Abstract
Unmanaged riparian grazing may negatively impact rivers. The objective was to determine the influence of riparian grazing by cattle on selected channel morphology properties and riparian health of the Lower Little Bow River in southern Alberta. Three study reaches with increasing levels of riparian grazing impact were selected: (1) a fenced reach with cattle exclusion (2001–2012) followed by two years (2013–2014) of periodic grazing of the riparian pasture, (2) an unfenced and grazed reach with low cattle impact, and (3) an unfenced and grazed reach with high cattle impact. Selected channel morphology properties were measured for 3 years (2013–2015), and streambank erosion was measured over 6 years (2009–2014). The riparian health of the fenced reach was also assessed before and after 4, 8 and 11 yr of cattle exclusion, and then after 2 yr of periodic grazing. The findings generally supported the hypothesis that reduced or no riparian grazing impact would significantly (P ≤ 0.10) increase bank undercut, water depth, bankfull depth and mean-weight diameter of riverbed sediment, and decrease flow width, flow width:depth ratio, bankfull width, bankfull width:depth ratio and bank erosion. The overall riparian health score of the fenced reach was increased from 65% (healthy but with problems) prior to fencing to 85% (healthy) after 11 yr of cattle exclusion, but then decreased to 78% (healthy but with problems) after two years of periodic grazing. Exclusion fencing generally improved most channel morphology variables and riparian health. However, non-utilization of the forage resource, excess litter build-up and greater fire risk, and increased disturbance-caused plants and invasive species were serious management concerns. These issues might be alleviated by periodic grazing of the riparian pasture, but the long-term effect of this practice on channel morphology and riparian health requires further research.
Introduction
Livestock are attracted to riparian areas along rivers and streams because of the availability of water and shade, and the quantity and quality of forage (Green and Kauffman Citation1989; Fleischner Citation2002). Unmanaged or excessive grazing of riparian pastures with excessive activity of cattle along riverbanks and in the river has generally been found to have a negative influence on channel morphology (Trimble and Mendel Citation1995; Fitch and Adams Citation1998; Belsky et al. Citation1999; Sarr Citation2002; Agouridis et al. Citation2005). Channel morphology of rivers and streams is important because these physical properties influences hydrology, riparian zone, water flows, water temperatures, sedimentation, and habitat for aquatic life such as fish and aquatic insects (Belsky et al. Citation1999).
Channel morphology may be influenced by watershed area, land use and cover, soils and geology, topography, climate and human impacts (Jennings Citation2006). In addition, channel morphology may be influenced by riparian vegetation or riparian health which integrates many of these factors (Harmel et al. Citation1999; Fitch and Ambrose Citation2003). Three major morphological components of rivers that may be influenced by land-use impacts such as grazing are stream banks, stream channel and the stream substrate (Kershner et al. Citation2004). The morphology of small streams in more arid regions may also be controlled by rare floods, which is in contrast to the dominant bankfull discharge of more humid regions (Baker Citation1977; Pizzuto Citation1986).
The majority of studies have generally found greater improvements in channel morphology properties along reaches with reduced or no riparian grazing compared to conventionally grazed riparian pastures (Platts Citation1981, Citation1982; Kauffman et al. Citation1983; Platts and Nelson Citation1985; Trimble Citation1994; Knapp and Matthews Citation1996; Dobkin et al. Citation1998; Lyons et al. Citation2000; Clary and Kinney Citation2002; Scrimgeour and Kendall Citation2003; Zaimes et al. Citation2005; Magner et al. Citation2008; Raymond and Vondracek Citation2011; Samuelson and Rood Citation2011). The riparian grazing management practices may include lower stocking rates, cattle exclusion, or streambank fencing.
In contrast, some studies have documented no recovery of channel morphology properties after reduced or no riparian grazing (Kondolf Citation1993; Allen-Diaz et al. Citation1998; George et al. Citation2002; Brand et al. Citation2014). No riparian grazing responses have been attributed to insufficient time of grazing practices, varying responses depending on channel type, and a lack of comparable grazed reaches (Magilligan and McDowell Citation1997). In addition, the dominance of river hydraulic processes over grazing effects may mask riparian grazing effects (George et al. Citation2002; Sarr Citation2002; Nellesen et al. Citation2011). Some studies have also found improvements in certain river morphology properties but not others (Magilligan and McDowell Citation1997).
Platts (Citation1982) cautioned that many studies may have overestimated the possible influence of riparian grazing on river morphology and other properties. This was because most study sites were chosen in highly degraded areas (i.e. worst-case scenarios), and they had poor study designs, poor data collection, incorrect interpretations and inadequate knowledge of the exact condition of study areas prior to grazing. Belsky et al. (Citation1999) concluded that it is difficult to make generalizations about riparian grazing studies. They reported the main problems were because of faulty or inadequate study designs, inherent variability within and among watersheds, insufficient replication, ambiguities or differences in study designs, grazing inside exclosures by small mammals and invertebrates, prior history, variable time lags and influences from outside the study area.
Only a few of these studies have examined the effect of reduced or no riparian grazing on channel morphology over the longer term, > 10 yr (Platts and Nelson Citation1985; Kondolf Citation1993; Magilligan and McDowell Citation1997; Raymond and Vondracek Citation2011). In addition, most studies have generally focussed on clean, cold-water and gravel-bed rivers with recreational trout fishing. Few studies have been conducted on the channel morphology of warm-water, sand-bed and regulated rivers in agricultural areas with no recreational fishing.
Certain morphological properties such as bank undercut, water depth and bankfull depth should be greater on reaches with reduced or no riparian grazing (Platts and Nelson Citation1985; Magilligan and McDowell Citation1997; Belsky et al. Citation1999; Doll et al. Citation2003; Agouridis et al. Citation2005). In contrast, other properties such as flow width, flow width:depth, bankfull width, bankfull width:depth ratio, bank angle and bank erosion should be lower on reaches with reduced or no riparian grazing. The riverbed elevation should also be more stable on reaches with reduced riparian grazing (Belsky et al. Citation1999). More stable rivers have a more uniform cross-sectional area and bed elevation, whereas unstable rivers have more irregularities in cross-sectional area and bed elevation (Doll et al. Citation2003). Riverbeds with reduced riparian grazing should have fewer fine particles and more coarse particles (Agouridis et al. Citation2005). This suggests that the overall cumulative distribution should shift from fine to coarser particles. The particle sizes and distribution of bed materials in small streams influence channel characteristics, bedload transport, food supplies for fish, spawning conditions, cover and fish rearing habitat (Beschta and Platts Citation1986).
Riparian health evaluations conducted in the field using visual assessments integrate physical (soils and hydrology) and vegetation features, because no one factor provides a complete picture of site health or trends in health over time (Fitch and Ambrose Citation2003). The qualitative evaluations focus on vegetation because many plant features integrate the effects of soil and hydrologic factors, which form and operate in riparian areas, and because plant features are more visible than soil or hydrologic features. Although the individual effects of riparian grazing on vegetation, soil and hydrologic properties (Beschta and Platts Citation1986; Trimble and Mendel Citation1995; Belsky et al. Citation1999; Agouridis et al. Citation2005; Samuelson and Rood Citation2011) have been quantified, few studies have integrated these three properties or conducted detailed riparian health assessments over long-term (> 10 yr) periods (Miller et al. Citation2010, 2011). In addition, no other studies were found that examine the influence of riparian grazing on both river morphology and overall riparian health. In a study in southern Alberta, Samuelson and Rood (Citation2011) reported that riparian zones in upper mountain environments (higher elevations) were particularly vulnerable to livestock grazing compared to fescue prairie at lower elevations.
The objective of this study was to determine the influence of riparian grazing on selected channel morphology properties and riparian health. The overall hypothesis was that reduced or no riparian grazing should result in a positive response (i.e. improvement) of certain river morphology properties and riparian health.
Materials and methods
Study site description
The three study reaches (50°0’11.59”N, 112°36’47.39”W) were on the Lower Little Bow (LLB) River located within the LLB watershed and Mixedgrass natural subregion (Adams et al. Citation2005) in southern Alberta, Canada. Detailed site descriptions and locations, and a map of the watershed have been previously reported (Miller et al. Citation2014). The dominant vegetation is Pascopyrum smithii (Rydb.) Á. Löve (western wheat grass), Elymus lanceolatus (Scribn. & J.G. Sm.) Gould (northern wheat grass) and Hesperostipa comata (Trin. & Rupr.) Barkworth Stipa comata (needle-and-thread grass). The soils are primarily Dark Brown Chernozems (Typic Haplustoll). The long-term (1961–2010) mean annual precipitation in the area (Iron Springs, Alberta) is 383 mm. Monthly and total precipitation from May to October for the study period (2009–2015) were taken from the station at Iron Springs (Alberta Agriculture and Forestry Citation2017), about 9 km from the study area, and compared to the long-term mean or LTM (1973–2015). Monthly river discharge or flow was determined from historical data for the station at the mouth (Station 05AC023) of the LLB River (Environment Canada Citation2016), which is about 23 km downstream of the study reach. This station is the outlet for the entire LLB watershed (55,664 ha) and discharges into the Oldman River, and its immediate sub-basin upstream is 10,800 ha (Little et al. Citation2003). Although flows at the mouth of LLB River are greater than upstream at the three study reaches, the peak discharges generally occur at the same time (Little Citation2001).
Characteristics of Lower Little Bow River
The LLB River is a sand-bed, regulated, warm-water and turbid river whose flows are mainly controlled upstream at the Travers Reservoir dam. Although some small sections of the river in the study area are incised due to steep vertical bluffs, the majority of the river is non-incised. The width of the river ranges from 8 to 9 m and the depth from 0.5 to 1.0 m (Miller et al. Citation2011). The study reaches are approximately 30 km downstream of the dam. The Travers Reservoir was built on the LLB River in 1954, to store water for the Bow River Irrigation District and to facilitate the flow of water from McGregor Lake to Little Bow Lake Reservoir (Mitchell and Prepas Citation1990). The dam releases about 0.57 m3 s−1 during the winter and 0.85 m3 s−1 during the summer (Little Citation2001). Release rates and flows are more variable in the summer, depending on irrigation demand, downstream inputs from irrigation and quantity of supply waters. However, flows of up to 13 m3 s−1 have occurred in years with extreme rainfall (Miller et al. Citation2010). Irrigation return flow channels may contribute up to 0.6 m3 s−1 to the LLB River during the irrigation period between May and October (Little Citation2001).
The channel of the LLB River is moderately meandering within the coulee bottom, the channel pattern is single-threaded, and instream habitat consists mainly of runs (Scott Citation2012). Turbidity and total suspended solids most frequently exceed the surface water quality guidelines in the LLB River (Miller et al. Citation2010). Fish families in the reaches are mainly Cyprinidae (minnows) and Catostomidae (suckers), with also some Esocidae (pike) and Percopsidae (trout-perch) families (J.L. Scott Citation2012, personal communication). There is generally no recreational fishing in the LLB River. The main benthic invertebrates (Insecta) found on the riverbed of the LLB River are Trichoptera (caddisflies), Ephemoptera (mayflies) and Odanata (dragonflies, damselflies), but densities are very low (≤ 21 individuals m−2) in this turbid and sand-bed river (unpublished data).
Riparian grazing treatments
This study focused on river morphology along three reaches with different riparian grazing treatments, as reported previously in more detail (Miller et al. Citation2014). The three riparian grazing treatments (reaches) were: (1) an 800-m fenced (since 2001) reach between LB4-14 and LBW1 (Reach 1) with cattle-exclusion for 11 yr (2001–2012) and periodic grazing of riparian pasture for 2 yr (2013–2015), which is referred to as the fenced treatment; (2) an unfenced grazed reach (1156 m long) between LBW4 and LBW2 (Reach 3) with low cattle impact on the riparian zone (unfenced-low impact, UNF-L); and (3) an unfenced reach (919 m long) between LB4-14 and LBW4 (Reach 2) with high cattle impact on the riparian zone (unfenced-high impact, UNF-H). The fenced, UNF-L and UNF-H reaches have been previously referred to as ungrazed, low-impact grazed and high-impact grazed treatments, respectively (Miller et al. Citation2014).
Periodic grazing of the fenced riparian pasture was conducted for 2 weeks in July or August at a stocking rate of 3.8 animal unit months (AUM) ha−1. The stocking rate for upland and riparian pastures (grazed from June to August) adjacent to the UNF-H reach ranged from 0.3 to 1.5 AUM ha−1, and it was 6.0 AUM ha−1 (grazed for 2 months in spring and fall) for the UNF-L reach. As discussed in detail by Miller et al. (Citation2014), the stocking rates in riparian and upland pastures adjacent to these grazed reaches did not reflect the impact of cattle grazing in the riparian pastures. Reasons for this include that there were more cattle access sites on the UNF-H reach (12 access sites) than the UNF-L reach (six access sites), and the area of the riparian pasture was greater for UNF-H (4.7 ha) than UNF-L (2.2 ha). The grazing season was also longer for the UNF-H reach (3 months) than for UNF-L (2 months), and the grazing season for UNF-H was during a hot summer compared to a cooler spring and fall for UNF-L. The upland pastures were also much closer to the river along the UNF-H reach (20 m) compared to the UNF-L reach (65–398 m), and off-stream watering was closer to the river along the UNF-H reach (< 20 m) compared to the UNF-L reach (300 m). These factors were more important in determining the impact by cattle on the riparian pastures than was the overall stocking rate on riparian and upland pastures.
The riparian grazing treatments on the three reaches were not replicated and measurements and sampling were conducted at different locations within each reach (i.e. pseudo-replication), which may make interpretations of treatment differences less confident (Hurlbert Citation1984). Despite this, unreplicated exclosures and other grazing treatments on reaches often provide the only option for the study of grazing impacts, and these studies provide most of the information on effects of riparian grazing on stream and riparian ecosystems (Knapp and Matthews Citation1996). Similar experimental designs using non-replicated, riparian-grazing treatments on reaches have been previously used (Kondolf Citation1993; Trimble Citation1994; Knapp and Matthews Citation1996; Magilligan and McDowell Citation1997; Zaimes et al. Citation2004; Miller et al. Citation2014).
Scott (Citation2012) reported that riparian vegetation impact by livestock in 2009 (after 8 yr of fencing) was greatest for the UNF-H reach, followed by the UNF-L reach, and lowest for the fenced reach. J.L. Scott (Citation2012, personal communication) also found the same trend for the fenced and UNF-H reaches after 11 yr of fencing in 2012. The riparian habitat characteristics measured included major species, vegetation type, presence of wetlands, signs of wildlife use or browse, and cattle impact indicators (hoof-prints, urine and manure). Soil texture (Soil Classification Working Group Citation1998) of the surface (0–15 cm) soil from the streambanks ranged from loam to sandy loam for the fenced reach, loamy to loamy sand for the UNF-H reach, and loam to silty clay loam for the UNF-L reach. Land use adjacent to the fenced and unfenced reaches is native rangeland, and adjacent to the low-impact grazed reach it is irrigated tame pasture or irrigated, annual cropland.
Channel morphology measurements
Selected channel morphology properties were measured at 10 locations within each of the three reaches over 3 years (2013–2015) using methods outlined by Gordon et al. (Citation2004). The sites were selected on a straight stretch of river (i.e. runs) with banks of relatively equal height on both sides, and were all located within non-incised reaches where the river could access the floodplain. The measurements and sampling were conducted on 3–9 July in 2013, 9–28 June in 2014, and 10–15 June in 2015. Vertical metal (rebar) stakes were installed on either side of the riverbank at each of the 10 locations to allow a measuring tape to be strung horizontally across the river at the time of measurement (reference elevation). The elevations of the riverbed were measured at nine locations across the river at 1-m intervals from the south bank using a survey staff, and the vertical distance from the reference elevation to bottom of the riverbed was recorded. For the river profile measurements, statistical analysis was conducted on the absolute difference in mean relative elevation of the riverbed at nine distances (0 to 8 m) for the yearly comparisons of 2013 vs 2014 and 2014 vs 2015. Significantly lower mean differences in bed elevation between years were assumed to indicate more stable riverbeds.
Water depth was measured at three locations (near left bank, center of river, near right bank) across the riverbed using a survey staff, as the vertical distance from water level to riverbed. Flow (wetted) width was measured with a measuring tape along the top of the water column. Bankfull depth (height) for both banks was measured with a survey staff from the top of the bankfull stage to the riverbed. The bankfull height is generally defined as the height reached by a river on average every 1 to 2 years, and is the discharge that just fills the river to its banks or bankfull discharge (Gordon et al. Citation2004). Bankfull height on non-incised sections of reaches was visually estimated as the point (crest or crown of vertical bank) at which river flow exits the channel and spills out onto the floodplain. Bankfull width was measured with a measuring tape as the horizontal distance from the bankfull stage on either side of the river. The bank angle for both banks was measured by laying the survey staff along the bank and then measuring the angle using a portable slope meter. The bank angle was the arc between the horizontal plane on the river side of the slope and the slope angle, which is opposite to the Bank Erodibility Hazard Index (BEHI) method (Rosgen Citation1996). Bank undercut or undercut for both banks was measured as the horizontal distance of maximum undercut to the vertical plane of bankfull depth.
Streambank erosion rates were measured using the erosion pin method (Gordon et al. Citation2004) over 6 years (2009–2014). Metal erosion pins (60 cm long × 0.635 mm in diameter) were installed at seven locations along the fenced reach, five locations along the UNF-H reach and six locations along the UNF-L reach. Ten erosion pins were installed at each location. Therefore, there were 70 pins installed on the fenced reach, 50 pins on the UNF-H reach and 60 pins on the UNF-L reach. Three pin readings were conducted in each of 2009 (7July, 19 August, 15 September), 2010 (27 April, 24 September, 4 November) and 2011 (24 June, 5 August, 19 October), four readings in 2012 (9 May, 10 July, 11 October, 19 October), three readings in 2013 (2 May, 2 July, 22 October) and two readings in 2014 (7 May, 7 July).
All pins were installed at least 10 cm above the water level of the river. If the riverbank height was low (laterally exposed face), the 10 pins were installed laterally along the bank for a maximum distance of 3 m. If the riverbank was high (vertically exposed face or cutbank), the 10 pins were installed along the top, middle and lower face of the bank. The pins were inserted horizontally (no pre-drilling) into the vertical face of the banks until only about 5 cm of the pin was exposed. The horizontal distance from the bank to the end of each pin was then recorded (initial reading) and then subsequent readings were conducted throughout the year, depending on river flows and safe wading conditions. If erosion was high enough to fully expose pins and they were ejected from the riverbank, erosion was assumed to be the maximum distance (55 cm) and the pins were reset to the standard 5-cm exposure distance. Erosion pin readings were converted to annual erosion rates in mm yr−1, and erosion rates were reported as negative values (loss) while deposition or accretion was reported as positive values (gains).
Riverbed substrate samples (180–200 g) were taken by hand from three sites (near left bank, center of channel, near right bank) at each of 10 locations along each of the three reaches over 3 years (2013–2015) on the same dates that other morphological properties were measured in the field. The samples were oven-dried and the particle size was measured for 10 particle size classes using the dry sieving method (Gordon et al. Citation2004). The 10 particle size classes were > 8 mm (medium-coarse gravel), 4–8 mm (fine gravel), 2–4 mm (very fine gravel), 1–2 mm (very coarse sand), 0.50–1.0 mm (coarse sand), 0.25–0.50 mm (medium sand), 0.125–0.25 mm (fine sand), 0.063–0.125 mm (very fine sand), 0.032–0.063 mm (coarse silt) and < 0.032 mm (fine to medium silt). The sieves were stacked from coarsest to finest from top to bottom, the sediment sample was placed on the top sieve, and then the nest of sieves was shaken on an Eberbach® reciprocal shaker for 10 min. The mean-weight diameter (MWD) was calculated using the method outlined by Larney (Citation2008).
Riparian health of fenced reach
Riparian health assessments of the fenced reach of the LLB River were conducted prior to fencing being installed in 2001, after 4 (2005), 8 (2009) and 11 (2012) yr of total cattle exclusion, and then after 2 yr of periodic grazing (2015). It should be emphasized that these riparian health assessments with percentage scores and health categories are qualitative, categorical, subjective and based on visual observations in the field, and they were not designed to be quantitative evaluations for research. Despite being qualitative, riparian health assessments allow a better understanding of the overall health of the riparian area by integrating visual assessments of vegetation, soil and hydrology factors. Another goal was to determine whether riparian health might help explain possible changes in certain channel morphology properties that were quantitatively measured. Therefore, the qualitative health assessments were used to better understand overall riparian health and supplement the quantitative measurements of channel morphology. Riparian health assessments have also been previously used in this context along the same reach (Miller et al. Citation2010, 2011).
The riparian health assessments were conducted by the Alberta Riparian Habitat Management Society (Cows and Fish) on 11 October 2001, and then between 15 and 19 July in 2005, 2009, 2012 and 2015. The riparian health of the fenced reach after 4 yr of fencing and cattle exclusion was previously reported (Miller et al. Citation2011). Riparian health assessments were also conducted on the UNF-L reach prior to off-stream watering installation in 2005 and then after two years of off-stream watering in 2007 (Miller et al. Citation2011). Riparian health could not be assessed for the UNF-H reach because permission from the landowner could not be obtained.
The assessments were conducted by the Alberta Riparian Habitat Management Society (Cows and Fish) using the field workbook for riparian health assessment for streams and small rivers (Fitch et al. Citation2001, Citation2009). Details of the assessment for this same reach have been reported (Miller et al. Citation2010). Six vegetation criteria (questions) in 2001 and 2005 and seven vegetation criteria thereafter, and five soil and hydrology criteria, were visually assessed by riparian health specialists. Riparian health evaluation integrates both vegetation and physical (soils and hydrology) criteria (Fitch and Ambrose Citation2003). Many factors deal with the element of ‘coverage’ or how much of the reach area is covered, influenced or affected by vegetation or structural impacts.
Riparian health is classified into three categories: healthy (H, total score between 80 and 100%), healthy but with problems (HP, total score between 60 and 79%), and unhealthy (UH, total score < 60%) ratings (Fitch and Ambrose Citation2003). Healthy riparian areas have little or no impairment to riparian functions; they are resilient and stable and provide a long list of benefits and values. Riparian areas that are healthy but with problems have some impairment to riparian functions due to human or natural causes. Many riparian functions are still being performed, but some signs of stress are apparent. Unhealthy riparian areas have impairment to many riparian functions due to human or natural causes, and most riparian functions are severely impaired or have been lost.
Hypotheses and statistical analyses
The hypotheses for influence of reduced or no riparian grazing on the river morphology properties and riparian health are shown in Table . A mixed model (analysis of variance, ANOVA) analysis was used to analyze the channel morphology variables by year using SAS (SAS Institute Inc. Citation2002). For the mixed model analysis, the variables were first evaluated to determine whether transformations were required to make the residuals follow a normal distribution and have homogeneous variances. If required, a logarithmic (base 10) transformation was conducted.
Table 1. Hypotheses for influence of reduced or no riparian grazing impact on channel morphology properties.
For the mixed model analysis conducted by year, the class variables were riparian grazing treatment (reach) and location (i.e. locations within each reach), the fixed effect was riparian grazing management (fenced, UNF-L and UNF-H reaches), and the random effect was location. For yearly changes in riverbed elevation, the distances across the riverbed transect were used as a random effect. A least significant difference (LSD) means test was used to compare means at P ≤ 0.10, except when there were more than five means, in which case a Tukey test was used. A non-parametric Wilcoxon rank-sum test (NPAR1WAY procedure) was conducted to analyze the streambank erosion data. Median bank erosion rates were compared for fenced vs UNF-L, fenced vs UNF-H and UNF-L vs UNF-H reaches at P ≤ 0.10. The probability level of 0.10 was used for all statistical analyses, as recommended for landscape studies (Pennock Citation2004).
Results
Precipitation and river discharge
Total precipitation from May to October was higher (115–133% of LTM) in 2009, 2010 and 2014, normal in 2011, 2012 and 2013 (101–106%), and lower than normal in 2015 (70%) (Figure a). The maximum monthly precipitation occurred in May 2010 and 2011, June 2012, 2013 and 2014, July 2009, and September 2015. Monthly discharge was generally greater than normal for all 6 years (Figure b). The maximum monthly flows occurred in June 2011, 2013, and 2014, July 2010 and 2015, and August 2009 and 2012. The minimum monthly flows occurred in October of all 6 years.
Selected channel morphology properties
Mean bank angle was similar (P > 0.10) among the three grazing treatments in all 3 years from 2013 to 2015 (Table ). Bank undercut was greater (P < 0.10) for the fenced than the UNF-H reach in all years and greater for the fenced than the UNF-L reach in 2013 (Table ). Bank angles ranged from 90 to 109°. Undercut banks have angles of 0 to 89°, and laid-back banks have angles of 90 to 180° (Knapp and Matthews Citation1996). Therefore, all the bank angles in this study were classified as laid-back.
Table 2. Influence of riparian grazing management on selected river morphology properties during a 3-yr study (2013–2015). Measurements conducted on the fenced reach in 2013 and 2014 were done after 12 and 13 yr of total cattle exclusion, respectively. Measurements in 2015 were done after 11 yr of cattle exclusion followed by 2 yr of periodic grazing.
Mean water depth in 2013 was significantly greater for the fenced than two unfenced reaches, while in 2014 it was significantly greater for the UNF-L than the UNF-H reach. Water depth was not determined in 2015. Mean flow width in 2013 and 2014 was significantly lower for the UNF-L than the UNF-H reach (Table ). In 2015 it was significantly lower for the fenced and UNF-L reaches compared to UNF-H reach.
Mean bankfull depth in 2013 was significantly greater for UNF-L than UNF-H (Table ), while in 2014 it was greater for the fenced and UNF-L reaches compared to the UNF-H reach. Bankfull depth was also considerably greater in 2013 than 2014, and it was not determined in 2015. Mean bankfull width was similar for all three reaches in 2013 (Table ). Bankfull width in 2014 was significantly lower for the UNF-L than the UNF-H reach, while in 2015 it was significantly lower for fenced and UNF-L reaches compared to the UNF-H reach.
The ratio of flow width to flow depth (FW:FD) ranged from 11.2 to 21.3 (Table ). The flow width to water depth (FW:WD) ratio was significantly lower for the fenced reach than the UNF-H reach in 2013, and was significantly lower for UNF-L than UNF-H in 2014. The ratio of bankfull width to bankfull depth (BW:BD), or bankfull ratio, ranged from 5.6 to 12.1 (Table ). The bankfull ratio was significantly lower for UNF-L than UNF-H in 2013, and significantly lower for fenced and UNF-L reaches compared to the UNF-H reach in 2014.
The ratio of water depth to bankfull width (WD:BW) ranged from 0.35 to 0.74 (Table ). In 2013, this ratio was significantly greater for the fenced than the two unfenced reaches, and in 2014 it was significantly lower for the fenced reach than for the UNF-H reach. The flow width to bankfull width ratio (FW:BW) ranged from 0.92 to 0.95 (Table ). This ratio in 2013 and 2015 was significantly greater for the fenced than the UNF-L reach, and it was similar for all three reaches in 2014.
Riverbed elevation and stability
The changes in riverbed elevation for the nine sampling distances were evaluated for 2013 vs 2014 and for 2014 vs 2015 (Figure ). The mean change in elevation for 2013 vs 2014 was lowest (i.e. indicating a more uniform or stable riverbed) for the fenced reach (6.0 ± 1.9 cm), followed by UNF-H (9.3 ± 2.3 cm), and was highest for UNF-L(13.4 ± 2.4 cm). Significant (P ≤ 0.10) differences among means occurred between the fenced and UNF-L reaches, but not fenced vs UNF-H, or UNF-H vs UNF-L reaches. The mean change in elevation for 2014 vs 2015 was lowest (more uniform or stable riverbed) for the UNF-H reach (10.1 ± 1.9 cm), followed by UNF-L (11.4 ± 2.5 cm), and was highest for the fenced reach (16.2 ± 4.8 cm). However, there were no significant differences in mean elevation differences among the three reaches for 2014 vs 2015.
Bank erosion
Median annual rates of bank erosion were significantly lower for the fenced and UNF-L reaches (−2.6 to −3.4 mm yr−1) compared to the UNF-H reach (−11.3 mm yr−1; Table ). Maximum erosion was −445 mm and maximum deposition was 173 mm. Negative and positive erosion rates indicate erosion and deposition, respectively. Visual observations in the field indicated that bank erosion was considerably greater with depth along the vertical face of the riverbank, and the lowest bank erosion often occurred in the top of the bank where plant root densities were greatest and where the soil was above the water level in the river. Slumping or sloughing of bank material was visually observed in the field, and sometimes occurred in association with seepage at saline seeps.
Table 3. Influence of riparian grazing treatment on streambank erosion rates for the Lower Little Bow River over 6 years (2009–2014).
Particle size analysis of riverbed
The cumulative distribution of particle sizes in 2013 and 2014 showed that the fenced reach had a greater percentage of larger particles (gravel or > 8 mm to medium sand or 0.25–0.50 mm) than the UNF-L and UNF-H reaches (data not shown). In 2015, the fenced and UNF-H reaches had a greater percentage of these same larger particles compared to UNF-L. The MWD was significantly greater for the fenced reach than the two unfenced reaches, by 60 to 212%, in 2013 and 2014, and it was significantly greater for the fenced and UNF-H reaches compared to the UNF-L reach, by 127 to 133%, in 2015 (Figure ).
Figure 3. Influence of grazing on mean-weight diameter (MWD) of riverbed sediment in 2013, 2014 and 2015. The three grazing treatments are fenced reach, unfenced reach with low-cattle impact on riparian pasture (UNF-L), and unfenced reach with high-cattle impact on riparian pasture. Vertical bars are means plus 1 standard error. Means within each year followed by different lower case letter are significantly (P ≤ 0.10) different.
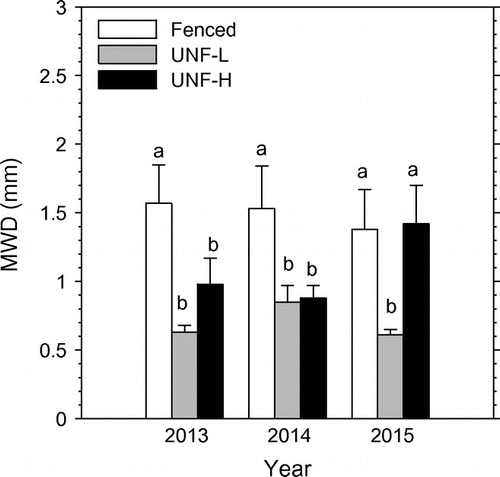
Riparian health of fenced reach
The overall riparian health score of the fenced reach was 65% (HP, healthy but with problems) in 2001 just prior to installation of exclusion fencing (Table ). The overall health score increased to 81% (H, healthy) in 2005 after 4 years of cattle exclusion, decreased to 74% (HP) in 2009 after 8 years of cattle exclusion, and then increased to 85% (H) after 11 yr of cattle exclusion. In 2015, after 12 yr of cattle exclusion followed by 2 yr of periodic grazing (initiated in 2013), the riparian health decreased to 78% (HP). Caution is advised in interpreting the changes in riparian health scores over time because the percentage scores are qualitative and the health classifications are categorical.
Table 4. Riparian health inventory of the fenced reach of the Lower Little Bow River prior to fencing being installed in 2001 and then 4, 8, 11 and 14 years after fencing was installed. The assessments in 2005, 2009 and 2012 were conducted after total cattle exclusion from riparian pasture. The assessment in 2015 was conducted after 11 yr (2001–2012) of total cattle exclusion followed by 2 yr (2013–2014) of periodic grazing of the riparian pasture.
The health score for vegetative cover (Criterion #1) increased after 14 yr (Table ). Vegetative cover prior to fencing in 2001 was dominated by Hordeum jubatum (foxtail barley). Vegetation after 14 yr was dominated by Agropyron dasystachyum (northern wheat grass), Distichlis stricta (salt grass), Hordeum jubatum, Agropyron pectiniforme (crested wheat grass) Phalaris arundinacea (reed canary grass), and Scirpus pungens (three-square rush). Although reed canary grass is a native species, aggressive hybrids may form extremely dense stands in the riparian area, including right up to the water’s edge, and exclude colonization by preferred trees and shrubs such as willows and cottonwoods (Rood and Kalischuk Citation2008).
The score for invasive plants (Criterion #2) decreased over time, due to increased cover of Cirsium arvense (Canada thistle), Sonchus arvensis (perennial sow-thistle), Convolvulus arvensis (field bindweed) and Centaurea repens (Russian knapweed). The score for disturbance-caused undesirable plants (Criterion #3) increased after 14 yr, and the major undesirable species were Hordeum jubatum, Poa pratensis (Kentucky blue grass) and Bromus inermis (smooth brome grass).
The score for preferred trees and shrubs (Criterion #4) and utilization of these woody plants (Criterion #5) varied over the 14 yr; and coverage was generally ≤ 3% for individual species. Rosa woodsii (common wild rose) and Symphoricarpos occidentalis (buckbrush/snowberry) were found in all 5 years except 2001. Atriplex nuttallii (Nuttall’s atriplex) was found in 2005, 2012 and 2015. Salix pseudomonticola (false mountain willow) was found only in 2015.
The health scores for live woody vegetation by non-browsing (Criterion #6) activity (e.g. human clearing or cutting, beavers, etc.) and amount of decadent and dead woody material (Criterion #7) remained constant over the 14 yr. The health score of streambank root mass protection (Criterion #8) increased slightly over the 14 yr (Table ). In contrast, the score for human-caused (including livestock) bare ground (Criterion #9) increased dramatically after only 4 yr of fencing. The health scores for streambank structurally altered (Criterion #10) and stream channel incisement (Criterion #12) remained constant over time. The health score for human (including livestock) physical alteration to the streambank (Criterion #11) decreased slightly after 8 yr of fencing.
The increase in riparian health after 11 yr of cattle exclusion was due to improvements in vegetative cover of floodplain and streambanks (Criterion #1), an increase in streambank root mass protection (Criterion #8), and a decrease in human-caused bare ground (Criterion #9). The specific criterion that had the most drastic decrease in riparian health was for invasive plant species (Criterion #2). For the related channel morphology criteria, there was no change in riparian health of the streambank being structurally altered (Criterion #10) and stream channel incisement (Criterion #12). The decrease in riparian health after 2 yr of periodic grazing was due to a decline in preferred tree and shrub establishment and regeneration (Criterion #4), and an increase in utilization of preferred trees and shrubs (Criterion #5).
Discussion
Similar mean bank angles or slopes for the fenced (97–101°), UNF-L (90–105°) and UNF-H (95–109°) reaches did not support the hypothesis of lower bank slopes with reduced or no grazing. Increased grazing impact is expected to decrease streambank slopes (i.e. more laid back) due to streambank sloughing and trampling caused by livestock (Belsky et al. Citation1999). Most studies have reported lower bank slopes with reduced or no grazing (Patts 1981; Platts and Nelson Citation1985; Knapp and Matthews Citation1996; Clary and Kinney Citation2002; Kershner et al. Citation2004; Herbst et al. Citation2012). This was not evident in the current study, possibly because of the sandy soils in the study area, which tend to already have more sloping sides (Gordon et al. Citation2004). The lack of response to cattle impacts may also have been due to insufficient time (12–14 yr) for the grazing treatments, or streambanks that were more resistant to bank deformation by cattle because of lower soil moisture or more cover of riparian vegetation on the banks.
The texture of surface soil from the streambanks ranged from loam to sandy loam for the fenced reach, loamy to loamy sand for the UNF-H reach, and loam to silty clay loam for the UNF-L reach. Sandy soils are generally less responsive to soil compaction compared to finer textured soils (Brady Citation1974). Although surface soil compaction of the streambank was generally significantly lower with reduced or no grazing (Miller et al. Citation2014), the compaction and trampling by cattle may not have been sufficient to cause bank deterioration and more laid-back slopes. Higher bank heights and cutbanks along these three reaches may also have discouraged cattle from coming near the banks. Riparian vegetation cover was also generally high on the streambanks of all three reaches, and may have been a major factor contributing to no positive response of bank angle with reduced or no grazing. Bank angles may also have been controlled mainly by natural channel processes rather than by riparian grazing. George et al. (Citation2002) reported that streamflow was the largest potential confounder in testing for the effect of grazing on river morphology.
Significantly greater bank undercut (overhang) for the fenced than unfenced treatments in 2013, and significantly greater bank undercut for fenced than UNF-H reaches in 2014 and 2015, support the hypothesis of greater bank undercut with reduced or no grazing impact. Increased grazing reduces the quality and quantity of undercuts caused by streambank breakdown by livestock and loss of stabilizing vegetation, which results in a degraded habitat for fish (Belksy et al. Citation1999). Most studies have reported a positive response of bank undercuts with reduced or no grazing (Gunderson Citation1968; Platts Citation1981; Platts and Nelson Citation1985; Kershner et al. Citation2004), while others have reported inconsistent responses (Knapp and Matthews Citation1996). The higher-than-normal river flows in these 3 years may have also contributed to undercutting of the banks.
Although bank undercut followed the order fenced > UNF-L > UNF-H, fish densities (seining, electrofishing, minnow traps) in this same river were inconsistent after 8 yr (2009) and 11 yr (2012) of streambank fencing (CitationScott 2012; J.L. Scott 2012, personal communication). Fish densities determined by seining (netting), electrofishing and minnow traps followed the order fenced > UNF-H > UNF-L after 8 yr of fencing (Scott Citation2012). However, fish densities after 11 yr followed the order UNF-H > fenced from seining, and densities were similar for the two reaches from electrofishing (J.L. Scott Citation2012, personal communication). Therefore, greater bank undercuts with no grazing may be associated with greater fish densities in certain years, but not in others.
Our range in bank undercuts (5–23 cm) of the sand-bed LLB River were considerably greater than values reported for reaches (2–7 cm) in a gravel-rubble bed creek in Utah (Platts and Nelson Citation1985), and for reaches (1–8 cm) for gravel-bed rivers in California (Knapp and Matthews Citation1996). Greater bank undercuts for the LLB River than these other rivers were likely related to the sand-bed substrate of LLB River. Sand-bed rivers are more erodible than gravel- or gravel-rubble rivers, and sand-bed rivers are in near-continual bed motion, and sediment concentrations can increase exponentially with discharge (ASCE Task Committee Citation1992). This continual bed motion with suspended sand in the water column is very abrasive and likely results in greater undercutting (erosion) of the banks.
Significantly lower FW:WD ratios for the fenced reach than the UNF-H reach in 2013, and for the UNF-L reach than the UNF-H reach in 2014, support the hypothesis that reduced or no grazing impact should decrease this ratio. Reduced or no grazing impact shifts the shape of channels from wide and shallow to narrower and deep (Platts Citation1981; Knapp and Matthews Citation1996; Magilligan and McDowell Citation1997; Belsky et al. Citation1999; Agouridis et al. Citation2005). In addition, bank destabilization and sedimentation from agriculture often increase the FW:WD ratio (Belsky et al. Citation1999). Channel widening of small streams may be responding to increased flows, increased sediment availability, other factors such as loss of streamside vegetation, or a combination of all three (Beschta and Platts Citation1986). Most researchers have reported lower flow (wetted) width:water depth ratios with reduced or no grazing (Platts Citation1981; Platts and Nelson Citation1985; Stuber Citation1985; Knapp and Matthews Citation1996; Lyons et al. Citation2000), while some have found no or inconsistent responses (Raymond and Vondracek Citation2011).
Water depth is important for providing pools, cover and instream movement areas for fish; and lower FW:WD ratios are associated with better fish habitat (Stuber Citation1985). An optimum ratio of ≤ 7 has been suggested for small (< 10 m width) streams for adequate habitat for freshwater fish such as trout (Simonson et al. Citation1994). The ratios (11.2–21.3) in the three reaches of this study suggest that this river habitat would be unsuitable for other fish of similar size, because of insufficient depth of water for protective cover. The flow widths in this study ranged from 8 to 10 m, so minimum water depths of 1.1 to 1.4 m would be required for adequate fish habitat using the optimum ratio of 7. However, measured water depths in this study ranged from 0.47 to 0.79 m, which suggests inadequate depth of water for suitable fish habitat. However, the turbid water in the LLB River would likely increase protective cover despite the shallow depth.
Significantly lower BW:BD for UNF-L than UNF-H reaches in 2013, and for the fenced and UNF-L reaches compared to the UNF-H reach in 2014, supports the hypothesis that reduced or no grazing impact should decrease this bankfull ratio. Similar to the wetted ratio, reduced grazing impact shifts the shape of bankfull channels from wide and shallow to narrower and deep (Platts Citation1981; Knapp and Matthews Citation1996; Magilligan and McDowell Citation1997). Lower bankfull ratios with reduced or no grazing have been reported (Knapp and Matthews Citation1996; Magilligan and McDowell Citation1997; Lyons et al. Citation2000; Kershner et al. Citation2004; Herbst et al. Citation2012), but others found no response (George et al. Citation2002; Magner et al. Citation2008).
The ratios of water depth:bankfull depth were greater in 2014 (0.62–0.74) than 2013 (0.33–0.49), and were consistent with greater precipitation and flow discharges in 2014 than 2013. The high ratios of FW:BW suggest that flows were very close (92–95%) to bankfull stage. Hillman et al. (Citation2016) reported that increased flows on the upper LLB River resulted in greater mean channel width, but sinuosity was unaffected.
Mean bankfull depths were 35, 43 and 55 cm lower in 2014 than 2013 for the fenced, UNF-L and UNF-H reaches, respectively. Possible explanations for this unexpected finding could be uncertainties and subjectivity in determining bankfull depth in the field, erosion of the crests of the streambanks, deposition of sediment on the riverbed, and other possible explanations the authors are not aware of. The estimation of bankfull depth in the field is often a difficult and subjective measurement, and depends on the experience of the observer (Johnson and Heil Citation1996). The latter authors reported that bankfull depth may not be well defined where bank tops are not at the same elevation, where stream reaches are unstable, where an obvious break between streambank and floodplain doesn’t exist, and where complexities such as benches and terraces exist. Since some of these conditions existed on the LLB River, and the observer did not have a lot of experience measuring bankfull depth, these factors may have contributed to uncertainty and variability in measuring bankfull depth,
There was a peak (maximum) daily flow event of 25 to 26 m3 s−1 in early June of 2014 prior to river morphology measurements being conducted later that month. Peak flow events of ≥ 23 m3 s−1 for the LLB River are generally associated with this river exceeding bankfull discharge and overflow into the floodplain. Therefore, some erosion of the crests of the streambanks and deposition of sediment on the riverbed may have occurred because of this high-flow event in June of 2014 prior to bankfull depth being measured. However, the crests of the streambanks were generally vegetated, which would have minimized erosion of the crest during flooding. In addition, the riverbed elevation adjacent to the streambanks was relatively stable between 2013 and 2014. Therefore, it is unlikely that erosion of bank crests and sediment deposition alone could have accounted for the drastic decline in bankfull depth from 2013 to 2014.
River morphology in dryland regions is generally controlled by rare but extreme flows or floods (Baker Citation1977; Pizzuto Citation1986; Vyverberg Citation2010). Flows, peak discharges, stream power and sediment-carrying capacity are generally reduced in regulated rivers by increased reservoir storage and evaporation (Brandt Citation2000). However, peak flows and flooding of the banks still occur in the LLB River because of periodic releases from the dam, extreme rainfall events and sudden increases in irrigation return flows (Little Citation2001; Little et al. Citation2003).
Significantly lower mean differences in riverbed elevations between 2013 vs 2014 for the fenced than the UNF-L reach support the hypothesis of a more uniform or stable riverbed with reduced grazing. However, there was no significant grazing effect on riverbed stability for the 2014 vs 2015 comparison. The drastic changes in river discharges due to upstream dam releases, extreme rainfall and irrigation return flow may have contributed to high flow velocities in the river that prevented possible grazing effects on riverbed stability for the 2014 vs 2015 comparison. The annual change of 6 to 16 cm for the riverbed elevation was consistent with the unstable and near-continual motion of sand-bed rivers (ASCE Task Committee Citation1992).
Significantly lower median annual rates of bank erosion for the fenced and UNF-L reaches (−8.2 to −8.5 mm yr−1) compared to the UNF-H reach (−11.3 mm yr−1) support the hypothesis of lower bank erosion with reduced or no grazing. The risk of bank erosion (BEHI determined in the field by visual observations) is generally greater with an increasing ratio of bank height:bankfull height, lower ratios of rooting depth:bank height, lower root density, steeper bank angles with greater undercuts, and lower surface cover of vegetation (Rosgen Citation1996). Bank erosion is also enhanced with greater river discharge or flow (Goldstein et al. Citation2007). The trend was consistent with visual observations of greater coverage and growth of riparian vegetation on the fenced and UNF-L reaches compared to the UNF-H reach (Scott Citation2012). Riparian vegetation helps stabilize channel banks of small streams and contributes in various ways to fish productivity (Beschta and Platts Citation1986). Streambank stability is reduced with greater grazing impact because of fewer plant roots to anchor the soil, less plant cover to protect the soil surface from disturbance, and the shear-force of trampling hooves (Belksy et al. 1999). Some erosion pin studies have reported lower bank erosion rates with reduced or no grazing (Kauffman et al. Citation1983; Zaimes et al. Citation2005). Some bank erosion studies using other methods have also reported a positive response of bank erosion or stability with reduced or no grazing (Stuber Citation1985; Lyons et al. Citation2000; Clary and Kinney Citation2002; Scrimgeour and Kendall Citation2003; Kershner et al. Citation2004), while others found no response (Trimble Citation1994; Nellesen et al. Citation2011).
Our range in bank erosion rates from −445 (erosion) to 173 (deposition) mm yr−1 was considerably greater than the ranges reported by others for grazed and cattle-excluded reaches (Kauffman et al. Citation1983; Zaimes et al. Citation2005; Nellesen et al. Citation2011). The finding of both erosion and deposition was consistent with pins installed on a mixture of straight reaches, outside bends and inside bends. Erosion generally occurs on the outside of bends where water velocity is greater, and deposition occurs on the inside of bends where water velocity is lower (Kondolf Citation1996). Kauffman et al. (Citation1983) reported erosion rates ranging from −90 to −270 mm yr−1. Zaimes et al. (Citation2005) reported rates from −31 (erosion) to 2.5 (deposition) mm yr−1, and Nellesen et al. (Citation2011) found erosion rates from −114 to −266 mm yr−1. In contrast, other non-grazing studies have reported wider ranges of bank erosion (Hooke Citation1980; Lawler Citation1993; Harmel et al. 1999) than are reported here.
Particle size and MWD tended to increase with protection from grazing, which is consistent with the observations reported by others (Belsky et al. Citation1999; Agouridis et al. Citation2005; Raymond and Vondracek Citation2011). Therefore, the particle size findings generally support the hypothesis of a positive response to reduced or no grazing. Erosion that increases channel bed fines (silt and fine sand) adversely affects benthic habitat (Magner et al. Citation2008). Therefore, greater particle size with reduced or no grazing may improve the habitat for aquatic life such as benthic invertebrates and fish. Poorly graded sediments with a sizeable fraction of larger material offer a wider range of interstitial void sizes used by different benthic organisims (ASCE Task Committee Citation1992).
Some of the improvements in channel morphology, reductions in bank erosion, and increases in particle-size of riverbed sediment for the fenced reach compared to other two grazed reaches may have been due to improvements in riparian health over time. Although the overall riparian health of the fenced reach was improved after 11 yr of cattle exclusion, some major management issues arose. First, the forage in the fenced riparian pasture could not be utilized by livestock, and standing biomass became excessive. Second, the absence of grazing caused excessive litter accumulation on the soil surface, which increased the fire risk. Third, invasive plants or noxious weeds and disturbance-caused undesirable species became major management issues after 4 to 8 yr of fencing. Therefore, a change from cattle exclusion to periodic grazing of the fenced, riparian pasture was initiated in 2013 to utilize the forage resource, prevent litter build-up and reduce fire risk, as well as control invasive plants and disturbance-caused, undesirable species. Although the riparian health assessments showed no change in channel-related parameters such as streambank structurally altered and channel incisement, quantitative measurements of some channel morphology properties indicated significant differences between grazing treatments. This discrepancy may have been due to the qualitative and visual riparian health assessment conducted over time, whereas the channel morphology measurements were quantitative measurements along different reaches (spatially) within each year.
The management scenario of 11 yr of cattle exclusion followed by 2 years of periodic grazing of the riparian pasture resulted in an improvement of the riparian health score from 2001 to 2015, but the health rating remained unchanged at ‘healthy but with problems’. This rating included a small reduction (7%) after grazing was resumed in 2013. This loss in health score was mainly due to a reduction in preferred tree and shrub establishment and regeneration (Criterion #4) and increased utilization of preferred trees and shrub (Criterion #5). This was most likely caused by increased browsing and trampling of small trees and shrubs by cattle that were re-introduced into the riparian pasture in 2013.
Conclusions
Our findings suggest that managed grazing of riparian pastures is likely required to maintain and improve channel morphology properties and riparian health. The physical properties of rivers and streams are important because they influence hydrology, riparian zone, water flows, water temperatures, sedimentation and habitat for aquatic life such as fish and benthic invertebrates.
Although cattle exclusion generally improved most channel morphology variables and riparian health, serious management issues arose. These included non-utilization of the forage resource, excess litter build-up and fire risk, and increase of disturbance-caused plants and invasive species. Periodic grazing of the fenced riparian pasture when the soils are not wet (to prevent compaction) may be an option to address these management issues. However, long-term research is required to determine the influence of periodic grazing of the fenced riparian pasture on the environment. Additional research is also required to determine whether an improvement in river morphology translates into an improvement for aquatic organisms such as fish and benthic invertebrates.
Funding
Funding for this study was provided by Agriculture and Agri-Food Canada.
Acknowledgements
We thank the landowners for allowing us access to the reaches to conduct this study. We also thank Dwayne Rogness and the County of Lethbridge for their assistance. Field and laboratory assistance was provided by various summer students – Taylor Scriber, Elisha Jones, David Tavernini, Raygan Boyce and Peter Pfitchar – and is gratefully acknowledged. The riparian health assessment of the fenced reach was provided by the Alberta Riparian Habitat Management Society or Cows and Fish program (Noreen Ambrose, Christy Sikina). We also thank the two reviewers for their constructive comments.
References
- Adams, B. W., L. Poulin-Klein, and D. Moisey. 2005. Range plant communities and range health assessment guidelines for the mixedgrass natural subregion of Alberta. Alberta Sustainable Development: Rangeland Management Branch, Public Lands and Forests Division, Lethbridge, AB.10.5962/bhl.title.115370
- Agouridis, C. T., S. R. Workman, R. C. Warner, and G. D. Jennings. 2005. Livestock grazing management impacts on stream water quality: A review. Journal of the American Water Resources Association 41 (3): 591–606.10.1111/jawr.2005.41.issue-3
- Alberta Agriculture and Forestry. 2017. Alberta climate information system. Weather station data viewer. http://agriculture.alberta.ca/acis/ (accessed June, 2017).
- Allen-Diaz, B., R. D. Jackson, and J. S. Fehmi. 1998. Channel morphology change in California’s hardwood rangeland spring ecosystems. Journal of Range Management 51 (5): 514–518.10.2307/4003367
- American Society of Civil Engineers (ASCE) Task Committee. 1992. Sediment and aquatic habitat in river systems. Journal of Hydraulic Engineering 118 : 669–687.
- Baker, V. R. 1977. Stream-channel response to floods, with examples from central Texas. Geological Society of America Bulletin 88 (8): 1057–1071.10.1130/0016-7606(1977)88<1057:SRTFWE>2.0.CO;2
- Belsky, A. J., A. Matzke, and S. Uselman. 1999. Survey of livestock influences on stream and riparian ecosystems in the western United States. Journal of Soil and Water Conservation 54 (1): 419–431.
- Beschta, R. L., and W. S. Platts. 1986. Morphological features of small streams: Significance and function. Water Resources Bulletin 22 (3): 369–379.10.1111/jawr.1986.22.issue-3
- Brady, N. C. 1974. The nature and properties of soils. New York, NY: MacMillan Publ.
- Brand, G., B. Vondracek, and N. R. Jordan. 2014. Influence of grazing and land use on stream-channel characteristics among small dairy farmers in the eastern United States. Renewable Agriculture and Food Systems 30 : 524–536.
- Brandt, S. A. 2000. Classification of geomorphological effects downstream of dams. Catena 40: 375–401.10.1016/S0341-8162(00)00093-X
- Clary, W. P., and J. W. Kinney. 2002. Streambank and vegetation response to simulated cattle grazing. Wetlands 22 (1): 139–148.10.1672/0277-5212(2002)022[0139:SAVRTS]2.0.CO;2
- Dobkin, D. S., A. C. Rich, and W. H. Pyle. 1998. Habitat and avifaunal recovery from livestock grazing in a riparian meadow system of the northwestern Great Basin. Conservation Biology 12 (1): 209–221.10.1046/j.1523-1739.1998.96349.x
- Doll, B. A., G. L. Grabow, K. R. Hall, J. Halley, W. A. Harman, G. D. Jennings, and D. E. Wise. 2003. Stream restoration: A natural channel design handbook. North Carolina Stream Restoration Institute, North Carolina State University, 128 pp. http://www.bae.ncsu.edu/programs/extension/wqg/sri/stream_rest_guidebook/guidebook.html (accessed February, 2017).
- Environment Canada. 2016. Real-time hydrometric data graph for Little Bow River near the mouth (Station 05AC023). http://wateroffice.ec.gc.ca/report/report_e.html?type=realTime&stn=05AC023 (accessed February 7, 2017)
- Fitch, L., and B. W. Adams. 1998. Can cows and fish co-exist? Canadian Journal of Plant Science 78 (2): 191–198.10.4141/P97-141
- Fitch, L., and N. Ambrose. 2003. Riparian areas: A user’s guide to health. Lethbridge, Alberta: Cows Fish Program. ISBN No. 0-7785-2305-5.
- Fitch, L., B. W. Adams, and G. Hale. 2001. Riparian health assessment for streams and small rivers-field workbook. Lethbridge, AB: Cows and Fish Program, 90 pp.
- Fitch, L., B. W. Adams, and G. Hale. 2009. Riparian health assessment for streams and small rivers - field workbook. Second Edition. Lethbridge, Alberta: Cows and Fish Program, 94 pp.http://cowsandfish.org/publications/assessment.html (accessed September, 2017).
- Fleischner, T. L. 2002. Ecological costs of livestock grazing in Western North America. Conservation Biology 8: 629–644.
- George, M. R., R. E. Larsen, N. K. McDougald, K. W. Tate, J. D. Gerlach Jr, and K. O. Fulgham. 2002. Influence of grazing on channel morphology of intermittent streams. Journal of Range Management 55 (6): 551–557.10.2307/4003998
- Goldstein, R. M., M. R. Meador, and K. E. Ruhl. 2007. Relative influence of streamflows in assessing temporal variability in stream habitat. Journal of the American Water Resources Association 43 (3): 642–650. doi:10.1111/j.1752-1688.2007.00051.x.
- Gordon, N. D., T. A. McMahon, B. L. Finlayson, C. J. Gippel, and R. J. Nathan. 2004. Stream hydrology-an introduction for ecologists. Chichester, England: John Wiley and Sons Ltd.
- Green, D. M., and J. B. Kauffman. 1989. Nutrient cycling at the land-water interface: The importance of the riparian zone. Technical Paper No. 8724. Corvallis, OR: Oregon Agricultural Experiment Station.
- Gunderson, D. R. 1968. Floodplain use related to stream morphology and fish populations. The Journal of Wildlife Management 32 (3): 507–514.10.2307/3798929
- Harmel, R. D., C. T. Haan, and R. Dutnell. 1999. Bank erosion and riparian vegetation influences: Upper Illinois River, Oklahoma. Trans. ASAE 42: 1321–1329.
- Herbst, D. B., M. T. Bogan, S. K. Roll, and H. D. Safford. 2012. Effects of livestock exclusion on in-stream habitat and benthic invertebrate assemblages in montane streams. Freshwater Biology 57: 204–217.10.1111/fwb.2011.57.issue-1
- Hillman, E. J., S. G. Bigelow, G. M. Samuelson, P. W. Herzog, T. A. Hurly, and S. B. Rood. 2016. Increasing river flow expands riparian habitat: Influences of flow augmentation on channel form, riparian vegetation and birds along the Little Bow River, Alberta. River Research and Applications 32: 1687–1697.10.1002/rra.v32.8
- Hooke, J. M. 1980. Magnitude and distribution of rates of river bank erosion. Earth Surface and Processes 5: 143–157.10.1002/(ISSN)1931-8065
- Hurlbert, S. H. 1984. Pseduoreplication and the design of ecological field experiments. Ecological Monographs 54 (2): 187–211.10.2307/1942661
- Jennings, G. 2006. Essential stream fundamentals. Powerpoint presentation in Stream restoration construction training, December 13-15. Sponsored by Ecosystem Enhancement Program and NC Stream Restoration Institute, Raleigh https://www.bae.ncsu.edu/bae/workshops/constructiontraining/fundamentals.pdf (accessed February, 2017).
- Johnson, P. A., and T. M. Heil. 1996. Uncertainties in measuring bankfull conditions. Water Resources Bulletin 32 (6): 1283–1291.10.1111/jawr.1996.32.issue-6
- Kauffman, J. B., W. C. Krueger, and M. Vavra. 1983. Impacts of cattle on stream banks in north-eastern Oregon. Journal of Range Management 36 (6): 683–685.10.2307/3898184
- Kershner, J. L., B. B. Roper, N. Bouwes, R. Henderson, and E. Archer. 2004. An analysis of stream habitat conditions in reference and managed watersheds on some federal lands within the Columbia River basin. North American Journal of Fisheries Management 24 : 1363–1375.10.1577/M03-002.1
- Knapp, R. A., and K. R. Matthews. 1996. Livestock grazing, golden trout, and streams in the golden trout wilderness, California: Impacts and management implications. North American Journal of Fisheries Management 16 : 805–820.10.1577/1548-8675(1996)016<0805:LGGTAS>2.3.CO;2
- Kondolf, G. M. 1993. Lag in stream channel adjustment to livestock exclosure, White Mountains, California. Restoration Ecology 1: 226–230.10.1111/rec.1993.1.issue-4
- Kondolf, G. M. 1996. A cross section of stream channel restoration. Journal of Soil and Water Conservation 51 : 119–125.
- Larney, F. J. 2008. Dry-aggregate size distribution. In Soil sampling and methods of analysis, eds. M.R. Carter and E.G. Gregrorich, 821–832. Boca Raton, FL: CRC Press.
- Lawler, D. M. 1993. The measurement of river bank erosion and lateral channel change: A review. Earth Surface Processes Landforms 18 : 777–821.10.1002/(ISSN)1096-9837
- Little, J. 2001. Water quality in the Battersea drain and lower little Bow river (1998–2000)-interim report. Lethbridge, AB: Irrigation Branch, Alberta Agriculture, Food and Rural Development.
- Little, J. L., K. A. Saffran, and L. Fent. 2003. Land use and water quality relationships in the lower little bow river watershed, Alberta, Canada. Water Quality Research Journal of Canada 38 (4): 563–584.
- Lyons, J., B. M. Weigel, L. K. Paine, and D. J. Undersander. 2000. Influence of intensive rotational grazing on bank erosion, fish habitat quality, and fish communities in southwestern Wisconsin trout streams. Journal of Soil and Water Conservation 55 (3): 271–276.
- Magilligan, F. J., and P. F. McDowell. 1997. Stream channel adjustments following elimination of cattle grazing. Journal of the American Water Resources Association 33 (4): 867–878.10.1111/jawr.1997.33.issue-4
- Magner, J. A., B. Vondracek, and K. N. Brooks. 2008. Grazed riparian management stream channel response to southeastern Minnesota (USA) streams. Environmental Management 42: 377–390.10.1007/s00267-008-9132-4
- Miller, J. J., D. S. Chanasyk, T. Curtis, T. Entz, and W. Willms. 2010. Influence of streambank fencing with a cattle crossing on riparian health and water quality of the Lower Little Bow River in southern Alberta. Canada. Agricultural Water Management 97 (2): 247–258.10.1016/j.agwat.2009.09.016
- Miller, J., D. Chanasyk, T. Curtis, T. Entz, and W. Willms. 2011. Environmental quality of Lower Little Bow River and riparian zone along an unfenced reach with off-stream watering. Agriculture Water Management 98 (10): 1505–1515.10.1016/j.agwat.2011.05.006
- Miller, J. J., T. Curtis, D. S. Chanasyk, and W. D. Willms. 2014. Influence of streambank fencing and river access for cattle on riparian zone soils adjacent to the Lower Little Bow River in southern Alberta. Canada. Canadian Journal Soil Science 94 (2): 209–222.10.4141/cjss2013-0981
- Mitchell, P., and E. E. Prepas. 1990. Atlas of Alberta lakes. Edmonton, Alberta: University of Alberta Press. http://albertalakes.ualberta.ca/?page=lake&lake=123®ion=4 (accessed September, 2017).
- Nellesen, S. L., J. L. Kovar, M. M. Haan, and J. R. Russell. 2011. Grazing management effects on stream bank erosion and phosphorus delivery to a pasture stream. Canadian Journal of Soil Science 91 (3): 385–395.10.4141/cjss10006
- Pennock, D. J. 2004. Designing field studies in soil science. Canadian Journal of Soil Science 84 : 1–10.10.4141/S03-039
- Pizzuto, J. E. 1986. Flow variability and the bankfull depth of sand-bed streams of the American midwest. Earth Surface Processes and Landforms 11 (4): 441–450.10.1002/(ISSN)1096-9837
- Platts, W. S. 1981. Sheep and streams. Rangelands 3: 158–160.
- Platts, W. S. 1982. Livestock and Riparian-Fishery interactions: What are the facts? USDA Forest Service / UNL Faculty Publ. Paper 76. http://digitalcommons.unl.edu/usdafsfacpub/76 (accessed June 2017)
- Platts, W. S., and R. L. Nelson. 1985. Stream habitat and fisheries response to livestock grazing and instream improvement structures, Big Creek, Utah. Journal of Soil and Water Conservation 40 (4): 374–379.
- Raymond, K. L., and B. Vondracek. 2011. Relationships among rotational and conventional grazing systems, stream channels, and macroinvertebrates. Hydrobiolgia 669 : 105–117.10.1007/s10750-011-0653-0
- Rood, S.B., and A.R. Kalischuk. 2008. Weed invasion in Alberta’s River valley corridors. World Landscape Architecture (WLA) Magazine, 16 August: 1–3. https://albertawilderness.ca/wordpress/wp-content/uploads/200808_AR6.pdf (accessed Septemeber, 2017).
- Rosgen, D. L. 1996. Applied river morphology. Pagosa Springs CO: Wildland Hydrology Books.
- Samuelson, G. M., and S. B. Rood. 2011. Elevated sensitivity: Riparian vegetation in upper mountain zones is especially vulnerable to livestock grazing. Applied Vegetation Science 14 : 596–606.10.1111/j.1654-109X.2011.01137.x
- Sarr, D. A. 2002. Riparian livestock exclosure research in the western United States: A critique and some recommendations. Environmental Management 30 (4): 516–526.10.1007/s00267-002-2608-8
- SAS Institute Inc. 2002. Release 9.1. Cary, NC: SAS Institute, Inc..
- Scott, J. L. 2012. Evaluating water quality and biotic indices in the Lower Little Bow River, Alberta. M.Sc. Thesis, Univ. of Alberta, Edmonton, AB.
- Scrimgeour, G. J., and S. Kendall. 2003. Effects of livestock grazing on benthic invertebrates from a native grassland ecosystem. Freshwater Biology 48 : 347–362.10.1046/j.1365-2427.2003.00978.x
- Simonson, T. D., J. Lyons, and P. D. Kanehl. 1994. Guidelines for evaluating fish habitat in Wisconsin streams. General Technical Report NC-164. St. Paul, MN: U.S. Department of Agriculture, Forest Service, North Central Forest Experiment Station. https://babel.hathitrust.org/cgi/pt?id=umn.31951d029774196;view=1up;seq=25. (accessed February, 2017).
- Soil Classification Working Group. 1998. The Canadian system of soil classification. Ottawa, ON: NRC Press.
- Stuber, R. J. 1985. Trout habitat, abundance, and fishing opportunities in fenced vs. unfenced riparian habitat along Sheep Creek, Colorado. In Riparian Ecosystems and Their Management, eds. U.S. Forest Service General Technical Report RM-120, 310–314.
- Trimble, S. W. 1994. Erosional effects of cattle on streambanks in Tennessee, U.S.A. Earth Surface Processes and Landforms 19 : 451–464.10.1002/(ISSN)1096-9837
- Trimble, S. W., and A. C. Mendel. 1995. The cow as a geomorphic agent – A critical review. Geomorphology 13 : 233–253.10.1016/0169-555X(95)00028-4
- Vyverberg, K. 2010. A review of stream processes and forms in dryland watersheds. Sacramento, CA: California Department of Fish and Game. https://nrm.dfg.ca.gov/FileHandler.ashx?DocumentID=25779 (accessed July, 2017).
- Zaimes, G. N., R. C. Schultz, and T. M. Isenhart. 2004. Stream bank erosion adjacent to riparian forest buffers, row-crop fields, and continuously-grazed pastures along Bear Creek in central Iowa. Journal of Soil and Water Conservation 59 (1): 19–27.
- Zaimes, G. N., R. C. Schultz, T. M. Isenhart, S. K. Mickelson, J. L. Kovar, J. R. Russell, and W. P. Powers. 2005. Stream bank erosion under different riparian land-use practices in northeast Iowa. In Proceedings of the North American Agroforestry Conference. 9th North American Agroforestry Conference, June 12-15, 2005, Rochester, MN. 2005 CD-ROM. http://www.cinram.umn.edu/sites/cinram.umn.edu/files/zaimes.pdf (accessed February, 2017).