Abstract
This paper presents a reanalysis of trends in water properties around the Gulf of Alaska. The datasets used are the lighthouse time series and the Line-P time series augmented with data from the Argo global float array. It is shown that though trends have changed, the essential story that was told 15 years ago has not changed in any meaningful way. Sea-surface temperatures are rising over a large part of the Gulf of Alaska, and sea-surface salinities are declining. Both of these lead to a decrease in surface density. We show that the temperature change extends deep into the Gulf of Alaska, and the freshening trend extends to a depth of about 100 db. Potential energy in the water column is decreasing, and the result of the decreasing potential energy and the increasing stratification implies a decrease in mid-winter mixed layer depths.
RÉSUMÉ [Traduit par la rédaction] Cet article présente une réanalyse des tendances des propriétés de l'eau dans le golfe d'Alaska. Les ensembles de données utilisés sont les séries chronologiques des phares et les séries chronologiques de la ligne P augmentés des données du réseau mondial de flotteurs Argo. Il ressort que même si les tendances ont changé, à peu près tout ce que l'on a pu dire il y a 15 ans demeure valable. Les températures de la surface de la mer augmentent dans une grande partie du golfe d'Alaska et les salinités de la surface diminuent. Ces deux facteurs entraînent une diminution de la densité de la surface. Nous montrons que le changement de température se produit jusqu’à une bonne profondeur dans le golfe d'Alaska et que la tendance à l'adoucissement s’étend jusqu’à une profondeur d'environ 100 db. L’énergie potentielle dans la colonne d'eau diminue et cette diminution, de pair avec l'accroissement de la stratification, implique une diminution des profondeurs de la couche de mélange au milieu de l'hiver.
1 Introduction
Freeland (Citation1990), hereinafter F90, showed evidence, based on daily observations of sea-surface temperature at lighthouse stations around the coast of British Columbia, that the coastal ocean was warming. Seven years later this problem was revisited by Freeland, Denman, Wong, Whitney, and Jacques (Citation1997), hereinafter F97. In F97 the warming problem was re-examined with longer time series and it was shown that though some changes had occurred the essential story remained unchanged. Trends in salinity were also examined in F97 and Ocean Station Papa was included in the analysis. It was shown that significant freshening trends existed, in addition to the warming trends, with both of these contributing to decreasing sea-surface density. Assuming that trends in deeper waters were weak this must imply steadily increasing barriers to mid-winter mixing. The F97 paper then examined the mid-winter mixed-layer depths at Ocean Station Papa and showed that indeed the mid-winter mixed layer was getting shallower at the surprisingly large rate of 58 ± 28 m per century. It was recognized that this was a very large rate of decline that simply could not be sustained for a long time. In two centuries such a trend would completely eliminate the mid-winter mixed layers, an option that must be extremely unlikely. Data from a few profiling floats that had been launched near Ocean Station Papa were also used in F97.
A detailed reanalysis of coastal stations to review the results of F90 is difficult because so many of the coastal sampling stations have been terminated. However, a significant number remain and we will re-examine three of those stations. Further, the sampling frequency along the line of oceanographic stations known as Line-P, extending from the mouth of Juan de Fuca Strait to Ocean Station Papa at 50°N and 145°W, has also decreased and that makes a direct comparison with F97 also problematic. Fortunately, a large array of floats has been maintained in the Gulf of Alaska as part of the Argo project since 2002, and this can be used to make up the recent deficits in shipboard sampling along Line-P, especially at Ocean Station Papa.
This paper will re-examine the temperature and salinity trends reported in F90 and F97. It will be shown that though the specific numbers have changed the basic story has not. The paper will then examine the variation in trends with depth and show that, with 15 years more data, the conclusions in F97 concerning the increasing stability of the water column remain valid. Finally, the time series of mixed-layer depths will be extended to the present time. We will see that a statistically significant shallowing trend remains, though the trend is smaller than the 58 m per century reported in F97.
2 British Columbia shore stations
Sea-surface temperature and salinity have been sampled daily along the Pacific coast of Canada since 1935. At a few locations in inside waters sampling began much earlier, but these stations will not be discussed here. The paper F90 reported temperature trends at 19 lighthouse stations, and since that time six of those stations have been terminated because the lighthouses have been automated. The F97 study was mainly concerned with the effects of changes on the formation of ocean mixed layers and so focussed on only three of the lighthouse stations that were well exposed to the open sea. Here we will re-examine those three stations. The 95% confidence intervals are quoted, and the method for allowing for autocorrelation in the time series is described in Masson and Cummins (Citation2007) which is itself based on the methods outlined by von Storch and Zwiers (Citation1999).
illustrates the trends observed at three lighthouse stations well exposed to the open Pacific Ocean. The F90 study only examined temperature trends but also reported trends for a large number of other stations. Other stations have also been examined recently both by this author and also as part of a paper by Cummins and Masson (unpublished manuscript). The new trends shown here are consistent with those shown by Cummins and Masson. One significant change is the rather large trend in salinity that has developed at Langara Island. When the salinity started to decline rapidly samples were obtained to check that the sampling protocols were being observed and no problem was detected. Further, the onset of a rapid decline is also occurring at another nearby light station at Bonilla Point.
Table 1. Temperature, salinity, and density trends at three lighthouse stations well exposed to the open ocean. F90 and F97 are the trends reported in the previously cited studies.
The data shown in indicate that the temperature trends shown in 1990 continued through the 1997 report without significant change and are continuing through the end of 2011. We consistently see trends towards warmer and fresher waters around the coast of British Columbia. Both warming and freshening trends contribute to decreasing surface density and so it is not surprising that the trends in density show higher significance levels than do either temperature or salinity. There is also a tendency for short-term variations in temperature to be anti-correlated with short-term variations in salinity and so are density-compensating. The result is that the density time series has a lower background noise level than do either the temperature or salinity time series. This also results in higher significance levels.
The observations of the freshening trends shown in are also broadly consistent with the trends in the Gulf of Alaska shown by Durack and Wijffels (Citation2010) and Durack, Wijffels, and Matear (Citation2012) in their global analyses.
3 Line-P trends in temperature, salinity and density
Before we examine trends along Line-P it is necessary to understand some of the history of sampling along that line. Systematic ocean observations at Ocean Station Papa itself began in 1949, but these were largely observations using mechanical bathythermographs, and the data are not very useful. In 1956 systematic sampling began using the regular trips completed by the weather ships CCGS Vancouver and CCGS Quadra between the mouth of Juan de Fuca Strait and Ocean Station Papa at 50°N and 145°W, producing surveys at approximately six-week intervals. In the early years sampling was restricted to a set of 13 stations shown by the large dots in . In 1980 the weather ship program was terminated and sampling was continued using various research vessels. As part of this change the number of stations was increased to 27 but sampling was significantly less frequent. Gradually, funding cuts have resulted in fewer and fewer surveys per year. At present, research vessels complete surveys along Line-P three times per year, typically in February, June, and September. After 1981 sampling was carried out at 27 stations of which the original 13 stations were a subset (Ocean Station Papa was designated as P26 rather than P13).
Fig. 1 This map shows the principal locations used in the paper. Amphitrite Point, Kains Island, and Langara Island are lighthouses where sea-surface temperature and salinity have been observed daily since the mid-1930s. The dots in the offshore regions show the locations of stations comprising Line-P. Station P26 is more commonly known as Ocean Station Papa.
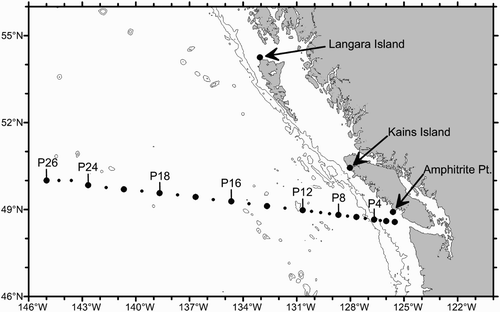
The various changes in sampling strategies are clearly evident in the upper panel of . The dots in show only when sampling has been carried out in any month/year combination at Ocean Station Papa. Some of the other stations are sampled more frequently than is P26, for example the inshore stations P1 and P2 are very well sampled in most months, and some stations, P14 being a good example, are not sampled as frequently as P26. This makes it difficult to estimate trends uniformly because the data coverage varies so much from station to station and especially from one month to the next. However, since 2001 the international Argo program has been supplying a wealth of data in the upper 2000 m of the Gulf of Alaska. For the purposes of this paper we will view the start of the Argo era in January 2002, continuing to the present time. For any particular station along Line-P we can draw a large circle around that station and accept, for any month, all Argo profiles falling inside that circle, then use those observations to interpolate onto the Line-P station using objective analysis as described by Bretherton, Davis and Fandry (Citation1976). For this particular application, data that lay within 800 km of the interpolation location were accepted into the interpolation process and interpolation was carried out using a Gaussian autocovariance function with a decay scale of 400 km. This allows us to simulate Line-P stations for every month of each year starting in 2002 as shown in the lower panel of . The data selection process provides a large range in the number of profiles within 800 km, varying from a low of 20 to a maximum of about 70. Sections of temperature and salinity anomalies have been computed and compare well with those computed directly from shipboard surveys. This interpolation process was completed for 11 of the 13 original Line-P stations only, the two neglected being the two stations closest to the coast, because including these would require extrapolating beyond the Argo array. The sampling at the two innermost stations was judged to be excellent without the assistance of Argo. All of the following results will be based on analyses at the original 13 stations only because the time series for the other stations (the small circles on ) are substantially shorter.
Fig. 2 Sampling at Ocean Station Papa (P26) in the upper panel. In the lower panel the distribution of samples as shown after the sampling along Line-P is augmented with interpolations using Argo data.
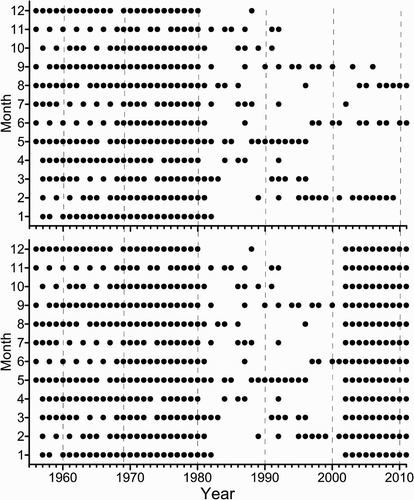
shows the distribution of trends in temperature, salinity and σt plotted against depth in the top 200 m of the water column and listed as the appropriate units per century.
The shaded bands show the 95% confidence bounds, and it is interesting that though the warming trend is persistent, in the top 100 m it is generally not significant at the 95% confidence level. The salinity trends by contrast are highly significant in the top 100 m as are the density trends. Below 100 m the density trend is smaller and not significantly different from zero; hence, we conclude that the density contrast between the surface waters and water below 100 m is increasing. Another way of stating this is that the potential energy of the water column is decreasing. It follows from this that we require an increasing work rate from mid-winter winds to produce any given mixed-layer depth. Or, if the rate of working by the winds remains unchanged, then we require that the mixed layers are getting shallower. This was the important result from the paper F97 and inevitably must result from this paper. The important remaining questions are, by how much are mixed layers changing, and how are the changes distributed?
To compute the trends in σt was computed for each month for which data exist at station P26 (i.e., at each of the dots in ). The trend was then computed for each month. The number of years for which data exist varies from one month to another; also, the amount of variability is significantly variable from one season to another. Given the observations shown in that the density trend is fairly constant between the surface and a depth of about 80 m, then decreases to zero between 80 and 100 m, the density was averaged over the top 80 m of the water column before the trends in were computed. The shaded bands again show the 95% confidence levels. We see that the trend towards decreasing density is evident in all months of the year, though not always passing the significance test. The wider confidence bands in the late summer months are primarily a result of an increase in natural variability. The plot suggests that the observed trends are significantly different from zero in 8 of the 12 months, but some of these carry very marginal significance. The trends are most clearly distinguishable from zero in the winter months, November through March, when the surface mixed layer is always deeper than the base of the layer (80 m) used for averaging the density trends in .
shows the distribution of the trend in σt versus depth and distance along Line-P. For this display data are used irrespective of month, which is sensible given the observations in that the density trends are present in each month. For this computation we used only the data from the original 13 stations, shown by the large circles in . The data from the other stations have a significantly shorter duration and in particular miss the early period of frequent sampling which is a deficit that Argo cannot correct.
Fig. 5 Density anomaly trends (kg m−3 per century) along Line-P averaged over all months of the year; the symbols above the top axis indicate the location of the stations used.
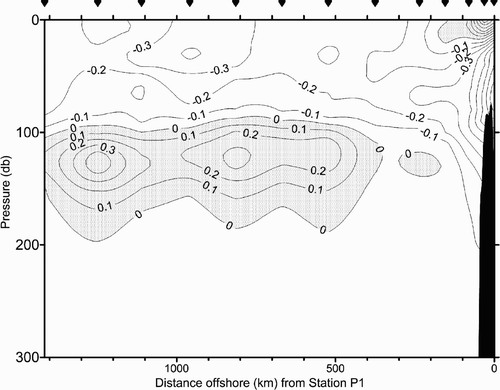
In we see essentially the same behaviour at all stations as is illustrated at Ocean Station Papa (i.e., P26) in . Thus, we conclude from our survey of trends seen by the lighthouse stations and the Line-P observations that warming and freshening are, in general, continuing along all of Line-P and in the surface waters along the outside coasts of British Columbia. The freshening, in particular, is the dominant driver towards less dense near-surface waters, but this tendency does not persist below about 100 m depth. Thus, we conclude that, in at least the bulk of the Gulf of Alaska, the density contrast between near-surface waters and deeper waters is increasing. In other words the potential energy of the water column is decreasing everywhere in the Gulf of Alaska. This is in agreement with the observations made recently by Durack and Wijffels (Citation2010) and Durack et al. (Citation2012). In the latter paper Durack et al. make a strong case for the driving force of this change being a strong intensification of the global hydrological cycle yielding freshening of surface waters at high latitudes and increasing salinity at low latitudes.
It remains now to examine the effect of these changes on the development of the mixed layers in the Gulf of Alaska and the effects on the potential energy of the water column.
4 Line-P trends in mid-winter mixed-layer depth
There are a great many papers written describing different methods of representing the continuous vertical profiles we see in the ocean as a two-layer system. For example, Li, Myers, and Freeland (Citation2005) use a method first described by Kara, Rochford, and Hurlburt (Citation2000) that claims to be an objective method. It is similar to many other methods in that it requires the specification of a threshold change that is used to detect the depth of the mixed layer. A different approach was described by Thomson and Fine (Citation2003) which uses neither a threshold nor conservation of integral properties of the water column. In this case we note that certain properties of the ocean need to be conserved when making the transition from a continuous profile to a two-layer system. Specifically, when a representative layer depth, h, is determined then the potential energy and total mass of the two-layer representation must equal that of the continuous profile. So with that in mind let us explore how we would take a continuous profile of the density anomaly σt (z) and reduce it to a two-layer system where shallow and deep values of σt , call them σs and σd , respectively, are separated by a mixed layer of depth h. All computations are made relative to a deep reference level H = 250 db.
Following Ladd and Stabeno (Citation2012) we define a parameter χ proportional to the potential energy of the water column as
If we then set these two integral values equal to the values that would be obtained in the two-layer representation then
Substituting between these two equations we obtain an expression for the mixed layer depth of
Evidently we need one more constraint to determine the mixed-layer depths from the integral constraints and, somewhat arbitrarily, we choose to set σs , the surface value of σt , equal to the average value over the top 60 m of the water column. This is selected because the depth of 60 m is about the greatest depth one can choose without ever coming close to the shallowest mixed-layer depth ever observed in winter months, which is about 90 m. This will not work well during the summer when the mixed-layer depths can become as shallow as 20 m, so in all that follows it will be assumed that this method applies only to mid-winter mixed-layer depths.
shows the results of this computation. In the upper panel the mid-winter mixed-layer depths are plotted where “mid-winter” is defined as an average over the months of November to March inclusive. As part of the computation of the mixed-layer depth an integral quantity that is proportional to the potential energy of the water column is computed and that is plotted in the lower panel. The dashed lines show the regression slopes. For the mixed-layer depth the slope indicated a shallowing trend of 15.4 ± 15.2 db per century and the potential energy–like parameter shows a declining trend of 410 ± 342 units per century. The error bars listed here are the 95% confidence levels, each of the parameters just barely being significant at that level. Both are significant at the 90% confidence level (±12.7 and ±286, respectively).
5 Discussion and summary
This paper has compared trends in temperature and salinity in several long time series around the coast of British Columbia and into the Gulf of Alaska with computations published 15 and 22 years ago. The original conclusion in earlier papers, that the waters of the Gulf of Alaska are both warming and freshening, remains valid. The temperature trend is weak and generally does not pass significance tests, but the salinity trend in the upper ocean is robust and does pass 95% significance tests. The specific trends computed have changed from the earlier papers but not sufficiently to change the essential conclusions. Additional to the conclusions of the earlier papers we now conclude that the surface freshening extends over almost the entire length of the Line-P series of oceanographic stations into the centre of the Gulf of Alaska.
It is clear from the trends computed that the changes in potential energy of the water column in the Gulf of Alaska are primarily being driven by changes in salinity rather than changes in temperature. This conclusion was also reached in F97, but that paper could only speculate rather vaguely about the possible cause. Durack and Wijffels (Citation2010) looked at a global analysis of salinity in the upper ocean and came to the same conclusion in the Gulf of Alaska. The centre panel of b in Durack and Wijffels shows a contour of −0.2 (practical salinity scale used) per 50 years passing close to Ocean Station Papa. This is not far from the value of −0.3 per century shown in of this paper. The Durack and Wijffels (Citation2010) paper shows a clear pattern of changing salinity that is close to the distribution of actual salinity. Essentially, all low-salinity areas of the oceans are getting fresher and the high-salinity areas are getting saltier, a scenario described by the authors as “the rich get richer.” In Durack et al. (Citation2012) modelling studies are used to show that this is completely consistent with an acceleration of the global water cycle between 1950 and 2000, an idea that was only vaguely speculated about in F97.
A simple box model is enlightening because it shows how little additional fresh water is needed to change the Gulf of Alaska in the way we describe. Consider a vertical column of water at Ocean Station Papa of height H = 700 db. At Station Papa we compute a trend in salinity, averaged over the top 700 db of the water column of −3.9 × 10−4 per year. If we ignore horizontal advection the rate of change of total salt in the water column is given by
Now let us keep the deep vertical velocity constant and perturb this steady state by increasing R, so
Setting H = 700 m, S 0 at Station Papa is 33.77, and the rate of change in salinity at −3.9 × 10−4 per year we find a needed value of δR = 0.81 cm y−1. This is not a very large change in total rainfall and is not measurable by any available technique except inference from salinity changes.
The results of a persistently shallowing mixed layer are potentially profound, leading to changes in the ocean environment that can have effects on organisms. Two effects immediately spring to mind. If we assume that a phytoplankter is cycled between the surface and the base of the mixed layer by turbulent motions, then as the mid-winter mixed layer shallows it will spend a larger fraction of its time in the photic zone and be able to use nutrients as we approach spring. However, as the base of the mid-winter mixed layer becomes shallower we must also expect the supply of nutrients to decrease. It seems important, therefore, that we continue to monitor the evolution of the upper ocean in response to global climate change.
Acknowledgements
The author would like to acknowledge the assistance of Peter Chandler, Patrick Cummins, and Germaine Gatien for critical readings of this manuscript and some very useful comments from two reviewers. The Argo data were collected and made freely available by the International Argo Program and the national programs that contribute to it (http://www.argo.ucsd.edu, http://argo.jcommops.org). The Argo Program is part of the Global Ocean Observing System.
References
- Bretherton , F. P. , Davis , R. E. and Fandry , C. B. 1976 . A technique for objective analysis and design of oceanic experiments . Deep-Sea Research , 23 : 559 – 582 .
- Durack , P. J. and Wijffels , S. E. 2010 . Fifty-year trends in global ocean salinities and their relationship to broad-scale warming . Journal of Climate , 23 : 4342 – 4362 . doi:10.1175/2010JCLI3377.1
- Durack , P. J. , Wijffels , S. E. and Matear , R. J. 2012 . Ocean salinities reveal strong global water cycle intensification during 1950 to 2000 . Science , 336 : 455 – 458 . doi:10.1126/science.1212222
- Freeland , H. J. 1990 . Sea surface temperatures along the coast of British Columbia: Regional evidence for a warming trend . Canadian Journal of Fisheries and Aquatic Science , 47 ( 2 ) : 346 – 350 . (doi:10.1139/f90-035)
- Freeland , H. J. , Denman , K. , Wong , C. S. , Whitney , F. and Jacques , R. 1997 . Evidence of change in the winter mixed layer in the Northeast Pacific Ocean . Deep-Sea Research , 44 ( 12 ) : 2117 – 2129 . (doi:10.1016/S0967-0637(97)00083-6)
- Kara , A. B. , Rochford , P. A. and Hurlburt , H. E. 2000 . An optimal definition for ocean mixed layer depth . Journal of Geophysical Research , 105 : 16803 – 16821 . (doi:10.1029/2000JC900072)
- Ladd , C. and Stabeno , P. J. 2012 . Stratification on the eastern Bering Sea shelf revisited . Deep-Sea Research II , 65–70 : 72 – 83 . doi:10.1016/j.dsr2.2012.02.009
- Li , M. , Myers , P. G. and Freeland , H. 2005 . An examination of historical mixed layer depths along Line-P in the Gulf of Alaska . Geophysical Research Letters , 32 ( 5 ) : L05613 doi:10.1029/2004GL021911
- Masson , D. and Cummins , P. F. 2007 . Temperature trends and interannual variability in the Strait of Georgia, British Columbia . Continental Shelf Research , 27 : 634 – 649 . (doi:10.1016/j.csr.2006.10.009)
- von Storch , H. and Zwiers , F. W. 1999 . Statistical analysis in climate research , 115 – 116 . Cambridge , , UK : Cambridge University Press .
- Thomson , R. E. and Fine , I. V. 2003 . Estimating mixed layer depth from oceanic profile data . Journal of Atmospheric and Oceanic Technology , 20 ( 2 ) : 319 – 329 . (doi:10.1175/1520-0426(2003)020<0319:EMLDFO>2.0.CO;2)