Abstract
The seasonal characteristics of water masses, mixed-layer depth and the current field east of Luzon Strait are studied using Argo profiling float data. Based on data from March 2006 to November 2010, the temperature-salinity relation indicates that the seasonal variation of water masses is not obvious, except that the surface temperature is lower and the salinity is higher in spring and winter; the water masses in other layers change little. The seasonal variation of the mixed-layer depth, which is deepest at over 170 m in winter and shallowest at about 25 m in summer, is also discussed. Based on the P-vector method, multi-year seasonal mean Argo data and Levitus data are used to calculate the respective current fields. The surface geostrophic current derived from altimetric data is compared with those computed from Argo data and Levitus data. It is shown that, the current field computed from Argo data, which shows the Kuroshio and eddies clearly, is similar to that obtained from altimetric data, except that the current speed is less in the former case than in the latter one; this may be related to the scarcity and uneven distribution of Argo floats, the subsequent interpolation and extrapolation errors, the shortcomings of the P-vector method, the lack of a barotropic component in the calculation or the altimetry-derived current error associated with the marine geoid, but the resultant current field is much better than that obtained from Levitus data. Moreover, Argo data have an advantage in that they can be used to derive a three-dimensional current field, whereas the altimetric data can only be used to derive the surface current field. The seasonal variations in the vertical current structure and the Kuroshio transport east of Taiwan Island are also discussed; it is found that the thickness of the northward Kuroshio is about 700 m, and the seasonal zonal variation in the main axis of the Kuroshio is obvious.
RÉSUMÉ [Traduit par la rédaction] Nous étudions les caractéristiques saisonnières des masses d'eau, la profondeur de la couche de mélange et le champ de courant à l'est du détroit de Luçon à l'aide des données des flotteurs profileurs Argo. D'après les données recueillies entre mars 2006 et novembre 2010, la relation température-salinité indique que la variation saisonnière des masses d'eau n'est pas évidente, sauf que la température de surface est plus basse et la salinité plus élevée au printemps et en hiver; les masses d'eau dans les autres couches changent peu. Nous discutons aussi de la variation saisonnière de la profondeur de la couche de mélange, qui est maximale à plus de 170 m en hiver et minimale à environ 25 m en été. En nous basant sur la méthode des vecteurs P, nous utilisons les données Argo et les données Levitus pluriannuelles de moyennes saisonnières pour calculer les champs de courant respectifs. Nous comparons le courant géostrophique de surface déduit des données altimétriques avec ceux calculés à l'aide des données Argo et des données Levitus. Il apparait que le champ de courant calculé d'après les données Argo, qui montre clairement le Kuroshio et les remous, est semblable à celui obtenu des données altimétriques, sauf que la vitesse du courant est moindre dans le premier cas que dans le dernier; cela peut être lié à la rareté et à la distribution inégale des flotteurs Argo, aux erreurs subséquentes d'interpolation et d'extrapolation, aux faiblesses de la méthode des vecteurs P, au manque d'une composante barotropique dans les calculs ou à l'erreur dans le courant déduit des données altimétriques associée au géoïde marin, mais le champ de courant résultant est bien meilleur que celui obtenu à l'aide des données Levitus. De plus, les données Argo présentent l'avantage de pouvoir être utilisées pour déduire un champ de courant tridimensionnel, alors que les données altimétriques ne permettent que de déduire le champ de courant de surface. Nous discutons aussi des variations saisonnières dans la structure verticale du courant et dans le transport par le Kuroshio à l'est de l’île de Taïwan; nous trouvons que la profondeur du Kuroshio circulant vers le nord est d'environ 700 m et la variation saisonnière zonale dans l'axe principal du Kuroshio est évidente.
1 Introduction
The Argo project is a major component of the oceanic observational system which began in 2000. Up to January 2011, 7407 floats, including 3079 active floats, have been deployed by 33 countries and regions in the world's oceans, with the exception of the Arctic Ocean (http://www.argo.gov.cn/argo-china/index.asp). Generally, each Argo float works as follows: first, it dives to a prescribed drifting depth of about 1000 m; after drifting for approximately 8–10 days, it then descends to an observational depth of 2000 m; in due time, it ascends to the sea surface and measures temperature and salinity during its ascent. When the float reaches the sea surface, the data are transmitted to satellites and then received by users. Each cycle lasts about 10 days (http://www.argo.org.cn/english/about_argo/about02.html).
The Argo program enables continuous real-time observations of the surface and lower layers (above 2000 m) of the global ocean, producing a large amount of high-quality, high-resolution temperature and salinity profile data with broad coverage, which is also a good supplement to satellite observations of the sea surface. The program offers new opportunities to study the world's oceans and climate. Argo data are widely used and play an important role in the study of air–sea interactions (Bhaskar, Swain, & Ravichandran, Citation2007; Ramesh & Krishnan, Citation2005), changes in global upper-ocean characteristics (Freeland & Cummins, Citation2005; Schiller, Wijffels, & Meyers, Citation2004), the quantitative description of marine physical conditions (Guinehut, Le Traon, Larnicol, & Philipps, Citation2004), climate and ocean variability and prediction (Menna & Poulain, Citation2009; Roemmich & Gilson, Citation2009), data assimilation and model development (Acreman & Jeffery, Citation2007; Forget, Mercier, & Ferron, Citation2008; Guinehut, Larnicol, & Le Traon, Citation2002), measurement of sea surface topography and so on (Chang, Rosati, & Vecchi, Citation2010; Leuliette & Miller, Citation2009; Roemmich et al., Citation2009; Willis, Chambers, & Nerem, Citation2008).
The northwestern Pacific Ocean is affected by the Kuroshio. Many Argo profiling floats are deployed in this area to help further the understanding of ocean dynamics, and the observational data retrieved are widely used. For example, Liu, Xu, Zhu, Sun, and Zhang (Citation2006) analyzed the upper oceanic response to tropical cyclones in the northwestern Pacific at different times, and suggested that tropical cyclones caused the deepening of the mixed-layer depth (MLD), the cooling of the mixed-layer temperature and the freshening of the mixed-layer salinity. Xu, Liu, Sun, and Zhu (Citation2006) and Sun, Xu, Liu, Tong, and Zhu (Citation2008) analyzed the water masses in the northwest Pacific Ocean, which are categorized into eight types (i.e., North Pacific Tropical Surface Water, North Pacific Subsurface Water, North Pacific Intermediate Water, North Pacific Subtropical Water, North Pacific Deep Water, Equatorial Surface Water, South Pacific Subsurface Water and South Pacific Intermediate Water).
The current field east of Luzon Strait is also studied extensively using Argo data; for example, Centurioni, Niiler, and Lee (Citation2004) demonstrated that a branch of the Kuroshio intruded far into the South China Sea (SCS) from the Philippine Sea through Luzon Strait only between October and January, while in other seasons, the Kuroshio branch looped across the strait and exited along the south coast of Taiwan Island. Xie, Zhu, Xu, and Guo (Citation2005) evaluated the mid-depth currents using reanalysis data from the National Centers for Environmental Prediction (NCEP) in the tropical Pacific using Argo floats, and showed that the current determined using NCEP reanalysis data was smaller than the current determined from Argo data. Wang, Liu, and Xu (Citation2006) studied the seasonal variations in the Pacific warm pool, the equatorial currents and the west boundary currents using Argo data with a resolution of 3°. Using drifter trajectory data, both Hu, Xiong, Qiao, Guo, and Lin (Citation2008) and Ma, Wu, and Lin (Citation2009) showed that the Kuroshio intrusion into the SCS only occurred in fall and winter; Hu et al. (Citation2008) also suggested that, east of Taiwan Island, the Kuroshio turned cyclonically in spring when no eddy existed, but it appeared as an almost straight line in other seasons. However, only the surface current field was discussed in their studies.
The magnitude of the volume transport is one of the most important and evident indices characterizing the variations of the Kuroshio. The region east of Taiwan Island is very important in monitoring the transport and variation of the Kuroshio. Chu (Citation1976) showed that the Kuroshio transport along the Taiwan–Ishigakijima section was about 33.4 Sv (where 1 Sv = 106 m3 s−1) in fall, 29.6 Sv in spring and 25.0 Sv in summer, with a reference level at 800 × 104 Pa, while Johns et al. (Citation2001) showed that the annual mean Kuroshio transport along this section was about only 21.5 Sv with an uncertainty of ±2.5 Sv. Yuan et al. (Citation1998) suggested that the Kuroshio transport south of this section was about 30 Sv in early summer, but Gilson and Roemmich (Citation2002) suggested that the annual mean Kuroshio transport along this section was only about 22 ± 1.5 Sv. Note that these estimates of the Kuroshio transport depend greatly on the sampling resolution and the distribution of in situ observational stations.
Our study examines several questions. With more and more Argo data being obtained in the northwestern Pacific Ocean, can the seasonal variation in the hydrological parameters be understood? Can the three-dimensional seasonal current fields here be well reconstructed? Is there any seasonal variation of the Kuroshio transport east of Taiwan Island?
To answer the questions above, the seasonal characteristics of water masses and the MLD east of Luzon Strait are studied using Argo profiling float data from 2006 to 2010. Moreover, based on the P-vector method, multi-year seasonal mean Argo data and Levitus data are used to calculate the respective current fields, and each result is compared with the current field obtained using altimetric data. The data and methods are presented in Section 2; the results and discussion are presented in Section 3, and Section 4 presents the conclusions.
2 Data and methods
a Data
The data used in this study are the observational temperature and salinity profiles from Argo floats within the northwest Pacific Ocean (121°–127.5°E, 17°–25°N) from March 2006 to November 2010. shows the distribution of the Argo floats.
Fig. 1 Seasonal distribution of Argo profiling floats from 2006 to 2010 in (a) spring (the black line shows the Taiwan–Ishigakijima section and the star denotes Ishigakijima island), (b) summer, (c) fall and (d) winter.
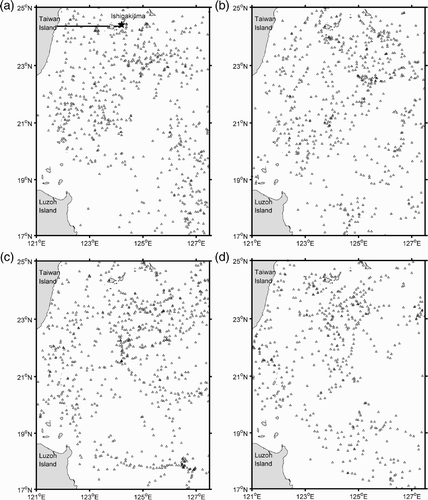
One type of altimetric product, the gridded absolute geostrophic velocity from multi-satellite measurements, is provided by Archiving, Validation and Interpretation of Satellite Oceanographic data (Aviso), and has a spatial resolution of 1/3° and a temporal resolution of 7 days (Le Traon, Dibarboure, & Ducet, Citation2001). The Aviso products are widely used to describe the surface circulation in oceans and marginal seas (e.g., Cai & He, Citation2010; Liu, Weisberg, & Yuan, Citation2008). The absolute geostrophic current velocity computed using sea surface height (SSH) from altimetric data only represents the surface current field. In this study, the absolute geostrophic current velocity from March 2006 to November 2010 is extracted, and its seasonal mean is computed for further comparison with those computed using different data. Moreover, spring, summer, fall and winter are defined to be from March to May, June to August, September to November and December to the following February, respectively.
Furthermore, the Levitus data (1/4° × 1/4°; Boyer et al., Citation2005), the 5-minute gridded global elevation/bathymetry data (ETOPO5) and the QuickSCAT monthly mean wind velocity (1/4° × 1/4°) from the Asia-Pacific Data-Research Center (APDRC) are also employed in the study. shows the multi-year seasonal mean wind fields.
b P-Vector Method
Chu (Citation1995) developed a P-vector method to determine the absolute current velocity from hydrographic data. Chu and Li (Citation2000) further developed the P-vector method on the isopycnal surface based on the conservation of both potential density and potential vorticity. With the assumptions of geostrophic balance, mass conservation and no major cross-isopycnal mixing, the potential density equation can be obtained. By differentiating the potential density, a potential vorticity equation can be obtained according to the geostrophic and hydrostatic balance. The P-vector method should obey the following conditions: (1) the isopycnal surface and the isopotential vorticity surface cannot be parallel, and (2) the current velocity deflects with depth. The main disadvantage of the P-vector method is that it cannot be applied to the computation of the current field at the sea surface, sea bottom, lateral boundary or in shallow water. Because the water depth in the area studied is deeper than 3000 m, the P-vector method can be applied. The P-vector method was applied extensively, for example, in the Atlantic Ocean, the Pacific Ocean, the South China Sea and in other locations (Bu, Yuan, & Liu, Citation2001; Cai, Long, Chen, & Hong, Citation2004; Chu, Citation1995; Wang et al., Citation2006). The controlling equations (Chu, Citation1995) are,
c Ekman Drift Current
The geostrophic current computed using Argo data is different from the actual oceanic current. In order to obtain a current field as close to reality as possible, the wind-driven current should be taken into account. The current field obtained using the P-vector method with Argo data is combined with the wind-driven current field to calculate the Kuroshio transport. The QuickSCAT monthly mean wind data are used to compute the Ekman drift current using the following equations,
3 Results and discussion
a Seasonal Variations in Water Masses
In this study, a T-S diagram is used to analyze the seasonal characteristics of the water masses in the study area from March 2006 to November 2010.
shows that the seasonal T-S diagram in the study area is a reverse “S” in shape, which means there are four water masses, that is, high temperature and low salinity water at the surface, cooler temperature and high salinity water in the subsurface layer, cool temperature and low salinity water in the intermediate layer and cold temperature and higher salinity water in the deep layer. The T-S diagram is consistent with the results of Xu et al. (Citation2006); however, there are some differences, for example, according to the least squares curves (red) in , the turning point of the cooler temperature and high salinity water in the subsurface layer is at a temperature of about 21°C and a salinity of about 34.82 (practical salinity scale used), which is different from the turning point in Xu et al. (Citation2006) at a temperature of 24°C and a salinity higher than 35. This may be caused by the use of different Argo time series data in each study. Moreover, the turning point of the low temperature and higher salinity water in the deep layer occurs at a temperature of about 7°C and a salinity of about 34.3.
Fig. 3 T-S diagram from Argo data in the northwestern Pacific Ocean from 2006 to 2010 in (a) spring, (b) summer, (c) fall and (d) winter. The curves in red are fitted using the least squares method. The lines of constant density are shown in the figure.
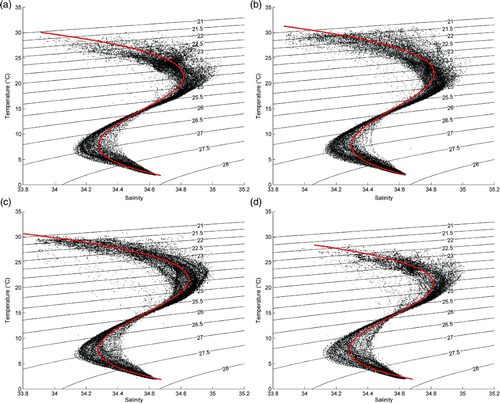
Seasonal variations in temperature and salinity are obvious in the surface and subsurface layers when compared with those in the intermediate and deep layers. In the surface layer, the temperature and salinity in spring and winter are about 26°C and 34.5, respectively, while they are 29°C and 34.3 in summer and fall. The lower temperature and higher salinity of the surface layer in spring and winter may be related to solar radiation and precipitation.
b Seasonal Variation in the MLD
There are several definitions used for the MLD, but two definitions are used commonly. One is the T-S method which defines the MLD as the depth of the seasonal thermocline where the temperature is 0.5°C lower than the sea surface temperature; the other is the Vertical Gradient method, which is based on the vertical gradient of the water density (temperature) in the upper layer, and the MLD is the depth at which the vertical density gradient (temperature) exceeds a threshold value (Cai & Gan, Citation2000; Zhang, Wu, & Lin, Citation2006). The second method is used in this paper, and we define the MLD as the depth at which the temperature gradient exceeds 0.05°C m−1.
The seasonal variations in the MLD are shown in and . In spring, the mean MLD in the region is about 81 m (a), and it decreases gradually from north to south. In the north, the isolines of MLD form semi-closed loops with a maximum of 118 m at the centre, while in the south, the isolines of MLD form two closed loops with a minimum of 29 m at the centre of the southern loop. As can be seen from the current field shown in a, the closed loop with a larger extremum of 90 m, corresponds to an anticyclonic eddy, while the other closed loop, with a smaller extremum of 30 m, corresponds to a cyclonic eddy. This suggests that a better geostrophic adjustment relation exists between the MLD and the upper oceanic circulation structure. The MLD is affected by several factors such as wind stress, solar insolation and current shears (Bhaskar et al., Citation2007). In spring, because of increased solar radiation, the wind weakens and the northeast wind changes to a southeast one, thus the MLD here becomes shallower with time, with a mean value of about 30 m.
Table 1. Seasonal mean MLD for 2006–10.
Fig. 4 Distribution of the multi-year seasonal mean MLD (units are metres) in (a) spring, (b) summer, (c) fall and (d) winter.
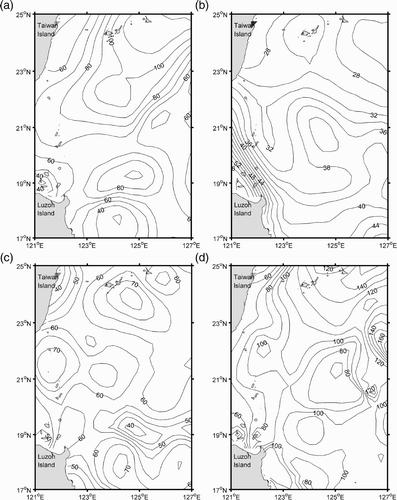
Fig. 5 Current field in spring. (a) current field at 10 m computed from Argo data, (b) current field at 200 m computed from Argo data, (c) geostrophic current field extracted from altimetric data, and (d) current field at 10 m computed from Levitus data.
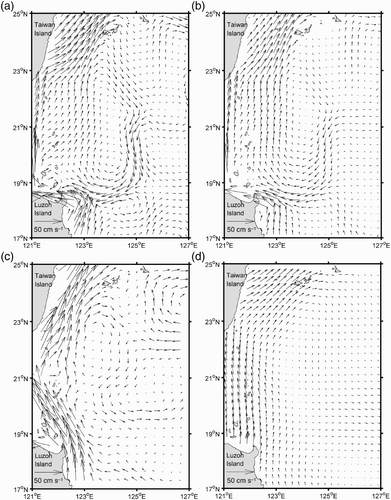
In summer, the MLD is at a minimum with a mean value of about 33 m (b). The MLD gradually decreases from south to north; the opposite of what happens in the spring. In Luzon Strait, the isolines of the MLD are crowded and parallel to the Kuroshio, with a maximum of 59 m in the west. The isolines of the MLD are sparse in the north, with a minimum of 25 m. In the central region, the isolines of the MLD are closed, with an extremum of 38 m, which corresponds to an anticyclonic eddy (a). In summer, this region is affected by the hot, wet and weak south wind, and because of the large amount of precipitation and intense solar radiation, the MLD is the shallowest in this season.
As fall approaches, the MLD begins to deepen with a mean value of about 62 m, a maximum of 77 m and a minimum of 35 m (c). The MLD is deep in the north, south and in Luzon Strait, where the isolines form multi-closed loops. The two closed loops in the south, one with an extremum of 70 m and the other with an extremum of 40 m, correspond to the anticyclonic and cyclonic eddy dipole shown in the current field (a). During this season, the wind becomes stronger, and the precipitation and solar radiation decrease, thus the MLD deepens.
In winter, the MLD reaches a maximum with a mean value of about 101 m, a maximum of 179 m and a minimum of 49 m (d). The MLD is deeper in the east with a steeper gradient than in the west. The closed loop with an extremum of 70 m east of Luzon Strait corresponds to a cyclonic eddy as shown in the current field (a). It seems that the stronger winds in winter strengthen the mixing.
According to and , a distinct seasonal variation in the MLD exists in the study area; the MLD deepens from summer to winter and begins to shallow in the spring. The seasonal variation in the MLD east of Luzon Strait is basically consistent with that in the South China Sea (Sun, Liu, & Jia, Citation2007). The maximum MLD occurring in winter and the minimum occurring in summer is also consistent with the results of Ohno, Kobayashi, Iwasaka, and Sato (Citation2004) for the northwest Pacific Ocean, although there are some differences in values.
also shows the seasonal mean MLD in 2006, which is deeper in spring, fall and winter and shallower in summer than the seasonal mean for 2006–10. This suggests that the resulting seasonal mean MLD depends largely on which Argo time series data are used.
c Seasonal Variations in the Current Field
Here the P-vector method is used to compute the seasonal current field. According to the ETOPO5 topography data and the distribution of Argo profiling floats shown in . Argo data are checked to determine whether there are any anomalous data or missing data. Because missing data occur mainly in the deep ocean where the water temperature and salinity are steady, they could be replaced by Levitus data. The water column is divided into 43 levels in the vertical: 10, 20, 30, 40, 50, 60, 70, 80, 90, 100, 120, 150, 180, 200, 250, 275, 300, 350, 400, 450, 500, 550, 600, 650, 700, 750, 800, 850, 900, 950, 1000, 1050, 1100, 1150, 1200, 1300, 1400, 1500, 1600, 1700, 1800, 1900 and 2000 m, respectively. The temperature and salinity in each layer are interpolated/extrapolated on a rectangular domain with a grid resolution of 1/4°. Meanwhile, to reduce the bottom topographic effect, the zero-velocity reference level selected is 2500 m.
Because the current obtained using altimetric data only reflects the structure of the surface current, the current derived from the altimetric data is compared with the geostrophic currents at 10 m obtained using Argo data and Levitus data, respectively.
In spring, the current field at 10 m computed using Argo data is shown in a. East of Luzon Strait, there is a large-scale anticyclonic eddy which covers a domain within 18.5°–24°N, 122.7°–125°E with its centre at about 19.2°N, 124°E. East of this eddy, there is a cyclonic meander; south of this eddy, the current field is complicated with a cyclonic meander in the west and an anticyclonic one in the east; northeast of this eddy, there is also an anticyclonic meander. The northward western boundary current (i.e., the Kuroshio) along the east of Taiwan Island is strong, while it is relatively weak in southern Luzon Strait. The current speed decreases gradually with depth. A similar current pattern appears at 200 m (b), but the meanders and eddies disappear at 500 m. c shows the geostrophic current extracted using altimetric data. The Kuroshio is very distinct, with a current speed of about 50 cm s−1. East of Luzon Strait, there is an anticyclonic eddy, which covers a domain within 1°–24°N, 122.5°–124.5°E with its centre at about 22°N, 123.5°E. East of the eddy, there is a cyclonic meander. In the northeast, there is also a strong anticyclonic meander with a speed of about 15 cm s−1. Comparing a with c, the current pattern calculated using Argo data is similar to that obtained using altimetric data; however, the current speed in the whole domain obtained using Argo data is smaller, and the Kuroshio is not as evident in southern Luzon Strait. The current field at 10 m computed using Levitus data is shown in d. Although the Kuroshio is distinct, the current speed is also very small. In the rest of the domain, the current field is much weaker and different from those computed using Argo data and altimetric data.
In summer, the current field at 10 m computed using Argo data is shown in a. There are two cyclonic eddies and an anticyclonic eddy, with their centres at 24.2°N, 125.8°E; 18.8°N, 126°E; and 22°N, 123.5°E, respectively. In addition, there is an anticyclonic meander near Luzon Island. The current speed of the Kuroshio in Luzon Strait is high, but it decreases near the east of Luzon Island and to the northeast of Taiwan Island where Argo floats are sparse. The Kuroshio, and the anticyclonic and cyclonic eddies which appeared at the surface, are also evident at 200 m depth (b), but the cyclonic eddy and the anticyclonic meander in the south are weaker. In the geostrophic current field extracted using altimetric data (c), the current speed of the Kuroshio exceeds 50 cm s−1, but the current speed in the rest of the domain is low. A westward current seems to separate the study region into two parts; the northern part is dominated by an anticyclonic eddy with its centre at 21.2°N, 123.5°E) while in the southern part, there is a cyclonic eddy with its centre at 18.5°N, 125°E. The current structure in a agrees well with that in c, except that the positions of the eddies are somewhat different. In d, the Kuroshio obtained using Levitus data is weak but distinct; however, the eddies east of the Kuroshio are not evident.
In fall, there are several eddies in the current field at 10 m computed using Argo data (a). Several features can be seen from north to south: an anticyclonic eddy which covers a domain within 21.5°–25°N, 125°–127°E with its centre at 22.9°N, 126.7°E), a weak anticyclonic eddy centred at 21.5°N, 123.5°E, a strong cyclonic eddy centred at 19.2°N, 124.5°E, and an anticyclonic eddy centred at 17.7°N, 126.5°E, respectively. The current pattern remains thus until 200 m depth (b). The geostrophic current field (c) obtained using altimetric data agrees well with that obtained using Argo data (a); for example, there are also an anticyclonic eddy centred at 21.5°N, 123°E, a cyclonic eddy at 19°N, 124.5°E and an anticyclonic eddy centred at 17.8°N, 126.5°E; furthermore, a weak cyclonic eddy and an anticyclonic meander are located in the north of the study region. The major differences between a and c is that the current speeds of the Kuroshio are different and the scales of the eddies and meanders are different. The current field obtained using Levitus data is shown in d, where only the Kuroshio is evident, and the speed of the current is lower over the entire region.
In winter, the current field at 10 m computed from Argo data is shown in a. A cyclonic eddy is located at the centre of the region, covering a domain within 19.5°–22.5°N, 123°–126°E centred at about 21°N, 124°E with a maximum current speed of about 25 cm s−1. In addition, northeast of Luzon Island, there is an anticyclonic eddy. East of Luzon Island, there is a cyclonic meander in the west and an anticyclonic eddy, centred at 18°N, 126°E, in the east. The current is weaker at 200 m depth (b); however, the Kuroshio and the cyclonic eddy are still distinct. The current field obtained from altimetric data is shown in c. Except for the northward flowing Kuroshio and the anticyclonic meander in the northeast, the rest of the domain is mainly dominated by a large anticyclonic circulation. Comparing a with c, it can be seen that the current fields are very different, which may be related to the sparse and uneven distribution of Argo float data during this season (d). The current field (d) obtained from Levitus data is similar to that in spring.
Except for winter, the seasonal current fields calculated from Argo data agree well with those obtained from altimetric data; however, the resultant current speed from the former is lower than the speed from the latter; this may be related to the sparse and uneven distribution of Argo profiling floats, the subsequent interpolation and extrapolation errosr and/or the lack of a barotropic component in the calculation using the P-vector method. In a to 8a, there is a strong divergence near the northwest corner of Luzon Island; this strong divergence area is situated in the area where Argo floats are very scarce (see ), especially in spring and winter. There are no Argo floats near the northwest corner of Luzon Island. Because the computed current field near the northwest corner of Luzon Island is based on extrapolations from distant Argo data, this leads to a false velocity field with strong divergence. On the other hand, altimetric data are not accurate in shallow water or on the continental slope, and the current derived from altimetric data may be affected by the marine geoid error (Smith, Citation2010). In fact, the current structure calculated from Argo data can give us an estimation of the three-dimensional current field as discussed in the following, which is not available using altimetric data. The current fields in different seasons computed from Levitus data are mainly shown as large anticyclonic meanders, with a north-northwestward Kuroshio in Luzon Strait, the results are distinctly worse than those using Argo data or altimetric data. This indicates that the climatological Levitus temperature and salinity data cannot reflect the detailed characteristics of the current field.
d Seasonal Variations in the Vertical Current Structure and Transport of the Kuroshio East of Taiwan Island
In the above, the current field computed from Argo data does not consider the Ekman drift current. In order to approach the true sea surface current field, the Ekman drift current should be taken into account in calculating the transport of the Kuroshio. According to Eq. (5), the Ekman depth is about 70 m in this region. Thus, the Ekman drift currents at four vertical levels (10, 20, 30 and 50 m) are computed, and they are combined with the current computed using the P-vector method based on Argo data to estimate the Kuroshio transport east of Taiwan Island. A section along 121.5°–123.5°E at 24.3°N, as shown by the black line in a, is chosen for the study. The vertical distribution of the combined seasonal longitudinal current obtained from Argo data with the Ekman drift current along this section is shown in . The maximum northward current speed of the Kuroshio in spring (a) is over 40 cm s−1; it decreases with depth and is about zero at 700 m, then the current below this depth flows southward at less than 3 cm s−1. The vertical distribution of the southward current below 700 m changes slightly in other seasons. However, some distinct differences exist in the upper 700 m; for example, the main flow axis of the Kuroshio is in the east in spring, while it moves to the west in fall. In fact, the vertical distribution of the northward Kuroshio current speed contour in is very similar to that obtained by Johns et al. (Citation2001), except that our resultant surface maximum Kuroshio speed is only about 40 cm s−1, which is much lower than the value of over 80 cm s−1 obtained by Johns et al. (Citation2001). Another possible reason for our underestimation of the transport in the strong western boundary current domain is that some important factors, such as the non-linear effect and the western intensification, are ignored in the P-vector method. As shown earlier, the near-surface currents from the P-vector method (b, 6b, 7b and 8b) are weaker than the altimetric geostrophic currents (c, 6c, 7c and 8c). In the future, we could follow Han and Tang (Citation2001) and Han et al. (Citation2010) to improve the estimation of the Kuroshio transport, by combining altimetric surface currents with Argo data.
Fig. 9 Vertical distribution of the combined seasonal longitudinal current (obtained from the current derived from Argo data with the Ekman drift current) speed contour along the section east of Taiwan Island shown in a (units cm s−1; the black contours denote the northward current while the grey contours denote the southward current) in (a) spring, (b) summer, (c) fall and (d) winter.
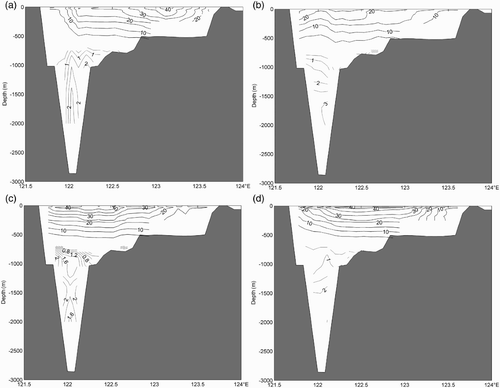
According to , the maximum northward transport of the Kuroshio is about 17.9 Sv in fall; the minimum is 11.5 Sv in summer, and the transport in winter is larger than that in spring. In fact, the calculated northward Ekman transport is not significant, with values of 0.19 Sv in spring, 0.12 Sv in summer, 0.16 Sv in fall and 0.28 Sv in winter. also shows the seasonal Kuroshio transport along the Taiwan–Ishigakijima section from Chu (Citation1976) and the Kuroshio transport south of this section in summer from Yuan et al. (Citation1998). Note that, the resultant annual mean Kuroshio transport of about 20.7–22.1 Sv from Johns et al. (Citation2001) is much less than the resultant seasonal Kuroshio transport from Chu (Citation1976). Similarly, the resultant annual mean Kuroshio transport south of the above section by Gilson and Roemmich (Citation2002) is much less than the resultant Kuroshio transport in summer from Yuan et al. (Citation1998). The basic trend in our resultant seasonal variation in the transport is similar to those obtained using observational current data, except that the results from previous studies are much larger. The reasons for the difference may be related to the sparse distribution of Argo profiling floats, the subsequent interpolation/extrapolation error and the shortcomings of the P-vector method in the strong western boundary current domain; meanwhile, the estimations of the Kuroshio transport depend greatly on the sampling resolution and the distribution of in situ observational stations, as suggested by the comparison with previous studies; furthermore, the frequent mesoscale eddy activity in this region may also affect the estimation of the Kuroshio transport (Yuan et al. Citation1998; Zhang, Lee, Johns, & Liu, Citation2001).
Table 2. Seasonal variations of the Kuroshio transport east of Taiwan Island.
4 Conclusions
In this paper, Argo profiling float data from March 2006 to November 2010 are used to study the seasonal characteristics of the water masses and the seasonal mean variations in the MLD east of Luzon Strait. Furthermore, based on the P-vector method, multi-year seasonal mean Argo data and Levitus data are used to calculate the respective current fields. The results are analyzed and some conclusions can be drawn.
First, the seasonal variation in the water masses is not obvious, especially in the intermediate and deep layers. At the surface and in the subsurface layer, the water temperature is lower and the salinity is higher in spring and winter than in summer and fall. While the seasonal variations in the MLD is evident, it is deepest at about 179 m in winter and shallowest at about 25 m in summer. This may be related to the variation in factors such as the prevailing wind, precipitation or solar radiation.
Second, the seasonal geostrophic current field calculated from Argo data using the P-vector method is compared with that extracted from altimetric data. In the study region, the eddies and the Kuroshio are evident in the current field calculated from Argo data. Other than in winter, the current pattern computed from Argo data agrees well with that computed from altimetric data; however, the current speed from the former is relatively smaller. This may be related to the sparse and uneven distribution of Argo profiling floats, the subsequent data interpolation/extrapolation error, the shortcomings of the P-vector method in the strong western boundary current domain or the marine geoid error in the current derived from altimetric data. However, the basic current pattern could not be reproduced from Levitus data, which demonstrates that the Argo data would be sufficient to reproduce the basic current pattern if more Argo data were available.
The vertical current structure east of Taiwan Island was also investigated; it was found that the thickness of the northward Kuroshio is about 700 m and the seasonal eastward/westward variation of the Kuroshio main axis is obvious; below 700 m, the southward current speed is lower. The seasonal variation in the Kuroshio transport east of Taiwan Island is also obvious, with a minimum of about 11.4 Sv in summer and a maximum of about 17.8 Sv in fall.
The Argo program is important in improving our understanding of ocean dynamics. With more and more Argo floats deployed, Argo data, together with remotely sensed data and other in situ observational data, could be widely used to investigate the oceanic spatio-temporal characteristics of the temperature, salinity and current field using data assimilation technology and numerical simulation.
Acknowledgements
This work is jointly supported by the National Basic Research Program (No. 2007CB816003), National Science Foundation Council (NSFC) Grants No. 40976009, No. 41025019 and No. 41206009. The altimetric products were produced by Ssalto/Duacs and distributed by Aviso, with support from the Centre National d'Etudes Spatiales (CNES). QuikScat data are produced by Remote Sensing Systems and sponsored by the NASA Ocean Vector Winds Science Team. Data are available at http://apdrc.soest.hawaii.edu/.
References
- Acreman , D. M. and Jeffery , C. D. 2007 . The use of Argo for validation and tuning of mixed layer models . Ocean Modelling , 19 ( 1–2 ) : 53 – 69 . (doi:10.1016/j.ocemod.2007.06.005)
- Bhaskar , T. V. S. U. , Swain , D. and Ravichandran , M. 2007 . Mixed layer variability in Northern Arabian Sea as detected by an Argo float . Ocean Science Journal , 42 ( 4 ) : 241 – 246 . (doi:10.1007/BF03020915)
- Boyer , T. , Levitus , S. , Garcia , H. , Locarnini , R. , Stephens , C. and Antonov , J. 2005 . Objective analyses of annual, seasonal, and monthly temperature and salinity for the world ocean on a 1/4 degree grid . International Journal of Climatology , 25 : 931 – 945 . (doi:10.1002/joc.1173)
- Bu , X. , Yuan , Y. and Liu , Y. 2001 . Application of P vector method to the diagnostic calculation of circulation in the South China Sea in summer . Acta Oceanologica Sinica , 23 ( 3 ) : 8 – 17 .
- Cai , S. and Gan , Z. 2000 . The application of a three-dimensional baroclinic shelf sea model: The seasonal variation of the South China Sea upper mixed layer . Acta Oceanologica Sinica , 3 : 7 – 14 .
- Cai , S. and He , Y. 2010 . Association of the Sulu Sea surface circulation with the South China Sea . Journal of Marine Systems , 81 ( 4 ) : 335 – 340 . (doi:10.1016/j.jmarsys.2010.02.010)
- Cai , S. , Long , X. , Chen , R. and Hong , Q. 2004 . A study on circulation structure in spring in Nansha Islands Sea area, South China Sea . Journal of Tropical Oceanography , 23 ( 2 ) : 37 – 44 .
- Centurioni , L. R. , Niiler , P. P. and Lee , D. K. 2004 . Observations of inflow of Philippine Sea surface water into the South China Sea through the Luzon Strait . Journal of Physical Oceanography , 34 : 113 – 121 . (doi:10.1175/1520-0485(2004)034<0113:OOIOPS>2.0.CO;2)
- Chang , Y. S. , Rosati , A. J. and Vecchi , G. A. 2010 . Basin patterns of global sea level changes for 2004–2007 . Journal of Marine Systems , 80 : 115 – 124 . (doi:10.1016/j.jmarsys.2009.11.003)
- Chu , P. C. 1995 . P-vector method for determining absolute velocity from hydrographic data . Marine Technology Society Journal , 29 ( 2 ) : 3 – 14 .
- Chu , P. C. and Li , R. 2000 . South China Sea isopycnal-surface circulation . Journal of Physical Oceanography , 30 ( 9 ) : 2419 – 2438 . (doi:10.1175/1520-0485(2000)030<2419:SCSISC>2.0.CO;2)
- Chu , T.-Y. 1976 . Study of the Kuroshio Current between Taiwan and Ishigakijima . Acta Oceanographica Taiwanica , 6 : 1 – 24 .
- Forget , G. , Mercier , H. and Ferron , B. 2008 . Combining Argo profiles with a general circulation model in the North Atlantic. Part 2: Realistic transports and improved hydrography, between spring 2002 and spring 2003 . Ocean Modelling , 20 ( 1 ) : 17 – 34 . (doi:10.1016/j.ocemod.2007.06.002)
- Freeland , H. J. and Cummins , P. F. 2005 . Argo: A new tool for environmental monitoring and assessment of the world's oceans, an example from the NE Pacific . Progress in Oceanography , 64 ( 1 ) : 31 – 44 . (doi:10.1016/j.pocean.2004.11.002)
- Gilson , J. and Roemmich , D. 2002 . Mean and temporal variability in Kuroshio geostrophic transport south of Taiwan (1993–2001) . Journal of Oceanography , 58 : 183 – 195 . (doi:10.1023/A:1015841120927)
- Guinehut , S. , Larnicol , G. and Le Traon , P. Y. 2002 . Design of an array of profiling floats in the North Atlantic from model simulations . Journal of Marine Systems , 35 ( 1–2 ) : 1 – 9 . (doi:10.1016/S0924-7963(02)00042-8)
- Guinehut , S. , Le Traon , P. Y. , Larnicol , G. and Philipps , S. 2004 . Combining Argo and remote-sensing data to estimate the ocean three-dimensional temperature fields—a first approach based on simulated observations . Journal of Marine Systems , 46 ( 1–4 ) : 85 – 98 . (doi:10.1016/j.jmarsys.2003.11.022)
- Han , G. , Ohashi , K. , Chen , N. , Myers , P. G. , Nunes , N. and Fischer , J. 2010 . Decline and partial rebound of the Labrador Current 1993–2004: Monitoring ocean currents from altimetric and CTD data . Journal of Geophysical Research , 115 : C12012 doi:10.1029/2009JC006091
- Han , G. and Tang , C. L. 2001 . Interannual variation of volume transport in the western Labrador Sea based on TOPEX/Poseidon and WOCE data . Journal of Physical Oceanography , 31 : 199 – 211 . (doi:10.1175/1520-0485(2001)031<0199:IVOVTI>2.0.CO;2)
- Hu , X. , Xiong , X. , Qiao , F. , Guo , B. and Lin , X. 2008 . Surface current field and seasonal varibility in the Kuroshio and adjacent regions derived from satellite tracked drifter data . Acta Oceanologica Sinica , 30 ( 6 ) : 1 – 16 . (in Chinese)
- Johns , W. E. , Lee , T. N. , Zhang , D. , Zantopp , R. , Liu , C. T. and Yang , Y. 2001 . The Kuroshio east of Taiwan: Moored transport observations from the WOCE PCM-1 array . Journal of Physical Oceanography , 31 : 1031 – 1053 . (doi:10.1175/1520-0485(2001)031<1031:TKEOTM>2.0.CO;2)
- Le Traon , P. , Dibarboure , G. and Ducet , N. 2001 . Use of a high-resolution model to analyze the mapping capabilities of multiple-altimeter missions . Journal of Atmospheric and Oceanic Technology , 18 ( 7 ) : 1277 – 1288 . (doi:10.1175/1520-0426(2001)018<1277:UOAHRM>2.0.CO;2)
- Leuliette , E. W. and Miller , L. 2009 . Closing the sea level rise budget with altimetry, Argo, and GRACE . Geophysical Research Letters , 36 ( 4 ) : L04608 doi: 10.1029/2008GL036010
- Liu , Y. , Weisberg , R. H. and Yuan , Y. 2008 . Patterns of upper layer circulation variability in the South China Sea from satellite altimetry using the self-organizing map . Acta Oceanologica Sinica , 27 ( Suppl. S ) : 129 – 144 .
- Liu , Z. , Xu , J. , Zhu , B. , Sun , C. and Zhang , L. 2006 . Upper ocean response to the tropical cyclones in the northwestern Pacific during 2001–2004 by Argo data . Journal of Tropical Oceanography , 25 ( 1 ) : 1 – 8 .
- Ma , C. , Wu , D. and Lin , X. 2009 . Variability of surface velocity in the Kuroshio Current and adjacent waters derived from Argos drifter buoys and satellite altimeter data . Chinese Journal of Oceanology and Limnology , 27 ( 2 ) : 208 – 217 . (doi:10.1007/s00343-009-9260-6)
- Menna , M. and Poulain , P. M. 2009 . Mediterranean subsurface circulation estimated from Argo data in 2003–2009 . Ocean Science Discussions , 6 : 2717 – 2753 . (doi:10.5194/osd-6-2717-2009)
- Ohno , Y. , Kobayashi , T. , Iwasaka , N. and Sato , Y. 2004 . The mixed layer depth in the North Pacific as detected by the Argo floats . Geophysical Research Letters , 31 : L11306 doi:10.1029/2004GL019576
- Ramesh , K. and Krishnan , R. 2005 . Coupling of mixed layer processes and thermocline variations in the Arabian Sea . Journal of Geophysical Research , 110 ( C5 ) : C05005 doi:10.1029/2004JC002515
- Roemmich , D. and Gilson , J. 2009 . The 2004–2008 mean and annual cycle of temperature, salinity, and steric height in the global ocean from the Argo Program . Progress in Oceanography , 82 ( 2 ) : 81 – 100 . (doi:10.1016/j.pocean.2009.03.004)
- Roemmich , D. , Johnson , G. C. , Riser , S. , Davis , R. , Gilson , J. , Owens , W. B. , Garzoli , S. L. , Schmid , C. and Ignaszewski , M. 2009 . The Argo program: Observing the global ocean with profiling floats . Oceanography , 22 ( 2 ) : 34 – 43 . (doi:10.5670/oceanog.2009.36)
- Schiller , A. , Wijffels , S. E. and Meyers , G. A. 2004 . Design requirements for an Argo float array in the Indian Ocean inferred from observing system simulation experiments . Journal of Atmospheric and Oceanic Technology , 21 ( 10 ) : 1598 – 1620 . (doi:10.1175/1520-0426(2004)021<1598:DRFAAF>2.0.CO;2)
- Smith , W. 2010 . “ The marine geoid and satellite altimetry ” . In Oceanography from space Edited by: Barale , V. , Gower , J. F. R. and Alberotanza , L. (Collection of invited papers from the Oceans from Space: Venice 2010 symposium), New York: Springer
- Sun , C. , Liu , Q. and Jia , Y. 2007 . Annual and interannual variations of the mixed layer in the South China Sea . Periodical of Ocean University of China , 37 ( 2 ) : 197 – 203 .
- Sun , C. , Xu , J. , Liu , Z. , Tong , M. and Zhu , B. 2008 . Application of Argo data in the analysis of water masses in the Northwest Pacific Ocean . Marine Science Bulletin , 25 ( 3 ) : 1 – 12 .
- Wang, G., Z. Liu, & J. Xu. (2006). Three dimensional Pacific temperature, salinity and circulation reconstructions with Argo data. In Collection of Argo Application papers (in Chinese) (pp. 16–26). Beijing: China Ocean press.
- Willis , J. K. , Chambers , D. P. and Nerem , R. S. 2008 . Assessing the globally averaged sea level budget on seasonal to interannual timescales . Journal of Geophysical Research , 113 ( C6 ) : C06015 doi:10.1029/2007JC004517
- Xie , J. , Zhu , J. , Xu , L. and Guo , P. 2005 . Evaluation of mid-depth currents of NCEP reanalysis data in the tropical Pacific using Argo float position information . Advances in Atmospheric Sciences , 22 ( 5 ) : 677 – 684 . (doi:10.1007/BF02918711)
- Xu, J., Z. Liu, C. Sun, and B. Zhu. (2006). Study on the circulation and water masses in the northwest Pacific Ocean using Argo profiling floats. In Collection of Argo Application Papers (in Chinese) (pp. 14–28). Beijing: China Ocean Press.
- Yuan , Y. , Kaneko , A. , Su , J. , Zhu , X. , Liu , Y. , Gohda , N. and Chen , H. 1998 . The Kuroshio east of Taiwan and in the East China Sea and the currents east of Ryukyu Islands during early summer of 1996 . Journal of Oceanography , 54 ( 3 ) : 217 – 226 . (doi:10.1007/BF02751697)
- Zhang , D. , Lee , T. N. , Johns , W. E. and Liu , C. T. 2001 . The Kuroshio east of Taiwan: Modes of variability and relationship to interior mesoscale eddies . Journal of Physical Oceanography , 31 : 1054 – 1074 . (doi:10.1175/1520-0485(2001)031<1054:TKEOTM>2.0.CO;2)
- Zhang , Y. , Wu , D. and Lin , X. 2006 . A study of thermocline and pycnocline calculations in the East China Sea in summer . Periodical of Ocean University of China , 36 ( Sup ) : 1 – 7 .