Abstract
To identify flow pathways through the Canadian Arctic Archipelago, numerical model results were examined using two configurations of the Nucleus for European Modelling of the Ocean (NEMO). After correcting for shortwave radiation, the models captured much of the observed spatiotemporal structure of the sea-ice and ocean circulation in the Canadian Arctic Archipelago, especially the southward flow in M'Clintock Channel and the cyclonic circulation in eastern Lancaster Sound. The southward flow in M'Clintock Channel is driven by ageostrophic accelerations and is controlled by topography. Vorticity dynamics analysis showed that both stratification and bathymetry have a strong impact on the circulation in eastern Lancaster Sound.
RÉSUMÉ Pour identifier les trajets d’écoulement à travers l'archipel Arctique canadien, nous avons examiné les résultats de modèles numériques en utilisant deux configurations du NEMO (Nucleus for European Modelling of the Ocean). Après avoir appliqué une correction visant à tenir compte du rayonnement de courtes longueurs d'onde, les modèles capturent la majeure partie de la structure spatiotemporelle de la glace de mer et de la circulation océanique dans l'archipel Arctique canadien, en particulier l’écoulement vers le sud dans le détroit de M'Clintock et la circulation cyclonique dans l'est du détroit de Lancaster. L’écoulement vers le sud dans le détroit de M'Clintock est dû à des accélérations agéostrophiques et est contrôlé par la topographie. L'analyse de la dynamique tourbillonnaire a montré que tant la stratification que la bathymétrie ont un effet marqué sur la circulation dans l'est du détroit de Lancaster.
1 Introduction
The Canadian Arctic Archipelago (CAA) is a complex network of straits and basins connecting the Arctic Ocean and the Atlantic Ocean (). Parry Channel, which runs from M'Clure Strait, through Viscount Melville Sound, Barrow Strait to Lancaster Sound and has a 131 m sill in Barrow Strait, is one of three major passageways for water flow from the Arctic Ocean to Baffin Bay. The other two passageways are through Nares Strait and Jones Sound.
Fig. 1 The name of the islands, straits and locations in the model domain, AG: Amundsen Gulf, B: Bellot Strait, D: Dolphin and Union Strait, BM: Byam Martin Channel, BaS: Barrow Strait, FH: Fury and Hecla Strait, FS: Franklin Strait, GB: Gulf of Boothia, LS: Lancaster Sound, JS: Jones Sound, MaS: Mackenzie Shelf, MC: M'Clintock Channel, MS: McClure Strait, NS: Nares Strait, Pe: Peel Sound, PS: Penny Strait, VMS: Viscount Melville Sound, VS: Victoria Strait, C: Cape Bathurst, CR: Clyde River, De: Devon Island, CI: Cornwallis Island, KW: King William Island, R: Resolute Bay, PP: Prince Patrick Island, PWI: Prince of Wales Island. PRI: Prince Regent Inlet, QEI: Queen Elizabeth Islands, SI: Somerset Island, The thick red line shows the location of a section in . MS, VMS, BaS, and LS constitute Parry Channel.
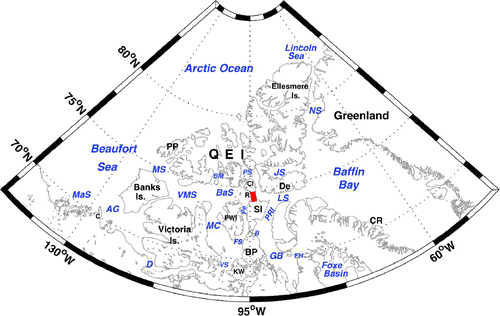
The CAA is one of the main pathways for freshwater outflow from the Arctic Ocean to the Atlantic Ocean (Dickson et al., Citation2007). This fresh water may affect many aspects of the north Atlantic subpolar gyre from deep water formation (Aagaard and Carmack, Citation1989, Citation1994) to nutrient supply and productivity (Carmack, Citation2007). The importance of the freshwater outflow from the CAA maybe become more important in future climate scenarios (Dickson et al., Citation2007). The waters of the CAA are also very important locally as a biological habitat (Stephenson and Hartwig, Citation2010) and provide hunting grounds for the local Inuit peoples (Wenzel, Citation1978) as well as a possible transportation corridor (Pharand, Citation1984).
Flow through the central archipelago is directed from the Beaufort Sea to Baffin Bay. The zeroth order driving mechanism is the sea level difference (Stigebrandt, Citation1984) with temporal anomalies strongly correlated with the wind stress over the Beaufort Sea (Prinsenberg et al., Citation2009). Extended current meter measurements have been made in Barrow Strait () over the past decade (Prinsenberg and Hamilton, Citation2005) and provide the single long-term record of currents and transports in the region (Melling et al., Citation2008). Otherwise, only limited short-term current records exist (Melling et al., Citation1984). General ideas related to the sea ice, such as the southward flowing ice stream in M'Clintock Channel (), extend back to the 1850s and the search for Franklin's expedition. But detailed sea-ice concentration maps only extend back to the 1960s (Canadian Ice Service, Citation2007), and detailed studies of ice motion are few (Agnew et al., Citation2008).
The surface layer (above 200 m) in the Beaufort Sea is fresher and lighter than that in Baffin Bay (Rudels, Citation1986), and the difference in steric height from the Beaufort Sea to Baffin Bay, with respect to the 250 db level, was calculated by Muench (Citation1971) to be 0.3 m. This difference creates pressure gradients and drives the flow through the CAA (Rudels, Citation1986). In M'Clure Strait, the flow is southeastward and the baroclinic flow component dominates the geostrophic flow (Melling et al., Citation1984).
There is a southward flow towards Parry Channel via Byam Martin Channel and Penny Strait, with speeds of 5–13 cm s−1 based on current meter measurement (Fissel et al., Citation1988). In M'Clintock Channel, available current meter measurements shows the current is southward (Barry, Citation1993).
Based on hydrographic data and month-long sets of current meter data, part of the eastward transport through Barrow Strait loops southward into northern Peel Sound before continuing eastward through Barrow Strait at the northern entrance to Peel Sound (Prinsenberg and Bennett, 1989). In April 1981, the northward transport (0.17 Sv; 1 Sv = 106 m3 s−1) in eastern Peel Sound was higher than the southward transport (0.02 Sv) in western Peel Sound and was a significant contributor to Barrow Strait transport (Prinsenberg and Bennett, Citation1989).
There is a strong eastward current (10–20 cm s−1) on the south side of western Lancaster Sound and the flow is mainly barotropic while the current is weak on the north side of the sound, and the flow is mainly baroclinic (Prinsenberg and Hamilton, Citation2005). Based on a section through western Lancaster Sound coincident with the moorings of Prinsenberg and Hamilton (Citation2005), the annual mean volume transport at the section, over 1998–2006 based on mooring data, is 0.7 ± 0.3 Sv (Prinsenberg et al., Citation2009). M'Clure Strait and Viscount Melville Sound, Byam Martin Channel, Penny Strait and Peel Sound are all sources of the transport through western Lancaster Sound.
In eastern Lancaster Sound, the Baffin Current penetrates westward along the north side of eastern Lancaster Sound, crosses to the south side and then flows out to the east, based on satellite-tracked drifter measurements, hydrographic data and current meter data from the summers of 1978 and 1979 (Fissel et al., Citation1982). In the core of the intrusive current at the north side of eastern Lancaster Sound, the near-surface speed was 75 cm s−1, decreasing to 50 cm s−1 at 40 m depth and 25 cm s−1 at 250 m depth (Fissel et al., Citation1982). Because the flow is eastward throughout Barrow Strait between Cornwallis Island and Somerset Island (Prinsenberg and Bennett, Citation1987), the Baffin Current does not extend as far as Resolute Bay, which is located to the south of Cornwallis Island. Jones and Coote (Citation1980) analyzed water masses and nutrient distribution in Parry Channel and Baffin Bay and also suggested that the Baffin Water could not intrude into the region near Resolute Bay.
Based on data from conductivity, temperature, depth (CTD) data, the flow on the north side of Viscount Melville Sound, Barrow Strait and Lancaster Sound is westward (de Lange Boom et al., Citation1987). However, as far as we know, there is no direct current meter data from sensors moored on the north side of Viscount Melville Sound. Holloway and Wang (Citation2009) successfully simulated the westward current on the north side of Parry Channel in a global ice–ocean coupled model. They did this using the Neptune effect, which is a forcing of mean flows by eddy-topography interaction.
Numerical modelling of the CAA in the past has been very limited and the flow dynamics within the CAA are poorly understood. Kliem and Greenberg (Citation2003) used a diagnostic model to study the summer mean circulation in the CAA. Their model results revealed that the currents in the CAA depend on the elevation difference between the Arctic Ocean and Baffin Bay and on the baroclinic pressure gradients. Sou and Flato (Citation2009) used a high resolution ice–ocean regional model to examine how sea-ice conditions in the archipelago region respond to climate projections. The Neptune effect is also considered in their model. Aksenov et al. (Citation2010) studied the sources of fresh water in the CAA and the routes of different water masses and their transport passing through the CAA.
There are still many open questions related to the circulation in the central CAA. Why is the ice motion in M'Clintock Channel southward? Especially because, in August, the wind is directed to the north out of M'Clintock Channel. Why does the Baffin Current not intrude into the region near Resolute Bay? Because the Arctic is rapidly evolving (Serreze et al., Citation2007), understanding the dynamics that explain these observed features is important if we wish to know how these processes may change in the future.
We used a pair of numerical configurations of the CAA and the Arctic to examine these questions, focusing on the ocean dynamics of the central archipelago. In Section 2, we will discuss the configurations. After showing that the model results reproduce the main features in the observations in Section 3, in Sections 4 and 5 we will provide answers to the questions we posed above. Finally we will summarize and examine the significance of our results.
2 Model configurations
We used two configurations of version 3.1 of the Nucleus for European Modelling of the Ocean (NEMO) ocean/sea-ice general circulation model numerical code (Madec, Citation2008) including the Louvain-la-Neuve sea-ice model (LIM2) (Fichefet and Maqueda, Citation1997) in which ice dynamics are updated with an elastic-viscous-plastic (EVP) rheology (Hunke and Dukowicz, Citation1997) implemented on a C-grid (Bouillion et al., Citation2009). We mainly focused on an experiment using a limited domain configuration of the CAA. We also present results from one experiment that used a pan-Arctic configuration, to help explain aspects of the cyclonic flow in eastern Lancaster Sound that are not as well represented in the CAA configuration.
Both configurations have 46 levels in the vertical, with thicknesses from 6 m at the surface to 250 m at the bottom. A partial step representation of bathymetry is used. The two configurations use a linear free surface formulation, Laplacian horizontal viscosity, non-linear bottom boundary friction, and a turbulence closure scheme (order 1.5) for vertical mixing (Blanke and Delecluse, Citation1993).
The two configurations were spun up from 1 September, using initial climatological temperature and salinity based on the Polar Science Center Hydrographic Climatology (PHC) 3.0 dataset (Steele et al., Citation2001) and run under climatological forcing (normal year forcing). The coupled sea-ice–ocean model was forced with 6-hourly surface air temperature, specific humidity and 10 m winds, daily radiative fluxes, monthly precipitation and snow from Large and Yeager (Citation2004). Normal year forcing data were used in the two configurations. Turbulent fluxes at the sea surface were implemented according to the Coordinated Ocean Reference Experiments (CORE) strategy, utilizing the bulk forcing methodology for global ocean ice models developed by Large and Yeager (Citation2004). Surface albedo was parameterized following Shine and Henderson-Sellers (Citation1985). At the ocean boundaries we needed data for temperature, salinity and normal velocities. A detailed description of the numerics of the open boundaries can be found in Treguier et al. (Citation2001).
The CAA model domain is shown in a. A tri-polar grid (Murray, Citation1996) is used in the horizontal, with one pole situated in the Pacific Ocean near Alaska and one pole situated in the northern Atlantic Ocean near Greenland. There are 446 × 268 points in the horizontal, and the model resolution varies between 6.5 km and 9.5 km and is 7.5 km in Lancaster Sound. The bathymetry in the CAA model was obtained from the International Bathymetric Chart of the Arctic Ocean (IBCAO) (Jakobsson et al., Citation2008). The water depth in Bellot Strait, which is located between Somerset Island and Boothia Peninsula, was set to 17 m according to Melling (Citation2000) (a). The bathymetry was smoothed with a Shapiro filter. There is a relaxation to the PHC climatology for sea surface salinity. The coefficient (0.167 m d–1) amounts to a decay time of 60 days for 10 m of water depth; under the ice cover restoring is five times stronger. No restoring to the sea surface temperature was applied. Some parameters of the coupled ice–ocean model are given in .
Fig. 2 (a) The CAA configuration and (b) The pan-Arctic configuration with the background bathymetry (m).
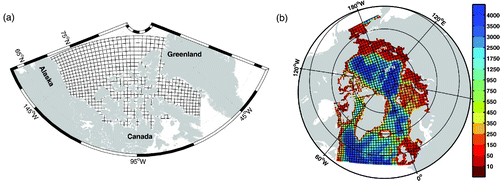
Fig. 3 Bathymetry (m) in the (a) CAA control experiment, (b) the CAA-MC experiment, (c) the CAA-FS experiment and (d) the CAA-BS experiment.
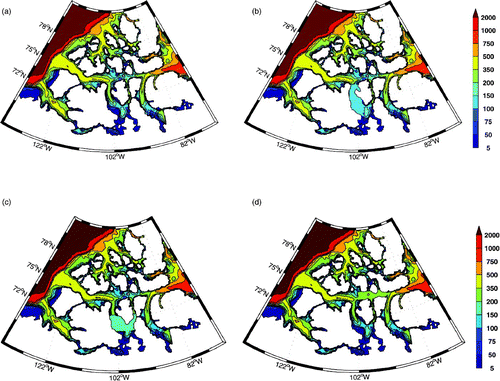
Table 1. Details of the model configurations and experiments used in the paper.
There are two boundaries. To the east, the boundary is located in Baffin Bay. To the north, the model is connected to the Arctic Ocean and the Chukchi Sea. The monthly temperature, salinity and the ocean velocities at the boundary were taken from a global model with a 1° horizontal resolution (Holloway and Wang, Citation2009) and were averaged over the years 1958–2004. In northern Foxe Basin, a closed boundary was used. According to observations, the volume transport through Fury and Hecla Strait is between 0.04 Sv (Sadler, Citation1982) and 0.1 Sv (Barber, Citation1965).
The monthly sea-ice boundary data (ice velocities, ice concentration and ice thickness) were derived from the Pan-Arctic Ice-Ocean Modeling and Assimilation System (PIOMAS) datasets (Zhang and Rothrock, Citation2003). The monthly data were averaged over 1978–2004. The initial ice concentration was taken as the mean ice concentration on 1 September from 1984 to 2004 derived from SSM/I datasets (Cavalieri et al., Citation2008). The initial ice thickness was 2 m. In Nares Strait, the ice velocity was set to zero from early February to mid-July according to Kwok et al. (Citation2010) in order to simulate the ice bridging in Nares Strait. Tides were not considered in the model. Becausce tides might have an important impact on polynyas in the CAA (Hannah et al., Citation2009), tidal forcing will be considered in a future version of the CAA configuration. The CAA control experiment ran for eight years. Unless specified otherwise, mean quantities for the CAA configuration in this paper refer to averages over years 6–8.
Because the mountainous topography in the northern Archipelago is not resolved by the National Centers for Environmental Prediction/National Center for Atmospheric Research (NCEP/NCAR) model, surface air temperatures over Nares Strait and much of the Archipelago in the NCEP/NCAR reanalysis are too cold in summer and represent high-altitude glaciers of Greenland and Ellesmere Island (Sou and Flato, 2009). The Large and Yeager (Citation2004) surface air temperature is based on NCEP/NCAR reanalysis data (Large and Yeager, Citation2004). Although the NCEP/NCAR surface air temperatures are corrected monthly by the mean monthly climatological difference between the International Arctic Buoy Program/Polar Exchange at the Sea Surface (IABP/POLES) dataset (Rigor et al., Citation2000) and the NCEP/NCAR reanalysis over the Arctic cap north of 70°N (Large and Yeager, Citation2004), the revision did not consider the spatial variability of surface air temperature. Thus, for the CAA configuration, the Large and Yeager (Citation2004) surface air temperatures were adjusted again using IABP/POLES data, the same approach used by Sou and Flato (Citation2009). Our adjustment simply removes the monthly climatological difference between the Large and Yeager (Citation2004) and IABP/POLES datasets in the normal year forcing. There are still large differences around Nares Strait, with the differences being larger than 2.5°C in August and September.
Downwelling shortwave radiation (DSR) is a major term in the surface heat budget and is an important factor in controlling sea-ice melting and growth (Maykut and Untersteiner, Citation1971). The Large and Yeager (Citation2004) DSR is based on the International Satellite Cloud Climatology Project (ISCCP) dataset which was created using a General Circulation Model (GCM) radiative transfer model (Large and Yeager, Citation2004). We compared the DSR from Large and Yeager (Citation2004) with the DSR from the Arctic Global Radiation (AGR) dataset (Box et al., Citation1998). In the AGR, the DSR is obtained from land stations, ocean drifting stations and empirically derived long-term climatological estimates from earlier Russian studies (Serreze et al., Citation1998). The ocean drifting stations include the Soviet North Pole drifting ice stations (Marshunova and Mishin, Citation1994) and the U.S. drifting stations (ice island “T-3”, “Arils II”) (Roulet, Citation1969; Marshunova and Chernigovskii, Citation1971; Weller and Holmgren, Citation1974).
Both the DSR from the AGR dataset (a) and from Large and Yeager (Citation2004) (b) show that, in June, the DSR is high over the Greenland ice sheet and is relatively low over the central and southern archipelago, for example. The DSR is high over the Greenland ice sheet because of the elevation effect on atmospheric path length and the tendency for the high central portions of the ice sheet to be above the bulk of the cloud cover (Serreze et al., Citation1998). In summer, the DSR decreases away from the North Pole when the latitude is less than 70°N because of the combined effects of length of day and solar zenith angle (Serreze et al., Citation1998). The DSR difference between the AGR data and Large and Yeager's (Citation2004) data (c), which is higher than 60 W m−2, in June is highest in northern Baffin Bay. The DSR difference between the AGR data and Large and Yeager's (Citation2004) data in northern Baffin Bay is also higher than 60 W m−2 in May, and still approximately 30 W m−2 in July and August. An interpolation was used to map the irregularly spaced data to data on a regular grid in AGR data (Serreze et al., Citation1998), which may lead to errors. However, since there are several observational sites around northern Baffin Bay (Serreze et al., Citation1998), we think Large and Yeager's (Citation2004) underestimation of the DSR around northern Baffin Bay is real. This leads to heat fluxes that are too small and too much sea ice in summer. Since the AGR dataset covers the CAA configuration's domain, we used these data for our shortwave radiation. The monthly mean AGR data value was used as the forcing for every day in a given month.
Fig. 4 Downwelling shortwave radiation (W m−2) in June (a) from the Arctic Global Radiation dataset, (b) from the Large and Yeager (Citation2004) dataset. (c) The difference between the AGR dataset and the Large and Yeager (2004) dataset for June.
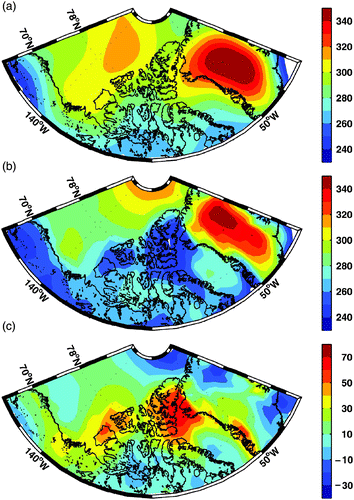
In order to study the effect of bathymetry and sea surface salinity restoring on the circulation in the CAA, four sensitivity experiments (CAA-NS, CAA-MC, CAA-FS, CAA-BS) were conducted (). The CAA-NS experiment is the same as the CAA control experiment except sea surface salinity restoring is removed. We then continued with sea surface salinity restoring removed in all further sensitivity experiments. In CAA-MC, the water depth between 70°–73.7°N and 105°–100°W, was set to 106 m which is the same as the water depth over the sill to the south of Prince of Wales Island (b). In CAA-FS, the water depth between 70.5°–72.3°N and 108°–95.2°W and the sill south of Prince of Wales Island were removed (c). In CAA-BS, the sill in Barrow Strait was removed and the water depth was set to 300 m between 70°–73.7°N and 105°–100°W (d).
Table 2. List of the six numerical experiments.
The pan-Arctic configuration domain, model grids and bathymetry are shown in b. There are 568 × 400 points in the horizontal and the model resolution ranges from 9 km to 15 km, with 11 km in Lancaster Sound. The bathymetry in the pan-Arctic configuration is obtained from 1-minute global relief data ETOPO1 (Amante and Eakins, Citation2009). There are two open boundaries, one located in the Atlantic Ocean and the other located in the Pacific Ocean. The monthly boundary data were derived from a 1/4° global model based on the eddy permitting ORCA025 configuration (Barnier et al., Citation2006) and were averaged over the years 1979–2004. ORCA025 is a global configuration of NEMO implemented on an ORCA grid at 1/4° resolution. There are three buffer zones, two located near the open boundaries with another located in Foxe Basin. The width of the buffer zones is 40 grid points. The temperature and salinity were restored to the monthly climatology (PHC data), with the relaxation time scale ranging from 1 day near the boundary to 40 days at the outer edge of the buffer zone. There was no sea surface salinity restoring in this configuration. The surface air temperature and DSR were taken from the CORE dataset and were not adjusted. The pan-Arctic experiment ran for 18 years. Unless specified otherwise, mean quantities for the pan-Arctic configuration in this paper refers to years 16–18.
3 Model evaluation
Focusing on the regions important for our scientific questions (M'Clure Strait to Lancaster Sound, in the central archipelago), we will first show that the model results agreed well enough with the observations to allow us to use the model fields to explain the relevant dynamics.
a Sea Ice
Sea-ice concentration is an important parameter to examine because there are satellite measurements to validate the performance of the models. a and 5b compare the 1984–2004 mean sea-ice concentration fields in July derived from Scanning Multichannel Microwave Radiometer (SMMR) (1984-August 1987) and SSM/I (September 1987–2004) datasets (Cavalieri et al., Citation2008) with the results from the CAA control experiment. Overall, the spatial pattern of the model-simulated sea-ice concentration was consistent with the satellite measurements. The North Water Polynya, located in northern Baffin Bay between Ellesmere Island and Greenland, is an important habitat for wildlife. The model-simulated spatial patterns of the North Water Polynya are similar to observed patterns. In M'Clure Strait and Viscount Melville Sound, the sea-ice is nearly stagnant from December to May while the sea ice moves eastward in eastern Lancaster Sound. The sea-ice motion pattern in Parry Channel agrees well with observations (Agnew et al., Citation2008). Here the sea-ice concentration in July was used because the sea-ice concentration in Baffin Bay is better simulated after correcting the DSR using the AGR dataset. In southeastern Baffin Bay, the sea-ice concentration was low because of the warm West Greenland Current. In the southern Beaufort Sea, the Cape Bathurst polynya in Amundsen Gulf opens in July when the sea ice is driven westward by the wind. Both model results and observational data show that Baffin Bay is nearly ice free in August and September and the sea ice gradually expands to cover the entire model domain by January (not shown here).
Fig. 5 (a) Mean sea-ice concentration in July for the period 1984–2004 from SSM/I data. (b) Simulated mean sea-ice concentration in July and (c) mean sea-ice thickness (m) in April averaged over years 6–8 in the CAA control experiment.
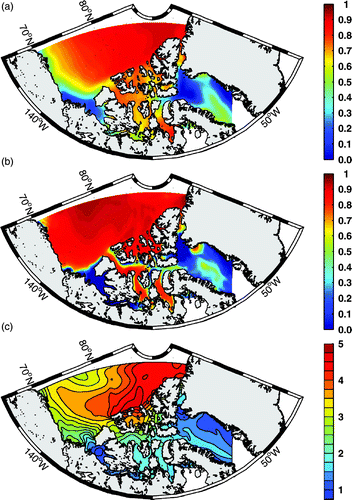
Another important aspect of sea ice is its thickness. In April, the modelled ice thickness was highest near the northwest coast of the Queen Elizabeth Islands, which was consistent with observations (Bourke and Garrett, Citation1987; Melling, Citation2002). The ice thickness decreases from the west coast of Prince Patrick Island to the Beaufort Sea north of Alaska (c). The ice thickness also decreases with latitude from northwest of the Queen Elizabeth Islands to Parry Channel (c). The difference in ice thickness from February to May in most of the model domain is less than 0.5 m. shows some observations of ice thickness and modelled ice thickness. The modelled ice thickness compares well with observations in most regions. Interannual variability in ice thickness may affect the comparison. For example, the ice thickness ranges from 0.9 m to 2 m at Clyde River in April.
Table 3. Observed ice thickness and modelled ice thickness (m).
b Ocean Currents
The flow through M'Clure Strait is southeastward. Part of the current through M'Clure Strait comes from the southern Beaufort Sea, while part of the inflow comes from a southwestward flow north of the CAA. The core of the current is on the north side in M'Clure Strait and diverts to the south side in eastern Viscount Melville Sound (). The current that originates north of the archipelago also flows southward into Parry Channel via Byam Martin Channel and Penny Strait, with surface velocities in the range of 2–9 cm s−1. Near the northern entrance of M'Clintock Channel, part of the current from M'Clure Strait bifurcates into M'Clintock Channel and then flows into Peel Sound via Franklin Bay. The southward surface current peaks at 7 cm s−1 in northwestern M'Clintock Channel.
Fig. 6 (a) The mean currents in the CAA averaged over the top 300 m and over years 6–8 in the CAA control experiment, with one-fifth of the horizontal grid points shown. An enlargement of the inset box is shown in . (b) as in (a) but for the pan-Arctic experiment averaged over years 16–18, with one-third of the horizontal grid points shown.
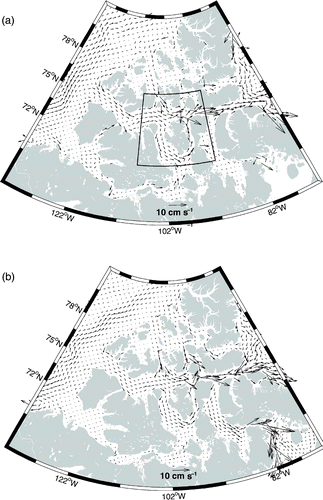
The current through Barrow Strait intrudes into northern Peel Sound as a cyclonic current loop (a). The mean volume transport through Peel Sound in April is 0.19 Sv in the CAA control experiment and 0.20 Sv in the pan-Arctic experiment, which are similar to a previous estimate (0.15 Sv) (Prinsenberg and Bennett, Citation1989). Because the sill in Dolphin and Union Strait is 26 m in the IBCAO dataset, the mean volume transport through Dolphin and Union Strait was 0.02 Sv in the CAA control experiment. The water flowing through Peel Sound came mainly from M'Clintock Channel. In eastern Barrow Strait, the core of the eastward current is located on the south side (). The model currents show a jet just off the shelf break at the south side in April, with velocities decreasing to the north (), as seen by Prinsenberg and Bennett (Citation1987). Velocities at 50 m are approximately 13 cm s−1, close to the observed maximum (Prinsenberg and Bennett, Citation1987), albeit the model maximum of 18 cm s−1 was at the surface. Based on a section through western Lancaster Sound coincident with the moorings of Prinsenberg and Hamilton (Citation2005), the long-term mean volume transport was 0.77 Sv in the CAA control experiment and 0.85 Sv in the pan-Arctic experiment, slightly larger than the 0.7 Sv transport (with interannual variations of ± 0.3 Sv), based on observations (Prinsenberg et al., Citation2009).
Fig. 7 Enlargement of the inset area in a showing the currents. The background field is log10(f/h). The red line shows the position of line A, along which the momentum balance is averaged in .
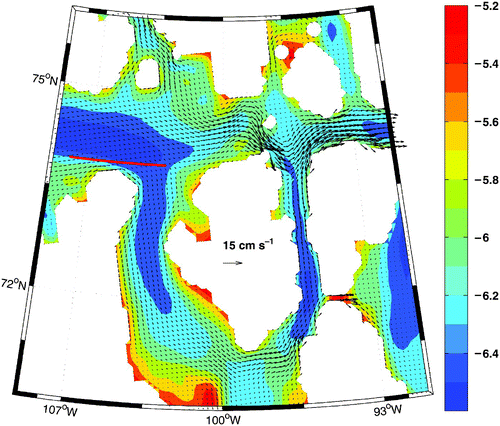
Fig. 8 The mean cross-section current speed (cm s−1) in Barrow Strait (section shown in ) in April averaged over years 6–8 in the CAA control experiment.
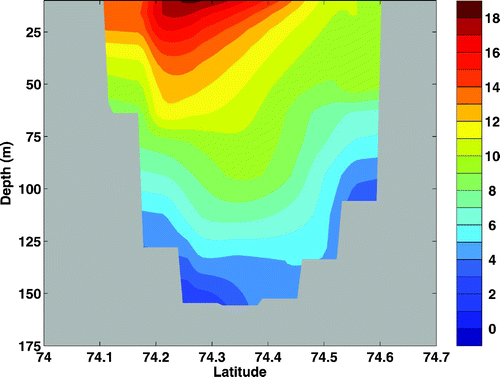
In eastern Lancaster Sound, there was a persistent cyclonic flow throughout the year and the intrusive flow of the Baffin Current reached 85°W on the north side of the sound in the pan-Arctic experiment (a). In the vicinity of Cape Warrender (Devon Island, a), the current flowed southward, which agrees with observations (Fissel et al., Citation1982). The westward current speed at the north side of the sound, which is very barotropic in the model, was about 10 cm s−1 (b). The eastward current speed at the south side of the sound was more than 20 cm s−1, which is surface trapped at the shelf break (b). Although the mean current fields in the CAA configuration were eastward in eastern Lancaster Sound, the penetration of the Baffin Current is more intermittent.
Fig. 9 (a) The mean Ertel's potential vorticity and currents averaged over the top 300 m in eastern Lancaster Sound. (b) The cross-section current speed (cm s−1) at 81.4°W with the location of the section shown by the thick bold line in panel (c). (c) Surface salinity in eastern Lancaster Sound. (d) The vertical velocity (10−5 m s−1) (contours) over the top 140 m overlying Ertel's potential vorticity at 81.4°W. Downward velocity is negative. The units for Ertel's potential vorticity are 10−10 m−1 s−1. All values are averaged over years 16–18 in the pan-Arctic experiment.
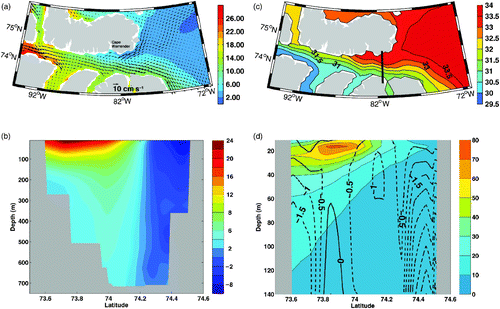
Fig. 10 Momentum balance averaged over the top 50 m along line A, shown in . Ageo represents ageostrophic acceleration. The values have been multiplied by 107.
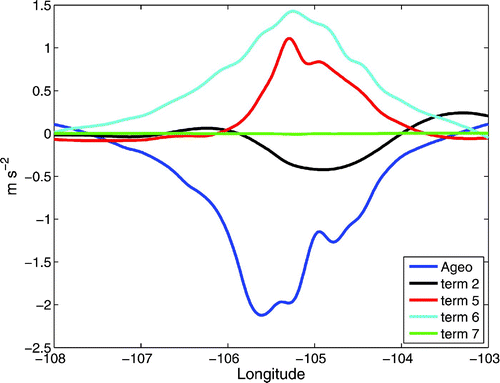
In the pan-Arctic configuration, part of the flow bifurcates into Prince Regent Inlet and flows through the Gulf of Boothia and Fury and Hecla Strait into Foxe Basin, as discussed by Collin (Citation1962) and Barry (Citation1993). Since the CAA configuration uses a closed boundary condition in northern Foxe Basin, the currents in Prince Regent Inlet are weak. Thus, the CAA control experiment had a stronger eastward transport into Lancaster Sound, making it more difficult for the Baffin Current to penetrate the sound.
4 M'Clintock Channel
The southward flow in M'Clintock Channel is persistent throughout the year. The mean volume transport through Viscount Melville Sound was 0.50 Sv in the CAA control experiment, while the mean volume transport through M'Clintock Channel was 0.27 Sv in the CAA control experiment. In the pan-Arctic experiment, the volume transports through Viscount Melville Sound and M'Clintock Channel were 0.37 Sv and 0.21 Sv, respectively. More than half of the water from Viscount Melville Sound flows southward into M'Clintock Channel, before eventually looping around Prince of Wales Island and flowing northward into Peel Sound and then eastern Barrow Strait. Why is the flow in M'Clintock Channel southward? The importance of the southward flow in M'Clintock Channel is discussed further in the summary. In winter (December to May), because the sea ice in M'Clintock Channel is unable to move as a result of strong internal ice stress, the ice stress on the ocean retards the surface currents in M'Clintock Channel. Therefore, the surface stress on the ocean cannot drive the southward flow in M'Clintock Channel.
Now we study the momentum balance of the currents near the northern end of M'Clintock Channel. The horizontal momentum equation is given in the following:
shows the meridional momentum balance along line A over the top 50 m. We studied the meridional momentum balance because we tried to understand why the water flows southward into M'Clintock Channel. The ageostrophic acceleration is defined by the sum of the pressure gradient term and the Coriolis term. At line A, the meridional pressure gradient term is northward and is mainly balanced by the Coriolis term, and the ageostrophic acceleration is small. When the water flows to the northern end of M'Clintock Channel, the ageostrophic acceleration is negative because the pressure gradient weakens as a result of the change in direction of the coastline. Therefore, the water turns southward into M'Clintock Channel because of the Coriolis term. The ageostrophic acceleration is mainly balanced by the vertical diffusion term and the non-linear advection term, with the horizontal viscosity term less than 1 × 10−8 m s−2.
The Coriolis effect is one of the major reasons that the water flows into M'Clintock Channel, but topography also plays an important role in the southward flow in M'Clintock Channel. The water depth is more than 300 m in central Viscount Melville Sound west of M'Clintock Channel decreasing to 131 m at a sill in Barrow Strait (a). Near the northern entrance of M'Clintock Channel, the reduction in water depth decreases more slowly than to the east into Barrow Strait. There is also a sill lying 106 m from the surface to the south of Prince of Wales Island.
Here we examine some sensitivity experiments, focusing on the volume transport averaged over the second year of integration. The volume transport through M'Clintock Channel in CAA-MC was 0.26 Sv, which is nearly the same as the value in CAA-NS. In CAA-FS, the volume transport through M'Clintock Channel increased to 0.37 Sv because of topographic steering. Therefore, the volume transport is strongly influenced by the depth of the sill in Franklin Bay instead of the deep trough in M'Clintock Channel. The volume transport through M'Clintock Channel dereased to 0.13 Sv in CAA-BS as the flow tried to follow the f /h contours, which were approximately parallel to the local isobaths, h. The sill depth in Barrow Strait can also have a strong influence on the flow through M'Clintock Channel.
5 Eastern Lancaster Sound
In the pan-Arctic experiment, we see inflow into the northern part of Lancaster Sound from Baffin Bay, following the bathymetry and flowing to the west before recirculating south of Devon Island to join the eastward flowing circulation from Barrow Strait (a). Holloway and Wang (Citation2009) show that the Neptune effect can explain the penetration of the Baffin Current. Now we try to understand why the Baffin Current does not penetrate farther into the archipelago.
We used vorticity dynamics to study the circulation in eastern Lancaster Sound. We computed Ertel's potential vorticity, averaged over the upper water column (top 300 m or the bottom, whichever is shallower) from the pan-Arctic experiment (a). Note the choice of 300 m is arbitrary, but the results are not sensitive to the exact depth used. Ertel's potential vorticity (EPV) is , where f is the Coriolis parameter, ζ is the relative vorticity, ρ
z
is the vertical density gradient, and ρ0 takes the constant value of 1020 kg m−3. The average water depth at the entrance to eastern Lancaster Sound is between 700 and 800 m. Lancaster Sound gradually shoals westward to a sill lying less than 200 m from the surface in Barrow Strait. Although the topography applies a strong constraint on the EPV field, the stratification also has a significant impact. In order to understand the effect of stratification, the surface salinity in eastern Lancaster Sound is shown in c. Because the flow is from relatively fresh water in the Beaufort Sea to saline water in Baffin Bay, the surface salinity decreases from Barrow Strait to Baffin Bay. The surface water on the south side of the sound is fresher than that on the north side, which agrees with observations (Fissel et al., Citation1982). Because the salinity difference is smaller at depth compared to the surface layer, the stratification pattern is similar to the surface salinity pattern. Thus, there is an EPV gradient along and across Lancaster Sound matching the salinity contours (c). The vertical density gradient ρ
z
becomes larger when the water flows along the north side of eastern Lancaster Sound. Because of the conservation of potential vorticity, the water must flow southward because f decreases when the current moves south. d shows the EPV contours and vertical velocity at 81.4°W in eastern Lancaster Sound. In general, at the same depth, EPV increases from the north side of the sound to the south side (d). We thus find that there is downwelling in the central part of eastern Lancaster Sound (d).
6 Summary and discussion
A coupled ocean–sea-ice CAA model has been developed to simulate the circulation in the CAA. Both sea-ice concentration and ice thickness are consistent with observations after adjusting the surface air temperature and the DSR. The vertical structure and transports in Barrow Strait agree with observations. Furthermore, the southward flow in M'Clintock Channel and northward flow in Peel Sound were reproduced.
The classic view is that the flow through Parry Channel is controlled by the sea surface gradient between the Arctic Ocean and Baffin Bay (Stigebrandt, 1984). However, model results show that the flow is more complex than previously thought. More than half of the water flowing through Visount Melville Sound then looping around Prince of Wales Island and flowing through Peel Sound came mainly from M'Clintock Channel. Our analysis shows that the flow in M'Clintock Channel is produced by a southward ageostrophic acceleration. The southward flow is also strongly influenced by sills to the south of Prince of Wales Island and another one located in Barrow Strait. Our analysis also revealed that the stratification in combination with the topography prevents westward flow farther into Barrow Strait, and downwelling occurs as the flow recirculates towards the south in eastern Lancaster Sound.
a and 11b show the sea-ice concentration, mean ice motion and wind vectors in August and September, respectively. Because of the southward current underneath, the ice moves upwind to the south in M'Clintock Channel. It is thus driven by the ocean currents whose paths are governed by the topographic and ageostrophic acceleration discussed above. In September, the sea-ice concentration is lower in Peel Sound than that in M'Clintock Channel (b), consistent with results derived from SSM/I data. Because of southeastward winds and southward surface currents in M'Clintock Channel, the sea ice moves southward in M'Clintock Channel, and the dynamical import of sea ice is large. This agrees with Agnew et al. (Citation2008) who used Advanced Microwave Scanning Radiometer Earth Observing System (AMSR-E) imagery to estimate sea-ice drift in the CAA from September to June. In Peel Sound, because the winds are southeastward and the surface currents are northward, the sea-ice motion is not fast, thus the amount of dynamical import of sea ice into Peel Sound is not large. Since the dynamical import of sea ice into M'Clintock Channel is larger than into Peel Sound, the sea-ice concentration in M'Clintock Channel is higher than that in Peel Sound in September. Similarly, the concentration of multi-year ice (MYI) is high in M'Clintock Channel in summer (Howell et al., Citation2008). Terwisscha van Scheltinga et al. (Citation2010) studied sea-ice concentration and fluxes in the CAA using a sea-ice model coupled to a slab ocean. In their experiments, the lack of ocean currents led to their model producing similar sea-ice concentrations in both M'Clintock Channel and Peel Sound, again highlighting the importance of the underlying oceanic currents.
Fig. 11 (a) The CAA control experiment ice concentration (color contours) and ice motion vectors (black arrows) and wind vectors (magenta arrows) in (a) August and (b) September.
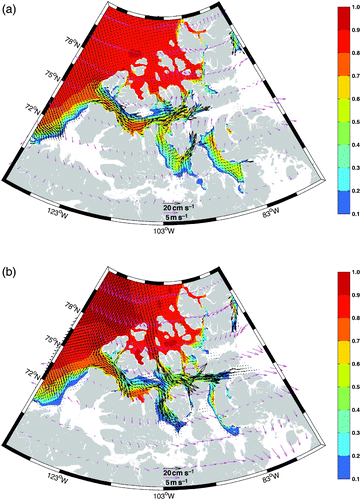
The migration of beluga whales, narwhals and harp seals are linked to ice-edge productivity (Tynan and Demaster, Citation1997). Bowhead whales are adapted to live in the loose ice edges and their migrations roughly track the advance and retreat of the ice edge (Dyke et al., Citation1996). Although Peel Sound is part of the summer home range of harp seals, bowhead whales, beluga whales, and narwhal, they are not found in M'Clintock Channel (Dietz et al., Citation2008; Stephenson and Hartwig, Citation2010). The persistent sea ice in M'Clintock Channel limits the movements of narwhals, bowhead whales, beluga whales, and various species of seal (Dyke et al., Citation1996; Harington et al., Citation1966). Therefore, the southward currents and sea ice in M'Clintock Channel have a significant impact on the marine mammals. Bacause the currents are mainly controlled by the Coriolis force and topography, one may not expect significant changes (such as enhanced sea-ice import to Peel Sound) even in a changing climate.
One of the possible shipping routes in the Northwest Passage is through Peel Sound, Victoria Strait and Amundsen Gulf (Pharand, Citation1984). This route has always been sensitive to the import of MYI into M'Clure Strait and M'Clintock Channel. It was probably the fate of the Franklin Expedition in 1845 to be trapped in this oceanic advected MYI northwest of King William Island (M'Clintock, Citation1859). Again, because the currents are mainly controlled by the Coriolis force and topography, as long as thick MYI enters M'Clure Strait, one may expect it to be advected into M'Clintock Channel. Thus, even in a warmer climate, one may expect difficulties using this route for navigation.
Although the modelled circulation agrees well with observations, the CAA configuration can be improved. This includes consideration of open boundaries and tidal forcing. Additionally, the model has been run with interannual forcing, which will be used in future studies.
Acknowledegements
The authors would like to acknowledge Dr. Z. Wang for sharing his ocean model output with us. The authors also wish to thank C. Haas, S.J. Prinsenberg, C.G. Hannah, Y. Lu and F. Dupont for discussions on the flow in the CAA and sea-ice boundary conditions. This work was supported by the Natural Sciences and Engineering Research Council (NSERC) and Government of Canada International Polar Year awards to PGM, as well as an NSERC graduate scholorship awarded to QW. We are grateful to Westgrid for computational resources. The NCEP/NCAR reanalysis data were provided by the National Oceanic and Atmospheric Administartion/office of Oceanic and Atmospheric Research/ Earth System Research Laboratory: Physical Sciences Division (NOAA/OAR/ESRL:PSD), Boulder, CO, USA. The sea-ice concentration data were provided by NSIDC,Boulder, CO, USA. The PIOMAS output were provided by the University of Washington, Seattle, USA. We thank two anonymous reviewers for their comments which helped improve the manuscript significantly.
References
- Aagaard , K. and Carmack , E. 1989 . The role of sea ice and other fresh water in the Arctic circulation . J. Geophys. Res. , 94 : 14485 – 14498 .
- Aagaard, K. and E. Carmack. 1994. The Arctic Ocean and climate: A perspective. In The Polar Oceans and Their Role in Shaping the Global Environment: The Nansen Centennial Volume, O. M. Johannessen, R. D. Muench, and J. E. Overland, Eds., Vol. 85 (pp. 4–20). Am. Geophys. Union, USA, Geophysical Monograph.
- Agnew , T. , Lambe , A. and Long , D. 2008 . Estimating sea ice area flux across the Canadian Arctic Archipelago using enhanced AMSR-E . J. Geophys. Res. , 113 : C10011 doi:10.1029/2007JC004582
- Aksenov , Y. , Bacon , S. , Coward , A. C. and Holliday , P. N. 2010 . Polar outflow from the Arctic Ocean: A high resolution model study . J. Marine Syst. , 83 : 14 – 37 . doi:10.1016/j.jmarsys.2010.06.007
- Amante, C. and B.W. Eakins. 2009. ETOPO1 1 Arc-Minute global relief model: Procedures, data sources and analysis. NOAA Technical Memorandum NESDIS NGDC-24.
- Barber , F. 1965 . Current observations in Fury and Hecla Strait . J. Fish. Res. Board Can. , 22 : 225 – 229 .
- Barnier , B. , Madec , G. , Penduff , T. , Molines , J. M. , Treguier , A. M. , Sommer , J. L. , Beckmann , A. , Biastoch , A. , Böning , C. , Dengg , J. , Derval , C. , Durand , E. , Gulev , S. , Remy , E. , Talandier , C. , Theetten , S. , Maltrud , M. , McClean , J. and Cuevas , B. D. 2006 . Impact of partial steps and momentum advection schemes in a global ocean circulation model at eddy-permitting resolution . Ocean Dyn. , 56 : 543 – 567 . doi: 10.1007/s10236-006-0082-1
- Barry , R. G. 1993 . “ Canada's cold seas ” . In Canada's cold environment , Edited by: French , H. M. and Slaymaker , O. 29 – 61 . Canada : McGill Queen's University Press . NATO Science for Peace and Security Series
- Blanke , B. and Delecluse , P. 1993 . Variability of the tropical Atlantic Ocean simulated by a general circulation model with two different mixed-layer physics . J. Phys. Oceanogr. , 23 : 1363 – 1388 .
- Bouillon , S. , Maqueda , M. A.M. , Legat , V. and Fichefet , T. 2009 . An elastic viscous plastic sea ice model formulated on Arakawa B and C grids . Ocean Modelling , 27 : 174 – 184 . doi: 10.1016/j.ocemod.2009.01.004
- Bourke , R. H. and Garrett , R. P. 1987 . Sea ice thickness distribution in the Arctic Ocean . Cold Reg. Sci. Technol. , 13 : 259 – 280 .
- box, J., Key, J., Maslanik, J. and Serreze, M. 1998. Arctic Global Radiation (AGR) data set [data]. Retrieved from the National Snow and Ice Data Center, Boulder: http://nsidc.org/data/arcss066.html
- Canadian Ice Service . 2007 . Canadian Ice Service archive documentation series regional charts: History, accuracy, and caveats , Ottawa : Environment Canada .
- Carmack , E. 2007 . The alpha/beta ocean distinction: A perspective on freshwater fluxes, convection, nutrients and productivity in high-latitude seas . Deep-Sea Res. II , 54 : 2578 – 2598 . doi:10.1016/j.dsr2.2007.08.018
- Cavalieri, D.C., C. Parkinson, P. Gloersen and H.J. Zwally. 2008. Sea ice concentrations from Nimbus-7 SMMR and DMSP SSM/I passive microwave data [Data]. Retrieved from the National Snow and Ice Data Center, Boulder: http://nsidc.org/data/nsidc-0051.html
- Collin , A. 1962 . Oceanography in the Canadian Arctic . Can. Geogr-Geogr. Can. , 6 : 120 – 128 .
- de Lange Boom , B. , Melling , H. and Lake , R. A. 1987 . Late winter hydrography of the Northwest Passage , Washington , , D.C, USA : Institute of Ocean Science . Canadian technical report of hydrography and ocean sciences
- Dickson , R. , Rudels , B. , Dye , S. , Karcher , M. , Meincke , J. and Yashayaev , I. 2007 . Current estimates of freshwater flux through Arctic and subarctic seas . Prog. Oceanogr. , 73 : 210 – 230 . doi:10.1016/j.pocean.2006.12.003
- Dietz , R. , Heide-Jrgensen , M. P. , Richard , P. , Jack Orr , K. L. and Schmidt , H. C. 2008 . Movements of narwhals (Monodon monoceros) from Admiralty Inlet monitored by satellite telemetry . Polar Biol. , 31 : 1295 – 1306 . doi:10.1007/s00300-008-0466-4
- Dyke , A. S. , Hooper , J. and Savelle , J. M. 1996 . A history of sea ice in the Canadian Arctic Archipelago based on postglacial remains of the bowhead whale (Balaena mysticetus) . Arctic , 49 : 235 – 255 .
- Fichefet , T. and Maqueda , M. M. 1997 . Sensitivity of a global sea ice model to the treatment of ice thermodynamics and dynamics . J. Geophys. Res. , 102 : 12609 – 12646 .
- Fissel , D. , Lemon , D. D. and Birch , J. 1982 . Major features of the summer near-surface circulation of western Baffin Bay, 1978 and 1979 . Arctic , 35 : 180 – 200 .
- Fissel , D. , Birch , J. , Melling , H. and Lake , R. A. 1988 . “ Non-tidal flows in the Northwest Passage ” . In Canadian technical report of hydrography and ocean sciences , Sidney , , BC, Canada : Department of Fisheries and Oceans .
- Haas , C. , Hendricks , S. and Doble , M. 2006 . Comparison of the sea-ice thickness distribution in the Lincoln Sea and adjacent Arctic Ocean in 2004 and 2005 . Ann. Glaciol. , 44 : 247 – 252 .
- Hannah , C. G. , Dupont , F. and Dunphy , M. 2009 . Polynyas and tidal currents in the Canadian Arctic Archipelago . Arctic , 62 : 83 – 95 .
- Harington , C. R. 1966 . Extralimital occurrences of walruses in the Canadian Arctic . J. Mammal , 47 : 506 – 513 .
- Holloway , G. and Wang , Z. 2009 . Representing eddy stress in an Arctic Ocean model . J. Geophys. Res. , 114 : C06020 doi:10.1029/2008JC005169
- Howell , S. E. , Tivy , A. , Yackel , J. J. and McCourt , S. 2008 . Multi-year sea-ice conditions in the western Canadian Arctic Archipelago region of the Northwest Passage: 1968-2006 . Atmosphere-Ocean , 46 : 229 – 242 . doi:10.3137/ao.460203
- Hunke , E. and Dukowicz , J. 1997 . An Elastic-Viscous-Plastic model for sea ice dynamics . J. Phys. Oceanogr. , 27 : 1849 – 1867 .
- Jakobsson , M. , Macnab , R. , Mayer , L. , Anderson , R. , Edwards , M. , Hatzky , J. , Schenke , H. W. and Johnson , P. 2008 . An improved bathymetric portrayal of the Arctic Ocean: Implications for ocean modeling and geological, geophysical and oceanographic analyses . Geophys. Res. Lett. , 35 : L07602 doi:10.1029/2008GL033520
- Jones , E. P. and Coote , A. R. 1980 . Nutrient distributions in the Canadian Archipelago: Indicators of summer water mass and flow characteristics . Can. J. Fish. Aquat. Sci. , 37 : 589 – 599 .
- Kliem , N. and Greenberg , D. A. 2003 . Diagnostic simulations of the summer circulation in the Canadian Arctic Archipelago . Atmosphere-Ocean , 41 : 273 – 289 .
- Kwok , R. , Pedersen , L. T. , Gudmandsen , P. and Pang , S. S. 2010 . Large sea ice outflow into the Nares Strait in 2007 . Geophys. Res. Lett. , 37 : L03502 doi:10.1029/2009GL041872
- Large, W. and S. Yeager. 2004. Diurnal to decadal global forcing for ocean and sea-ice models: The data sets and flux climatologies. NCAR, NCAR technical note: NCAR/TN-460+ STR.
- Madec, G. 2008. NEMO ocean engine. Institut Pierre-Simon Palace (IPSL), France, Notes de Pole de modelisation, Vol. 27.
- Marshunova, M. and N.T. Chernigovskii. 1971. Radiatsionnyi Rezhim Zarubeznhoi Arktiki (Radiation Regime of the Foreign Arctic). Gidrometeorologicheskoe Izdatel'stvo.
- Marshunova, M. and A. Mishin 1994. Handbook of the radiation regime of the Arctic basin (results from the drift stations). Applied Physics Laboratory, University of Washington, Seattle, WA, Tech. Rep. APL UW TR 9413.
- Maykut , G. A. and Untersteiner , N. 1971 . Some results from a time-dependent thermodynamic model of sea ice . J. Geophys. Res. , 76 : 1550 – 1575 .
- McLaren , A. , Wadhams , P. and Weintraub , R. 1984 . The sea ice topography of M'Clure Strait in winter and summer of 1960 from submarine profiles . Arctic , 37 : 110 – 120 .
- M'Clintock, F.L. 1859. The voyage of the ’Fox’ in the Arctic seas: A Narrative of the discovery of the fate of Sir John Franklin and his companions. London: J. Murray.
- Melling , H. 2000 . “ Exchanges of freshwater through the shallow straits of the North American Arctic ” . In Freshwater Budget of the Arctic Ocean , Edited by: Lewis , E. , Jones , E. P. , Lemke , P. and Prowse , P. 479 – 502 . The Netherlands : Kluwer Academic Publishers . NATO Science Series
- Melling , H. 2002 . Sea ice of the northern Canadian Arctic Archipelago . J. Geophys. Res. , 107 3181, doi:10.1029/2001JC001102
- Melling , H. , Lake , R. , Topham , D. and Fissel , D. 1984 . Oceanic thermal structure in the western Canadian Arctic . Cont. Shelf Res. , 3 : 233 – 258 .
- Melling , H. , Riedel , D. A. and Gedalof , Z. 2005 . Trends in the draft and extent of seasonal pack ice, Canadian Beaufort Sea . Geophys. Res. Lett. , 32 : L24501 doi:10.1029/2005GL024483
- Melling , H. , Agnew , T. A. , Falkner , K. K. , Greenberg , D. A. , Lee , C. M. , Münchow , A. , Petrie , B. , Prisenberg , S. J. , Samelson , R. M. and Woodgate , R. A. 2008 . “ Freshwater fluxes via Pacific and Arctic outflows across the Canadian Polar Shelf ” . In Arctic-Subarctic Ocean fluxes, defining the role of the northern seas in climate , Edited by: Dickson , R. R. , Meincke , J. and Rhines , P. 193 – 247 . New York : Springer .
- Muench , R. 1971 . “ The physical oceanography of the northern Baffin Bay region ” . In The Baffin-North Water project, scientific report 1 , Washington , , D.C, USA : Arctic institute of North America .
- Murray , R. J. 1996 . Explicit generation of orthogonal grids for ocean models . J. Comput. Phys. , 126 : 251 – 273 . doi:10.1006/jcph.1996.0136
- Pharand , D. 1984 . The Northwest Passage: Arctic straits , 199 The Netherlands : Dordrecht .
- Prinsenberg , S. J. and Bennett , E. 1987 . Mixing and transports in Barrow Strait, the central part of the Northwest passage . Cont. Shelf Res , 7 : 913 – 935 . doi: 10.1016/0278-4343(87)90006-9
- Prinsenberg , S. J. and Bennett , E. 1989 . Transport between Peel Sound and Barrow Strait in the Canadian Arctic . Cont. Shelf Res. , 9 : 427 – 444 . doi:10.1016/0278-4343(89) 90008-3
- Prinsenberg , S. J. and Hamilton , J. 2005 . Monitoring the volume, freshwater and heat fluxes passing through Lancaster Sound in the Canadian Arctic Archipelago . Atmosphere-Ocean , 43 : 1 – 22 . doi:10.3137/ao.430101
- Prinsenberg , S. J. , Hamilton , J. , Peterson , I. and Pettipas , R. 2009 . “ Observing and interpreting the seasonal variability of the oceanographic fluxes passing through Lancaster Sound of the Canadian Arctic Archipelago ” . In Influence of Climate Change on the Changing Arctic and Sub-Arctic Conditions , Edited by: Nihoul , J. C. and Kostianoy , A. G. 125 – 143 . The Netherlands : Springer . NATO Science for Peace and Security Series
- Rigor , I. G. , Colony , R. and Martin , S. 2000 . Variations in surface air temperature in the Arctic from 1979–1997 . J. Clim. , 13 : 896 – 914 .
- Roulet, R.R. 1969. Radiation regime of Arctic drifting station ARLIS II, January 1964–May 1965. Department of Atmospheric Sciences, University of Washington, NR 307-252, 58.
- Rudels , B. 1986 . The outflow of polar water through the Arctic Archipelago and the oceanographic conditions in Baffin Bay . Polar Res. , 4 : 161 – 180 .
- Sadler , H. 1982 . Water flow into Foxe Basin through Fury and Hecla Strait . Nat. Can. , 109 : 701 – 707 .
- Serreze , M. C. , Key , J. R. , Box , J. E. , Maslanik , J. A. and Steffen , K. 1998 . A new monthly climatology of global radiation for the Arctic and comparisons with NCEP-NCAR reanalysis and ISCCP-C2 fields . J. Clim. , 11 : 121 – 136 .
- Serreze , M. C. , Holland , M. M. and Stroeve , J. 2007 . Perspectives on the Arctic's shrinking sea ice cover . Science , 315 : 1533 – 1536 . doi:10.1126/science.1139426
- Shine , K. P. and Henderson-Sellers , A. 1985 . Development of a turbulence closure model for geophysical fluid problems . J. Geophys. Res. , 90 : 2243 – 2250 .
- Sou , T. and Flato , G. 2009 . Sea ice in the Canadian Arctic Archipelago: Modeling the past (1950–2004) and the future (2041–60) . J. Clim. , 22 : 2181 – 2198 . doi:10.1175/2008JCLI2335.1
- Steele , M. , Morley , R. and Ermold , W. 2001 . PHC: A global ocean hydrography with a high-quality Arctic Ocean . J. Clim. , 14 : 2079 – 2087 .
- Stephenson, S. and L. Hartwig. 2010. The Arctic marine workshop. Proceedings of the Arctic Basin Symposium, Freshwater Institute, Winnipeg, Manitoba, Can. Manuscript Rep. Fish. Aquat. Sci. No. 2934.
- Stigebrandt , A. 1984 . The North Pacific: A global-scale estuary . J. Phys. Oceanogr. , 14 : 464 – 470 .
- Tang , C. C. , Ross , C. K. , Yao , T. , Petrie , B. , DeTracey , B. M. and Dunlap , E. 2004 . The circulation, water masses and sea-ice of Baffin Bay . Prog. Oceanogr. , 63 : 183 – 228 . doi:10.1016/j.pocean.2004.09.005
- Terwisscha van Scheltinga , A. , Myers , P. G. and Pietrzak , J. D. 2010 . A finite element sea ice model of the Canadian Arctic Archipelago . Ocean Dyn. , 60 : 1539 – 1558 . doi: 10.1007/s10236-010-0356-5
- Treguier , A. M. , Barnier , B. , De Miranda , A.P. , Molines , J. M. , Grima , N. , Imbard , M. , Madec , G. , Messager , C. , Reynaud , T. and Michel , S. 2001 . An eddy permitting model of the Atlantic circulation: Evaluating open boundary conditions . J. Geophys. Res. , 106 : 22 115 – 22 129 .
- Tynan , C. T. and DeMaster , D. P. 1997 . Observations and predictions of Arctic climatic change: Potential effects on marine mammals . Arctic , 50 : 308 – 322 .
- Weller, G. and B. Holmgren. 1974. Summer global radiation and albedo data for three stations in the Arctic basin, Ice Island T-3, Barrow, Prudhoe Bay, 1971–1973. Tech. Rep. No. 2, December 1974, Geophysical Institute, University of Alaska, Fairbank.
- Wenzel , G. 1978 . The harp-seal controversy and the Inuit economy . Arctic , 31 : 3 – 6 .
- Zhang , J. and Rothrock , D. A. 2003 . Modeling global sea ice with a thickness and enthalpy distribution model in generalized curvilinear coordinates . Mon. Weather Rev. , 131 : 845 – 861 .