Abstract
In late June 2013, heavy rainfall and rapidly melting alpine snow triggered flooding throughout much of the southern half of Alberta. Heavy rainfall commenced on 19 June and continued for 3 days. When the event was over, more than 200 mm and as much as 350 mm of precipitation had fallen over the Front Ranges of the Canadian Rocky Mountains. Tributaries to the Bow River including the Ghost, Kananaskis, Elbow, Sheep and Highwood, and many of their tributaries, all reached flood levels. The storm had a large spatial extent causing flooding to the north and south in the Red Deer and Oldman Basins, and also to the west in the Elk River in British Columbia. Convergence of the nearly synchronous floodwaters downstream in the South Saskatchewan River system caused record high releases from Lake Diefenbaker through Gardiner Dam. Dam releases in Alberta and Saskatchewan attenuated the downstream flood peak such that only moderate flooding occurred in Saskatchewan and Manitoba. More than a dozen municipalities declared local states of emergency; numerous communities were placed under evacuation order in Alberta and Saskatchewan. The media reported that more than 100,000 people needed to evacuate their homes, and five people died. At CAD$6 billion, the Province of Alberta estimated that it was the costliest natural disaster in Canadian history. At their peak, the water levels were the highest in the past 60 years, and nearing those of historic events of the late 1800s and early 1900s where records exist. There was major damage to infrastructure roads, bridges and culverts. Downtown Calgary was inundated, as were many communities such as High River and Bragg Creek. Debris flows and debris torrents affected communities such as Canmore and Exshaw, and isolated many mountain communities including closing the TransCanada Highway for several days. Environment Canada called it the “Flood of Floods.” Insured losses of CAD$2 billion included thousands of cars and homes demolished and damaged by backed-up sewers. Flood damage losses and recovery costs from the flood are projected to exceed CAD$6 billion.
Vers la fin juin 2013, d’intenses précipitations et la fonte rapide de la neige alpine ont entraîné des inondations dans une bonne partie de la moitié sud de l’Alberta. Les pluies abondantes ont commencé le 19 juin et n’ont pas cessé pendant trois jours. Une fois l’événement terminé, plus de 200 mm et jusqu’à 350 mm de précipitations s’étaient abattues sur les chaînons frontaux des Rocheuses canadiennes. Les affluents de la rivière Bow, y compris les rivières Ghost, Kananaskis, Elbow, Sheep, Highwood et bon nombre de leurs affluents, ont tous atteint le niveau des crues. La tempête avait une vaste étendue spatiale, ce qui a inondé le nord et le sud du bassin des rivières Red Deer et Oldman, de même que la partie ouest de la rivière Elk en Colombie-Britannique. La convergence des eaux de crue à peu près synchrones, en aval de la rivière Saskatchewan, a provoqué des apports d’eau d’un niveau record depuis le lac Diefenbaker jusqu’au barrage Gardiner. Les déversements d’eau de barrages en Alberta et en Saskatchewan ont permis d’atténuer le débit de pointe de crue en aval, de telle sorte que seulement des inondations modérées se sont produites en Saskatchewan et au Manitoba. Plus d’une douzaine de municipalités ont déclaré l’état d’urgence; de nombreuses collectivités ont dû se conformer à un ordre d’évacuation en Alberta et en Saskatchewan. Plus de 100 000 personnes ont été contraintes d’évacuer leur domicile et les autorités ont déploré le décès de cinq personnes. Les médias ont souligné qu’il s’agissait de la catastrophe naturelle la plus coûteuse de l’histoire du Canada. Les sommets atteints par les niveaux d’eau étaient les plus élevés des 60 dernières années et ils se rapprochaient de ceux enregistrés au cours d’événements historiques vers la fin des années 1800 et le début des années 1900, là où des dossiers peuvent en témoigner. Cet événement a provoqué des dommages majeurs aux infrastructures telles que les routes, les ponts et les ponceaux. Le centre-ville de Calgary a été inondé, tout comme de nombreuses collectivités comme High River et Bragg Creek. Des coulées de débris et des torrents de débris ont frappé des hameaux et de petites localités, notamment Canmore et Exshaw, et ont isolé de nombreuses collectivités situées en région montagneuse. La Transcanadienne a même dû être fermée pendant plusieurs jours. Environnement Canada a qualifié d’« inondation des inondations » cette catastrophe naturelle. Des milliers de maisons et de voitures ont été démolies et endommagées par des refoulements d’égout et ces pertes se chiffrent à un montant record de CAD$2 milliards de dollars en sinistres assurés. Par ailleurs, les économistes estiment qu’en tout et partout l’inondation aura entraîné des dommages, des pertes et des coûts de rétablissement s’élevant à plus de CAD$6 milliards de dollars.
Geographical and hydrological setting
The storm affected an area from the Oldman River north to the Red Deer River and from eastern British Columbia west to Calgary (Figure ). All tributaries to the Bow River in the Front Ranges of the Rocky Mountains were affected, and many streams in the Oldman and Red Deer river basins as well. Flooding was widespread and manifested in different ways throughout the region. Inundation flooding affected the communities of Calgary, Bragg Creek and High River, by the Bow, Elbow and Highwood Rivers, respectively. In other areas, such as Exshaw and Canmore, the flood mechanism involved debris flow and debris torrents on alluvial fans of local creeks or groundwater-induced flooding. Debris mechanisms caused damage to the TransCanada Highway in Banff National Park and Canmore, and highways and secondary roads in the Kananaskis, Elbow, Sheep and Highwood headwaters. Flooding in all tributaries was essentially simultaneous, and the time of travel of the flood peak along the Bow River reflects this synchrony. Downstream, record high-water releases from the Gardiner Dam on Lake Diefenbaker along with high flows on the North Saskatchewan River resulted in evacuation orders for the community of Cumberland House, 960 km east on the Saskatchewan River near the Manitoba border (Shook and Pomeroy 2015, this issue). The flood waters from the eastern slopes of Alberta eventually drained into Lake Winnipeg and the Nelson River flowing to Hudson Bay.
Figure 1. The Red Deer, Bow, Oldman and Elk river basins, showing the locations of the Water Survey of Canada gauging stations used, Marmot Creek Research Basin, and the places affected by the flood.
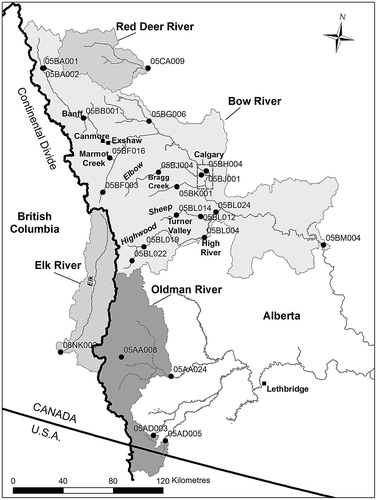
Peak flows in all streams in this region usually occur in May and June as a result of the melt of snow accumulated over the winter (Osborn Citation1987; Wilson Citation1987); however, peak flows that produce major floods in the region (Oldman and Red Deer river basins) such as in 1995 and 2005 are primarily generated by rainfall runoff (Shook Citation2015, this issue). In the 2013 event, the heavy rain generated rainfall runoff at low and middle elevations, but was supplemented by rain-on-snow runoff at high elevations due to a late lying snowpack which had been supplemented by a late May snowstorm. Similar magnitude events have occurred in the past, but not recently. Floods in the Bow River occurred in 1879, 1884 (Dawson Citation1886), 1897, 1902 and 1909 (Sauder Citation1914), 1923 (Ford Citation1924), 1929 (Hoover Citation1929) and 1932 (Hoover and MacFarlane Citation1932). The rainstorms that generated the floods in 1929 and 1932 appear to be similar in extent and volume to the event of 2013 (Hoover Citation1929; Hoover and MacFarlane Citation1932).
Causes of the flood
Antecedent conditions
The winter preceding the flood had above-normal precipitation, but the peak snow water equivalents (SWE) were generally between the 25th and 75th quantiles at local Alberta Environment and Sustainable Resource Development (AESRD) snow pillows, and fifth highest of the previous 6 years of University of Saskatchewan (U of S) snow surveys in the upper elevations of Marmot Creek Research Basin (MCRB), as shown in Figure . The SWE was much less than in the preceding year. Once snowmelt began, the melt was slower than average, and the remaining SWE exceeded the 75th quantile after 10 June at the AESRD Little Elbow Summit (05BJ805) snow pillow in the headwater region for the Elbow, Kananaskis and Highwood rivers. The spring was wet with several storms during May; Calgary and some foothill climate stations received rainfall amounts on 23 and 24 May that were greater than those experienced during the flood. For instance, on 25 May, 96 mm of rain was recorded at the Livingstone Ranger Station in the Kananaskis. This rain fell as snowfall at higher elevations, which can be seen in Figure a increasing the late May SWE to the levels of early May. Spring warming started melting the snowpack in May, and snowcover depletion was observed to occur by U of S for many lower and middle elevations in MCRB in this month, with complete depletion below 2000 m by the time of the flood. Groundwater levels measured at AESRD observation wells in MCRB were observed to be higher than average (Buttle et al. 2015, this issue), indicating a reduced capacity to store additional water from rain and melting snow. Volumetric soil moisture measurements by the U of S at middle elevations at MCRB in the front ranges show saturated conditions until about 5 days before the flood, and slow drying just before the flood. At high elevations (MCRB) and wetland valley bottoms (Sibbald wetland; Dr. Cherie Westbrook, personal communication) soils were saturated, frozen or both.
Figure 2. Snow accumulation in the region. (a) Snow water equivalent for the snowpillow at 2120 m at Little Elbow Summit (05BJ805); (b) snow water equivalent from University of Saskatchewan snow surveys just above the treeline (2325 m) on Fisera Ridge in Marmot Creek Research Basin, Kananaskis Valley.
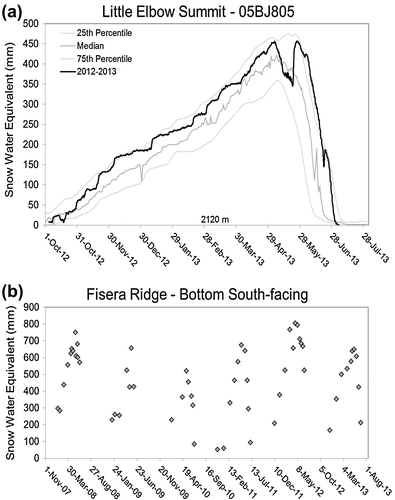
Event mechanisms
Large-scale atmospheric conditions played a crucial role in the flooding event (Milrad et al Citation2015). The storm of 19–22 June featured an intense and slow-moving moist upper low that parked itself over southern Alberta, delivering heavy rains (Figure ). To the south, a large high-pressure system that was stalled over Colorado contributed to hot, dry conditions with record forest fires in that state, and to the north a high-pressure system stalled over Yukon contributed to record high temperatures in northwest Canada and Alaska. An upper-level cut-off low was situated just off the west coast on 18 June and it drifted slowly eastward over the next few days. This configuration produced an upper-level flow over southwestern Alberta that was conducive for ascending air. Low surface pressures were simultaneously present in the lee of the mountains. On 18 June, there was a surface low centre situated in Idaho that drifted slowly northward; it was located over southern Alberta by 20 June and started to move eastward out of the area by 22 June. The circulation around this low centre produced winds with an easterly, and therefore upslope, component over the southern Alberta foothills that persisted for more than 36 h. This upsloping air was moist and warm which led to high freezing levels in the atmosphere (approximately 3.5 km above sea level). Rainfall therefore occurred at high elevations in the mountains, as opposed to the more typical alpine snowfall from early summer storms in this region. This moist air was also quite unstable so that convection broke out over many parts of southern Alberta including over the prairies and foothills, but only over a small section of the front ranges. Convection contributed to pockets of very high precipitation amounts such as one in excess of 300 mm that centred on the Highwood River, Elbow River, and Kananaskis River headwaters. However, a lack of lightning activity (denoting the absence of convection) occurred over a large area of the front and central ranges that also received high rainfall. An important aspect of this event was the persistence of the upper and lower level circulations. This is in contrast to a major rainfall event which led to catastrophic flooding over southern Alberta and more easterly regions in the summer of 2002. That event was linked with a similar coupling between upper and lower circulations but the system moved eastward appreciably (Szeto et al. Citation2011).
Figure 3. The accumulated precipitation for 19–22 June 2013 in Alberta – calculations and map provided by Alberta Environment and Sustainable Resource Development, Edmonton. Used with permission of Alberta Environment and Parks.
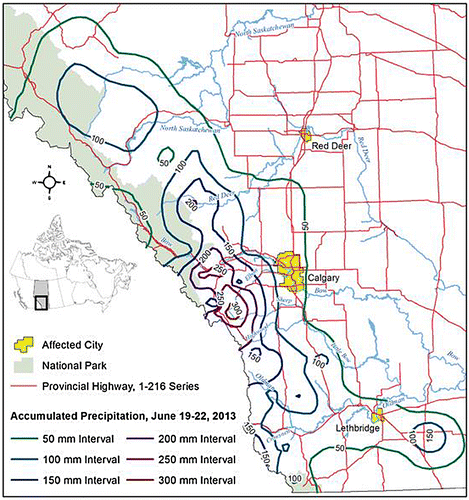
Calgary Airport only received 68 mm of rainfall during the event. However, west of Calgary, in the foothills and mountain headwaters, the precipitation was torrential. While rainfall rates of 3 to 5 mm/h are considered high for this area, the U of S measured rates of 6 to 13 mm/h of rainfall over 24 h at the Kananaskis valley bottom site in MCRB; with 122 mm/d of rainfall over 20 June, these rates are more typical of a tropical storm in quantity and intensity (Figure a). The spatial distribution of accumulated precipitation during the events is shown in Figure and shows the greatest depths in the Front Ranges, specifically the Bow and Ghost valleys near Canmore and Exshaw, the Kananaskis and Spray valleys and the headwaters of the Elbow and Highwood rivers. Burns Creek (at 1800 m and west of High River) recorded the greatest precipitation of the storm: 345 mm. There were no observed elevational gradients in precipitation depth (Figure b) measured by the U of S at MCRB over elevations from 1400 m to 2350 m – this is rare in the long observational record at MCRB, which usually shows higher precipitation at higher elevations (Fang et al. Citation2013) and deviates from observations of precipitation gradients with elevation in the nearby foothills (Smith 2009). At elevations above 2000 m, U of S meteorological stations in MCRB showed that rainfall turned to snowfall on the 21st and 22nd, resulting in snow accumulation which was later visible as a snowline in the Bow and Kananaskis valleys.
Figure 4. Precipitation in Marmot Creek Research Basin, Kananaskis Valley during the flood. (a) Rainfall rates measured at 1436 m in the valley bottom and recorded every 15 min. (b) daily precipitation depths from 19 to 25 June, from 1436 to 2325 m. These depths were measured using a Geonor weighing gauge at 1436 m, a Geonor and a tipping bucket rain gauge at 1844 m, and a Geonor and tipping bucket rain gauge for rainfall and the depth of fresh snow on the ground (assuming density = 100 kg/m3) for snowfall at 2325 m where a transition to snowfall occurred on the 21st.
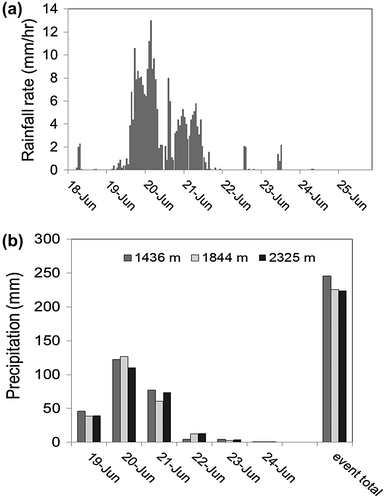
Principal flood processes
The storm event generated floods through several mechanisms. Rainfall runoff was the primary mechanism in the foothills and lower elevations in the front ranges as elevations below about 2000 m were snow-free (Figure ). As shown in Figure , SWE on 19 June in the central and front ranges of the Oldman, Bow, Kananaskis, Elbow, Highwood, Elk, Ghost and Sheep river basins from the US National Weather Service national snow analysis (SNODAS) shows large areas ranging from 150 to 750 mm SWE (National Operational Hydrologic Remote Sensing Center Citation2013). Therefore, at higher elevations in the Oldman and Elk river basins and Bow River tributaries in the Front Range and for a wide range of elevations in the upper Bow River above Banff, there was potential for rain-on-snow mechanisms to contribute substantial runoff. SNODAS calculated snowmelt on 20 June ranging from 25 to over 75 mm over a vast area of the Central Range and Front Range headwaters of the Bow and Oldman river basins. Longwave radiation from warm clouds, sensible heat from warm air, latent heat from condensation of humid air onto the snowpack and advection of energy from initially warm rain melted approximately 100 mm SWE above treeline at MCRB (Figure b). Similar amounts were ablated from AESRD snow pillows in the Kananaskis, Elbow and Bow River basins (Figure a); much of this occurred later on the 20th and early on the 21st during the warmest part of the storm. The transition of precipitation from rainfall to snowfall on the 21st and subsequent warmer weather meant an increase in the snowmelt contributions for the 22nd; post-flood snowmelt contributed to substantial streamflow generation in the upper Bow River for the rest of the month. Near-surface soil moisture storage at middle elevations in MCRB filled in the first day and a half of heavy rain. Snowmelt over partially frozen soils during the event contributed an additional 30% to precipitation delivery to upper elevation spruce/fir/larch forests and alpine zones of MCRB. It is difficult to estimate the rain-on-snow contribution to the flood at large basin scales because of the lack of measurements of alpine snowpacks just before the flood, apart from U of S snow surveys at MCRB and point estimates from the five snow pillows operated by AESRD in the Bow River basin.
Figure 5. Snow water equivalent (cm or inches) as predicted from the US National Weather Service SNODAS national snow analysis for 19 June 2013 in the “Northern Rockies” including parts of the Canadian Rockies headwaters of the Bow, Elk and Oldman river basins (National Operational Hydrologic Remote Sensing Center, Citation2013).
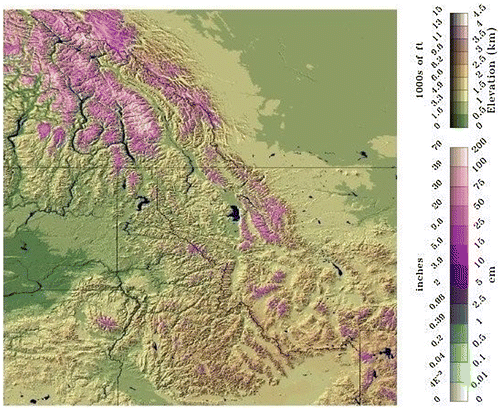
In the headwaters of many of these basins, rain and rain-on-snow generation of runoff triggered debris torrents and debris flows that resulted in large movements of water and sediment. Avulsions on alluvial fans were common as many streams changed courses. The occurrence of these types of events is well documented for the region (e.g. Desloges and Gardner Citation1984; CitationKostachuk et al. 1986).
Extensive groundwater discharge occurred in some localized areas where the water table rose to about the ground surface. This was observed in many valley bottom areas of Canmore and in some other communities with similar settings. Detailed observation of wetland hydrology in Sibbald wetlands east of Marmot Creek show that all beaver dams were eventually overwhelmed and damaged by the high flows, but that the dams provided some storage before they were overwhelmed (Cherie Westbrook, personal communication).
Emergency opening of flood spillways on the Barrier Lake Dam in Kananaskis in the early afternoon on 20 June and for the Cascade Dam on Lake Minnewanka late on 21 June contributed greatly to temporarily increased outflows that were ungauged due to the destruction of downstream gauges by the flows.
Flood time course
The generation of runoff in headwaters occurred nearly simultaneously across the north–south extent of the event. The timings of water levels of the flood peak in upper tributaries on the eastern slopes of the Rockies were spatially synchronous as shown for the Ghost, Highwood, Sheep and other tributaries (Figure ). Synchronized timings on the east slope headwaters and later peak times on the western slopes of the Rockies (Elk River) reflect the dominance of the heavy rainfall and then rapid snowmelt that typified this event, and the westward flow of the low-pressure system into the mountains. Several gauges were destroyed during the flood, which can be seen in Figure where recorded water levels terminate abruptly. The times of the recorded highest water levels are given in Table . The temporal progress of water levels along the Bow River, from Lake Louise to below Bassano Dam, during the event is shown in Figure , where the rapid travel time and short duration of the flood peak are evident. Table and Figure both show later peak times in the mountains than the foothills – this likely reflects the lag associated with flow through snow and the increasing snowmelt contribution from the central ranges as well as the westward progression of the storm.
Figure 6. The timing of tributary water levels in the affected area in 2013 – Crowsnest River at Frank, Elbow River at Bragg Creek, Sheep River at Black Diamond, Cataract Creek near Forestry Road, Highwood River near the mouth and Elk River at Fernie. Manual observations made at these stations are shown with points. Data courtesy Water Survey of Canada.
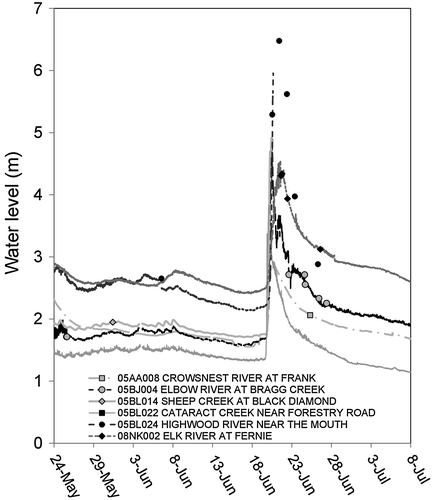
Table 1. Timing of observed highest water level during the 2013 event. Station names shown in italics have some degree of flow regulation.
Statistical assessment
The estimation of the return periods of this event is complex and uncertain for a variety of reasons. Most of the gauges in the affected area do not have sufficiently long records, particularly with respect to the history of the Bow River at Banff and Calgary where a number of large floods are reported to have occurred before stream gauging began. Return periods using only the published records from the Water Survey of Canada might suggest a return period in excess of 500 years. However, when detailed analysis of the long gauged record for the Bow River at Banff is done using knowledge of the floods prior to the start of formal gauging in 1909, a much shorter return period results – this gauge was upstream of the heaviest precipitation but is a natural flow without reservoirs or significant abstractions. The Geological Survey of Canada’s future director, Dr. George Dawson, visited Banff in 1885 and later published his analysis of the flood that had occurred the year before (Dawson, Citation1886). The long period between the series of floods that occurred between 1879 and 1932 and the present, and the published reports analysing those events (Dawson Citation1886; Sauder Citation1914; Ford Citation1924; Hoover Citation1929; Hoover and MacFarlane Citation1932) caution against using only the available Water Survey records since 1909 for estimating return periods for any of the streams in this region. As a demonstration, Figure shows the fitting of the commonly used Log Pearson Type III distribution to the flood series from 1884 to 2013 on the Bow River at Banff. Estimates from 1884 to 1912 were used where available to supplement the Water Survey of Canada gauged flows. Rank analysis on this reconstructed data set suggests the return period of the 2013 event (439 m3/s) might only be on the order of 30–40 years for the Bow River at Banff. If the estimate is done with Log Pearson Type III and the two historical estimates are included, then the return period is ~38 years; if infilling is done to fairly weight the time representing the period that includes the two historical estimates, then the estimated return period is ~41 years.
Figure 8. Estimated return periods for the Bow River at Banff from 1884 to 2013 by rank and fitted using the log Pearson type III distribution (solid line), with 95% confidence intervals plotted as dashed lines. Circle is measured peak flow; triangle is annual maximum of a partial year; hollow square is estimated as noted by the Water Survey of Canada. The 2013 event is third from the right (439 m3/s).
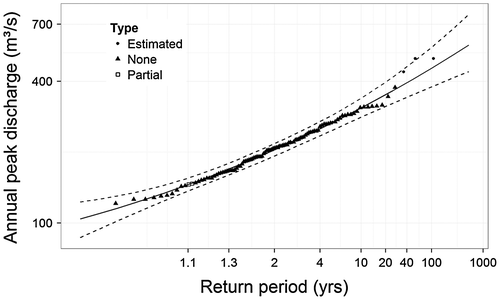
The Log Pearson Type III distribution, commonly used in engineering practice and by many governments, was fitted as an example of how well the data could fit a single frequency distribution; the fit to the Generalized Extreme Value distribution (not shown) was poorer than to the Log Pearson Type III distribution. The large errors fitting these data to any distribution for discharges greater than 400 m3/s mean that great caution must be exercised in any extrapolation to estimate return periods from these data. The peak flow series at Banff derive from a mixture of events, snowmelt, rainfall and rain-on-snow, with the latter two being more rare and of larger magnitude. The uncertainty in the return period estimate shown in Figure is due to the failure of the Log Pearson Type III distribution to fit that of mixed-process high-flow events. This failure is not specific to the Log Pearson Type III distribution or gap infilling, but is a result of deriving flow frequencies from a mixture of flood generation mechanisms. Therefore, a more detailed analysis of return period, stratifying floods based upon flow-generation mechanisms (Waylen and Woo Citation1981), and an examination of possible trends in the flow generation mechanisms would be warranted.
River discharges for a series of gauges for which preliminary discharges were available at the time of writing are shown in Figure . The discharges show the effect on streamflow of the wet weather around 24 May, and the rising hydrographs that reflect the contribution of increasing volumes of mountain snowmelt as weather warmed in mid-June. The hydrographs indicate that the storm had an effect on the upper Bow Valley (Bow River at Lake Louise and Pipestone River near Lake Louise; Bow River at Banff), but the largest impact was downstream of Banff where the river is joined by tributaries from the Front Ranges and then the foothills. Figure a shows this to be a common occurrence; during larger flood events the basin peak discharge expressed as runoff per unit area of the basin (mm/day) is higher at Calgary than at Banff, indicating the importance of the Front Range and foothills during these large events. Analysis of the data in Figure a can be used to classify peak flows by their source area; when the runoff per unit area in the Bow River Basin is greater at Calgary than at Banff, then the peak flow is classified as a “Front Ranges and Foothill event,” and when runoff per unit area is higher at Banff than at Calgary then the peak flow is classified as a “Central Ranges event”. It is evident in Figure a that several large events before 1935 and the 2013 flood were “Front Ranges and Foothill events” and that these are the largest events in the record. When return periods are calculated from rank of the events from 1879 to 2013 and plotted against peak discharge at Calgary it is apparent that up to the 1:10-year event the primary source area is the Central Ranges (upstream of Banff), and for events greater than the 1:15-year event the primary source area is the Front Ranges and Foothills, downstream of Banff (Figure b). The statistical analysis in Figure b also shows substantial deviation from standard flood return period calculations, likely due to mixing multiple populations from flow-generation mechanisms (snowmelt and rainfall or rain-on-snow) and geographical source areas. This demonstrates that a single flood frequency curve cannot be fitted to these data. A return period for the Bow River at Calgary of about 40 years is indicated from the rank analysis – it was likely that this was reduced somewhat from what a natural flow would show by the retention of some floodwater in the Ghost Reservoir and other dams (Cascade, Kananaskis and Spray) in the Bow tributaries upstream of Calgary and below the Bow at Banff.
Figure 9. Time series of Bow River discharges from upstream to downstream. Bow River at Lake Louise, at Banff, at Calgary and below Bassano Dam. Also shown are discharges for the Pipestone River near Lake Louise and Fish Creek near Priddis. Note that discharges downstream of the Bow River at Banff, except for Fish Creek near Priddis, are not natural flows.
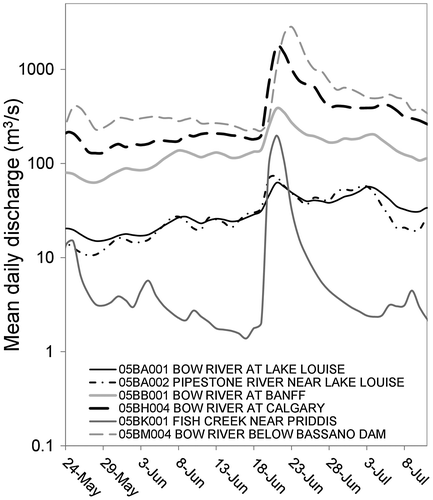
Figure 10. Peak flow source areas for the Bow River and impact on peak flow frequency analysis for Calgary from 1879 to 2013. (a) Annual peak flow time series expressed as runoff per unit area over the gross basin area for the Bow River at Banff and at Calgary. (b) Return period analysis of peak discharge by rank for the Bow River at Calgary fitted to a log Pearson type III distribution (solid line with 95% confidence intervals as dashed lines) and segregated by primary source area; Central Ranges (upstream of Banff) or Front Ranges and foothills (downstream of Banff).
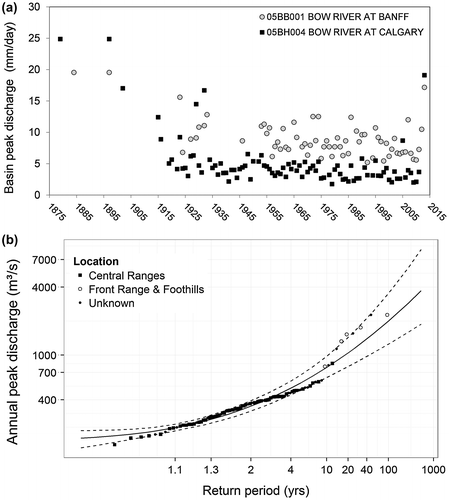
Flood damages
In the mountain headwaters, damage from high- and fast-flowing water was combined with that from erosion of channels, shifting channels and avulsion onto low-lying areas. Many of these resulted in destruction of hydrometric stations, bridges, highways and culverts. The 51-year-old Water Survey of Canada V-notched weir streamflow gauge at MCRB (Bruce and Clark Citation1965) and three U of S MCRB hydrometric stations and a number of autosamplers for an isotope study were destroyed by debris flows on the 20th. The Marmot Creek channel shifted as much as 30 m, and showed both erosion and avulsion. This was endemic in the headwaters where exceptionally heavy rainfall combined with alpine snowmelt. Notable cases include the impacts on the TransCanada Highway at Carrot Creek in Banff National Park, and the impacts on the homes near Cougar Creek, the TransCanada Highway, Canadian Pacific Rail mainline and #1A highway in Canmore and Exshaw. Such events were widespread in the Kananaskis Valley as well and caused the washout of bridges, campgrounds, parking areas and trails.
There is little doubt that the Lake Minnewanka reservoir created by the Cascade Dam stored some floodwaters and reduced overall discharge downstream early in the flood event. However, the spillway of Cascade dam had not been operated since construction in the early 1940s and so when opened had consequences downstream with the washing out of the Canadian Pacific Rail mainline, severing of the TransCanada Highway, and disruption of the hydroelectric facility and electrical supply to the town of Banff. The Barrier Dam spillway had also not been operated since construction in 1946, and when opened during peak flow on 20 June caused disruption to the hydroelectric facility, extreme channel erosion in the Kananaskis River and higher inflows to the Ghost Reservoir in the Bow River upstream of Calgary. The “restoration” of the Cascade River by opening the spillway on the Cascade Dam resulted in fish migration into the channel after the flood and then fish abandonment when the spillway was closed later in the summer. Though Parks Canada attempted to rescue trapped fish, the Cascade River became a feasting ground for local bears and this popular area therefore remained closed to human visitation for much of the summer and fall of 2013.
Despite the relatively short 40-year return period of this event on the Bow River at Calgary, thousands of businesses and buildings on the floodplain in Calgary were affected. In Stampede Park, stables and barns were under more than 2 m of water, and in the Saddledome, water levels reached the 10th row of the hockey arena. On an island in the Bow River, the Calgary Zoo became partially submerged and officials moved several large exotic animals to an alternate facility in extremely challenging conditions that included the near-escape of several hippopotamuses into the floodwaters. Also in Calgary, the inundation of the flood plain from the Bow and Elbow Rivers eroded roads, rail lines, pedestrian bridges and riverside pathways.
Flooding of the Highwood River caused water to rise over the top of vehicles in the Town of High River; more than 150 people had to be rescued from the rooftops of their homes. All 13,000 residents of High River were ordered to evacuate on 20 June, and the community was largely abandoned within 3 days as the town suffered what local officials called “unprecedented” damage. Some neighbourhoods, which were not anticipated to flood, flooded due to the Highwood River spilling into an internally drained depression, creating a large pond. Water was removed from this filled depression by pumping; it would likely take several years for evaporation and deep percolation to drain the pond.
The communities of Crowsnest Pass, Lethbridge, Black Diamond, Turner Valley, Cochrane, Sundre and Rocky View County declared states of emergency on 20 June. More communities declared a state of emergency on 21 June, including several First Nations reserves. The City of Medicine Hat, downstream from the confluence of the Bow and Oldman Rivers, was also hit with significant flooding. The floodwaters caused the evacuation of Cumberland House far downstream on the Saskatchewan River in Saskatchewan, near the Manitoba border, due to unprecedented high releases of water through the Gardiner Dam spillway from Lake Diefenbaker. Flooding was largely averted in Saskatoon and surrounding farmland downstream of Gardiner Dam due to attenuation of the flood wave by dam operation (Shook and Pomeroy Citation2015, this issue) and Saskatchewan's 1:500 year flood plain designation. Five people were reported to have died during the flood, and there were reports of more fatalities after the event due to post-flood health impacts. Thousands of Canadians were affected directly and indirectly. The floods have left visible changes to the landscape in southern Alberta; many channels were substantially modified during the flood. A spectacular example is in the upper tributaries of Ghost River which washed out near to the diversion channel into Lake Minnewanka; the diversion channel itself filled in with sediment which blocked flow into the reservoir. A breached berm then diverted the river into Lake Minnewanka, which created enough concern for the Cascade Dam spillway to be opened for the first time in its history, as discussed above.
Flood benefits
At present, it is impossible to assess the benefits of this event. Certainly, there are ecological processes that will have been strongly affected by the inundations of wetlands and other areas that would result in the recharge of riparian and upland wetlands. These renewal processes were reported by provincial and national park officials to be showing benefits to local fish populations in the summer of 2014. Removal of old bridges and trails in parks give park officials the opportunity to reconsider access to wilderness areas. The flood damaged a former water supply reservoir for the town of Banff – the damaged dam has since been removed by the town and Parks Canada, restoring 40 Mile Creek to nearly its natural state.
Observing a flood helps immensely in measuring and estimating the behaviour of the next flood. New rating curves that reduce the considerable uncertainty at high flows have been established for many Water Survey of Canada gauges in part of the South Saskatchewan River basin as a result of the flood and its high flows. Previous floods of this magnitude on the Bow River were not gauged, and so extension of rating curves to the high stages experienced in this event was very problematic. Similarly, the area and duration of inundation in urban areas were previously estimated using hydraulic models, but now the extent of inundation is well documented and this can be used to calibrate and evaluate the performance of future hydraulic flood modeling. Detailed observations of the flood in Marmot Creek Research Basin have provided insight into rain-on-snow processes in the region that will eventually contribute to better hydrological simulation of these events in the Canadian Rockies.
The potential for greatest benefits to society must come from a re-evaluation of the level of preparation for floods and the degree of warning that was available for this flood. Alberta only protects to the 1% flood event which is recognized as the lowest level of flood protection that is politically acceptable (Lord, Citation2011). The substantial destruction in Calgary resulted from a flood with a return period of approximately 1:40 years. With the continued development in this region of Alberta, it was hoped that discussions of higher levels of protection and risk reduction from avoidance might occur following this event. Implementation of greater protection using channelization and debris nets along mountain creeks in Canmore and berms along the flood plains in High River and Calgary to date (2015) may reduce damage from smaller high-flow events, but not large floods such as that of 2013. Discussions of “dry dams” upstream of Calgary borrow from the experience of the Miami Conservancy District in preventing floods around Dayton, Ohio, after the “Great Flood” of 1913, but have not been implemented yet, and innovative solutions such as a tunnel from the Glenmore Reservoir to the Bow River downstream of downtown Calgary to permit the bypassing of floodwaters from the Elbow River do not appear to be progressing. The impact of headwaters basin forest protection and wetland restoration on flood generation in the region has not been fully investigated or considered, though a modelling study by Pomeroy et al. (2012) in MCRB suggested up to a 23% increase in peak flows associated with complete forest cover removal in Marmot Creek. While discussions have occurred, progress in implementing risk reduction is uneven; in Canmore, homes along Cougar Creek were almost entirely rebuilt despite creek-bed mitigation that cannot handle a debris flow the size of the 2013 event. In Calgary, very few homes were removed from the floodplain, and in Kananaskis Country CAD$18 million was recently earmarked to rebuild a flood-damaged golf course in its floodplain location along Evan Thomas Creek. Only in High River were whole sub-divisions in a floodplain declared untenable and slated for removal.
The lack of the promotion of a credible coordinated and enhanced scientific research and mitigation implementation effort into the causes and future deterrence of mountain generated floods upstream of Calgary is a wasted opportunity to learn from the most expensive natural disaster in Canadian history and use this knowledge to prevent its repeat. After the devastating floods of 1913 in Ohio and Indiana, regional and national governments inspired by engineer Arthur E. Morgan developed the Miami Conservancy District, which was a model for others such as the Muskingum Conservancy District and the Tennessee Valley Authority and led to internationally recognized innovations in watershed management, dam construction, reservoir operation and flood routing, and a network of agricultural research basins across the United States (Williams Citation2013). After the devastating floods in Southern Ontario from Hurricane Hazel in 1954, the Ontario watershed conservation authorities were established and a substantial federally led national hydrometeorological research program and flood damage reduction program resulted. Whilst there have been many mitigation proposals and local discussions in Alberta, and a few scientists from universities, provincial and federal governments have been able to focus modest existing resources towards this, no enhanced coordinated research program has been established by senior governments to look into the fundamental hydrometeorological and hydrological causes and prediction of the flood, and avoidance of future flood damage. Nor have coordinated watershed planning mechanisms such as a conservancy district for the Bow or Oldman River basins been proposed. This is similar to the lack of response to the 2005 Alberta flood documented by Shook (Citation2015, this issue).
Despite the concerns listed above, it is encouraging to note that the federal and Alberta governments are reviewing and intending to improve their operational procedures and observations for weather and flood forecasting, including improved coordination of weather forecast information to flood forecasting. This would be an improvement given that during the 2013 event, flood forecasts for headwater communities were generally issued hours after evacuations had commenced and after major infrastructure had been destroyed by high flows. Future implementation of credible flash flood warnings from coupled atmospheric and hydrological models in the region could reduce infrastructure and property damage and save lives.
What would a coordinated response to the flood of 2013 look like? There are three main categories of responses that could be implemented upstream of Calgary and even nationally to reduce the exposure to and damage from floods: better prediction, avoidance and active mitigation.
• | Better prediction involves improved seasonal weather predictions, better severe weather prediction and precise forecasting for rivers, streams, overland flow, groundwater and lakes. Better prediction permits short-term adaptation by sandbagging for floods, managing reservoirs, and conducting community evacuations in an orderly and safe manner. | ||||
• | Avoidance involves land-use zoning of appropriate locations for development and conservation based on careful and continuously updated floodplain mapping – without prejudice as to whether the floods are caused by rainfall, snowmelt, overland flood, ponding, streamflow, groundwater, lake level change, sea level change, wind storms or bank erosion. All water sources are connected and so the sources of flooding should not be considered separately. | ||||
• | Active mitigation involves dikes, flood-control structures such as dams and detention ponds, channel modifications, and management of agricultural fields, urban development, forests and wetlands in the watershed. These structures, modifications and land-use practices must be designed and evaluated for their performance under known or anticipated extremes of water flow. |
Advancing the science and technology of prediction is not enough – the technological tools have to be used effectively by trained professionals with comprehensive institutional support. Canada is exceptional amongst developed nations in that we have fragmented responsibility and capability for water prediction among multiple levels of government. The federal government collects most weather and water data and predicts weather and drought – but it does not generally forecast floods, streamflow, lake levels and water supply. The provinces have constitutional authority for water resource management and with this the obligation to predict floods, map flood zones and deliver frontline disaster management, reimbursement and repair. As a result, the flood of 2013 that started in Alberta and British Columbia and moved downstream from the eastern slopes of the Rockies across Alberta and Saskatchewan into Manitoba along the Saskatchewan River system, and from the Elk River into the Columbia River system, was forecast as weather by the federal government and then as streamflow by four different provinces, with very different methods, attention and capabilities. The flood of 2013 shows that Canada needs to develop a national strategy that would involve coordinated, integrated river-basin-wide delivery of improved prediction and information to support flood forecasting, water management and implementation of flood avoidance and active mitigation strategies. This can start with unified weather and water prediction models whose results are provided in real time so that the provinces can manage their water with the best possible information. These prediction systems need to be supported by enhanced weather and water observations from ground and satellite. Unified predictions are best accomplished by integrated river basin forecast centres; these have been shown to be very successful in the United States. Some examples even integrate university scientists along with multiple federal and state agencies to provide a powerful science-based water prediction and management centre.
Advance flood warning informed by unified water prediction systems would have helped in short-term flood mitigation and a more orderly evacuation in Alberta, British Columbia and Saskatchewan in the June 2013 flood. These same systems can help to map future flood plains, devise safer reservoir management operations, and allow better management of forests and agricultural lands for long-term flood and drought mitigations.
Summary
Rainfall in the June 2013 event was similar in intensity and depth to tropical rain, reflecting the origin of the air masses. The storm covered a large area of the Bow, Oldman and Elk river basins, was of long duration and produced > 200 mm depth of precipitation over substantial areas in the eastern slope front ranges and foothills of the Canadian Rockies. The storage capacity of the thin rocky soils of the headwaters was filled within the first day and a half of the event, and snowmelt over frozen soils augmented the precipitation by approximately 30% in higher elevations of the front ranges and over a wide range of elevations upstream of Banff.
Several flood-generation mechanisms were active during this flood; inundation by rivers, debris flows and torrents, and groundwater flooding all occurred in different areas. Large-magnitude floods with return periods of greater than 15 years in the Bow River Basin involve all of the Central Ranges, Front Ranges and foothills and are dominated by rainfall and rain-on-snow mechanisms. Smaller peak annual flows with return periods of less than 10 years arise from snowmelt in the Central Ranges of the upper Bow River. It is inappropriate to mix flow-generation and source-area mechanisms in statistical analysis, and so the return period calculation for this event is prone to great uncertainty. While the Canadian Rockies Flood of 2013 was the largest in the past 60 years, it was not extraordinary, and was likely neither the flood of a century, nor the flood of a lifetime for those in the region despite being the most expensive natural disaster in Canadian history. Downstream communities have now prepared for similar floods but need to be prepared for larger floods in the future. Improved and unified flood forecasting systems, integrated river basin management, structural mitigation and enforced flood plain development restrictions could reduce infrastructure and property damage and even save lives in future floods of this nature. The interprovincial nature of this flood demonstrates the critical need for basin-wide, transboundary, intergovernmental delivery of coordinated river basin management and flood prediction services in Canada.
Funding
Natural Sciences and Engineering Research Council of Canada Discovery Grants and Changing Cold Regions Network (CCRN); Alberta Environment and Sustainable Resource Development; Canada Research Chairs.
Acknowledgements
The authors thank Dave Moncour and Amber Brown of the Water Survey of Canada, Calgary, for their assistance with the streamflow and water level data reported here; Bob Kochtubajda of Environment Canada for providing information on lightning; Colleen Walford of Alberta Environment and Sustainable Resource Development for making the precipitation map available; Kabir Rasouli, Xing Fang and May Guan of the Centre for Hydrology for meteorological data interpretation; and Kevin Shook of the Centre for Hydrology for contributing to return period calculations. The authors would like to thank the Changing Cold Regions Network, University of Saskatchewan Global Institute for Water Security, Canada Research Chairs Programme, Alberta Environment and Sustainable Resource Development and the Natural Sciences and Engineering Research Council of Canada (NSERC) for their support of this research.
References
- Bruce, J. P., and R. H. Clark. 1965. Introduction to hydrometeorology. Toronto: Pergamon Press, 319 pp.
- Buttle, J. M, D. M. Allen, D. Caissie, B. Davison, M. Hayashi, D. L. Peters, J. W. Pomeroy, S. Simonovic, A. St-Hilaire, and P. Whitfield. 2015. Flood processes in Canada, regional and special aspects. Canadian Water Resources Journal, this issue.
- Dawson, G. M. 1886. Preliminary report on the physical and geological features of that portion of the Rocky Mountains between latitude 49° and 50°30’. Montreal: Geological and Natural History Survey of Canada, 169 pp.
- Desloges, J. R., and J. S. Gardner. 1984. Process and discharge estimation in ephemeral channels, Canadian Rocky Mountains. Canadian Journal of Earth Sciences 21: 1050–1060.
- Fang, X., J. W. Pomeroy, C. R. Ellis, M. K. MacDonald, C. M. DeBeer, and T. Brown. 2013. Multi-variable evaluation of hydrological model predictions for a headwater basin in the Canadian Rocky Mountains. Hydrology and Earth Systems Science 17: 1635–1659. doi:10.5194/hess-17-1635-2013.
- Ford, J. D. 1924. Floods in the southern parts of Alberta and Saskatchewan during 1923. Calgary: Department of the Interior, Dominion Water Power Branch, 85 pp.
- Hoover, O. H. 1929. Floods in Southern Alberta and Saskatchewan during 1929. Calgary: Department of the Interior, Dominion Water Power and Reclamation Service, 89 pp.
- Hoover, O. H., and W. T. MacFarlane. 1932. Floods in Southern Alberta during 1932. Calgary: Department of the Interior, Dominion Water Power and Hydrometric Bureau, 73 pp.
- Kostachuk, R. A., G. M. MacDonald, and P. E. Putnam. 1986. Depositional process and alluvial fan-drainage basin morphometric relationships near Banff, Alberta, Canada. Earth Surface Processes and Landforms 11: 471–484.
- Lord, W. B. 2011. Flood hazard delineation: The one percent standard. Journal of Contemporary Water Research and Education 95: 36–39.
- Milrad, S. M., J. R. Gyakum, and E. H. Atallah. 2015. A meteorological analysis of the 2013 Alberta flood: Antecedent large-scale flow pattern and synoptic-dynamic characteristics. Monthly Weather Review 143: 2817–2841. doi:10.1175/MWR-D-14-00236.1.
- National Operational Hydrologic Remote Sensing Center. 2013. Regional snow analyses: northern Rockies. NOAA National Weather Service National Snow Analyses. www.nohrsc.noaa.gov/nsa ( accessed April, 2015).
- Osborn, G. D. 1987. Chapter 11. Geologic and hydrologic hazards in Calgary. In Geology of the Calgary area, ed. L. E. Jackson, and M. C. Wilson, 121–127. Calgary, AB: Canadian Society of Petroleum Geologists.
- Pomeroy, J. W., X. Fang, and C. Ellis. 2012. Sensitivity of snowmelt hydrology in Marmot Creek, Alberta, to forest cover disturbance. Hydrological Processes 26: 1891–1904.
- Sauder, P. M. 1914. Maximum flood discharge of the Bow River. Annual report of the Department of the Interior for the fiscal year ending March 31, 1913. Sessional Paper No. 25-1914. Ottawa, ON: King’s Printer.
- Shook, K. 2015. The 2005 flood events in the Saskatchewan River Basin: Causes, assessment and damages. Canadian Water Resources Journal. doi: 10.1080/07011784.2014.1001439.
- Shook, K., and J. W. Pomeroy. 2015. The effects of the management of Lake Diefenbaker on downstream flooding. Canadian Water Resources Journal this issue.
- Smith, C. D. 2009. The relationship between monthly precipitation and elevation in the Canadian Rocky Mountain foothills. In Proceedings of the 77th Western Snow Conference, April 2009, Canmore, AB. ed. B. McGurk, Omnipress, Madison, WI, 37–46.
- Szeto, K., W. Henson, R. E. Stewart, and G. Gascon. 2011. The catastrophic June 2002 Prairie rainstorm. Atmosphere-Ocean 49: 380–395.
- Waylen, P., and M.-K. Woo. 1981. Regionalization and prediction of annual floods in the Fraser River Catchment, British Columbia. Water Resources Bulletin 17: 655–661.
- Williams, G. 2013. Washed away – How the Great Flood of 1913, America’s most widespread natural disaster, terrorized a nation and changed it forever. New York, NY: Pegasus Books, 336.
- Wilson, M. C. 1987. Chapter 10: Geological history of the Bow River Valley in the Calgary region. In Geology of the Calgary area, ed. L. E. Jackson, and M. C. Wilson, 109–120. Calgary, AB: Canadian Society of Petroleum Geologists.