Abstract
This study focuses on cloud and precipitation features over southern Baffin Island and the adjacent oceanic regions for the period 1 October to 30 November 2007. This period corresponds with the Storm Studies in the Arctic (STAR) field research project based in Iqaluit, Nunavut, Canada. Satellite data from CloudSat and Aqua were obtained from which a number of parameters were used to examine clouds and precipitation. A total of 91 orbital segments were examined comprising 82,388 vertical profiles.
During the observation period, clouds were observed 86% of the time, but precipitation was only observed 13% of the time when clouds were present, and there was a preference for the precipitation to occur over regions of higher terrain. The mean cloud top height and temperature were 2.3 km above sea level (ASL) and −20°C, respectively, but 18% of tops were higher than 7 km. The majority (76%) of clouds were characterized by single-layer clouds although multi-layer clouds were also common with up to four cloud layers sometimes present.
Four significant storm events, including the remnants of Hurricane Noel, were studied in greater detail. These were deep cloud systems (more than 5 km thick) with high and cold cloud tops (higher than 7 km ASL and as low as −65°C, respectively) and layering (up to four layers). Strong vertical and horizontal variations in reflectivity indicated, for example, regions of sublimation and/or evaporation aloft as well as orographic precipitation. Frequency diagrams of radar reflectivity profiles illustrated varying precipitation production features including layers of precipitation growth and reduction.
R ésumé [Traduit par la rédaction] Dans la présente étude, nous nous penchons sur les caractéristiques des nuages et des précipitations dans la partie méridionale de l’île de Baffin ainsi que dans les zones océaniques adjacentes entre le 1er octobre et le 30 novembre 2007. C'est entre ces deux dates que s'est déroulée la campagne météorologique du projet de recherche Storm Studies in the Arctic (STAR) dans la localité d'Iqaluit au Nunavut, Canada. Nous avons utilisé les données des satellites CloudSat et Aqua pour établir un certain nombre de paramètres en vue d’étudier les nuages et les précipitations. En tout, nous avons examiné 91 portions d'arc d'orbite, comprenant 82,388 profils verticaux.
Au cours de la période visée, nous avons observé des nuages 86% du temps, mais des précipitations, 13% du temps seulement lorsqu'il y avait des nuages. Les précipitations se sont produites surtout dans les régions en plus haute altitude. En moyenne, le sommet des nuages atteignait 2,3 km de haut par rapport au niveau de la mer (ASL), et la température, −20°C, mais 18% des sommets dépassaient 7 km d'altitude. Dans la majorité des cas, il s'agissait de nuages monocouches (76%). Toutefois, nous avons aussi souvent observé des nuages multicouches, le nombre de couches superposées pouvant aller jusqu’à quatre.
Nous avons étudié de façon plus exhaustive quatre tempêtes majeures, notamment les restes de l'ouragan Noël. Il s'agissait de systèmes de nuages épais (plus de 5 km) caractérisés par des sommets froids de nuages élevés (plus de 7 km ASL et des températures inférieures à −65°C) et des couches superposées (jusqu’à quatre couches). Les fortes variations verticales et horizontales de réflexivité indiquaient notamment les secteurs de sublimation et d’évaporation dans le ciel, de même que les précipitations orographiques. Nous avons illustré, au moyen de diagrammes de fréquence de la réflexivité radar, la variation des caractéristiques de la production de précipitations, notamment la progression et la diminution des quantités de précipitations.
Keywords:
1 Introduction
A great deal of attention has been directed towards the impact of increasing temperatures in the polar regions. Aside from issues involving melting sea ice and its impact on the thermohaline circulation, the high latitudes are expected to witness an increase in precipitation as well as cloud cover over the course of the current century (IPCC, Citation2007).
This increase in overall Arctic cloudiness and precipitation is expected to have profound impacts on local ecosystems as well as on many communities scattered across the Arctic. However, continued research on clouds and precipitation across the Arctic is necessary because not all parts of the Arctic have been well studied. Because of this, the forecasting and modelling of clouds and precipitation in the Arctic continue to be difficult problems.
Extensive research on clouds and precipitation has already been carried out in the western half of the Canadian Arctic. A number of major field campaigns have been conducted in this region with the purpose of observing and analyzing Arctic clouds, precipitation and storm systems as they interact with the surrounding surface. The formation of snow crystals was first studied in the late 1990s in the western Canadian Arctic (Kikuchi and Kajikawa, Citation1979; Magano and Kikuchi, Citation1980). Other major field projects, such as the Beaufort and Arctic Storms Experiment (BASE; Hanesiak et al., Citation1997; Asuma et al., Citation1998), the Arctic Cloud Experiment and the Mixed-Phased Arctic Cloud Experiment (Curry et al., Citation2000; Yannuzzi et al., Citation2005), and the Mackenzie Global Energy and Water Cycle Experiment (GEWEX) (Stewart and Burford, Citation2002; Hudak et al., 2004; Stewart et al., Citation2004) have all contributed to understanding the role and impact of clouds and precipitation within the western Canadian Arctic.
Despite these research efforts, uncertainties in characterizing clouds and precipitation continue, especially within the poorly studied eastern Canadian Arctic. However, it is recognized that the formation of clouds and precipitation in the Arctic arises through a variety of mechanisms. Some of these include large-scale forcing conditions leading to local convection and synoptic systems (Hudak et al., Citation2004), alterations in the atmospheric moisture content from seasonal sea-ice coverage, and surface ice-pack sublimation (Curry et al., Citation1996; Beesley and Moritz, Citation1999). The production of precipitation is especially difficult because many Arctic storms actually produce little or no precipitation, in part, because of sublimation below the cloud base (Stewart et al., Citation2004).
Measurements of clouds and precipitation over large regions are typically made using satellites. However, satellites in geosynchronous orbit do not provide good coverage of the Arctic, and satellites such as the Tropical Rainfall Measuring Mission satellite concentrate solely on low or mid-latitudes.
To begin to address such concerns, the National Aeronautics and Space Administration (NASA), with contributions from the Canadian Space Agency (CSA) and other international agencies, launched the CloudSat satellite on 29 April 2006. CloudSat is one of six satellites flying in formation that collectively form the A-Train. More information about CloudSat can be found in Stephens et al. (Citation2002), and studies examining the validation of CloudSat information can be found in Barker et al. (Citation2008), Hudak et al. (Citation2008) and Noh et al. (Citation2011). The validation studies indicate that the data presented in this paper compare well to in situ measurements and the agreements are further enhanced when ancillary data from other satellites are used.
Using data collected by CloudSat in conjunction with data from Aqua, also an A-Train satellite, as well as other ancillary information, this paper characterizes the clouds and some aspects of the precipitation over southern Baffin Island and surrounding regions as part of the Storm Studies in the Arctic (STAR) autumn 2007 field campaign (Hanesiak et al., Citation2010). Such an investigation has not been carried out before over this region.
The article is arranged as follows. Section 2 discusses the instruments and datasets used and presents a general description of the region. Section 3 provides an analysis of clouds and precipitation features observed during the field campaign. Section 4 examines four major weather events in detail and Section 5 discusses and synthesizes key aspects of the observations. Concluding remarks are made in Section 6.
2 Data sources and methodology
a Location
The STAR autumn field campaign was the first to utilize CloudSat and Aqua satellite data as well as ancillary information to characterize clouds and precipitation in the eastern Canadian Arctic. The field campaign, conducted between 1 October and 30 November 2007 over southern Baffin Island, was the second Canadian-led project (the first was BASE in 1994) to focus solely on Arctic storms and their associated hazards (Hanesiak et al., Citation2010). The goal for STAR was to improve our understanding of the physical characteristics of Arctic storms and their associated hazards as well as the predictability of these types of events.
The STAR campaign was based in Iqaluit, Nunavut, which was selected because of its importance as a transportation hub and population centre. Iqaluit (63.75°N, 68.55°W) is located in a fjord-river valley combination at the head of Frobisher Bay on southern Baffin Island and flanked by mountain ridges on two peninsulae, Meta Incognita Peninsula and Hall Peninsula (). These mountain peaks reach altitudes greater than 0.6 km and 1.0 km above sea level (ASL), respectively. Because of its unique location with respect to the highly varied terrain and proximity to the ocean, numerous high wind speed events and high-precipitation events linked to the topography have been observed in Iqaluit (Maxwell, Citation1981; Nawri and Stewart, Citation2006; Gascon et al., Citation2010).
Fig. 1 (a) Illustrative example of 34 A-Train ground tracks to fall within a 1000 × 1000 km boxed region surrounding Iqaluit, Nunavut. Morning orbits (utc) are identified in red and afternoon orbits are identified in black. Arrows at the top represent the direction of the corresponding orbit. The spatial scale is shown by the bar on the right hand-side of the diagram. (b) Overview of the southern Baffin Island region with key landmarks identified (adapted with the permission of Natural Resources Canada, courtesy of the Atlas of Canada).
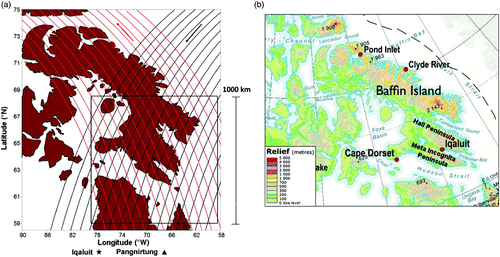
b Satellite Observations
Spaceborne observations between 1 October and 30 November 2007 (two complete months, 61 days in total) were collected using the A-Train constellation of satellites. The primary data were collected by the CloudSat and Aqua satellites which are two of the six A-Train satellites. The collective information from these satellites was the basis for the comprehensive analysis of clouds and precipitation occurring over the region.
Because of its geographic location, these spaceborne observations of the Baffin Island region are obtained two to three times over a 24-hour period. Overall, during the experimental period, a total of 138 orbital segments along 34 unique tracks were identified. These encompass a wide variety of terrain types and combinations. However, only data from 91 of the 138 orbital segments (comprising 82,388 vertical profiles) were available, retrieved and used in the analysis. It is not known at this time why 47 orbital segments were unavailable.
Each orbit of the three satellites within a 1000 × 1000 km boxed region surrounding Iqaluit is shown in . These orbits covered a mean distance of 996 km and most of the orbits passed over highly varied terrain and deep valleys which are defining features of southern Baffin Island. In total, the land area within the box is 424,600 km2, which represents 42% of the observational area, and this is consequently the percentage of vertical profiles over land rather than over open ocean and/or sea ice. As well, 21% and 1.3% of the vertical profiles were over terrain higher than 200 m and 1000 m, respectively.
CloudSat is a 94 GHz (W-band) cloud-profiling radar (CPR) in a 700 km high, sun-synchronous, near-polar orbit (Stephens et al., Citation2002). The main feature of the CPR instrument is its ability to measure cloud and precipitation features along its orbital track at a horizontal resolution of approximately 1.4 km (cross-track) × 2.5 km (along-track) with a 240 m range resolution, providing a view of the internal cloud and/or hydrometeor layers within surface storm systems. Each vertical profile from CloudSat comprises 125 range bins up to a height of 30 km. Radar sensitivity within the lowest 2–3 range bins (480–720 m above ground) was greatly diminished because of surface reflection and/or clutter over most of the terrain such that the data near the surface were compromised. As such, reflectivity data within 720 m above ground level (AGL) were ignored.
One of the main limiting factors for the CPR instrument is retrieving data on thin cirrus cloud since the minimum reflectivity threshold is approximately −28 dBZ with a calibration accuracy of 2 dBZ. Because of this restriction, complete cloud coverage as interpreted by the CPR is underestimated by approximately 5%–10% for radiatively significant ice clouds and 20% for liquid clouds (Stephens et al., Citation2002). Furthermore, the W-band CPR is susceptible to attenuation from large liquid cloud particles (multiple scattering) and high precipitation rates (L'Ecuyer and Stephens, Citation2002), resulting in reflectivity values that seldom exceed 20 dBZ. In the presence of liquid water, losses greater than 10 dBZ km−1 are possible. This factor is not corrected in this study. Additional technical details of the CPR are described by Im et al. (Citation2005) and Mace et al. (Citation2007).
The data obtained by CloudSat were analyzed along with data from the Moderate Resolution Imaging Spectroradiometer (MODIS) instrument onboard the Aqua satellite. MODIS acquires data in 36 spectral bands (0.4–14 μm) at varying horizontal resolutions (250 m, 500 m and 1000 m). The MODIS instrument collects data along a wide swath of the earth's surface (2330 km wide) as opposed to the thin swath observed by CloudSat (1.1 km wide). However, to relate the CloudSat vertical profiles to the complementary MODIS data, only the data that fell along the CloudSat orbital segment were extracted and used in the analysis. Because the CloudSat vertical profile has a smaller resolution than Aqua, the data were adjusted accordingly.
Other datasets from various sources that have been used in this study will be described or referenced when they first appear.
c Summary of Significant Events
The 61-day observational period was characterized by a number of storm events. On examining the CloudSat radar profiles, four stand out in terms of extensive horizontal and vertical size and intensity. These occurred on 3 October (Event 1), 5 November (Event 2), 8 November (Event 3) and 17 November (Event 4).
It should be noted that the macrophysical data for three of these events (Events 1, 3 and 4) were included in the calculations for the mean values discussed below because they fell within the boundaries defined in . The data for Event 2 were not considered in those calculations because the storm system (the remnants of Hurricane Noel) was outside the region of interest. However, it represented an ideal opportunity to analyze an extratropical storm as it transitioned in the Arctic region.
3 Cloud and precipitation observations
a Cloud and Precipitation Analysis
and and summarize a number of cloud characteristics in association with precipitating and non-precipitating profiles. The following subsections discuss this as well as other information.
Table 1. Frequency of occurrence of cloud features identified over open ocean and/or sea ice. The numbers in parentheses are the percentage of profiles over open ocean and/or sea ice relative to the total number of profiles.
Fig. 2 Frequency distribution of vertical profiles over the southern Baffin Island region that contained precipitation near the surface. Each colour-coded grid box represents the percentage of the total number of cloud profiles that contained precipitation as described in Section 3a. See for names of geographic features.
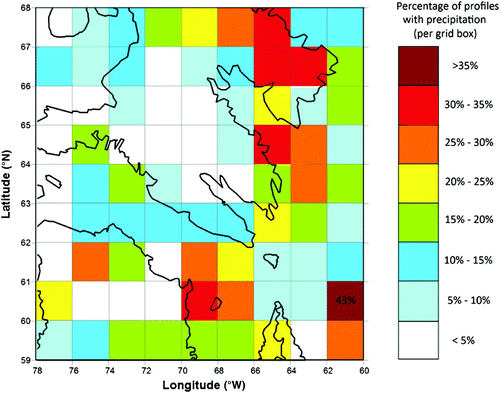
Fig. 3 Summary of cloud characteristic vertical profiles between 1 October and 30 November 2007 for (a) cloud top height, (b) cloud top temperature, (c) cloud thickness, and (d) number of cloud layers. The solid lines represent all clouds and the dashed lines represent precipitating clouds.
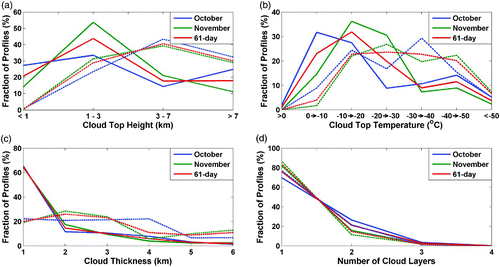
1 Cloud and Precipitation Occurrence
A total of 62 of the 91 CloudSat segments had orbital cloud coverage greater than 80%. The orbital cloud coverage is defined as the percentage of an orbital segment during which clouds were detected. For example, an orbital cloud coverage of 10% indicates that 1 out of 10 vertical profiles for a particular orbit contained clouds. None of the orbital segments were completely devoid of clouds because the lowest orbital cloud coverage was 29%, and total cloud coverage was observed in 18 of the CloudSat orbital segments.
Overall, clouds were observed 86% of the time over southern Baffin Island and the surrounding area during the observation period. The high occurrence of clouds over the region indicates that the atmosphere was often either saturated or near-saturated, yielding clouds at various levels of the atmosphere.
The majority of the clouds (57%) were over the open ocean and/or sea ice which surround the southern Baffin Island landmass (). This frequency is essentially the same value as the percentage of open ocean and/or sea ice in the domain (58%). As well, 20% of the profiles containing clouds were observed over terrain with an elevation greater than 200 m. This again is essentially the same as the percentage of this terrain in the domain (21%). This indicates that there was no overall preference for clouds to occur over land, over the open ocean and/or sea ice or over terrain above 200 m.
Precipitation was much less common. It was only observed in 13% of the vertical profiles containing clouds. As well, there was a strong tendency for precipitation to occur in November with 68% of these vertical profiles occurring in this month.
Clouds containing precipitation mainly occurred over the open ocean and/or sea ice (60%, ) although this percentage was somewhat higher (66%) during November. The overall percentage closely matches the percentage of open ocean and/or sea ice in the domain (58%) and this indicates that there was no overall preference for precipitation to occur over either land, open ocean or sea ice.
Vertical profiles containing precipitation over land varied with terrain. Overall, 22% occurred over terrain higher than 200 m which is similar to the percentage of cloud occurrence over the same terrain. However, 9% occurred over regions with a peak greater than 1 km; this is much higher than the percentage of this terrain within the observation region (1.3%) and indicates there was a preference for precipitation to occur over higher terrain.
depicts the spatial distribution of precipitation inferred from data collected by CloudSat. Most of the precipitation was localized in the Cumberland Peninsula where precipitation occurrence with cloud was more than 20%. This is expected because the existence of the Penny Ice Cap over this area is indicative of a long-term favoured region for precipitation. Other regions of enhanced precipitation generation were found along the coastline of Baffin Island and northern Quebec, the Hudson Strait–Ungava Bay region, and the North Atlantic and Davis Strait. These observations imply that a substantial amount of the precipitation was affected by topography. There was a lack of precipitation over other regions. Over the interior of Baffin Island (and to some extent the interior of northern Quebec), precipitation was relatively scarce with the frequency of occurrence over these regions being less than 5%.
2 Cloud Top Characteristics
The mean cloud top height for profiles with clouds was 3.4 km over the 61-day observation period but there was great variability. Cloud top height refers to the height of the uppermost cloud layer. In October, high (>7 km ASL), mid-level (3–7 km ASL) and low (1–3 km ASL) cloud tops occurred 25%, 14% and 34% of the time, respectively (a). From October to November, the frequency of high-level cloud tops decreased by 54%; however, mid-level and low-level cloud tops increased in frequency by 51% and 65%, respectively. The decrease in frequency of high level cloud top heights may partly be a result of the lowering of the tropopause as the colder winter months move in.
In the presence of precipitation, cloud tops were, on average, higher with the mean cloud top height being 4.2 km (a). This is 24% higher than the mean cloud height for non-precipitating clouds. In the profiles containing precipitating clouds, most clouds (41%) were mid-level (3–7 km ASL). High (>7 km) and low (1–3 km) cloud top heights represented the remaining 30% and 29%, respectively.
The 61-day mean cloud top temperature was −20°C but there were many variations. The mean cloud top temperature for October was −19°C, and the majority of clouds tops were between −20°C and 0°C (59% of vertical profiles) as shown in b. In contrast, the mean cloud top temperature for November decreased to −21°C, and 67% of these profiles had a cloud top temperature between −30°C and −10°C. The decrease in cloud top temperature values is expected because temperatures cool in the autumn. Additionally, the frequency of extremely cold cloud tops (cloud top temperature <−50°C) decreased from October to November. This is consistent with the previously mentioned reduction in the occurrence of high cloud tops in the month of November.
The decrease in mean cloud top temperatures from October to November, along with the decreased frequency in high cloud tops, may be a result of the region undergoing a general cooling as winter conditions take hold. However, the freezing of the surrounding water as well as colder surface temperatures may also have contributed to this decrease in high cloud top occurrences. Colder air can hold less water, and the freezing of the surrounding water would have allowed less moisture to enter the atmosphere.
The mean cloud top temperature with precipitation was −23°C, 3°C cooler than for clouds overall. As well, there were relatively more cloud tops below −30°C in the presence of precipitation. There was a relatively even distribution of vertical profiles with cloud top temperatures between −50°C and −10°C. However, cloud top temperature values above −20°C decreased in frequency from October to November.
3 Cloud Thickness and Layering
The mean cloud thickness was 2.3 km, and it did not change significantly during the observation period although a number of orbital passes did exhibit relatively thick clouds. Cloud thickness refers to the cloud depth from the base of the bottom layer to the top of the uppermost cloud layer. Of the vertical profiles with clouds, 21% had a mean cloud thickness greater than 4 km (e.g., c) with some orbital paths containing clouds more than 7 km thick.
The mean cloud thickness with precipitation was 3.4 km, a 50% increase over non-precipitating clouds. The frequency of precipitation associated clouds with thicknesses greater than 4 km increased from 23% with no precipitation to 57%.
Single-layer clouds were commonly observed in the profiles, but clouds with multiple layers were also present. Single-layer clouds were observed in 76% of the cloud profiles with an increase from October (70%) to November (82%) as shown in d. Multiple cloud layers were observed 24% of the time with up to four layers, but these occurred in less than 1% of the profiles. Two-layer clouds were more commonly observed in October (26% of the cloud profiles) than in November (16%). Overall, the mean number of layers seen during the observational period was 1.26.
Profiles containing precipitation exhibited somewhat similar characteristics to those associated with the overall cloud field. Single-layer clouds were the dominant layering type, found in 84% of the vertical profiles. This proportion is higher than that for the overall cloud field, which is to be expected because deeper clouds would allow precipitation production to occur. Multi-layering was also identified with precipitation; the maximum number of layers was 3 and the mean number of layers was 1.2.
b Analysis of Reflectivity Fields
By visually inspecting the reflectivity images, a number of features were identified. Maximum reflectivity values ranged between a minimum of −24 dBZ on 11 October at 0645 utc to a maximum of 20 dBZ on several occasions (not shown). As discussed in Section 2, values near the surface were not considered because these were probably associated with ground contamination. As well, the structure of the reflectivity information was, at times, consistent with bright band features, sublimation and/or evaporation, and/or advection, isolated and/or banded precipitation, and orographic precipitation. A definition of each term is given in .
Table 2. Definitions for cloud and precipitation features discussed in this study.
Bright band-like features were observed the fewest number of times. They were detected in 3.3% of the orbital passes. When present, they were associated with thick, high cloud-top storm systems (a). During each instance, it was determined that the atmospheric temperature was approximately 0°C at the height of the apparent melting layer, based on information from the European Centre for Medium-range Weather Forecasts (ECMWF).
Fig. 4 Examples of (a) bright band-like features (1715 utc, 3 October), (b) regions of sublimation and/or evaporation, and/or advection (0740 utc, 26 October), (c) isolated and/or banded precipitation (0650 utc, 19 November), and (d) orographic precipitation (0710 utc, 8 November).
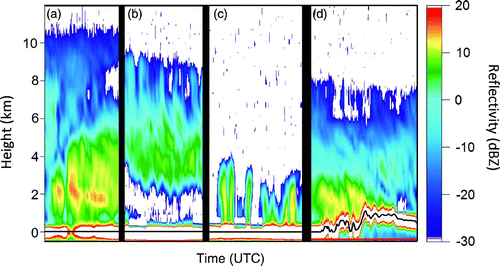
Regions of sublimation and/or evaporation, and/or advection (b) were observed in 13% of the orbital passes (). This feature was likely the result of unsaturated air and/or wind fields leading, respectively, to sublimation and/or evaporation, and/or to advection of the precipitation sideways before it could reach the surface in the vertical column. Areas of sublimation and/or evaporation, and/or advection were more prevalent in October with 9 of the 12 occurrences seen that month.
Table 3. Frequency distribution of various cloud and precipitation features observed during the period 1 October–30 November 2007. In total, there were 91 CloudSat observations. The numbers in parentheses are the percentages relative to the total number of CloudSat observations.
Regions of isolated and/or banded precipitation (c) were the most frequently observed feature overall as well as occurring more in November (). However, these all occurred over the ocean. The higher occurrence of this organizational type of precipitation in November is likely due to the increased temperature contrast between the boundary layer and the surface of the surrounding water, leading to a situation similar to that of lake-effect snow.
Orographic precipitation was evident in 9% of the passes (d, ). Given the highly varied terrain of southern Baffin Island and the surrounding area, the relatively low occurrence of orographic precipitation is possibly caused by air masses being too dry on the windward side of the mountain ridges. Another explanation could be weak winds or winds directed parallel to the mountain ridges. Either of these circumstances would result in reduced cloud generation and precipitation.
When orographic and stratiform precipitation were observed, radar reflectivity values near the mountain ridges were higher compared to those occurring within the same radar image but over a nearby flat region. In this study, stratiform precipitation was considered to be any radar reflectivity structure that was essentially unchanged over an extended horizontal distance. In most cases, the maximum reflectivity value for orographic precipitation was between 5 and 10 dBZ higher than over a flat region. In addition, the varying topography can cause the precipitation to be scattered and/or banded, within larger, more complex storm systems as noted in two of the four major events analyzed in the following section.
4 Case studies
During the observation period, four major storm events passed over southern Baffin Island. These events are listed in Section 2c. Each was characterized by high clouds, cold cloud tops and a number of other critical cloud and precipitation features ().
Table 4. Summary of cloud macrophysical properties for each event as observed along the orbital path of CloudSat. The observational mean (1 October–30 November 2007) is also given for comparison purposes.
a Synoptic Situation
shows a surface analysis for each event that is as close in time as possible to the orbital pass. Event 1, Event 2 (the remnants of Hurricane Noel) and Event 4 were each associated with a mid-latitude cyclone. Event 3 was associated with a non-frontal low pressure system over Hudson Strait. The central pressure for each event at the time of observation was 984 hPa, 968 hPa, 991 hPa and 968 hPa (Events 1 to 4, respectively).
Fig. 5 Synoptic surface analysis of (a) Event 1 (1800 utc, 3 October 2007), (b) Event 2 (0600 utc, 5 November 2007), (c) Event 3 (0600 utc, 8 November 2007), and (d) Event 4 (1800 utc, 17 November 2007). Synoptic analysis information provided by Environment Canada.
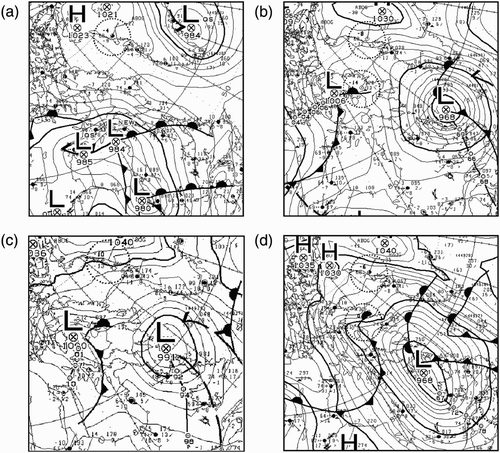
The pressure gradient fields varied over Baffin Island within these events. Because of the relative position of the low pressure system, the pressure gradient for Event 1 originated from the southeast and was parallel to the two mountain ridges continuing up the Frobisher valley along a southeast–northwest trajectory. In this case, the centre of the low was situated south of Iqaluit. For the other three events, the centre of the low was situated south or southeast of Iqaluit, allowing the pressure gradients to originate from the northeast over Iqaluit and the surrounding area. For these three events, the pressure gradients were generally perpendicular to the Meta Incognita Peninsula and Hall Peninsula mountain ridges surrounding Iqaluit.
Pressure gradient strength also varied. Only Event 4 exhibited a strong pressure gradient force as indicated by the tightly packed isobars surrounding the low pressure centre. The other three low pressure systems exhibited weaker pressure gradients and likely weaker large-scale surface winds.
b Cloud and Precipitation Characteristics
The four events exhibited different cloud and precipitation characteristics. illustrate the reflectivity field and cloud top temperature information for each of the events. Each radar image is approximately 3 minutes in duration and 1000 km in length.
1 Event 1
Event 1 was a large storm system with little precipitation reaching the surface (a). The mean cloud top temperature for the storm was −39°C with a range between −50° and −3°C. The cloud top temperature field depicts the storm as a large cloud shield over most of northern Quebec and southern Baffin Island. Along the orbital path, it was characterized by low cloud top temperatures although with a wide range of values.
Fig. 6 (a) MODIS image of cloud top temperature with the CloudSat orbital track indicated by the black line and (b) the CloudSat radar reflectivity of Event 1 for 3 October 2007. The MODIS image was obtained at approximately 1720 utc.
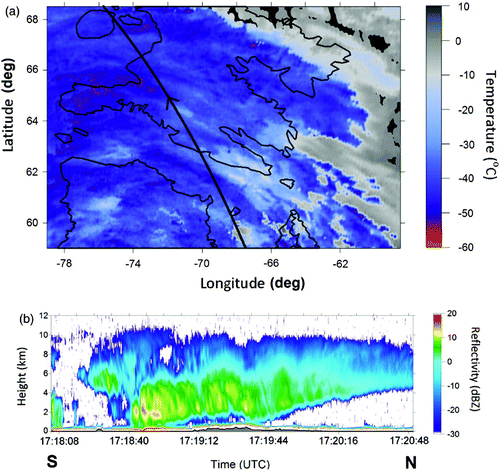
The mean cloud top height was 9.3 km and 88% of the cloud tops were higher than 7 km ASL. The storm also contained thick clouds with a mean cloud thickness of 7.4 km. Internally, the storm exhibited cloud layering with the mean number of layers along its orbital path being 1.21, which was the highest mean of the four events. However, the mean number of layers for Event 1 was still lower than the 61-day mean.
Event 1 illustrated several precipitation features. First, sublimation and/or evaporation occurred, especially after 1719:12 utc (b). Within this region, reflectivity values above 0 dBZ were identified aloft within a 4 km thick layer (2–6 km ASL). Underneath this layer, no detectable reflectivity values were present, suggesting a dry airmass. The structure of the reflectivity information within this part of the storm is the shape one would expect from an approaching warm front, which would suggest cold air advection ahead of the front at the surface.
The second feature is the presence of a bright band-like feature over Davis Strait at 1718:50 utc. Reflectivity values were as high as 16 dBZ and values above 0 dBZ were identified aloft where an inflection was located 2 km ASL. Below the radar inflection, reflectivity intensities decreased with decreasing height although they remained above 0 dBZ, indicating the possibility of precipitation reaching the surface within the region. This is unlike the region of sublimation and/or evaporation identified within the same figure in which there were no detectable reflectivity values near the surface. As such, this storm system represents one in which both a melting layer and a sublimation and/or evaporation region can be inferred.
Despite the fact that the storm spanned a large horizontal distance and contained multiple precipitation features aloft, very little precipitation was detected near the surface. This is especially true for the part of the storm discussed above (1719:20–1720:48 utc). In total, only 12% of the vertical profiles that contained clouds had any precipitation near the surface, which is low considering the intensity and extent of the storm.
2 Event 2
Event 2, which represented the remnants of Hurricane Noel, was observed as a large open wave storm system (a). There was widespread precipitation across the entire orbital path (b). Additionally, it was characterized by relatively low cloud top temperatures with some values reaching −45°C. The mean cloud top temperature for the storm was −37°C, which is the warmest mean cloud top temperature of the four events but still significantly colder than the 61-day mean.
Fig. 7 (a) MODIS image of cloud top temperature with the CloudSat orbital track indicated by the black line and (b) the CloudSat radar reflectivity of Event 2 for 5 November 2007. The MODIS image was obtained at approximately 0645 utc.
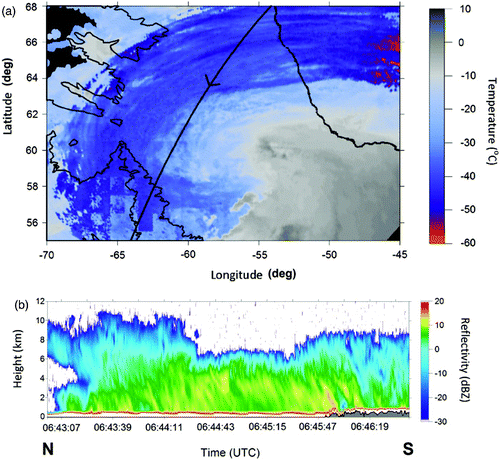
Of all the events, Event 2 had the most uniform cloud field along the orbital path. The mean cloud top height was 8.3 km (1 km lower than that for Event 1) with 73% of the cloud tops above 7 km ASL. Also, the mean cloud thickness along the length of the orbit was 7.2 km which was 0.2 km lower than that of Event 1.
The most significant difference between Events 1 and 2 was the mean number of layers. b shows the remnants of the hurricane to be largely a single-layer cloud. This storm averaged only 1.12 layers, and this was the lowest value of the four events.
Stratiform precipitation was mainly observed with a significant portion of the values above 0 dBZ values situated over Davis Strait. Overall, 93% of all the cloud profiles for the storm contained precipitation, representing the highest proportion of the four events.
One defining feature of the inferred precipitation was that the precipitation was tilted with respect to the surface. The precipitation exhibited a stratiform structure and had a tilt along a north–south axis with respect to the surface which is likely an indication of vertical wind shear and the movement of Hurricane Noel. Even though reflectivity values were nearly constant over the ocean, the precipitation intensity for the portion of the storm over northern Quebec and Labrador (landmass towards the right end of b) had diminished and this also resulted in less organized precipitation. This observation suggests that the precipitation was diminished, at least in part, because the local source of moisture and/or heat was cut-off over the land.
3 Event 3
Event 3 exhibited a cloud field similar to but smaller than Event 2. The mean cloud top temperature for the storm was −43°C, and it had the most values below −40°C, with some reaching −65°C.
It was the smallest storm of the four events in terms of depth and cloud top height. Its mean cloud top height was 7.3 km ASL, and 46% of its cloud tops were above 7 km ASL. The mean cloud thickness of 5.5 km was well below that of the other events, yet it had the highest mean number of cloud layers at 1.24 which was the closest to the 61-day mean. Compared to the 61-day mean values from , it was still a thick-layered system with high cloud tops.
Across the orbital segment, precipitation was scattered with three cells identifiable within b. Overall, 41% of the vertical profiles contained precipitation. This was significantly less than for Event 2 although it was still higher than Event 1 where sublimation and/or evaporation were prevalent.
Fig. 8 (a) MODIS image of cloud top temperature with the CloudSat orbital track indicated by the black line and (b) the CloudSat radar reflectivity of Event 3 for 8 November 2007. The MODIS image was obtained at approximately 0715 utc.
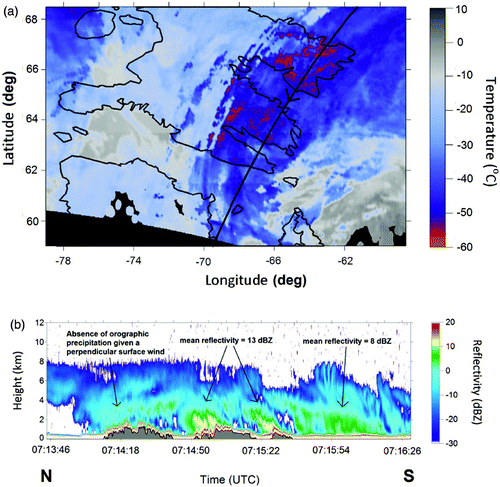
Unlike the other three events, most of the higher reflectivity values (>0 dBZ) were located near two of the mountain ridges of southern Baffin Island —Meta Incognita Peninsula and Hall Peninsula. On the windward side of these mountain ridges, reflectivity values were relatively high, reaching values of 16 dBZ. Throughout the remainder of the event, values above 0 dBZ occurred with a low frequency (lowest of the four events). The elevated reflectivity values in the vicinity of Meta Incognita Peninsula and Hall Peninsula are an indication of the influence that mountain ridges have on precipitation as further discussed in Section 5c.
4 Event 4
Event 4 was the largest of the four events () with significant precipitation reaching the surface. The mean cloud top temperature for the storm was −48°C, which is the lowest of the four events. The cloud top temperature field (a) shows the storm moving off the coast of eastern Labrador into southern Baffin Island. Event 4 was characterized by low cloud top temperatures with most of the storm exhibiting cloud top temperatures below −40°C and some regions with temperatures as low as −65°C.
Fig. 9 (a) MODIS image of cloud top temperature with the CloudSat orbital track indicated by the black line and (b) the CloudSat radar reflectivity of Event 4 for 17 November 2007. The MODIS image corresponds to approximately 1650 utc. Figure adapted from Hanesiak et al. (Citation2010) by permission of the American Meteorological Society.
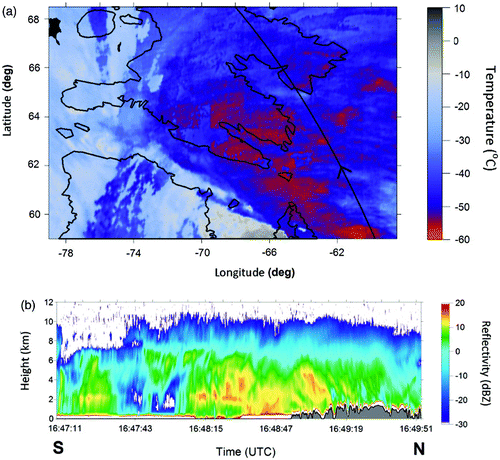
Event 4 had the highest mean cloud top height (10.2 km) of the four events, and 96% of the cloud tops were above 7 km ASL. With a mean cloud thickness of 9.3 km, Event 4 was, on average, the thickest of the events and up to 450% greater than the 61-day mean value. The mean number of layers was 1.18, which was not the closest to the observation period mean.
There were several precipitation regions along the orbital segment. The primary precipitation region was identified off the eastern coast of Cumberland Peninsula with reflectivity values frequently surpassing 10 dBZ. In total, precipitation was identified in 76% of the vertical profiles which contained clouds. Most of these were situated over the North Atlantic and Davis Strait.
The radar image for Event 4 depicts sublimation and/or evaporation regions as well as bright band-like features which is similar to that found in Event 1. In b, the radar image depicts two distinct bright band-like features separated by approximately 500 km with a drier region between the two. This drier region was also where sublimation and/or evaporation occurred. Within this region, reflectivity values near the surface decreased to below the minimum detectable threshold with higher values (>0 dBZ) situated aloft.
The radar image also shows that the heaviest precipitation region was within 300 km of the eastern coast of Baffin Island. The large mountain range on the right-hand side of b is Cumberland Peninsula. The surface water off the coast may have had an impact on the intensity of precipitation in that area. In addition, there is a suggestion of orographic stratiform precipitation over the peninsula. However, the reflectivity field for each of these features was not as distinct as in Events 2 and 3.
5 STAR climatology and case study discussion
a Case Study—STAR Comparison
The four events represent the most extreme situations observed for the months of October and November 2007. Excluding the data from Event 2 (which is explained in Section 2c), the combined results from Events 1, 3 and 4 contributed significantly to some of the observations over the 61-day period, especially with regard to cloud top properties. A summary of the observations is presented in .
Table 5. Summary of the number of vertical profiles observed for several cloud characteristics for both the 61-day observational period and Events 1, 3 and 4 combined. The numbers in parentheses are the percentages of the profiles relative to the 61-day observations.
Cloud top heights in excess of 7 km were observed in 18% of all the cloud profiles within the observation period. Overall, 18% of the high cloud observations occurred during Events 1, 3 and 4. This percentage is significantly higher for the month of November when high cloud tops for Event 3 and Event 4 together accounted for 35% of the high cloud top profiles for the month.
In addition to high cloud top heights, 15% of the cloud profiles exhibited cloud top temperatures below −40°C. The majority of these occurred in the month of October (b) and a significant portion (22%) were accounted for in Events 1, 3 and 4 combined. This proportion is significant considering that, out of the 91 orbital segments over the region, only three (Events 1, 3 and 4) were linked with significant events.
Only 8 of the 91 orbits (which include Events 1, 3 and 4) contained a mean orbital thickness greater than 5 km. Of these thick clouds, 22% occurred in Events 1, 3 and 4 (). This proportion is even higher when analyzing cloud thicknesses in excess of 10 km (50%, ).
Table 6. Number of cloud profiles of various thicknesses over the 61-day observation period and from Events 1, 3 and 4 combined. The numbers in parentheses are the percentages of profiles from Events 1, 3 and 4 relative to the 61-day totals.
Collectively, it is apparent that these major storm systems represented a significant contribution to high and cold cloud top mean features over the entire study period.
Even though some of the cloud characteristics for Events 1, 3 and 4 contributed greatly to some of the mean features for the 61-day observation period, there was at least one parameter in which they did not. For the overall observation period, single-layer clouds were observed 76% of the time when clouds were observed (d). The three major events only accounted for a combined 5% of these observations (). The percentage is even lower for multi-layer clouds (3.3%). Given that Events 1, 3 and 4 constitute 3.3% of the 91 orbits, it is clear that these large storm systems did not contribute dramatically to the overall occurrence of single- or multi-layer clouds.
b Vertical Radar Reflectivity Profile Analysis
The precipitation and cloud field for each event can be examined by analyzing the frequency distribution of reflectivity values as a function of height. The diagrams presented in are normalized Contoured Frequency Altitude Diagrams (CFAD), a technique used in Stewart et al. (Citation2004) to analyze radar information and examine the frequency of radar reflectivity echoes that occurred at different heights. The CFAD images illustrate the frequency of occurrence of points in a scatter diagram-type format.
Fig. 10 Normalized CFAD percentages for (a) Event 1, (b) Event 2, (c) Event 3, and (d) Event 4. The colour scale refers to the normalized frequency occurrence of reflectivity values observed with height.
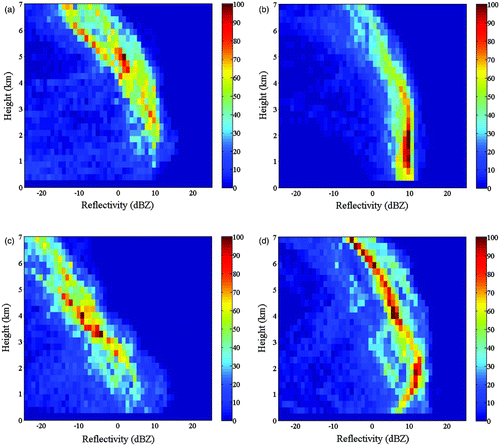
Event 1 (a) was characterized with continuous growth (deposition) aloft, whereas sublimation and/or evaporation were identifiable near the surface. Between 2 km and 5 km ASL, a large proportion of the reflectivity signals were observed between 0 and 10 dBZ, indicating weak to moderate precipitation. Above this, reflectivity values between −10 and 0 dBZ were common, indicating the presence of continuous growth (deposition) with decreasing height. For the lowest 2 km, the frequency of heightened reflectivity values decreased significantly with decreasing height, which is characteristic of the loss of hydrometeors as they fall through a dry airmass (associated with the large front-like structure discussed in Section 4b1 and shown in b). As such, the overall size of hydrometeors aloft diminished towards the surface, greatly reducing the return signal between 0 dBZ and 10 dBZ. The maximum reflectivity value observed was 16 dBZ, although most of the elevated values were no higher than 10 dBZ.
Unlike Event 1, the reflectivity field for Event 2 was more uniform and consistent where precipitation was widespread across Davis Strait. The CFAD diagram depicts a significantly high frequency of elevated reflectivity values (>0 dBZ) between the surface and 6 km ASL (b). High reflectivity values (9 dBZ) also occurred between 500 m and 3 km ASL, suggesting that hydrometeor size distributions were nearly constant with respect to height along the orbital segment. Higher reflectivity values were not commonly observed, although a maximum of 14 dBZ was recorded.
Event 3 (c) had the lowest occurrence of high reflectivity values compared to the other three events with most of the values above 0 dBZ limited to within 3 km ASL. The majority of these higher reflectivity values occurred in only three light-precipitation regions, as observed in b. The remainder of the orbital segments contained comparatively low values, resulting in the CFAD having a low occurrence of higher reflectivity values near the surface as well as aloft. The infrequent higher values near the surface are likely an indication of sublimation/evaporation, and this is observable in the radar image from b over Cumberland Peninsula and off the coast of Meta Incognita Peninsula. Deposition and/or aggregation were also apparent aloft (between 3 and 5 km ASL) given the frequency of reflectivity values between −10 and 0 dBZ is relatively high at this altitude.
Event 4 was the most complex of the events since multiple features were observed. Between 1.5 km and 2.5 km, there was a high occurrence of reflectivity values within the 10–14 dBZ range, which is indicative of a reflectivity inflection (two instances of an inflection were noted in b). Below the inflection, as heights decrease, the frequency of these higher reflectivity values also decreased (d). Simultaneously, the frequency of reflectivity values between 5 and 10 dBZ increased. Such a reflectivity signal is characteristic of a melting region though it may also be indicative of attenuation below 2 km ASL.
Throughout the remainder of the CFAD diagram for Event 4, values above 0 dBZ were commonly observed as high as 6 km ASL, which is the highest of the four events. Above this level, aggregation and/or deposition probably occurred because reflectivity values between −10 and 0 dBZ occurred frequently. Most of the aggregation and/or deposition was localized between 6 and 8 km altitude.
When examining sublimation and/or evaporation, the CFAD diagram for Event 4 does not show a signal as distinct as that observed for Events 1 and 3. For Event 4, d shows a gradual decrease near the surface which may indicate sublimation and/or evaporation. However, because of the complexity of the storm itself, it is mainly through analyzing the actual radar image (b) that the regions of sublimation and/or evaporation can be identified. This is most notable between 1647:45 and 1648:00 utc.
c Topographical Influence on Precipitation over Baffin Island
During the observation period, orographic precipitation was generally observed over relatively low-lying terrain, with mountain peaks between 565 and 1329 m in height. Event 3 represented an ideal case as both mountain ridges (Meta Incognita Peninsula and Hall Peninsula) exhibited orographic precipitation on the windward side. Meta Incognita Peninsula and Hall Peninsula reach 800 and 1200 m altitude, respectively. Within these mountain ridges, reflectivity values for orographic precipitation had a mean value of 13 dBZ, which was 5 dBZ higher than the isolated precipitation region located over Hudson Strait (mean of 8 dBZ; b). Orographic precipitation can also be observed in the radar images for Event 4 (b) although the feature was not as pronounced.
In Event 3, the CloudSat orbital track (a) and the large-scale surface wind regime surrounding Meta Incognita Peninsula and Hall Peninsula (as inferred in the synoptic map of c) were both oriented northeast–southwest. This allowed for the CloudSat radar to observe significant orographic precipitation in the vicinity of these two mountain ridges. However, for Event 4, the CloudSat orbital path was not as well oriented with respect to the surface wind regime. That is, winds were east–west whereas the CloudSat orbit was southeast–northwest. Regardless, some orographic enhancement for Event 4 was evident.
Orographic precipitation in Event 3 was not observed on the windward side of Cumberland Peninsula but was observed on the windward side of Meta Incognita Peninsula and Hall Peninsula (b). As evident on the synoptic map for the event (c), the flow was nearly perpendicular to Hall Peninsula and Meta Incognita Peninsula. However, the large-scale surface wind regime was easterly in the vicinity of Cumberland Peninsula (the peninsula itself having a northwest–southeast orientation). This implies that a significant component of the surface wind was parallel to the orientation of the mountain ridge and this would have reduced the upslope flow. In addition, the pressure gradient force was weak over Cumberland Peninsula, which would have meant low wind speeds.
d Comparison with Other Studies
Since its launch in April 2006, many research efforts have been undertaken utilizing CloudSat data as part of their analysis. Many of the articles have focused on tropical cloud formation and microphysical algorithm verification. However, some have analyzed global cloud distributions. These articles include, but are not limited to, Sassen et al. (Citation2008), Sassen and Wang (Citation2008) and Mace et al. (Citation2009). The current study complements these with a more in-depth investigation over one particular region, the eastern Canadian Arctic.
In addition, there are some articles not related to CloudSat that have focused on clouds in the Canadian Arctic. These include a study using operational information (Stewart and Burford, Citation2002) and two that were based on a one-month special observational study over the Mackenzie River basin (Hudak et al., Citation2004; Stewart et al., Citation2004). In the latter two studies, clouds were commonly observed (77% of the time using their method) as was the case in this study (86%).
There are a number of other similarities between the results of the Hudak et al. (Citation2004) and Stewart et al. (Citation2004) Mackenzie River basin studies and those from this study. In these earlier studies, there was a preference for cloud top heights to be either high (>7 km) or low (<4 km) with fewer observations of mid-level clouds, which is similar to the findings of this study. Also, there were few instances of cloud top heights above 9 km ASL; however, in this study one event in particular (Event 4) had cloud tops observed above 10 km ASL for the majority of the orbital path (). A similar inference can be made for the cloud top temperatures. The Mackenzie River basin observations (Hudak et al., Citation2004; Stewart et al., Citation2004) and the case studies analyzed in this study show similar cloud top temperature ranges although some differences exist. None of the Mackenzie River basin observations exhibited a cloud top temperature below −55°C, but both Events 1 and 4 in this study had cloud top temperatures as low as −65°C. Despite differences in the observations of these extreme values, the overall cloud field for both the western and eastern Canadian Arctic exhibited some similar cloud properties.
6 Summary and concluding remarks
An analysis of data from a two-month analysis of clouds and precipitation in autumn 2007 over the southern Baffin Island region and the surrounding ocean has been carried out. Data used in the study were mainly from the CloudSat and Aqua satellites. This study is the first to utilize a satellite-based radar to observe clouds and precipitation over this region. A number of key observations and scientific findings have been made:
• | Clouds were very common; they occurred in 86% of the vertical radar profiles. Most were low (1–3 km, 55%) although high clouds were also common (>7 km, 23%), and there was a general lack of mid-level clouds. Cloud thicknesses were as great as 10 km. Clouds were generally single layer (76%) but multi-layering was common with up to four layers. | ||||
• | Precipitation only occurred in 13% of the cloud profiles. When precipitation occurred, cloud tops were higher (mean value 30% greater), colder (3°C lower) and thicker (50% greater) than non-precipitating clouds. | ||||
• | Several cloud and precipitation features were documented. These included sublimation and/or evaporation, and/or advection of precipitation aloft, bright band-like features suggesting melting aloft, as well as isolated and/or banded precipitation preferentially occurring over the open ocean and/or sea ice. | ||||
• | Over Baffin Island, there was a preference for precipitation to occur over the Cumberland Peninsula with its large ice caps. In contrast, the frequency of precipitation was low over the flat interior of the island. | ||||
• | Orographic precipitation was sometimes evident on the windward side of both Meta Incognita Peninsula and Hall Peninsula. In these situations, the surface wind regime was oriented nearly perpendicular to the mountain ridges. | ||||
• | Three major storm events (Events 1, 3 and 4) passed over the region during the observational period. Cloud features associated with these three events contributed significantly to the 61-day mean values of several cloud top properties. These represented less than 5% of all profiles, but they represented 18% of the profiles with high cloud tops (>7 km), 22% of thick clouds (>5 km thickness) and 22% of cold cloud tops (<−40°C). Other parameters, such as cloud layering, were not substantially affected by the presence of these three events. It is clear that events that produce significant amounts of precipitation can vary considerably from the mean observations. | ||||
• | The remnant of Hurricane Noel (Event 2) was characterized by deep, generally single-layer clouds compared with other events. | ||||
• | Precipitation processes differed somewhat among the four observed events. In some, precipitation grew almost continuously as it fell, whereas, in others, it was reduced as it fell as the result of sublimation and/or evaporation. |
The features of clouds and precipitation observed in this study were, to some degree, similar to those observed over the Mackenzie River basin. Cloud properties such as mean cloud top temperature, the relative lack of mid-level clouds, and cloud layering were similar, although some extreme values (such as cloud top temperatures less than −55°C) were only observed in this Baffin Island region study.
It is recognized that this study only focused on a two-month period so generalizations are not possible. As well, the study is restricted to a certain extent by temporal, spatial, attenuation and threshold sampling limitations. Nonetheless, it represents an important benchmark for the evaluation and understanding of clouds and precipitation, and future research can expand on the analyses presented.
In summary, this study has provided the first in-depth analysis of clouds and precipitation over Baffin Island and nearby areas. Clouds with varying features occurred frequently, but precipitation did not, and it was sometimes affected by topographic features. The common but varying cloud and precipitation conditions represent a significant challenge to improving the prediction of Arctic storms and to understanding their role in the region's climate.
Acknowledgements
The authors would like to thank the Canadian Foundation for Climate and Atmospheric Sciences (CFCAS) for its support through the Storm Studies in the Arctic (STAR) project.
MODIS data are distributed by the Land Processes Distributed Active Archive Center (LP DAAC), located at the U.S. Geological Survey (USGS) Earth Resources Observation and Science (EROS) Center (http://lpdaac.usgs.gov). CloudSat data are distributed by the CloudSat Data Processing Center (CDPC), located at the Cooperative Institute for Research in the Atmosphere in Fort Collins, Colorado.
ECMWF data used in this study were obtained from the ECMWF Data Server.
References
- Asuma , Y. , Iwata , S. , Kikuchi , K. , Moore , G. W.K. , Kimura , R. and Tsuboki , K. 1998 . Precipitation features observed by Doppler radar at Tuktoyaktuk, Northwest Territories, Canada, during the Beaufort and Arctic Storms Experiment . Mon. Weather Rev , 126 : 2384 – 2405 .
- Barker , H. W. , Korolev , A. V. , Hudak , D. R. , Strapp , J. W. , Strawbridge , K. B. and Wolde , M. 2008 . A comparison between CloudSat and aircraft data for a multilayer, mixed phase cloud system during the Canadian CloudSat-CALIPSO validation project . J. Geophys. Res. , 113 : D00A16 doi:10.1029/2008JD009971
- Beesley , J. A. and Moritz , R. E. 1999 . Toward an explanation of the annual cycle of cloudiness over the Arctic Ocean . J. Clim. , 12 : 395 – 415 .
- Curry , J. A. , Rossow , W. B. , Randall , D. A. and Schramm , J. L. 1996 . Overview of Arctic cloud and radiation characteristics . J. Clim. , 9 : 1731 – 1764 .
- Curry , J. A. , Hobbs , P. V. , King , M. D. , Randall , D. A. , Minnis , P. , Isaac , G. A. , Pinto , J. O. , Uttal , T. , Bucholtz , A. , Cripe , D. G. , Gerber , H. , Fairall , C. W. , Garrett , T. J. , Hudson , J. , Intrieri , J. M. , Jakob , C. , Jensen , T. , Lawson , P. , Marcotte , D. , Nguyen , L. , Pilewskie , P. , Rangno , A. , Rogers , D. C. , Strawbridge , K. B. , Valero , F. P.J. , Williams , A. G. and Wylie , D. 2000 . FIRE Arctic Clouds Experiment . Bull. Am. Meteorol. Soc. , 81 : 5 – 29 .
- Gascon , G. , Stewart , R. E. and Henson , W. 2010 . Major cold-season precipitation events at Iqaluit, Nunavut . Arctic , 63 : 327 – 337 .
- Hanesiak , J. , Stewart , R. E. , Szeto , K. , Hudak , D. and Leighton , H. 1997 . The structure, water budget, and radiational features of a high-latitude warm front . J. Atmos. Sci. , 12 : 1553 – 1573 .
- Hanesiak , J. , Stewart , R. E. , Taylor , P. , Moore , K. , Barber , D. , Mcbean , G. , Strapp , W. , Wolde , M. , Goodson , R. , Hudson , E. , Hudak , D. , Scott , J. , Liu , G. , Gilligan , J. , Biswas , S. , Desjardins , D. , Dyck , R. , Fargey , S. , Field , R. , Gascon , G. , Gordon , M. , Greene , H. , Hay , C. , Henson , W. , Hochheim , K. , Laplante , A. , Martin , R. , Melzer , M. A. and Zhang , S. 2010 . Storm Studies in the Arctic: The meteorological field project . Bull. Am. Meteorol. Soc. , 91 doi: 10.1175/2009BAMS2693.1
- Hudak , D. , Currie , B. , Stewart , R. , Rodriguez , P. , Burford , J. , Bussières , N. and Kochtubajda , B. 2004 . Weather systems occurring over Fort Simpson, Northwest Territories, Canada, during three seasons of 1998–1999: 1. Cloud features . J. Geophys. Res. , 109 : D22108 doi:10.1029/2004JD004876
- Hudak , D. , Rodriguez , P. and Donaldson , N. 2008 . Validation of the CloudSat precipitation occurrence algorithm using the Canadian C band radar network . J. Geophys. Res. , 113 : D00A07 doi:10.1029/2008JD009992
- Im , E. , Wu , C. and Durden , S. L. 2005 . Cloud profiling radar for the CloudSat mission . IEEE Aerosp. Electron. Syst. Mag. , 20 ( 10 ) : 15 – 18 .
- IPCC (Intergovernmental Panel on Climate Change). 2007. Climate change 2007: The physical science basis. Retrieved from http://www.ipcc.ch/ipccreports/ar4-wg1.htm
- Kikuchi , K. and Kajikawa , M. 1979 . Comments on v-shaped snow crystals observed in Arctic Canada . J. Meteorol. Soc. Jpn. , 57 : 484 – 487 .
- L'ecuyer , T. S. and Stephens , G. L. 2002 . An estimation-based precipitation retrieval algorithm for attenuating radars . J. Appl. Meteorol. , 41 : 272 – 285 .
- Lhermitte , R. 1988 . Observation of rain at vertical incidence with a 94 GHz Doppler radar: An insight on Mie scattering . Geophys. Res. Lett. , 15 : 1125 – 1128 .
- Mace , G. G. , Marchand , R. , Zhang , Q. and Stephens , G. 2007 . Global hydrometeor occurrence as observed by CloudSat; Initial observations from summer 2006 . Geophys. Res. Lett. , 34 : L17811 doi:10.1029/2006GL029017
- G.G , Mace , Zhang , Q. , Vaughan , M. , Marchand , R. , Stephens , G. , Trepte , C. and Winker , D. 2009 . A description of hydrometeor layer occurrence statistics derived from the first year of merged Cloudsat and CALIPSO data . J. Geophys. Res. , 114 : D00A26 doi:10.1029/2007JD009755
- Magano , C. and Kikuchi , K. 1980 . Some observations of snowfall and meteorological conditions in Arctic Canada . Mon. Weather Rev. , 108 : 1656 – 1664 .
- Maxwell , J. B. 1981 . Climatic regions of the Canadian Arctic Islands . Arctic , 34 : 225 – 240 .
- Nawri , N. and Stewart , R. E. 2006 . Climatological features of orographic low-level jets over Frobisher Bay . Atmosphere-Ocean , 44 : 397 – 413 .
- Noh , Y. , Seaman , C. J. , Vonder Haar , T. H. , Hudak , D. R. and Rodriguez , P. 2011 . Comparisons and analyses of aircraft and satellite observations for wintertime mixed phase clouds . J. Geophys. Res. , 116 : D18207 doi:10.1029/2010JD015420, 2011
- Sassen , K. , Cambell , J. R. , Zhu , J. , Kollias , P. , Shupe , M. and Williams , C. 2005 . Lidar and triple-wavelength Doppler radar measurements of the melting layer: A revised model for dark- and brightband phenomena . J. Appl. Meteorol. , 44 : 301 – 312 .
- Sassen , K. and Wang , Z. 2008 . Classifying clouds around the globe with the CloudSat radar: 1-Year of results . Geophys. Res. Lett. , 35 : L04805 doi:10.1029/2007GL032591
- Sassen , K. , Wang , Z. and Liu , D. 2008 . Global distribution of cirrus clouds from CloudSat/Cloud-Aerosol Lidar and Infrared Pathfinder Satellite Observations (CALIPSO) measurements . J. Geophys. Res. , 113 : D00A12 doi:10.1029/2008JD009972
- Stephens , G. L. , Vane , D. G. , Boain , R. J. , Mace , G. G. , Sassen , K. , Wang , Z. , Illingworth , A. J. , O'connor , E. J. , Rossow , W. B. , Durden , S. L. , Miller , S. D. , Austin , R. T. , Benedetti , A. , Mitrescu , C. and the CloudSat Science Team . 2002 . The CloudSat Mission and the A-Train: A new dimension of space-based observations of clouds and precipitation . Bull. Am. Meteorol. Soc. , 83 : 1771 – 1790 .
- Stewart , R. E. and Burford , J. E. 2002 . On the features of clouds occurring over the Mackenzie River basin . J. Geophys. Res. , 107 : 4720 doi:10.1029/2001JD001559
- Stewart , R. E. , Burford , J. E. , Hudak , D. R. , Currie , B. , Kochtubajda , B. , Rodriguez , P. and Liu , J. 2004 . Weather systems occurring over Fort Simpson, Northwest Territories, Canada, during three seasons of 1998–1999: 2. Precipitation features . J. Geophys. Res. , 109 : D22109 doi:10.1029/2004JD004929
- Yannuzzi , V. T. , Clothiaux , E. , Verline , H. and Harrington , J. Weather and forecasting during mixed-phase Arctic Cloud Experiment . Fifteenth ARM Science Team Meeting Proceedings . Dayton Beach, FL .