prospects for only moderate flooding were completely transformed by a major snowstorm in the first week of April. Record snow and rain over the entire basin upstream of Winnipeg produced record discharges all along the river. At Winnipeg, the peak discharge was about 50% greater than the previous highest measured flow and was probably the second largest event in two centuries of documented flood history. At its peak, about 1840 km2 in Manitoba were inundated in a water body 100 km long with a maximum width of about 40 km. In Manitoba, 27,400 people were evacuated and damages and costs exceeded CAD $500 million (and were possibly closer to $1 billion). Nevertheless existing flood control structures, flood proofing and damage reduction programs, and emergency measures prevented losses which would have been truly catastrophic in Winnipeg. Furthermore, the peak stage might have been even greater except for manageable flows on the Assiniboine River and favourable weather during the flood itself. In the United States where flood control/flood proofing measures were less well developed, damages were many times greater and almost three times as many people were evacuated. The legacies of the flood in Manitoba include a CAD $665-million program to increase flood control and flood proofing for Winnipeg to the 700-year flood level. In the Red River valley outside Winnipeg, ring dikes were constructed around eight previously unprotected communities, individual properties were diked or elevated on pads, and flood proofing standards throughout the valley were raised to 0.6 m above the 1997 peak stage.
En mai 1997, la vallée de la rivière Rouge du Manitoba, le Dakota du Nord et le Minnesota ont connu une inondation record qui est passée à l’histoire sous le nom de l’« inondation du siècle ». Ce qui s’annonçait à peine comme une inondation modérée s’est en fait transformé en un événement beaucoup plus grand en raison d’une tempête de neige majeure au cours de la première semaine d’avril. Des chutes de neige et des précipitations records au-dessus du bassin tout entier en amont de Winnipeg ont produit des débits records pour l’ensemble de la rivière. À Winnipeg, le débit de pointe était d’environ 50 % plus élevé que le débit mesuré précédent le plus élevé et a probablement constitué le deuxième événement le plus important en deux siècles d’histoire d’inondations documentées. À la crête de l’inondation, une superficie d’environ 1 840 km2 au Manitoba avait été inondée dans une étendue d’eau d’une longueur de 100 km avec une largeur maximale d’environ 40 km. Au Manitoba, 27 400 personnes ont été évacuées. Les dommages et les coûts ont dépassé les 500 millions de dollars CAD (et ont probablement avoisiné un milliard de dollars). Néanmoins, les ouvrages de défense contre les inondations existants, les programmes de protection contre les inondations et de réduction des dommages, ainsi que les mesures d’urgence ont permis de prévenir des pertes qui se seraient avérées vraiment catastrophiques à Winnipeg. De plus, l’état de crête aurait pu être plus grand n’eut été des débits gérables de la rivière Assiniboine et du temps favorable au cours de l’inondation elle-même. Aux États-Unis, où les mesures de protection contre les inondations étaient moins bien développées, les dommages ont été plusieurs fois plus élevés et presque trois fois plus de gens ont dû être évacués. L’héritage légué par l’inondation au Manitoba englobe un programme de 665 millions de dollars CAD en vue d’accroître les mécanismes de protection contre les inondations pour Winnipeg à un niveau des crues à récurrence de 700 ans. Dans la vallée de la rivière Rouge à l’extérieur de Winnipeg, des cercles de digues ont été construits autour de huit collectivités non protégées auparavant, des propriétés individuelles ont été entourées de digues ou surélevées grâce à des pierres d’appui ou à des remblais. Par ailleurs, les normes de protection contre les crues pour toute la vallée ont été haussées jusqu’à 0,6 m au-dessus de l’état de crête de 1997.
Geographical and hydrological setting
The Red River has it headwaters in southern North Dakota (ND) and Minnesota (MN) and flows northward, forming the boundary between these states (Figure ). It crosses into Canada at Emerson, Manitoba, where its name changes from “Red River of the North” (as it is officially known in the United States) to simply “Red River” in Manitoba. Along its northward course, it receives tributaries from the east and west in a trellis pattern until, at the southern boundary of Winnipeg, it has a drainage area of 119,000 km2. At the “Forks” in downtown Winnipeg, the Red is joined from the west by its largest tributary, the Assiniboine River, which more than doubles the total drainage area to 285,000 km2. Although the Assiniboine has a drainage area which is 30% larger than the Red above the confluence, the drier climate of its basin produces a mean annual flow only about a third as large. From the Forks, the Red River continues to Lake Winnipeg 60 km to the north, with little additional inflow along this reach.
The landscape of the Red River valley is a crucial element to the nature of Red River floods. From its southernmost region, the river flows northward to Lake Manitoba in a strongly meandering course across the sensibly flat former floor of Glacial Lake Agassiz. Gradients are exceptionally small, both along the river’s entire downstream course, and to the east and west of the river for many tens of kilometres; in the Manitoba section of the river, the downvalley gradient averages only 0.00007 m/m (Brooks et al. Citation2005). This landscape has two consequences for Red River floods. First, the very gentle lateral gradients permit overbank flow to spread over a much broader area than is typical for most floodplains. Second, the extremely small downvalley gradient of the main channel combined with the capacity of the flooded area to store water produce very slow travel times for floodwaters. Floods build and dissipate over time periods measured in several weeks; a flood peak in Winnipeg, for example, may be two to three weeks later than in Grand Forks, ND, 220 km to the south. Finally, although the gradients of major tributaries are also relatively gentle, they are nevertheless greater than the downvalley gradient of the main channel by a factor of 10 or more, which means that water can be delivered to the main channel faster than it can be removed downstream.
All major floods on the Red River are spring snowmelt (freshet) events in late April and May. Typical antecedent conditions which prime the watershed for a spring flood begin with above-average precipitation in the previous fall which fills available surface and subsurface storage. Cold weather prior to the first major snowfall permits deep freezing of the saturated, clay-rich ground, creating an impermeable surface the following spring. Abundant snowfall during the winter may provide a large standing water content as the flood season approaches; late-winter precipitation is more important than the total for the whole winter season. As the flood season approaches, the importance of flood-forming factors increases. A late gradual spring warming may release the water from the snowpack slowly, producing a smaller peak, whereas a rapid transition may lead to a more rapid melt when warm weather finally arrives. Perhaps the most crucial factor in virtually all large floods is the occurrence of significant precipitation (either rain or snow) during the period from the beginning of flood formation up to the peak. Finally, once a flood is underway and a large expanse of water has formed in the valley, downstream areas such as Winnipeg are susceptible to setup from strong south winds which elevate the water surface beyond what would be produced by the discharge alone.
Most of the water which causes major Red River floods originates in the US and travels northward, crossing the border at Emerson. In some years, the Assiniboine River may make a major contribution, but the peaks on the Red and Assiniboine do not normally coincide. Also in some years, as in 1997 and 2009, the south-to-north orientation of the river’s course may cause floods to build as melt progresses in a northward direction, but, in many years, the melt occurs more or less simultaneously along the river such that the Manitoba tributaries are well past their peaks before the main floodwaters arrive.
As a result of the disastrous 1950 flood, Manitoba constructed a system of structural flood control and flood proofing measures to reduce flood damages (Figure ). This system is described in detail in Blais et al. (this issue). These measures proved extraordinarily successful in minimizing damages during floods in 1974, 1979 and 1996, all of which were large 1950-level events but were well within the design capacity of the protection system. In 1997, however, the system was tested to its limit.
Causes of the flood
The months preceding the 1997 flood exhibited all of the classic preconditions for large Red River floods. A wet fall and early onset to winter reduced available storage and potential infiltration for snowmelt in the spring. Winter was severe with virtually no breaks. Winter precipitation (mostly as snow) was much above average throughout the basin, particularly in the US portion above Emerson where record total snowfalls were measured at most stations (Todhunter Citation2001). At Fargo, ND, for example, average winter snowfall was 2867 mm, three times the average of 953 mm (Anderson and Braatz Citation1997) and locations with 200% or more of normal were common (Todhunter Citation1998). Below-average temperatures produced few thaws, ensuring that the water content of the snowpack as spring approached was very much above average throughout the watershed. In early February, an airborne gamma snow survey showed snow water contents which were the highest on record in the US basin, and among the highest in Manitoba (Warkentin Citation1997).
Flood forecasts beginning in February indicated the possibility of moderate flood conditions, about equivalent to the floods of 1950, 1979 or 1996 (approximately 3000 m3/sec). In Manitoba, this raised few major alarms since such a flow could be easily managed by the flood control system, passing through the flood-proofed valley with only minimal, routine measures such as closing the gaps in the community or individual ring dikes and localized diking in some unprotected areas within and outside Winnipeg, etc. Only a small number of evacuations would be necessary, and damages would be small; probably the greatest consequence would be the inconvenience and economic loss from the closure of Highway 75 south of Winnipeg, the main artery for car and truck traffic to and from the US. Even these anticipated preparations were being relaxed somewhat and forecast peaks revised downward in March as a gradual melt began to release the accumulated snowpack in an orderly fashion. Although some flooding began in late March in the US portion of the watershed, no extraordinary measures were contemplated in Manitoba.
All of this changed dramatically on 5–7 April, when a severe blizzard from a deep Colorado Low with hurricane force winds (nicknamed “Blizzard Hannah” in North Dakota) transformed the anticipated easily managed, modest flood into one of the largest Canadian natural disasters to that time. Hannah was the eighth such storm of the winter, and the worst. Described by Todhunter (Citation2001) as “the most intense blizzard over the past 50 years in the region,” the storm brought rain and/or snow to most of the watershed south of (and including) Winnipeg. Drifting under the extreme winds and the rain/snow mixture in the southern basin complicated the assessment of total water added by the blizzard but records were broken at many places, such as at Crookston, MN, where the 92 mm of water equivalent was 50% greater than the previous record 2-day April total (International Red River Basin Task Force Citation1997). Todhunter (Citation2001) reported total water equivalents of 35–70 mm across the US basin, and as much as 90 cm of snow may have fallen in some regions (Warkentin Citation1997); at Winnipeg, the storm total was 58 cm of snow. A crucial point which will be returned to below was that the storm missed most of the Assiniboine watershed.
The spatial pattern of the storm’s rain/snow mix was as important as the total amount to the way the flood evolved. Runoff and the beginning of flood formation was occurring prior to Hannah in late March and early April in the southern part of the basin upstream of Fargo, but had not begun north of Grand Forks, ND. Much of the storm’s precipitation in the southern regions was rain, which allowed runoff to continue to build despite a reduction in snowmelt caused by colder temperatures for several days after the storm. When strong thawing conditions returned to the southern basin, areas further north remained cold. In Manitoba, temperatures did not begin to rise into strongly positive values for almost 2 weeks after the storm (Figure ); Winnipeg, for example, accumulated only 10 total maximum degree-days above 0°C between 7 and 17 April. As a result, while the flood was building to the south, little melt was occurring on the Manitoba side of the border and, at the time the river was approaching its peak in Grand Forks, ND, it had scarcely begun to rise in Winnipeg.
Figure 3. Daily maximum temperatures, Winnipeg, April 1997 (Rannie Citation1998b).
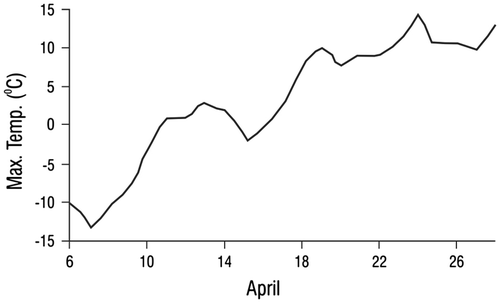
Such a strong south-to-north gradient in thawing conditions is not typical, despite the alignment of the river’s course (although it did occur again in the 2009 flood (Government of Manitoba Citation2009; Wazney et al. Citation2015). More commonly, melt and runoff occur at about the same time throughout the basin, such that by the time floodwaters arrive from the US, Manitoba streams are well below their peaks. In 1997, the differences in rain/snow mix and the south–north temperature gradient allowed the flood crest to build downstream in abnormal fashion. The main flood crest in Fargo occurred on 18 April. At Grand Forks, peak discharge also occurred on 18 April (when the city’s dikes failed) but because of local peculiarities in channel gradients and strong hysteresis in the rating curve, peak stage actually occurred 4 days later (Todhunter Citation2001). The downstream progression of peak discharges is given in Table .
Table 1. Peak discharges and dates, Fargo, North Dakota, to Winnipeg.
Flood progress and emergency measures
In anticipation of 1950/1979-level flooding, preparations had begun in March to protect the most vulnerable areas. These involved some diking of the lowest properties not protected by the Floodway and other structures, plans for the closure of the highway gaps through the community ring dikes, etc. These were routine non-emergency measures since the expected discharges were well within the ability of the system to pass the water through the valley with minimal disruption. After the April storm, however, forecast peak stages were quickly revised upward and subsequent revisions took them to unprecedented levels. The Province formally requested the assistance of the Canadian Armed Forces on 10 April, and full-scale emergency measures began in earnest. These measures involved closure of the gaps in the ring dikes, raising the community ring dikes and Winnipeg’s secondary dikes to provide 0.6 m of freeboard, construction of dikes around several thousand unprotected properties, evacuation of most farm animals and poultry, movement of equipment to higher ground, inspection of storm sewer pumps and outlets in Winnipeg, etc.
Perhaps the most dramatic emergency measure was the construction of the “Brunkild” “Z” Dike. As the crest approached Winnipeg, concern was expressed that floodwater might bypass the western end of the Floodway’s West Dike and enter the city via the La Salle River. To prevent this, a remarkable engineering operation extended the dike westward by 26 km and augmented 15 km of the existing west dike (Manitoba Natural Resources Citation1997d). The main work was completed within 5 days (and nights under flares dropped from aircraft), involved up to 400 pieces of earth-moving equipment which installed 750,000 m3 of earth, 142,000 tonnes of limestone, 2000 1.5-tonne “super sandbags” and 4000 large round bales of straw. Forty kilometres of snow fence, 8 km of oil boom and 2.4 km of derelict vehicles were placed along the dike to absorb wave energy (Manitoba Natural Resources Citation1997e).
Throughout this period, the flood was building in the US portion of the basin and a greater and greater area along the river was being inundated. At Fargo, ND, the river reached its peak on 18 April, and in Grand Forks, ND, on 21–22 April. The sense of urgency was forcefully impressed on the general public in Manitoba when the dikes at Grand Forks failed on 18 April and a major fire broke out in the city’s downtown the next day, providing compelling images of simultaneous flooding and fire broadcast across the US. In Manitoba, a State of Emergency was declared on 20–21 April for immediately threatened areas, and extended to the entire Red River valley on 22 April. As the scale of the emergency became apparent, additional military assistance was requested, eventually reaching a total of 8500 army, navy, air force and coast guard personnel, the largest deployment of Canadian military personnel since the Korean War. These augmented a civilian “army” of several thousand engineers, surveyors, equipment operators and other technical personnel from the private sector, all available provincial employees and tens of thousands of volunteers who built and patrolled dikes, managed evacuation centres, maintained pumps and provided general support with cooking, transportation, communications, etc. In all its aspects, the emergency management operation stretched over a month, making it the longest and most costly such operation in Canadian peacetime history to that date. In the United States, 5300 troops from the Minnesota and North Dakota National Guards were deployed.
Strong melting began occurring in Manitoba about 16 April under maximum temperatures of 10–15°C, causing local flooding along much of the Manitoba reach of the river. Minimum flood stage was reached on 19 April at Emerson and Morris, and on 22 April in Winnipeg. The flood crest continued its inexorable path northward from Grand Forks, reaching Emerson on 27 April, Morris on 30 April, Ste. Agathe on 2 May, St. Adolphe on 3 May and Winnipeg on 4 May (Table ). The delay in melting in Manitoba meant that many of the important tributaries were just reaching their peaks as the main body of floodwater arrived. This, and the fact that much of the potential storage on the floodplain was already filled by local melting, caused peaks to increase abnormally as the crest moved northward. When peak stages in 1997 are compared with those during the 1979 and 1996 floods, the differential increased downstream relative to both floods. At Emerson, for example, the peak stage in 1997 was 0.35 m higher than in 1979, but at Morris, St. Adolphe and the Floodway entrance, the differences increased to +0.63 m, +1.28 m and +1.93, respectively (City of Winnipeg Citation1997). In comparison with 1996, the 1997 peak stages were +0.86 m higher at Emerson but +2.05 m higher at the Floodway entrance (City of Winnipeg Citation1997). Thus, the atypical way the flood built downstream presented as great a problem as the management of the volume of water itself.
Overland flooding on an unprecedented scale presented another extraordinary problem. The breadth of the inundated area, the almost-flat landscape and the subtle human modifications to the topography produced by road and rail embankments, buildings, artificial drainage lines, etc. combined to create a lattice of barriers and basins which distorted flow patterns and complicated prediction of peak stages. Todhunter’s description of the progress of the flood northward in the United States applied equally to the Manitoba portion of the valley:
The staggering quantity of meltwater produced by the late thaw is difficult to describe. As snowmelt proceeded on the flat wetland terrain and former glacial lake-bed the distinction between channeled flow, surface storage, and overland flow became meaningless. Patterns of surface runoff changed with time as the late spring snow and ice melt progressed. Sections of farmland filled with water as rural roads acted like dams. As the sections filled, the water would spill over the roads onto adjoining farm sections. This domino-like pattern continued, producing a broad and flat surge of water that slowly moved northward, overwhelming everything in its path… As meltwater crossed indistinct drainage divides the entire glacial lake-bed became a shallow north-flowing lake. (Todhunter Citation2001, 1275)
This “shallow north-flowing lake” in Manitoba quickly became known in the media as the “Red Sea” (Figure ). At its maximum, the “Red Sea” covered an area of 1836 km2 (Warkentin Citation1997), with a maximum width of about 40 km and a length of 100 km in Manitoba from Winnipeg to Emerson; in the United States, a similar but longer, somewhat narrower, lake was created.
At Winnipeg, the Floodway began diverting water around the city on 21 April. This diversion was necessary to manage the increasing volumes of melt being generated just in the Manitoba section of the valley, not the main flood, the crest of which didn’t arrive until almost 2 weeks later. When the crest did arrive on 4 May, a peak discharge of 3908 m3/sec was recorded at the entrance to the Floodway (Manitoba Natural Resources Citation1997e). The routing of Red and Assiniboine River waters on that day is given in Figure .
Figure 5. (a) Routing of water during a design flood (after Mudry et al. Citation1981). (b) Routing of water on 4 May 1997.
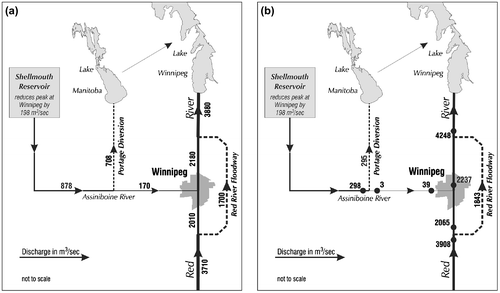
Comparison of the actual routing of water at the flood peak with the design routing reveals how critical the situation was for Winnipeg (Figure ). With 3900 m3/sec arriving from the south, the Floodway design discharge had to be exceeded to keep flows in the channel through Winnipeg below a stage of 7.5 m, to provide a necessary 0.6 m of freeboard below the levels of the primary and secondary dikes through the city (all stages are with reference to the James Avenue pumping station in downtown Winnipeg). In the absence of the overall control system (i.e. under “natural” conditions), it is estimated that peak stage would have been 10.5 m (A. Warkentin, personal communication), or 1.2 m above the disastrous 1950 flood level and 2.4 m above the level of the diking system within the city. Such a flow would have flooded a large area of the city, required evacuation of most or all of the population of 630,000, and produced the greatest natural disaster in Canadian history. To prevent this, however, the Floodway was required to carry almost 10% more than its design capacity. After the crest had passed, the Floodway continued to operate at a high level to permit stages within Winnipeg to be lowered as rapidly as possible to alleviate stress on the dikes and reduce the threat of their failure, or failure of the riverbanks.
Within Winnipeg, the Primary Line of Defence was the elevation of the permanent Primary Dike System which had been constructed after the 1950 flood, supplemented by permanent secondary dikes mostly on private property where space was restricted. In 1997, the Floodway was being operated to control peak stages at 0.6 m below the Primary Dike elevation, to provide a freeboard margin of safety on the dikes and enable the stormwater sewers to continue to operate. Secondary dikes were elevated and about 800 individual properties below the Primary Line of Defence were protected by sandbag or earthen dikes; almost 3.9 million sandbags were used in the sandbag dikes and 600,000 m3 of earth was excavated for earth dike construction. Beyond the protection of properties, much effort was required to inspect the pumping stations and gates of the waste and stormwater collection systems to ensure that they would operate at such high stages (McNeil and MacBride Citation1997).
Most of the towns and rural municipalities in the valley south of Winnipeg declared local states of emergency between 20 and 23 April. Evacuations which had begun on a voluntary basis a few days earlier became mandatory, beginning on 23 April and continuing through 27 April as the flood progressed northward. By 30 April, 17,000 people had been evacuated from the valley and 7000 more in Winnipeg (Manitoba Natural Resources Citation1997a). The communities with ring dikes, which had been raised on an emergency basis to 0.9–1.4 m above peak stages, sustained little damage, but other areas were less fortunate. Most affected were the communities of Ste. Agathe and Grand Pointe, which were protected only by emergency dikes (combined with elevated road and rail embankments in the case of Ste. Agathe). These defences failed on 29 April at Ste. Agathe and on 1–2 May at Grande Point. The fate of individual properties in the valley varied widely. A majority of those which had been raised on pads or were diked survived with little damage, but many did not.
A significant feature of all Red River floods is their extremely long duration. The same factors that cause the very slow buildup to the crest – the large volume of water stored on the “floodplain” and the exceptionally small gradients – also produce a recession limb which extends over several weeks. At Grand Forks, ND, the river was at flood stage from 4 April to 19 May (Todhunter Citation2001). Flows at Emerson and Morris did not fall below minimum flood stage until 25–29 May, 4 weeks after the crests; at most places throughout the valley, minimum flood stage along the river was exceeded for 36–40 days. Flow through the Floodway continued until 3 June. Along the margins of the flooded area, evacuees began to return within a few days of the crest’s passage, but closer to the river their return took much longer. Emerson was not re-occupied until 12 May and as late as 15 May, 12,000 people remained evacuated (Manitoba Natural Resources Citation1997c). Residents of other communities between Emerson and Winnipeg were allowed to return on 16–17 May (Manitoba Natural Resources Citation1997b).
Flood magnitude and statistical assessment
Prior to 1997, the three largest floods in the Red River valley were historic events in 1826, 1852 and 1861, with estimated maximum discharges of 6370, 4670 and 4620 m3/sec, respectively, calculated by the Red River Basin Investigation from peak stages and water slopes compiled and surveyed by Sandford Fleming in the 1870s during his surveys for the routing of the Canadian Pacific Railway (CPR) (Red River Basin Investigation Citation1953). Given the margin of error for these estimates, the 1997 flood can be considered to have at least tied 1852 as the second largest in 200 years of documented flood history (Rannie Citation1998a), and evidence is presented below to suggest that the flow in the Red upstream of the Assiniboine was greater than in 1852.
Contemporary flood frequency studies of the Red River indicate return periods of about 100–140 years for the 1997 flood at Canadian stations between Emerson and Winnipeg (International Red River Basin Task Force Citation1997; Hardin Citation1999; International Joint Commission [IJC] Citation2000; Burn and Goel Citation2001). In the United States, return periods were about 140 years (IJC Citation2000) except at Grand Forks, ND, where atypical circumstances produced a peak discharge 62% greater than the previous record flow and a stage 1.67 m higher; there, the return period has been estimated at > 200 years (Todhunter Citation1998, Citation2001).
As large as this flood was, it could have been even worse except for two mitigating factors. The first was the favourable state of the Assiniboine River. As was noted above, the early April storm missed most of the Assiniboine basin. Although the freshet discharge on the Assiniboine was still relatively high, peak discharge of 835 m3/sec occurred on 20 April, 2 weeks before the arrival of the Red River crest in Winnipeg. When the Red peaked on 4 May, the natural Assiniboine flow had fallen to about 300 m3/sec, all of which could be easily diverted to Lake Manitoba via the Assiniboine Diversion (Figure ). Thus, the Assiniboine made no meaningful contribution to the peak flows on the Red, reducing the burden on the Red River Floodway to keep channel flows through the city within (barely) manageable limits. In fact, Assiniboine flow downstream of the Portage Diversion was reduced to virtually zero as the crest on the Red mainstem arrived at Winnipeg from the south. The Assiniboine, however, is capable of generating much larger spring flows which far exceed the capacity of the Diversion. In 1976, a discharge of 1460 m3/sec was recorded upstream of Portage la Prairie, and even this improbably large flood was exceeded in 2011 (Government of Manitoba Citation2013; Blais et al. this issue). The Assiniboine Diversion is designed to reroute 708 m3/sec to Lake Manitoba; under emergency conditions and with very serious consequences for the level of Lake Manitoba, this was stretched to 963 m3/sec in 2011. At the 1997 flood peak, flow in the Red River channel through Winnipeg was dangerously high even with the Floodway operating above capacity. Any additional Assiniboine water that could not be diverted (i.e. in excess of 700 or perhaps 950 m3/sec) would have placed a critical burden on the ability to manage flows in Winnipeg, threatening the integrity of the city’s primary dike system. Under those conditions, the flow in the Floodway would have had to be increased even further by raising water levels upstream of Winnipeg (thereby imperiling more properties upstream of the Floodway). Any of these options would have greatly increased the magnitude of the disaster. If Assiniboine flows had equaled those of 1976 or 2011, even these desperate measures might not have been sufficient to prevent widespread flooding in Winnipeg. Interestingly, there is convincing evidence that the Assiniboine made such a major contribution to the extreme 1852 flood (Rannie Citation2002). If this was so, it would support the contention that the northward flow of floodwater arriving at Winnipeg was greater than in 1852, making the 1997 flood along the Red River proper (i.e. above the confluence with the Assiniboine) the second largest event in 200 years.
A second mitigating factor was the generally favourable weather during the rising phase and near the crest. Precipitation during the rising phase is a critical factor in many Red River floods. In 1997, precipitation was much below normal. No major rainstorm occurred until 3 days after the crest had passed, too late to seriously affect the peak. More serious were south winds on several days which raised the water level on the downstream (Winnipeg) end of the 1840-km2 “Red Sea,” but even these winds might easily have been stronger and/or more prolonged, presenting a greater threat to the Z dike to the west of the Floodway, and to the dikes around communities or individual properties.
Flood damages
A year after the flood, the Manitoba Water Commission estimated the “costs of flood preparation, flood fighting, damages and associated expenditures” in the Manitoba portion of the Red River Valley to have been about CAD $500 million (Manitoba Water Commission Citation1998). Much later, Etkin (Citation2010) cited a 2007 estimate by Public Safety Canada which placed the figure at CAD $815 million in year 2000 dollars, or approximately $750 million in 1997 dollars. If indirect costs such as lost business opportunities are included, the total might even have approached CAD $1 billion. These costs were incurred even with the flood control system and other protection measures working as intended. In the valley, for example, none of the ring-diked communities were flooded, although Ste. Agathe, which relied on a combination of dikes, highway and railway elevations, was overwhelmed. In Winnipeg, about 800 low-lying riverbank properties required protection but only about 60 sustained damage (City of Winnipeg Citation1997). Total costs for flood-fighting and cleanup, evacuations and infrastructure repair incurred by the city were about CAD $51 million (City of Winnipeg Citation1997). Without the flood control system, the river stage through the city would have been 3 m higher than the actual permitted 1997 peak and 1.3 m higher than in the 1950 disaster. Under those conditions, the damages that would have been incurred can only be guessed at. A “conservative” estimate by the City of Winnipeg placed it in the CAD $3.5–4 billion range (City of Winnipeg Citation1997), but Warkentin (Citation1997) suggested that the figure might be 2–3 times greater. In the Manitoba portion of the valley, 5507 claims for were filed with Manitoba Emergency Management Organization under the 1997 Disaster Assistance Financial Assistance Program, including municipal claims (Province of Manitoba Citation2000). Almost half of the dollar value of the awards was within the Rural Municipality of Ritchot, the Municipality immediately upstream of the Floodway where the depth of inundation was greatest and which contained the flooded communities of Ste. Agathe and Grande Pointe (Haque et al. Citation2001).
In the US, flood protection works were much less well developed than in Manitoba, and the more rapid flood formation allowed less preparation time. As a consequence, damages were far greater, despite a smaller population and infrastructure “in harm’s way.” Ninety percent of the damages occurred in the adjacent cities of Grand Forks, ND, and East Grand Forks, MN, where 85% (almost 13,000) of all structures were damaged, 1300 homes were destroyed and 55,000 people were evacuated (Todhunter Citation2001). Although their combined populations were 10 times smaller than Winnipeg’s, Shelby (Citation2003) placed the final damage estimate in Grand Forks–East Grand Forks at CAD $3.5 billion and overall costs and damages in the US portion of the basin exceeded US $4 billion (Todhunter Citation2001). In all, about 75,000 people were evacuated in the United States (Red River Basin Task Force Citation1997), nearly three times as many as in Manitoba.
The very short lead time for evacuating animals also caused greater agricultural losses in the US than in Manitoba. Shelby (Citation2003) reported that more than 10,000 head of cattle (including unborn calves), 1255 hogs, 3422 sheep and 1000 chickens and turkeys were lost to the combination of blizzard and flood. In Manitoba, farmers had several weeks’ longer lead time and very few domestic animals died. Ninety-four operators were assisted in the relocation of 7754 cattle, 211,664 poultry, 33,342 swine, 3000 mink, 213 goats and 97 horses away from the flooded area (CitationClayton 1997). In some cases, poultry were sold and other farm animals were successfully protected by dikes.
Flood benefits
It may seem paradoxical to find benefits in such a costly and traumatic natural disaster, but such has been the pattern in Manitoba. Every flood has led to measures which improve the ability of the region to cope with flooding. Most of the existing flood control system was constructed as a result of the 1950 flood. A flood throughout the valley in 1966 precipitated the construction of the community ring dikes. Another large flood in 1974 led to federal–provincial agreements about damage compensation, and a 1950-level flood in 1979 resulted in improved design standards for dikes in the valley, stronger land use controls and the protection of many individual properties. The cumulative effectiveness of these measures was certainly evident in 1996 when another approximately 1950-level flood inundated a large area but passed through the flood-proofed valley without serious consequence, other than the inconvenience of routine measures such as dike closures, a small number (about 100) of evacuations and disruption of traffic southward along the main highway between Winnipeg and the US entry point at Emerson. The total cost of this large flood in Manitoba was only CAD $12 million (Manitoba Government Information Services Citation1996).
The discharges and damages in the 1997 flood were far beyond previous experience. The existing flood control system had been stretched to the limit to avert unimaginably more catastrophic damage by the narrowest of margins; as was noted above, the situation could easily have been much worse. With high Assiniboine flow, strong, persistent south winds, and/or rain during the rising phase of the flood (all of which were within the range of expectable April/May conditions), the 1997 Red River might have been more like that of 1826, estimated to have been approximately 40% larger (6400 m3/sec) and far beyond the capacity of the existing control system. Under those conditions, damages would have run into the many billions and the evacuation of a large proportion of Winnipeg’s 630,000 residents would have been necessary. Even with everything working as intended, the experience was so traumatic and the consequences of failure so large that an extraordinary post-flood response was called for.
An agreement between the provincial and federal governments led to a dramatic, very costly improvement in the overall flood protection infrastructure which should ensure that future failure would be extremely unlikely. The most important measure was the enlarging of the Floodway (mostly by widening) which increased its normal design capacity from 1700 to 4000 m3/sec. Other components of the overall Floodway Expansion Project were the renovation of the machinery which operates the Floodway gates, improvements to the Floodway outlet at Lockport, improvements to the dikes along the Floodway and the West (Z) Dike, replacement or renovation of bridges and modifications to numerous utility crossings. Within Winnipeg, pumping stations and gates on the storm sewer system were improved, low-lying individual properties were protected and many sections of riverbanks were stabilized with rip-rap. These measures increased the reliable protection for Winnipeg from the approximately 100-year level of the 1997 flood to about the 700-year event (7700 m3/sec), 20% larger even than the historic 1826 flood (Floodway Expansion Authority Citation2004).
In the valley to the south of Winnipeg, ring dikes were constructed around 10 more communities, bringing the total to 18, and the height standards for ring dikes and flood proofing were increased to 0.6 m above the 1997 level. About 1700 individual homes, businesses and farm buildings were diked or raised on pads; where this was impractical, some houses were bought out and removed.
Since 1997, these and other flood protection projects throughout southern Manitoba have cost about CAD $1 billion. Of this, the Floodway Expansion Project accounted for CAD $665 million, approximately equivalent in 1970 dollars to the $110 million cost of the original flood protection system. In March 2014, it was announced that this project had actually been completed under the budgeted amount, permitting CAD $38 million to be reallocated to other flood protection measures elsewhere in southern Manitoba (Government of Manitoba Citation2014).
Summary
The Red River Valley of Manitoba, North Dakota and Minnesota experienced a record flood in May 1997 which came to be known as the “Flood of the Century.” Although antecedent conditions at the end of March indicated only a moderate, easily handled flood with minimal consequences, a major snowstorm in the first week of April changed the situation drastically. Record snow and rain fell over the entire basin upstream of Winnipeg. Record discharges occurred all along the river from southern North Dakota to Winnipeg, where the peak discharge was about 50% greater than the previous highest measured flow. The flood was probably the second largest event in two centuries of documented flood history, exceeded only by the epic 1826 flood in the early years of the Red River Settlement and approximately equal to the historic flood of 1852. About 1840 km2 in Manitoba were inundated in a water body 100 km long with a maximum width of about 40 km. The flood required the evacuation of 27,400 Manitobans (mostly in the Red River valley upstream of Winnipeg) and set off one of the largest Emergency Measures operations in Canadian peacetime history, but existing flood control structures, flood proofing and damage reduction programs, and emergency measures prevented losses which could have been 10 times greater. Furthermore, the peak stage might also have been greater except for some good luck from manageable flows on the Red’s major tributary, the Assiniboine River, and favourable weather during the flood itself. In North Dakota and Minnesota, where flood control/flood proofing measures were less well developed, damages were many times greater, and almost three times as many people were evacuated despite a much smaller population and infrastructure. The legacies of the flood in Manitoba include improved flood control and flood-proofing measures which increased the protection for Winnipeg from the 100-year to the 700-year flood level. In the Red River valley outside Winnipeg, ring dikes were constructed around 10 more communities and flood-proofing standards throughout the valley were raised to a level 0.6 m above the 1997 peak stage.
Acknowledgements
The writer is grateful to Weldon Hiebert, the University of Winnipeg cartographer, for his preparation of the figures.
References
- Anderson, M. E., and D. T. Braatz. 1997. An overview of hydrologic forecasting for the 1997 record flooding on the Red River of the North in the United States. In Proceedings of the Red River Valley’97 Flood symposium. Winnipeg, MB: Canadian Water Resources Association.
- Blais, E., S. P. Clark, K. Dow, W. Rannie, T. Stadnyk, and L. Wazney. 2015. Background to flood control measures in the Red and Assiniboine River basins. Canadian Water Resources Journal. doi:10.1080/07011784.2015.1036123
- Brooks, G. R., L. H. Thorleifson, and C. F. M. Lewis. 2005. Influence of loss of gradient from postglacial uplift on Red River flood hazard, Manitoba. Canada. The Holocene 15 (3): 347–352.
- Burn, D. H., and N. K. Goel. 2001. Flood frequency analysis for the Red River at Winnipeg. Canadian Journal of Civil Engineering 28(3): 355–362.
- City of Winnipeg. 1997. Brief to the Manitoba Water Commission, 2 December.
- Clayton, H. 1999. Emergency preparedness in the Province of Manitoba. In Proceedings of the Red River Flooding: Decreasing Our Risks conference, XV-1–XV-24. Winnipeg, MB: Canadian Water Resources Association.
- Etkin, D., ed. 2010. Canadians at risk: Our exposure to natural hazards. Toronto: Institute for Catastrophic Loss Reduction, Research Paper Series Number 48.
- Floodway Expansion Authority. 2004. Proposed floodway expansion project: Executive summary of environmental impact statement. Winnipeg, MB, 29 pp.
- Government of Manitoba. 2009. Manitoba 2009 spring flood report. August.
- Government of Manitoba. 2013. 2011 flood: Technical review of Lake Manitoba, Lake St. Martin and Assiniboine River water levels.
- Government of Manitoba. 2014. News release, 18 March. www.news.gov.mb.ca.
- Haque, C. E., B. Tait, and M. F. Myers. 2001. Institutional assistance for flood-disaster recovery and its impact on resilience in the Red River basin. In Prairie Perspectives 4, ed. D. Munski, 87–105. Grand Forks, ND: The University of North Dakota.
- Hardin, N., 1999. Red River flood frequency and risk analysis. In Proceedings of the Red River Flooding: Decreasing Our Risks conference, IV-1–IV-18. Winnipeg, MB: Canadian Water Resources Association.
- International Joint Commission (IJC). 2000. Living with the Red. A Report to the Governments of Canada and the United States on reducing flood impacts in the Red River Basin. Ottawa and Washington: International Joint Commission (IJC), 82 pp.
- International Red River Basin Task Force. 1997. Red River flooding: Short-term measures. Interim report of the International Red River Basin Task Force to the International Joint Commission. Ottawa and Washington: International Joint Commission (IJC), 65 pp.
- Manitoba Government Information Services. 1996. News release, 16 July. www.news.gov.mb.ca.
- Manitoba Natural Resources. 1997a. 1997 flood update. 1 May. www.gov.mb.ca/flood.
- Manitoba Natural Resources. 1997b. 1997 flood update. 16 May. www.gov.mb.ca/flood.
- Manitoba Natural Resources. 1997c. Chronology of events. 16 May. www.gov.mb.ca/flood.
- Manitoba Natural Resources. 1997d. Facts about the Brunkild “Z” Dike. EMO #61. 20 May.
- Manitoba Natural Resources. 1997e. Submission to the Manitoba Water Commission: Background information concerning Manitoba Natural Resources involvement in the 1997 flooding of the Red River valley. Winnipeg, MB.
- Manitoba Water Commission. 1998. An independent review of actions taken during the 1997 Red River flood. Winnipeg, MB.
- McNeil, D., and B. MacBride. 1997. The City of Winnipeg flood planning, preparations and operations. In Proceedings of the Red River Valley’97 Flood symposium. Winnipeg, MB: Canadian Water Resources Association.
- Mudry, N., P. J. Reynolds, and H. B. Rosenberg. 1981. Post-project evaluation of the Red and Assiniboine River flood control projects in the Province of Manitoba, Canada. In Proceedings of the International Commission on Irrigation and Drainage, Special Session R.9, 147–178. Grenoble, Switzerland,
- Province of Manitoba. 2000. News release: 1997 flood disaster program to close March 31. 9 March. www.news.gov.mb.ca.
- Rannie, W. F. 1998a. A survey of hydroclimate, flooding, and runoff in the Red River basin prior to 1870, Geological Survey of Canada Open File 3705. Ottawa, ON. 189 pp.
- Rannie, W. F. 1998b. The 1997 Red River flood in Manitoba, Canada. In Prairie Perspectives 1, ed. J. Lehr and J. Selwood, 1–24. Winnipeg, MB: Department of Geography, University of Winnipeg.
- Rannie, W. F. 2002. The role of the Assiniboine River in the 1826 and 1852 Red River floods. In Prairie perspectives 5, ed. B. Thraves, 56–75. Regina, SK: Department of Geography, University of Regina.
- Red River Basin Investigation. 1953. Report on investigation into measures for the reduction of the flood hazard in the Greater Winnipeg Area. Appendix D, Flood magnitudes and frequencies. Ottawa: Canada Department of Resources and Development, Engineering and Water Resources Branch.
- Shelby, A. 2003. Red River rising: The anatomy of a flood and the survival of an American city, St. Paul, MN: Borealis Books, 265 pp.
- Todhunter, P. E. 1998. Flood hazard in the Red River Valley: A case study of the Grand Forks flood of 1997. North Dakota Quarterly 65(4): 254–275.
- Todhunter, P. E. 2001. A hydroclimatological analysis of the Red River of the north snowmelt flood catastrophe of 1997. Journal of the American Water Resources Association 37(5): 1263–1278.
- Warkentin, A. A. 1997. The Red River flood of 1997: An overview of the causes, predictions, characteristics and effects of the Red River Flood of the Century. In Proceedings of the Red River Valley ’97 Flood symposium. Winnipeg, MB: Canadian Water Resources Association.
- Wazney, L., and S. P. Clark. 2015. The 2009 flood event in the Red River basin: Causes, assessment, and damages. Canadian Water Resources Journal. doi:10.1080/07011784.2015.1009949