Abstract
Little is known about clouds during drought. From 1999 to 2005 the Canadian Prairies experienced one of the most severe and prolonged droughts in the historical record. This study characterizes clouds during drought in the Canadian Prairie provinces with a particular focus on this recent drought.
Drought severity was determined using the Standardized Precipitation Index (SPI) based on monthly precipitation on a 1° × 1° grid. Cloud fields from the National Aeronautics and Space Administration/Global Energy and Water Experiment's (NASA/GEWEX) Surface Radiation Budget database were used to examine overall cloud amount, optical thickness, and top-of-the-atmosphere albedo. Anomalies in monthly precipitation in the satellite record from 1984 to 2004, with an emphasis on the recent drought from 1999 to 2004, were related to anomalies in cloud fields.
During drought, a decrease in cloud amount was observed. During the spring and summer months of the 1999–2004 drought, for example, the observed cloud cover fraction decreased by approximately 7% between severely wet and severely dry conditions. There was, however, large month-to-month and spatial variability, and the correlation of cloud cover fraction anomaly with precipitation was weak. A higher correlation was found between the top-of-the-atmosphere albedo and precipitation. The occurrence of thick clouds and clouds of medium thickness did decrease with drought severity. These trends also applied to subregions within the overall domain. These observations further the understanding of the role of clouds in feedback mechanisms during drought.
R ésumé [Traduit par la rédaction] On sait peu de choses à propos des nuages durant les sécheresses. De 1999 à 2005, les Prairies canadiennes ont connu l'une des périodes de sécheresse les plus longues et les plus intenses depuis que l'on tient des relevés. La présente étude porte sur les nuages durant les périodes de sécheresse dans les Prairies canadiennes, en mettant l'accent sur les sécheresses récentes.
Nous avons déterminé l'intensité des sécheresses au moyen de l'indice de précipitations normalisé (SPI) fondé sur les précipitations mensuelles sur une grille de 1° × 1°. Nous avons étudié les champs de nuages obtenus de la base de données du bilan radiatif de la surface du GEWEX (Expérience mondiale sur les cycles de l'énergie et de l'eau) de la NASA pour examiner l'étendue générale des nuages, l'épaisseur optique et l'albédo du sommet de l'atmosphère. Nous avons mis en relation les anomalies dans les précipitations mensuelles d'après les données satellitaires de 1984 à 2004, en mettant l'accent sur la sécheresse récente de 1999 à 2004, avec les anomalies dans les champs de nuages.
Pendant les sécheresses, nous avons noté une diminution de l'étendue des nuages. Durant les mois de printemps et d'été de la période de sécheresse de 1999 à 2004, par exemple, la fraction de couverture du ciel observée a diminué d'environ 7 % entre les conditions très humides et très sèches. Cependant, la variabilité intermensuelle et spatiale est grande, et la corrélation de l'anomalie de la fraction de couverture nuageuse avec les précipitations est faible. Nous avons trouvé une meilleure corrélation entre l'albédo du sommet de l'atmosphère et les précipitations. La quantité de nuages épais et de nuages d'épaisseur moyenne diminuait quand l'intensité de la sécheresse augmentait. Ces tendances s'observent aussi dans les sous-régions à l'intérieur domaine général. Ces observations permettent de mieux comprendre le rôle des nuages dans les mécanismes de rétroaction au cours des sécheresses.
1 Introduction
Drought can occur in any region across Canada but the Canadian Prairies are especially prone to this phenomenon. The location of the Prairies on the lee side of the Rocky Mountains disconnects them from the moderating influence of the Pacific Ocean and subsequently they experience a semi-arid to semi-humid climate (Maybank et al., 1994). At any given time, some degree of drought is likely affecting parts of the Prairies. This region's strong dependence on agriculture makes it particularly vulnerable to drought.
Some droughts in this region have been long-lived. Tree ring records from southern Alberta, southwestern Saskatchewan and eastern Montana have shown that, during the eighteenth and nineteenth centuries, these regions frequently experienced decade-long droughts that have been quite severe, interspersed with prolonged periods of wetness. During the twentieth century, however, droughts in this region, although frequent, were relatively short in duration (Sauchyn et al., Citation2003). Many Global Climate Models (GCMs) predict that as global greenhouse gas concentrations increase, the interior of the continent is likely to experience hotter, drier summers, although it is likely to receive more precipitation during the cold season (Sauchyn et al., Citation2003; Christensen et al., Citation2007). If this scenario materializes, especially for regions subject to prolonged droughts such as those that have occurred in the past, the water resources of the Prairies will become exceptionally stressed. Every effort should therefore be made to understand the meteorology of drought, and the triggers and feedback mechanisms associated with drought, in order to be able to predict, prepare for, and mitigate the effects of drought in the future.
Even though the Canadian Prairies are prone to drought due to large-scale factors (see, for example, Bonsal Citation(2008)), once initiated, surface effects can play an important role in maintaining drought. One of the first to suggest that drought begets drought was Namias Citation(1960), who proposed that dry spring conditions favoured the formation and persistence of anticyclones. Subsequent studies have suggested a positive feedback associated with soil moisture, clouds, and precipitation (Charney et al., Citation1977; Eltahir, Citation1998; Schar et al., Citation1999; Pal and Eltahir, Citation2001). For example, a decrease in precipitation initiates dry soil conditions. A decrease in evapotranspiration and moisture would then decrease the amount of moist static energy in the boundary layer (resulting in a decrease in convective available potential energy) and an increase in the cloud base height. This in turn would decrease the frequency of convective events, reducing the total amount of clouds and precipitation, and the cycle continues (Pal and Eltahir, Citation2001). These arguments are supported by a study by Raddatz Citation(2000) on the Canadian Prairies over three summers, from 1997 to 1999, who found that approximately 24–35% of the total rainfall in agricultural regions was recycled. Consequently, under dry conditions, one would expect less precipitation. Local effects, therefore, can play a significant role in the amount of precipitation received at a site, particularly during the warm season.
Roberts et al. Citation(2006) tested part of this feedback scenario for drought on the Canadian Prairies. They found that warmer surface temperatures, along with drier surface conditions and an increase in near-surface energy during drought, did indeed lead to an increase in the height of the cloud ceiling. At the same time, a decrease in the number of occurrences of overcast skies and an increase in the number of observations of clear skies were observed. Consequently, fewer hours and lower accumulations of precipitation were observed. Intriguingly, while it was thought that fewer clouds would be observed during drought, this was not the case. Even during drought and extreme drought, clouds were observed 87% and 84% of the time, respectively. They did find that there were fewer occurrences of low clouds and an increase in the occurrence of high clouds during drought. Roberts et al. Citation(2006) utilized surface observations and identified the need for additional studies including satellite-based studies of cloud fields, to obtain a more comprehensive depiction over drought regions.
The Canadian Prairies experienced a multi-year drought that began in the fall of 1999 and extended over multiple seasons, not being fully relieved in all sectors until midway through 2005 (Stewart et al., Citation2008). The 2001 and 2002 drought years were particularly harsh for farmers on the Prairies, as well as for the businesses that supported them. Southern Alberta and southwestern Saskatchewan started to experience well-below normal precipitation during the winter of 2000/01. This trend continued throughout 2001 and into the summer of 2002 (Wheaton et al., Citation2005). Overall, these two summers were not the driest on record for the southern Prairies, but the fact that they occurred during a long period of below-average precipitation contributed to the widespread devastation (Bonsal and Regier, Citation2007). For particular stations, such as Saskatoon and Prince Albert, Saskatchewn, the 2001/02 drought was indeed the driest on record (Bonsal and Regier, Citation2007).
This drought was distinct for several reasons. For example, the area affected by below-average precipitation was widespread; it was well documented, and it extended over multiple years (Stewart et al., Citation2008). The drought is the focus of the Drought Research Initiative (DRI), a research network of scientists with a wide spectrum of backgrounds (atmospheric, hydrological, land surface, and predictive), dedicated “to better understand the physical characteristics of and processes influencing Canadian Prairie droughts, and to contribute to their better prediction, through a focus on the recent severe drought that began in 1999 and largely ended in 2005.” (Stewart et al., Citation2008).
One of DRI's main objectives is to quantify the characteristics of drought better, including the associated cloud fields. Periods of reduced precipitation must be related to changes in the properties of clouds during those periods compared to normal conditions. For example, there may be changes in the cloud amount, cloud thickness or, although not addressed in this study, the microphysical properties of the clouds such as cloud droplet sizes and cloud droplet number concentrations. If we are to understand the causes of drought and the possible feedbacks that may affect the severity and length of a drought, we need to understand the ways in which the clouds are different during drought. Previous studies (Namias, Citation1960; Charney et al., Citation1977; Eltahir, Citation1998; Schar et al., Citation1999; Pal and Eltahir, Citation2001; Roberts et al., Citation2006) have proposed local feedbacks associated with drought in which clouds are an integral part. They have not, however, exploited the wealth of satellite information, nor has the contribution of clouds been quantified. In addition, the relationships generated here will be useful in evaluating the relationships between the precipitation anomalies and cloud anomalies from numerical models that are being used to simulate droughts on the Prairies as part of DRI.
It is expected that cloud amounts will decrease as precipitation decreases, but how much will it decrease? Are there exceptions? What types of clouds are present during drought? Where was the drought most severe during the 1999–2004 event, and how did the cloud fields differ in those locations compared to others that did not experience severe drought? How did the clouds during the 1999–2004 drought differ from other droughts during the satellite record? This study attempts to answer some of these questions by examining the satellite record to see how clouds over the Canadian Prairies differ during various moisture conditions. In particular, the relationship between drought and the overall mean cloud amount as well as the mean cloud amount for low, middle, and high clouds; optical thickness; and the top-of-the-atmosphere albedo over the Canadian Prairies with particular emphasis on the 1999–2004 drought are examined.
2 Data
There are many definitions of drought, and each is appropriate for a particular characterization or impact of drought. For the purpose of this study, since drought is being related to cloud fields, the Standardized Precipitation Index (SPI) developed by McKee et al. Citation(1993), which depends only on precipitation, is used to define drought severity. SPI is a measure of the departure of the precipitation for a particular time period (one month in this study) from the long-term mean with negative and positive values indicating below- and above-average precipitation, respectively. The monthly SPI over the Prairie provinces was calculated from the Canadian Gridded Climate Data (CANGRID) monthly precipitation (Seglenieks and Soulis, Citation2000) for the period 1950–2004 following the methodology of Edwards and McKee Citation(1997). The 50 km x 50 km CANGRID data were transcribed onto a 1° × 1° grid before calculation of the SPI so that the SPI had the same spatial resolution as the satellite data used to describe the cloud fields. lists the relationship between SPI and cumulative precipitation and it also defines the qualitative descriptors used in the text below.
Table 1. SPI, corresponding cumulative probability, and the precipitation classification used here (Edwards and McKee, Citation1997).
Cloud fields were taken from the Surface Radiation Budget (SRB) Longwave Cloud Properties release 2.5 dataset and the Shortwave Monthly Radiation release 2.81 dataset, for the period January 1984 to December 2004. These data were obtained from the National Aeronautics and Space Administration's (NASA) Langley Research Center, Atmospheric Science Data Center. From the Longwave Cloud Properties dataset, the data that were relevant to this work are the 1° gridded 3-hourly mean cloud amounts for high, middle, and low cloud, and the 3-hourly gridded cloud optical thickness. From the Shortwave Monthly Radiation dataset, the 1° gridded upward and downward fluxes at the top of the atmosphere were obtained to calculate the albedo and the mean cloud amount. These datasets are derived from the International Satellite Cloud Climatology Project (ISCCP) data (Rossow and Schiffer, Citation1991, Citation1999).
3 Drought distribution
One objective of this study is to understand the relationships between cloud fields and precipitation during the recent drought over the Canadian Prairie provinces from September 1999–December 2004 and to compare those relationships with equivalent relationships for the full satellite record from 1984–2004. The Canadian Prairie provinces are vast and not all areas were affected equally. It is important, therefore, to identify particular regions that were more affected by precipitation deficits than others. To investigate this further, the number of months during the recent drought period that experienced below-normal precipitation was determined for each 1° × 1° grid point.
shows the number of months during the drought period that experienced an SPI ≤ –0.5, which indicates mild or severe drought. The area that experienced drought conditions most frequently is located near Fort McMurray, Alberta (57°N, 110°–113°W), which experienced 30–31 months (i.e., approximately half of the possible months) of below-normal precipitation. While drought did occur throughout the entire period, it was especially dry during the early years of the drought, particularly during the winter months. The regions west of Edmonton (53°N, 112°–116°W), between Edmonton and Edson; the area north and east of Medicine Hat (50°N, 110°–112°W); and a larger region surrounding Fort McMurray (55°–58°N, 110°–113°W) experienced 28 or more months of drought. Secondary maxima of 24 or more months of drought include the region flanking the Saskatchewan/Alberta border, extending west towards Edmonton and north along the Alberta/Northwest Territories border.
Fig. 1 Number of months with (a) drought (SPI ≤ −0.5) and (b) severe drought (SPI ≤ −1.5) from September 1999–December 2004. The white circles indicate, from north to south, the locations of Fort McMurray, Edmonton, and Medicine Hat.
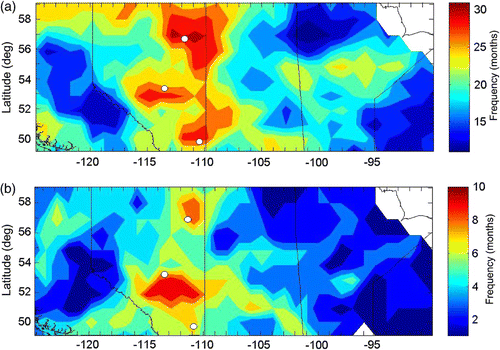
displays the frequency of severe monthly drought (SPI ≤ –1.5). Again the eastern half of Alberta was the area most affected in this respect, particularly the areas south and east of Edmonton (52°–53°N, 111°–115°W). This region had a maximum of 10 severely dry months. While severe drought did occur during all the years, this area was particularly stressed during the summer of 2002, when severe drought occurred from May to July. The region in the vicinity of Fort McMurray (57°–58°N, 111°W) also experienced a high number of months with severe drought (9 months). Although the definition of SPI implies that, if monthly precipitation were distributed randomly there would be a probability that during this five-year period some grid points would have an above-average number of dry and severely dry months, these probabilities are unlikely to account for the observed number and distribution of pixels with high frequencies of dry and severely dry months.
shows the number of months with drought (SPI ≤ –0.5) on a seasonal basis. Most areas experienced drought in all seasons; the maximum number of drought months for any particular season, however, occurred during the fall and winter. The area flanking the Alberta/Saskatchewan border stands out in the winter and fall as having a high occurrence of drought. In summer, no particular region featured a large number of drought months, but the northern parts of all three provinces as well as the southern half of Alberta experienced more drought during summer than other regions.
4 Cloud characteristics
Average cloud amount over the Canadian Prairie provinces varies both spatially and temporally. The average cloud amount from May–September is approximately 68% with a year-to-year standard deviation of approximately 6%, the summer months being slightly less cloudy, with an average cloud amount of approximately 63%. In order to relate monthly cloud amount to monthly precipitation, the monthly cloud anomaly was determined by subtracting the monthly mean cloud amount for each grid square calculated from the
21-year dataset. The mean cloud anomalies averaged over the three Canadian Prairie provinces (49°–60°N and 95°–120°W) for each of the precipitation categories listed in are shown in .
The average cloud cover anomaly almost always decreases as drought severity increases, the exception being during the winter (January, February, and March) when the cloud cover anomalies during severely wet conditions are actually more negative than for drought and normal conditions. It is likely that this inconsistency is due to the unreliable retrievals of cloud amounts over snow. The maximum difference in the anomalies between severely wet and severely dry conditions occurred in September with a 14% difference. The minimum, excluding February, occurred in June with a difference of 6%. A similar trend is obtained when looking only at the recent drought period from 1999–2004. If the anomalies for mean cloud amount are averaged over the warm season, May to September, then for this period, the mean cloud amount during severe drought is approximately 7% less than during severely wet conditions.
There is considerable spatial variability in the mean cloud amount anomaly throughout the Canadian Prairie provinces. The monthly mean cloud amount anomaly was averaged for severely dry conditions and for severely wet conditions, for each grid point during the warm season (May–September) from 1984–2004 (). shows a similar plot for drought conditions during the recent drought period (September 1999–December 2004).
Fig. 4 Average monthly mean cloud amount anomaly (%) for the period May–September from 1984–2004 for a) severely dry conditions and b) severely wet conditions.
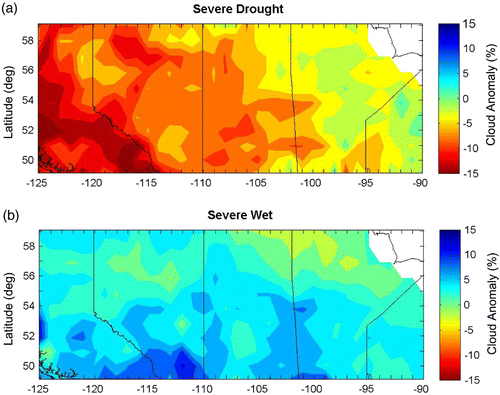
Fig. 5 Mean cloud amount anomaly (%) during drought for the recent drought period (September 1999–December 2004) over the Prairie provinces from May–September.
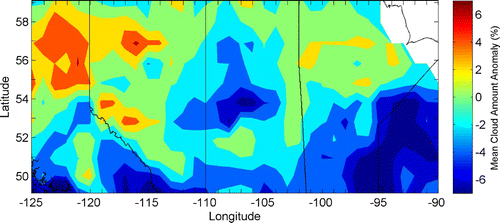
Over the satellite record, during severely dry conditions, higher anomalies existed in Saskatchewan and Alberta. Southern Alberta, bordering British Columbia, experienced the highest negative anomalies where cloud amounts during severely dry conditions were 11% to 15% below the long-term mean. Much of the rest of Saskatchewan and Alberta had cloud anomalies ranging from 5% to 11% below average. Manitoba generally had mean cloud amounts 1% to 5% below expected values but some cloud anomalies ranged from 9% below to 2% above expected values.
The highest values of mean cloud anomalies during severely wet conditions again occurred in southern Alberta (49°–51°N, 112°–115 °W ) ranging from 7% to 12% above expected values. Northern parts of all three provinces (57°–59°N, 95°–120°W) showed very little departure from the long-term mean. The average anomaly was 2% above normal but ranged from –2% to +6%.
While this analysis does show a general trend in the spatial variability of cloud anomalies during severe conditions, the cumulative probability of severe drought (from ), using the SPI, is a mere 0.067. With only 21 years of satellite data available, and focusing specifically on the warm season, each grid point is likely to experience severe drought eight times. This is not enough for a climatological record; a minimum of 30 occurrences of both severe drought and severe wet conditions would be best. Further analysis with a longer dataset would, therefore, be ideal to get a true representation of the differences in cloud cover between various moisture conditions.
Under drought conditions, anomalies averaged from 1984–2004 were generally smaller than during severe drought with no significant spatial variability. Mean cloud amount anomalies ranged from –1% to –4% during drought and from +1% to +4% during wet conditions. Similarly, during the recent drought, most regions experienced cloud amount anomalies 1% to 4% below the long-term mean. There were, however, a few exceptions (see ). A region in western and northern Alberta (52°–54°N, 121°–124°W and 56°–58°N, 121°–125°W) actually experienced cloud amounts 1% to 6% above the long-term mean. In central Saskatchewan (54°N, 104°–109°W), on the other hand, mean cloud amount anomalies were 4% to 7% below normal. While this does show the average cloud cover anomalies during this drought, some variability is to be expected from the long-term average, since means were taken over only five to six years.
Although there is a general increase in the mean cloud amount anomaly from negative values to positive as the SPI goes from negative (dry months) to positive (wet months) values, there is a large amount of variability. To illustrate this, displays the monthly cloud amount anomaly and the corresponding SPI as a scatter plot for each grid point and for each month during the warm season from 1984 to 2004. The data were fitted using a least squares technique. As is evident from the plot, the monthly mean cloud amount anomaly and the SPI are only weakly correlated, with only 17% of the variation in the monthly SPI accounted for by variations in mean cloud amount.
Fig. 6 Monthly mean cloud amount anomaly (%) and monthly Standardized Precipitation Index (SPI) for the months May to September over the Prairie provinces from 1984 to 2004. The solid line represents the line of best fit.
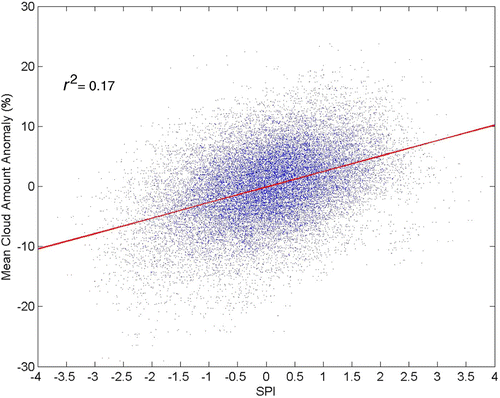
Similar regressions between mean cloud amount anomaly and SPI were calculated for individual months from May to September, for the 21-year cloud dataset and for the period of the recent drought. Also, the data for the entire 21-year period were divided geographically into the boreal forest region (54°–56°N and 95°–114°W), predominantly the northern portions of the provinces, and the agricultural region (49°–53°N and 103°–114°W and 49°–51°N and 50°–102°W), which is predominantly in the southern portions of the provinces; separate regressions were calculated for each region (). (A land cover map of the Canadian Prairie provinces may be seen on the DRI web site at http://www.drinetwork.ca/Prairie-River-LandCover.jpg). The most notable differences in the values of r 2 are seen in the values for May and June when the correlations were very low and the following three summer months when the correlations were much higher. The exception was the boreal region in May which had a correlation greater than or comparable to the correlations in the summer months. The correlations in the summer months of the recent drought were similar to those for the full 21-year period. Cloud amounts tended to be higher during the recent drought period, with average cloud amount anomalies being about 2% larger for every precipitation category compared to the anomalies over the 21-year period.
Table 2. The coefficient of determination, r 2, for the mean cloud amount anomaly and the SPI from May to September for four categories: the entire record (1984–2004) for the full domain and the agricultural and boreal sub-regions, and for the full domain during the 1999–2004 drought.
The weak correlations between monthly average cloud amount and monthly SPI, which are especially evident in May and June, may be due to a combination of errors in the retrieved cloud amounts, errors in the gridded values of precipitation, cloud characteristics other than cloud amount (e.g., cloud thickness, cloud microphysics) important in determining the amount of precipitation, and monthly precipitation dominated by small-scale events that do not have a strong effect on the grid-average cloud amount. To gain insight into possible explanations, correlations were calculated between monthly SPI and the monthly average albedo anomaly (). The albedo was calculated by taking the ratio of the upward flux of shortwave radiation to the downward flux at the top of the atmosphere. The correlations of SPI with the albedo anomaly, though still not high, are consistently higher than those with cloud amount anomaly. This is especially apparent during the months of May and June, suggesting that errors in cloud retrieval and/or the importance of cloud optical depth rather than cloud amount are responsible for some of the weak correlations in .
Table 3. Comparison of the coefficient of determination, r 2, between mean cloud amount anomaly and albedo anomaly against SPI for each month from May to September over the period from 1984 to 2004 and the 1994 to 2004 drought.
The SRB cloud data include information on the 3-hourly 1° × 1° grid average cloud thickness in three cloud thickness classes defined by Rossow and Schiffer Citation(1999). Grouping the data for May to September according to the SPI showed that, when clouds were present, the frequency of thin cloud was 15% greater in severely dry months compared to severely wet months and, conversely, the frequency of medium thickness clouds was 15% less.
The tendency for smaller cloud anomalies in months with lower values of SPI persists for high cloud (cloud top pressure less than 440 hPa) and medium cloud (cloud top pressure less than 680 hPa) with cloud anomalies being approximately 6% greater during severely wet months than during severely dry months (). The relationship between low cloud and cloud anomalies during severely dry months was found to have the opposite trend, being approximately 3% greater than during severely dry months. However, this might be a partial artifact since, as medium and high cloud amounts diminish, more low cloud may become visible to the satellite. The same trends were found for the 1999–2004 drought period but cloud amounts were, on average, slightly larger for all moisture categories.
Fig. 7 Cloud anomalies, for the period 1984–2004, for the months May to September grouped according to moisture (SPI) category.
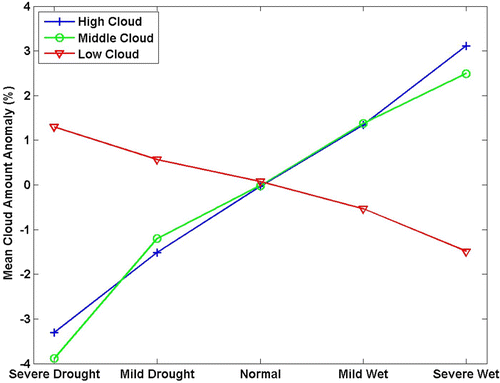
Higher amounts of low clouds observed during drought could, in fact, be due to the enhanced sensible heat flux associated with dry soil. Rabin et al. Citation(1990) observed that shallow cumulus clouds developed earliest over drier, hotter surfaces. The resolution of the SRB satellite data is relatively coarse, so to observe this increase cumulus development would have to be fairly widespread. This could also explain the observed increase in low cloud amounts during drought compared to normal or wet conditions.
5 Summary
It is a given that variations in precipitation must be a consequence of variations in cloud fields. For months that have below-average precipitation the clouds must be, on average, different from the clouds present during wetter months. Understanding the relationships between clouds and precipitation for the Canadian Prairies will aid in understanding the causes of prolonged drought. Those relationships also impose constraints on the numerical models that are used to predict precipitation or the lack thereof. If the model predictions of precipitation are to be believed, the models must be able to reproduce the observed relationships between clouds and precipitation.
During the Prairie drought of 1999–2004, the region that experienced the greatest number of months of drought or severe drought was concentrated in central and eastern Alberta and western Saskatchewan (). The seasons with the highest number of months with below-average precipitation are winter (December–February) and, to a lesser extent, fall (September–November).
The length of the 1999–2004 drought was too short to identify a relationship between cloud characteristics and precipitation anomaly; although some attention was given to the recent drought, this study focussed on the 21-year period from 1984 to 2004. The cloud fields that might be expected to relate to precipitation are cloud amount, cloud thickness, and cloud height, although microphysical properties and below-cloud humidity would also be factors. The cloud fields were obtained from satellite measurements and so are unreliable over high albedo snow-covered surfaces, accordingly this analysis concentrated on the months of May to September.
There is a clear trend for months with below-average precipitation to have negative cloud amount anomalies. However, the value of r 2 is small (0.17); very little of the SPI variation is explained by total cloud amount. The regressions are stronger during the late summer months, particularly in the southern agricultural domain (r 2 = 0.23–0.37) where the precipitation measurement network is denser. Subdividing cloud according to cloud height showed that medium and high cloud decreased with decreasing SPI.
Since using cloud amount and cloud optical thickness together is likely to predict precipitation better, and because cloud amount values may not be reliable when pixels are only partially cloud covered, correlations between SPI and albedo anomaly were calculated. This resulted in slightly better correlations with corresponding values between 0.27 and 0.40. However, because the correlation between albedo and SPI is restricted to daytime measurements, diurnal differences in cloud fields may also contribute to the differences in the correlations for cloud cover and albedo.
The analysis conducted in this study utilizes the best known available datasets; nevertheless, there are some limitations that need to be addressed. CANGRID data are an interpolated grid from surface observations. In Canada, southern portions of most provinces are well represented, often with numerous stations in a 1° × 1° area. Data coverage in northern areas, however, is sparse, particularly in northern Saskatchewan and Manitoba. This means that it is possible for a particular region to be represented by an incorrect value of precipitation as it is possible for the nearest station to be hundreds of kilometres away and hence reproduce an inaccurate SPI.
The question arises as to why the correlations are not stronger. Uncertainties in the measurements and smoothing of the gridded data may be partly responsible but what is probably more important are the contributions of intense but small sub-grid storms that have a greater effect on the grid-averaged precipitation than on the grid-averaged cloud amount or albedo.
Acknowledgements
We are grateful to William Henson for so willingly sharing his technical expertise and to Kit Szeto for providing the precipitation data. This work was part of the Drought Research Initiative funded by the Canadian Foundation for Climate and Atmospheric Sciences (CFCAS).
Additional information
Notes on contributors
Heather Greene
Current affiliation: Meteorological Service of Canada, Environment Canada, Edmonton, AlbertaRonald E. Stewart
Current affiliation: Department of Environment and Geography, University of ManitobaReferences
- Bonsal , B. 2008 . Droughts in Canada: an overview . CMOS Bull. , 36 : 79 – 86 .
- Bonsal , B. and Regier , M. 2007 . Historical comparison of the 2001/2002 drought in the Canadian Prairies . Clim. Res. , 33 : 229 – 242 .
- Charney , J. , Quirk , W. J. , Chow , S. and Kornfield , J. 1977 . A comparative study of the effects of albedo change on drought in semi-arid regions . J. Atmos. Sci. , 34 : 1366 – 1385 .
- Christensen , J. H. , Hewitson , B. , Busuioc , A. , Chen , A. , Gao , X. , Held , I. , Jones , R. , Kolli , R. K. , Kwon , W.-T. , Laprise , R. , Magaña Rueda , V. , Mearns , L. , Menéndez , C. G. , Räisänen , J. , Rinke , A. , Sarr , A. and Whetton , P. 2007 . “ Regional climate projections ” . In Climate Change 2007: The Physical Science Basis. Contribution of Working Group I to the Fourth Assessment Report of the Intergovernmental Panel on Climate Change , Edited by: Solomon , S. , Qin , D. , Manning , M. , Chen , Z. , Marquis , M. , Averyt , K. B. , Tignor , M. and Miller , H. L. Cambridge, United Kingdom and New York, NY, USA : Cambridge University Press .
- Edwards , D. C. and Mckee , T. B. 1997 . “ Characteristics of 20th century drought in the United States at multiple time scales ” . 155 Fort Collins, CO : Dept. of Atmos. Sci., CSU . Climatology Report 97-2
- Eltahir , E. A.B. 1998 . A soil moisture-rainfall feedback mechanism 1. Theory and observations . Water Resour. Res. , 34 : 765 – 776 .
- Maybank , J. , Bonsal , B. , Jones , K. , Lawford , R. , O'Brien , E. G. , Ripley , E. A. and Wheaton , E. 1995 . Drought as a natural disaster . Atmosphere-Ocean , 33 : 195 – 222 .
- Mckee , T. B. , Doeskin , N. J. and Kleist , J. The relationship of drought frequency and duration to time scales . Preprints, Proc 8th Conf Appl. Climatol. January 17–22 1993 . pp. 179 – 184 . Anaheim, CA : Am. Meterol. Soc. .
- Namias , J. 1960 . “ Factors in the initiation, perpetuation and termination of drought ” . In In Publication 51, Association of Scientific Hydrology, International Union of Geodesy and Geophysics 81 – 94 .
- Pal , J. S. and Eltahir , E. A.B. 2001 . Pathways relating soil moisture conditions to future summer rainfall within a model of the land-atmosphere system . J. Clim. , 14 : 1227 – 1242 .
- Rabin , R. M. , Stadler , S. , Wetzel , P. J. , Stensrud , D. J. and Gregory , M. 1990 . Observed effects of landscape variability on convective clouds . Bull. Am. Meteorol. Soc. , 71 : 272 – 280 .
- Raddatz , R. L. 2000 . Summer rainfall recycling for an agricultural region of the Canadian Prairies . Can. J. Soil Sci. , 80 : 367 – 373 .
- Roberts , E. , Stewart , R. E. and Lin , C. A. 2006 . A study of drought characteristics over the Canadian Prairies . Atmosphere-Ocean , 44 : 331 – 345 .
- Rossow , W. B. and Schiffer , R. A. 1991 . ISCCP cloud data products . Bull. Am. Meteorol. Soc. , 72 : 2 – 20 .
- Rossow , W. B. and Schiffer , R. A. 1999 . Advances in understanding clouds from ISCCP . Bull. Am. Meteorol. Soc. , 80 : 2261 – 2287 .
- Sauchyn , D. J. , Stroich , J. and Beriault , A. 2003 . A paleoclimatic context for the drought of 1999–2001 in the northern Great Plains of North America . Geograph. J. , 169 : 158 – 167 .
- Schar , C. , Luthi , D. , Beyerle , U. and Heise , E. 1999 . The soil-precipitation feedback: a process study with a regional climate model . J. Clim. , 12 : 722 – 741 .
- Seglenieks , F. and Soulis , R. 2000 . “ Generation of square grid normals for Canada – Phase 1 ” . Downsview : Meteorological Service of Canada . U. Waterloo Contractor's Report to Climate Research Branch
- Stewart , R. , Pomeroy , J. and Lawford , R. 2008 . A drought research initiative for the Canadian Prairies . CMOS Bull. , 36 : 87 – 96 .
- Wheaton , E. , Wittrock , V. , Kulshreshtha , S. , Koshida , G. , Grant , C. , Chipanshi , A. and Bonsal , B. 2005 . “ Lessons learned from the drought years of 2001 and 2002: synthesis report ” . Saskatoon : Agriculture and Agri-Food Canada, Saskatchewan Research Council Publ. No. 11602-46E03 .