Abstract
Because of a lack of sufficient observations over the Tibetan Plateau (TP), the measurement of the strength of the thermal effects of the TP is a challenging issue, yet of crucial importance for climate research. In this study, two sets of daily reanalysis data (1979–2001) from the National Centers for Environmental Prediction reanalysis version 1 (NCEP-I) and the European Centre for Medium-Range Weather Forecasts 40-year Reanalysis data (ERA-40) are used to calculate the atmospheric diabatic heating effects of the TP and the adjacent regions with an inverse algorithm. The observational data obtained from the recent atmospheric experiments over the TP are applied to verify and evaluate the results from NCEP-I and ERA-40 reanalysis data, respectively. It is found that the ERA-40 results are closer to the observations in terms of the structure and seasonal variation of the vertical profile of the diabatic heating over the TP. The horizontal distributions of the apparent heat source are quite reasonable over Asia for both the NCEP-I and ERA-40 results.
RÉSUMÉ [Traduit par la rédaction] À cause d'un manque d'observations sur le plateau tibétain, la mesure de la force des effets thermiques du plateau tibétain est un problème difficile tout en étant d'importance cruciale pour la recherche climatique. Dans cette étude, nous utilisons deux ensembles de données quotidiennes de réanalyse (1979–2001) issues de la version 1 de la réanalyse des National Centers for Environmental Prediction (NCEP-I) ainsi que les données de réanalyse de 40 ans du Centre européen pour les prévisions météorologiques à moyen terme (ERA-40) pour calculer les effets du réchauffement atmosphérique diabatique sur le plateau tibétain et les régions adjacentes au moyen d'un algorithme inverse. Nous appliquons les données d'observation recueillies lors des récentes expériences atmosphériques sur le plateau tibétain pour vérifier et évaluer ces résultats des données de réanalyse de NCEP-I et ERA-40, respectivement. Nous trouvons que les résultats de ERA-40 sont plus proches des observations en ce qui concerne la structure et la variation saisonnière du profil vertical du réchauffement diabatique sur le plateau tibétain. Les distributions horizontales de la source de chaleur apparente sont très raisonnables au-dessus de l'Asie, tant pour les résultats de NCEP-I que de ERA-40.
1 Introduction
As the third pole of the Earth, the Tibetan Plateau (TP) thermal conditions have a profound impact on the climate of East Asia and even the entire globe (e.g., Boos & Kuang, Citation2010; Flohn, Citation1953, Citation1968; Li & Yanai, Citation1996; Lin & Wu, Citation2011, Citation2012; Wang, Bao, Hoskins, Wu, & Liu, Citation2008; Wu, Li, Jiang, & Ma, Citation2012a; Ye, Citation1981; Zhang, Li, & Wang, Citation2004). An accurate representation of the apparent heat source (Q 1) is essential to study the climate impacts of the TP. Ye, Luo, and Zhu (Citation1957) first estimated the distribution of diabatic heating over the TP and noted that the TP is a heat source in summer and a heat sink in winter except for the southwestern TP. Chen and Li (Citation1982, Citation1985) calculated the summer apparent heat source distribution in the Asian monsoon regions and proposed that the strongest diabatic heating centre is located in the northern section of the Bay of Bengal (BOB) and in the South China Sea–western Pacific Ocean instead of over the TP. Yanai and Tomita (Citation1998), Li and Yanai (Citation1996) as well as Boos and Kuang (Citation2010) further confirmed the conclusions reached by Chen and Li (Citation1982, Citation1985).
There are currently two major methods of calculating the apparent heat source. One is a direct algorithm method which calculates, on the basis of observations, the sensible heating, radiative heating and the latent heat of condensation; the sum of these three terms provides the apparent heat source. However, because of limited observations, plus the fact that it is hard to determine the turbulent exchange coefficient and sensible heat exchange coefficient needed to calculate the sensible heat flux, the calculation of the apparent heat source using the direct algorithm method is difficult. The second is an inverse algorithm method which uses standard meteorological data (winds, temperatures, geopotential heights) to determine diabatic heating from the energy balance perspective of the heat influx equation. Because it is convenient to calculate the apparent heat source with this method, many researchers prefer to use the inverse algorithm method to calculate Q 1 (Li & Yanai, Citation1996; Yanai, Li, & Song, 1992; Yanai & Tomita, Citation1998).
The reliability of the diabatic heating calculation using the inverse algorithm depends, to a great extent, on the standard meteorological data. At present, the most commonly used standard meteorological datasets are versions 1 and 2 of the reanalysis datasets from the National Centers for Environmental Prediction (NCEP) (hereafter NCEP-I and NCEP-II, respectively) and the 40-year reanalysis dataset from the European Centre for Medium-range Weather Forecasts (ECMWF; ERA-40). Before the diabatic heating effect calculated from these reanalysis datasets can have a practical application, verification of the reliability of the calculation results are particularly important. Lan, Wen, and He (Citation2005) compared the annual mean values of Q 1 based on the ERA-40 and NCEP-II reanalysis datasets and found that the result from ERA-40 is better than that from NCEP-II. However, Yanai and Tomita (Citation1998) pointed out that the continuity of the ERA-40 data could be problematic, because the ERA-40 forecast and analysis formats have changed several times between 1985 and 1992. Besides, the time series of ERA-40 data is relatively short for calculating Q 1. Yanai and Tomita (Citation1998) used NCEP-I data to calculate the apparent heat source and moisture sink from 1980 to 1994. Their results indicated that the NCEP-I dataset also has many errors, especially in the southern hemisphere (Kanamitsu et al., Citation2002). Zhang, Chen, He, Zhu, and Li (Citation2009) employed the inverse algorithm method to calculate Q 1 using NCEP-I, NCEP-II, ERA-40, and the Japanese Global Energy and Water Cycle Experiment (GEWEX) research program Asian Monsoon Experiment (GAME) reanalysis data and suggested that NCEP-I data produced good results for the Asian region; NCEP-II data did not produce good results in the tropical regions of Asia but did produce good results for the rest of Asia; ERA-40 data produced good results for the tropical regions of Asia; GAME data produced good results for tropical and subtropical oceanic regions.
Obviously, the ability of different reanalysis data to represent diabatic heating exhibit significant regional differences. It is still not clear which data can better depict the thermal effects of the TP. In addition, previous studies focused mainly on the characteristics of the horizontal distribution of Q 1, without considering the vertical distribution of diabatic heating. In fact, the vertical distribution of diabatic heating can be quite different even when the horizontal distributions of Q 1 are the same. Considering that the vertical distribution of diabatic heating has a significant effect on the development of vorticity and even on the weather system as a whole (e.g., Lin & Derome, Citation1996; Liu, Wu, & Liu, Citation1999; Wu, Li, Jiang, He, & Zhu, Citation2012b; Wu, Wang, Li, & Jin, Citation2009), it is necessary to evaluate the structure (particularly the vertical structure) of the diabatic heating over the TP and the adjacent regions derived from different reanalysis datasets.
Because both the NCEP-I and ERA-40 reanalysis data are suitable for calculating atmospheric diabatic heating over Asia (Zhang et al., Citation2009) and both are a longer time series than the NCEP-II data, they are used for calculating Q 1 over the TP and adjacent regions in this paper. Various types of observations, especially those obtained from recent atmospheric experiments conducted over the TP, are used to verify the validity of the vertical profile of diabatic heating over the TP.
In this study, we attempt to answer the following questions. How well can the reanalysis datasets quantify the thermal effects of the TP and the surrounding region? Which reanalysis dataset performs better? This paper is structured as follows. Section 2 describes the datasets and methodology. Section 3 evaluates the vertical diabatic heating profile over the TP derived from the reanalysis data and using satellite data and station data, and Section 4 evaluates the horizontal distribution of the atmospheric diabatic heating over the TP and adjacent regions. The last section summarizes the major findings and discusses some outstanding issues.
2 Data and methodology
The reanalysis datasets used in this study are the ERA-40 and NCEP-I daily data (including wind, temperature and geopotential height), at a horizontal resolution of 2.5° × 2.5°, with a vertical stratification of 17 standard isobaric levels ranging from 1000 to 10 hPa (Gibson et al., Citation1997; Kalnay et al., Citation1996; Kistler et al., Citation2001; Uppala, Citation2002).
The observational data used here include (1) the moisture profile extending from the ground to 15 km, obtained from radiosondes at Anduo Station (91°.06′E, 32°.21′N) five times daily (0600, 0900, 1200, 1500 and 1800 lst) from 4 to 14 June 1998; (2) the 3A12 product of the Tropical Rainfall Measure Mission (TRMM; Kummerow, Barnes, Kozu, Shiue, & Simpson, Citation1998; Simpson, Adler, & North, Citation1988) (including the monthly mean instantaneous rainfall rate in a 0.5° × 0.5° grid between 40°S and 40°N, the monthly mean latent heat release, and the vertical stratification containing 14 levels, extending from the ground to 18 km; the standard height of each layer is 1, 2, 3, 4, 5, 6, 7, 8, 9, 10, 12, 14, 16 and 18 km, respectively); (3) the Global Precipitation Climatology Project (GPCP) global precipitation estimation field from 1979 to 2001 (Huffman et al., Citation1997); (4) the Outgoing Longwave Radiation (OLR) daily data from 1979 to 2001 (Liebmann & Smith, Citation1996); (5) the monthly precipitation data at 71 gauge stations on the TP from 1979 to 2001; and (6) the monthly precipitation data at 160 gauge stations across China.
The inverse algorithm calculates the apparent heat source Q 1 using the following formula:
In Eq. (1), T denotes temperature, θ the potential temperature, ω the vertical velocity in the pressure coordinates, V the horizontal wind vector, k = R/p, where R and p are, respectively, the gas constant of dry air and the specific heat at constant pressure. Equation (1) was used to calculate Q 1 in each layer. Here we adopt the scheme developed by Yanai, Li, and Song (Citation1992) for calculating Q 1. Assuming ω = 0 at the top of the atmosphere, we integrate vertically through the entire atmosphere to obtain the following formula:
In Eq. (1), there are three terms on the right-hand side; the first represents the local change, the second represents the temperature advection and the third represents the vertical advection of temperature.
Most satellite remotely sensed data have only been available since 1979. In order to avoid the problem of “false” climate change which may appear in the reanalysis product because of a change in observation systems (Bengtsson, Hagemann, & Hodges, Citation2004; New, Hulme, & Jones, Citation1999; Trenberth, Citation1995), the time periods for the data used in this study all start in 1979.
3 Vertical diabatic heating profile over the TP
a Comparison of the Q1 Profile on the TP with Satellite Data
shows the seasonal variation of the vertically integrated Q 1 over the TP. From October to February, the TP is a heat sink. The magnitude of the ERA-40 result is slightly less than that of the NCEP-I data. In December, the heat sink reaches its peak, and the magnitude of the ERA-40 result is close to that produced by the NCEP-I data, which is approximately −80 W m−2. From March to September, the TP becomes a heat source. The growth stage lasts from March to June, with the growth rate of the ERA-40 result being much higher than that of NCEP-I. In June, the magnitude of the heat source reaches its peak, with the ERA-40 result being 220 W m−2, which is higher than that of the NCEP-I by 100 W m−2. From then until September, ⟨Q 1⟩, with the rate of decrease of the ERA-40 result being faster than that of NCEP-I. The difference between the highest and lowest values of ⟨Q 1⟩ for the ERA-40 result and for the NCEP-I result are 300 and 200 W m−2, respectively.
Fig. 1 Regional averaged monthly ⟨Q 1> over the Tibetan Plateau (TP) in 1979−2001 (units are W m−2). The solid line with open circles indicates the ERA-40 result and the solid line with solid circles indicates the NCEP-I result.

It is evident in that the seasonal variation of Q 1 from the ERA-40 data is more significant than from its NCEP-I counterpart. Many research results (e.g., Yang & Jiang, Citation1995) show that the thermal conditions over the TP do have significant seasonal variation.
shows the seasonal variations of the mean vertical profiles of Q 1 over the TP. The main differences between the ERA-40 and NCEP-I data follow:
• | The thermal conditions in the near-surface atmosphere are different. The ERA-40 result shows that there are heating layers of different magnitudes near the surface of the TP for the entire year (except December), but according to the NCEP-I result, such heating layers occur only from March to September. Luo and Yanai (Citation1984) calculated and analyzed the intensities of Q 1 near the surface and in the atmosphere at mid- and high levels over the TP. They indicated that there was a heat source near the surface of TP all year, thus contradicting earlier studies suggesting that there was a heat sink at most of the surface of the TP in winter. This finding is consistent with our results based on ERA-40 data (but not on the NCEP data; i.e., the atmosphere is warmed by sensible heat for almost the entire year). | ||||
• | The seasonal variation in the atmospheric heating rate is different. According to various studies (Ye & Gao, Citation1979), there are two obvious intensifications of the atmospheric heat source over the TP; the first is caused mainly by sensible heating over the TP between April and June, with a maximum heating centre at a lower level, and the second is caused by the significant enhancement of the latent heat of surface evaporation over the TP between June and August, with a maximum heating centre at a higher level. The two intensifications are clearly shown in the ERA-40 results. From April to June, the 1.0 K d−1 isoline in the upper troposphere at 300–200 hPa displays a tongue-like projection, and from June to August, above 200 hPa, the 0.5 K d−1 isoline presents another tongue-like projection. This is not evident in the NCEP-I results. Obviously, the seasonal variation of the heating rate, calculated with ERA-40 data is more reasonable. |
Fig. 2 The seasonal variation of the vertical distribution of mean Q 1 over the TP (altitude ≥3000 m), averaged over the period 1979–2001 (units are K d−1).
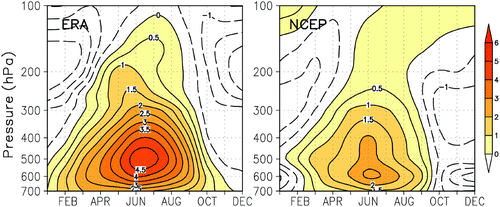
shows the monthly mean vertical profiles of the apparent heat source Q 1 over the TP (altitude ≥3000 m; i.e., approximately 700 hPa) between March and September, averaged over 1979 to 2001. In terms of the variation of the rate of heating with altitude, the two profiles seem very similar; the upper atmosphere cools while the lower atmosphere warms, showing an “S” shape, with the highest heating rates for both occurring at 500 hPa, but the intensity of the ERA-40 rate of heating is almost twice that of its NCEP-I counterpart. From March to May, the heat source intensifies, and the thickness of the warm layer increases. In June, the intensities of both the ERA-40 and NCEP-I rates of heating peak, at 4.2 and 2.1 K d−1, respectively. The height of the warm layer also peaks, at almost the 100 hPa level. Then, the thickness and the intensity of the heat source both decrease. Therefore, the seasonal variation of the ERA-40 heating profile is more pronounced than that of the NCEP-I heating profile.
Fig. 3 The monthly mean vertical profile (K d−1) of Q 1 over the TP (altitude ≥3000 m) between March and September of 1979 to 2001. Solid line: calculated value based on ERA-40 data, dashed line: calculated value based on NCEP-I data.
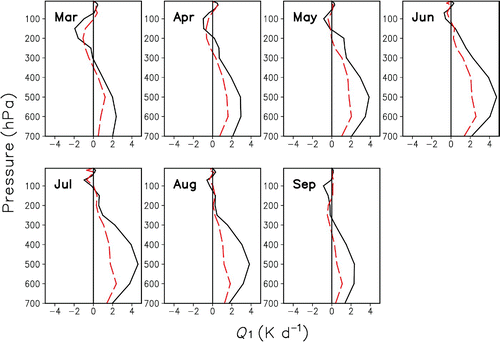
Because of the paucity of sounding data over the TP, we use the 3A12 latent heat profile () between March and September from 1998 to 2006 to perform a comparison analysis. Although these data contain some biases over the TP, they are still a valuable reference for making a qualitative judgment on the seasonal variation of the heating profile over the TP.
Comparison shows that the latent heating profile over the TP () is very similar to the Q 1 profile (), but the seasonal variation of the rate of latent heating is more clearly represented by the ERA-40 result than by the NCEP-I result. The height at which the maximum latent heating rate occurs is located around 400 hPa. It is reasonable that the maximum value of Q 1 over the TP is located at around 500 hPa, lower than the height of the maximum latent heating. The magnitude of the latent heating rate is equivalent to the value of Q 1, and it appears that the result based on the ERA-40 data is more accurate than its NCEP-I counterpart. Annamalai, Slingo, Sperber, and Hodges (Citation1999) pointed out that ERA-40 reanalysis data are superior to NCEP/NCAR reanalysis data in the seasonal mean values of certain climate features. Furthermore, the NCEP-I surface air temperature data are significantly lower on the TP, which may explain why the intensity of the NCEP-I heat source is relatively low.
Considering that the mean climate conditions may conceal differences in individual years, we use 1998 as an example for a further comparison study.
The distribution of the Q 1 vertical profile over the TP from March to September 1998 () is similar to the mean climate conditions, except the maximum heating rate occurs in July. The level of maximum heating from ERA-40 data is located near 500 hPa, while that from NCEP-I data is near 600 hPa. The monthly mean latent heating profile of 3A12 data shows that the maximum heating height remains at 550 hPa, with an apparent seasonal variation (). The most powerful heat source occurs between May and June. By comparison, the ERA-40 result is very close to the 3A12 latent heat profile.
b Evaluation of the Heating Profile using Gauge Station Data
The variable weather conditions and high-elevation environment make it difficult for humans to survive on the TP. Therefore, observations obtained from experiments on the TP are particularly valuable. We use moisture data obtained from soundings taken over Anduo station during a period of intensified observation conducted by Chinese and Japanese scientists in 1998 (for this reason, additional data in 1998 are chosen in the later sections) and combine them with the latent heating profile over the TP for further detailed examination of the heating profile.
displays the daily mean heating profiles over Anduo Station (altitude 4801 m) averaged over 4–14 June 1998, calculated from ERA-40 data and NCEP-I data and the observed relative humidity profile, as well as the latent heat profile. In terms of the maximum height of diabatic heating at Anduo station, the daily mean maximum heating rate, based on ERA-40 data, is located at 400 hPa and that based on NCEP-I data is located at 550 hPa. The maximum moisture layer occurs at 380 hPa and the maximum value of latent heating lies at about 420 hPa. Hence, the maximum heating height from the ERA-40 result is closer to the observations. The variation of the heating rate with altitude based on ERA-40 data is very similar to the observed sounding profiles of relative humidity and the latent heating, but the NCEP-I result deviates more from observations.
Fig. 7 (a) The daily mean vertical profile of Q 1 (K d−1) and (b) the relative humidity (%) from 4 to 14 June 1998; (c) the vertical profile of latent heat (K d−1) in June 1998 over Anduo station.
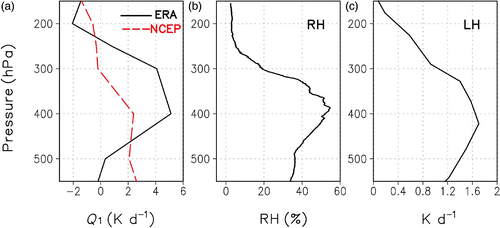
In order to verify the daily change of the TP heating rate, we select the heating profiles for the day before and after a precipitation event, the day of the precipitation event, as well as the days without precipitation and compare them with the observations. The precipitation at Anduo station for 4–14 June 1998 shows that it rained on 8 June (). We chose 7–9 June, which includes the day of precipitation event, as well as the day before and after the precipitation event to make a comparison. From 7 to 8 June, relative humidity increased and the moisture layer thickened. From 8 to 9 June, the relative humidity weakened, and the moisture layer became thinner. Correspondingly, b shows that, from 7 to 8 June, the heating rate based on ERA-40 data grew from 5 K d−1 to 8 K d−1, and the top of the warm layer increased from 300 hPa to 230 hPa (b). From 8 to 9 June, the heating rate dropped to 5 K d−1 as did the thickness of the warm layer. Therefore, the ERA-40 result shows the same trend in variation as that of relative humidity, while the NCEP-I result is not sensitive to precipitation.
Table 1. The precipitation at Anduo station from 4 to 14 June 1998 (units are millimetres).
Fig. 8 The comparison between the relative humidity and the Q 1 profile over Anduo station from 7 to 9 June 1998. (a) RH (%), (b) ERA-40 (K d−1), and (c) NCEP-I (K d−1).
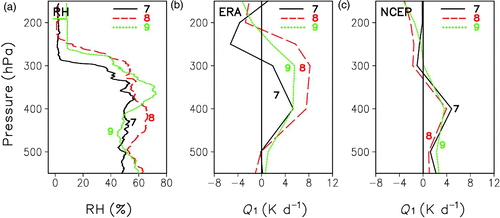
Of the days without precipitation, we selected and compared 10 June with a higher relative humidity and 12 June with a lower relative humidity (). On 10 June, the highest relative humidity was more than 70% and located at about 400 hPa. On 12 June, the highest relative humidity was only 30% and located at about 400 hPa. Comparison of the heating profiles shows that on days without precipitation the maximum heating rate appears at about 400 hPa according to the ERA-40 result. From 10 to 12 June, the atmospheric humidity decreases, as does the heating rate and the thickness of the warm layer, whereas the profile of the NCEP-I result indicates no corresponding change.
Fig. 9 The comparison between relative humidity and the Q 1 profile over Anduo station from 10 to 12 June 1998. (a) RH (%), (b) ERA-40 (K d−1), and (c) NCEP-I (K d−1).
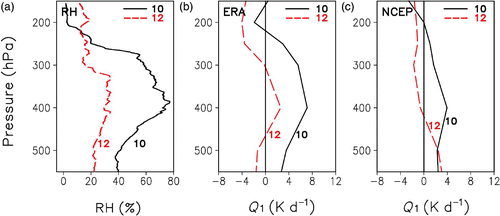
This indicates that the daily mean heating profile of the ERA-40 result and its sensitivity to daily changes are closer to the observations. In addition, according to the monthly mean vertical profile (), the height of the layer of maximum heating is located around 500 hPa, but the TP daily heating rate profile shows that there are variations in the height of the layer of maximum heating on a day-to-day basis (), and it can exceed 400 hPa in the troposphere. Thus, it can be inferred that in addition to surface heating, upper layer heating also occurs over the TP.
4 Comparison and evaluation of atmospheric diabatic heating in Asia
a Horizontal Distribution of Q1 in Summer
shows the distribution of the vertically averaged Q 1 in summer (from June to August) for 1979 to 2001. The ERA-40 dataset indicates that in summer there are two heat sources of relatively high intensity and wide range in Asia (a). One lies close to the ring of the BOB, which stretches from the northwest coast of the Indochinese Peninsula, across the southern TP, to the area between the east coast of the Arabian Sea and the west coast of the Indian Peninsula, forming a strong heat source in the shape of “∩”. The strongest centre in Asia, is located in the northeastern BOB and northwestern Indochinese Peninsula, with a maximum of about 500 W m−2.
Fig. 10 The distribution of average ⟨Q 1⟩ in summer (June to August) from 1979 to 2001 (units are W m−2). The orange dashed curve indicates the Tibetan Plateau area: (a) ERA-40 data calculation result, (b) NCEP-I data calculation result, and (c) the correlation of the ERA-40 data calculation result and the NCEP-I data calculation result. (Those correlation coefficients beyond the 95% confidence level, based on the Student's t-test, are shaded; the contour interval is 0.1.)
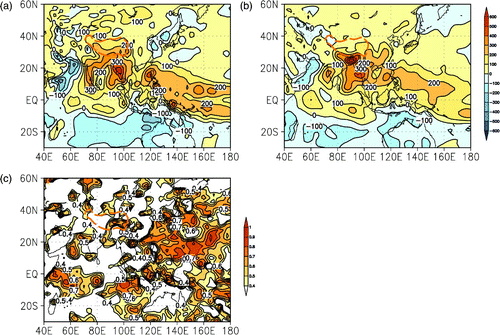
The centre of the other maximum lies in the South China Sea and the tropical western Pacific Ocean, with a maximum of 400 W m−2 (a). It is found that the area of strong heat source splits into two branches in the tropical western Pacific Ocean, forming a southern and a northern strong heat source belt along 5°S and 5°N, respectively. There are also two strong atmospheric heat sinks covering a wide range: one is in the western Arabian Sea and the western Indian Ocean, with two centres of similar intensity (about 300 W m−2) in the northwestern Arabian Sea and off the northeast coast of Somalia; the other is in the central Indian Ocean and the northwest of Australia, with an intensity centre (−200 W m−2) along the northwest coast of Australia.
The NCEP-I dataset shows strong atmospheric heat sources in the same two areas of Asia (b), but their ranges, the location of their centres and their intensity are different from those produced with the ERA-40 dataset. Instead of a ∩-shaped strong heat source belt, there are three centres in the vicinity of the BOB. The strongest centre is over Bangladesh, located between the south of the TP and the north of the BOB, with a central maximum of 600 W m−2. The second is over the west coast of the Indochinese Peninsula, with a central maximum of 400 W m−2. The weakest intensity centre occurs over the west coast of the Indian Peninsula, with a maximum value of 300 W m−2. Although the strong heat source belt in the South China Sea and the tropical western Pacific Ocean is weaker than that produced by the ERA-40 data, the highest intensity centre (300 W m−2) is in the eastern South China Sea, with no obvious south or north branches. The NCEP-I strong heat sink belt is similar in range to that of ERA-40, but its intensity is obviously weaker. The heat sink over the western Arabian Sea is in the shape of a north–south ribbon, similar in intensity to the heat sink over the western Indian Ocean. It has an even distribution with an intensity minimum of −100 W m−2. The heat sink area in the middle Indian Ocean and northwest Australia covers a smaller area than that of ERA-40, with a central minimum of about −100 W m−2.
In addition, by comparing a with b, it can be seen that the most pronounced differences between the two occur in the Sea of Japan, Korea, and the Yellow Sea, where the result based on ERA-40 data is negative. This means that in summer this area is dominated by a heat sink, while the result based on NCEP-I data is a heat source. In addition, the ERA-40 result shows that the TP heat source is obviously higher than the one located in China east of 105°E. The NCEP-I result shows that at the same latitude the TP heat source was weaker than that in the eastern part of China. The correlation coefficient between the two datasets is further confirmation that there are clear differences in the calculation of heat sources in these areas (c).
In summer, in tropical areas, latent heating is the dominant atmospheric heat source. The release of latent heat depends on the occurrence of precipitation. For subtropical areas, because of the relatively low water vapour content, latent heating is only a small part of the diabatic heating. The OLR reflects the intensity of convection, the condition of vertical movement and so forth. As well as applying it to the study of tropical seas, it is also used in research into the TP thermal conditions (Li, Zhang, & Wu, Citation1996; Yang & Jiang, Citation1995) to compensate for the lack of data over the TP. Therefore, we chose the GPCP precipitation and the 3A12 monthly mean TRMM instantaneous rate of precipitation with a finer grid and the observed precipitation at 160 gauge stations across China together with OLR data, to make a comprehensive judgement about the validity of the heat source distribution.
For GPCP and 3A12 precipitation distributions (a and 11b), the strongest precipitation centre in summer in Asia is in the northeastern part of the BOB and the northwestern Indochinese Peninsula. The OLR distribution also shows that the strongest area of cumulus convection is located over this region but slightly to the south (c). South of the TP there is no strong precipitation or convection centre. The NCEP-I dataset overestimates the heat source in Bangladesh and the estimate based on ERA-40 data is closer to observations. The latter shows a more reasonable Q 1 distribution in the ring of the BOB. Precipitation over the South China Sea and the tropical western Pacific Ocean nearly coincides with the OLR intensity centre. It is located in the northwest Philippines, which agrees well with the result based on ERA-40 data, while the NCEP-I result is relatively eastward. The ERA-40 result also more clearly represents the precipitation in the Pacific Ocean and the south and north branches of the OLR, which is consistent with the results of Newman, Sardeshmukh, and Bergman (Citation2000). From the above analysis, we suggest that the ERA-40 result gives a more reasonable estimate of the two major heat source areas.
Fig. 11 (a) the GPCP precipitation distribution in summer from 1979 to 2001, (b) the 3A12 precipitation distribution in summer from 1998 to 2006, (c) the OLR distribution in summer from 1979 to 2001, and (d) the summer precipitation in China from 1979 to 2001.
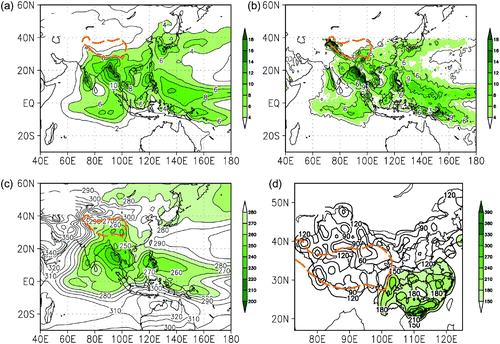
At the same latitude, the relative strength of the heat source over the TP and that over eastern China is different in the two datasets. The ERA-40 dataset shows that the strength of the heat source over the TP is stronger, while the NCEP-I dataset shows the opposite. In , the GPCP precipitation, the 3A12 precipitation and the gauge station precipitation distribution in China (d) show that the distribution of precipitation from the southeastern part of the TP to the eastern part of China is ∪-shaped. The strongest precipitation centre is in the southern part of China; the precipitation in the southeastern part of the TP is equivalent to that in the eastern part of China. The distribution of the OLR shows that at the same latitude, the OLR over the plateau was stronger than over eastern China, but equivalent to that over the western Indian Peninsula. So, in comparison with the heat source in the Indian Peninsula, the TP heat source produced with ERA-40 data is reasonable but the heat source in eastern China produced with ERA-40 data is too small. The NCEP-I estimate of the magnitude of the atmospheric heat source in eastern China is quite sound, but the estimate of the magnitude of the heat source over the southeastern TP is relatively weak. Xu, Ding, and Zhao (Citation2001) suggested that the NCEP/NCAR dataset is more credibile in eastern China and the low-altitude areas than in the western part of China and at high altitudes. Li, Zhang, and Chen (Citation2004) noted that the reanalysis temperature data, especially NCEP-I surface temperature data were obviously low for the TP. Therefore, for the calculation of the atmospheric heat source over the TP, the ERA-40 dataset is better than the NCEP-I dataset.
In addition, strong precipitation occurs over the ocean centred over southern coastal Japan, and the OLR data indicates that there is relatively intense convection near Japan. According to the ERA-40 result, southern Japan is dominated by a heat source of relatively weak magnitude. In the Pacific Ocean east of Japan, there even appears to be a heat sink. Therefore, for the estimate of diabatic heating in Japan and adjacent seas, the ERA-40 result is poor, but the NCEP-I result is better.
b Horizontal Distributions of Q1 in the Summer of 1998
As in the evaluation of the heating profile in the previous section, we now carry out a comparitive analysis on the vertically averaged apparent heat source Q 1 in June 1998. The ERA-40 and NCEP-I results are shown in . The ERA-40 result shows that around the ring of the BOB, there are three heat source centres (a). The strongest occurs over the coastal areas of the western Indian Peninsula extending to the central Arabian Sea and reaching a maximum of 700 W m−2. The second occurs over the coastal Indochinese Peninsula extending to the central BOB and reaching a maximum intensity around 500 W m−2, forming a weaker centre. A separate heat source centre of 400 W m−2 also formed south of the TP. The positions of these three centres coincide well with those based on the NCEP-I data (b). But the strongest heat source of 500 W m−2 is located south of the TP. The second strongest heat source occurs in the western part of the Indochinese Peninsula and along the western coast of the Indian Peninsula reaching a maximum of 400 W m−2. Another heat source centre reaches a maximum of 300 W m−2, which shows a reverse sequence of the three central intensities. Comparing the GPCP precipitation and the 3A12 precipitation (a and 13b), along the ring of the BOB, the position and the intensity of the three heat source centres calculated with the ERA-40 data match well with the precipitation in this region. At low latitudes, the observed precipitation corresponds well with the distribution of the OLR. This conclusion is also consistent with its distribution (c).
Fig. 12 The horizontal distribution of average ⟨Q 1⟩ in June 1998 (units are W m−2): (a) calculation result based on ERA-40 data and (b) calculation result based on NCEP-I data.

Fig. 13 (a) The distribution of the GPCP precipitation, (b) the 3A12 precipitation, (c) the OLR precipitation, and (d) precipitation in China in June 1998.
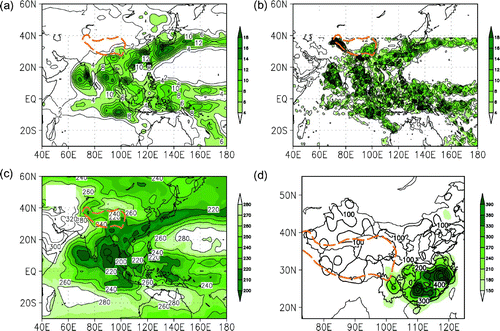
Both the ERA-40 and NCEP-I results show obvious heat source centres in the middle and lower reaches of the Yangtze River. The former is weaker (approximately 300 W m−2) than the latter (approximately 400 W m−2). The maximum of the centre of the ERA-40 result lies in the eastern area of the Sea of Japan, about 300 W m−2, and that of NCEP-I is in the southern part of Japan, about 300 W m−2. According to the precipitation data (), the strongest precipitation belt in east Asia covers southern China, the middle and lower reaches of the Yangtze River, southern Japan and the Kuril Islands, which is also clearly represented in the OLR distribution. But over the subtropical areas, the latent heat of condensation released by precipitation makes less contribution to diabatic heating than over the tropical areas. Heavy precipitation does not mean high heat source values. From the intensity distribution of the OLR in this region, it can be seen that the value of the OLR in the precipitation region is equivalent to that of the southern and southeastern parts of the TP. Therefore, in terms of the position of the heat source centre in this region, the NCEP-I dataset is better than ERA-40 data.
5 Conclusions and discussion
The thermal effects of the TP can have profound impacts on the global climate, serving as “an amplifier” of the central Asian drought, a modulator of the east Asian summer monsoon (e.g., Wu et al., Citation2012a), and even a predictor for the North American climate (Lin & Wu, Citation2012). Based on ERA-40 and NCEP-I reanalysis data, apparent heat sources over the TP and the surrounding areas have been calculated using different ground-based observations together with satellite remotely sensed data to evaluate ERA-40 and NCEP-I results. In particular, the observations from soundings during atmospheric science experiments over the TP are used to assess the vertical structure of the diabatic heating over the TP calculated with the ERA-40 and NCEP-I data.
For the vertical distribution of the apparent heat source over the TP, the heating profiles calculated with ERA-40 and NCEP-I data are reasonably similar to observations. The results using the ERA-40 data are closer to the observations and larger than the results using the NCEP-I data. The seasonal variation of the TP diabatic heating profiles calculated with ERA-40 data is more evident than when NCEP-I data are used. The sensitivity of the ERA-40 result to precipitation is also stronger than the NCEP-I result and closer to the observations.
In terms of the estimation of the horizontal distribution of the vertical mean apparent heat source in Asia, the ERA-40 and NCEP-I results are basically the same with respect to the distribution of strong heating centres, but the positions and the intensities of the centres are different. In general, the ERA-40 result provides a better estimation of the position and intensity of two heat source regions: the ring of the BOB between the eastern Arabian Sea to the Indochinese Peninsula and the region from the South China Sea to the tropical western Pacific Ocean. For eastern China and the southern part of Japan, the NCEP-I data works better.
Which reanalysis data are better suited for the estimation of the thermal effects of the TP depends on the quality of the data. Many factors influence the quality of the reanalysis data, such as changes in observation systems and the variety, quantity and quality of the data used in assimilation systems (Bengtsson et al., Citation2004, Citation2007; Wu, Kinter, & Kirtman, Citation2005). In addition to the error caused by changes in observation systems, the quality of the reanalysis data is also affected by systemic errors such as those in numerical prediction models and assimilation patterns (Kalnay et al., Citation1996; Kistler et al., Citation2001). There have been many related evaluations or verifications on the quality of the reanalysis data over East Asia. All these studies show that the reanalysis data mentioned in this study could reasonably reflect the space-time distribution characteristics of regional climate change in East Asia in general, but they still have biases. Besides, different reanalysis data in different regions and different time periods have different reliabilities (Annamalai et al., Citation1999; Frauenfeld, Zhang, & Serreze, Citation2005; Zhao & Fu, Citation2008). With the regular updating of observation systems and the development of numerical assimilation technology, the quality of the reanalysis data has been steadily improving. The reliability of reanalysis data after 1979 is much better than before 1979. The quality of ERA-40 and Japanese 25-year Reanalysis Project JRA-25 data has also improved in many aspects compared with that of NCEP-I and NCEP-II data.
Acknowledgements
The radiosonde data at Anduo were obtained from the GAME/Tibet project, supported by the Ministry of Education, Culture, Sports, Science and Technology (MEXT), the Frontier Research System for Global Change (FRSGC), the National Space Development Agency (NASDA) of Japan, the Chinese Academy of Science and the Asian Pacific Network. We thank ECMWF and NCEP for providing the related data. This study is jointly supported by the National Natural Science Foundation of China (Grant Nos. 41205066 and 41230528), the National Basic Research Program of China “973” (Grant No. 2013CB430202) and a project funded by the Priority Academic Program Development of Jiangsu Higher Education Institutions (PAPD).
References
- Annamalai , H. , Slingo , J. M. , Sperber , K. R. and Hodges , K. 1999 . The mean evolution and variability of the Asian summer monsoon: Comparison of ECMWF and NCEP-NCAR reanalysis . Monthly Weather Review , 27 : 1157 – 1186 . (doi:10.1175/1520-0493(1999)127<1157:TMEAVO>2.0.CO;2)
- Bengtsson , L. , Arkin , P. , Berrisford , P. , Bougeault , P. , Kallberg , P. , Simmons , A. J. , Uppala , S. , Folland , C. K. , Gordon , C. , Haines , K. , Hodges , K. I. , Rayner , N. , Thorne , P. W. , Jones , P. , Stammer , D. and Vose , R. S. 2007 . The need for a dynamical climate reanalysis . Bulletin of the American Meteorological Society , 88 : 495 – 501 . (doi:10.1175/BAMS-88-4-495)
- Bengtsson , L. , Hagemann , S. and Hodges , K. 2004 . Can climate trends be calculated from reanalysis data? . Journal of Geophysical Research , 109 : D11111 doi:10.1029/2004 JD004536
- Boos , W. and Kuang , Z. 2010 . Dominant control of the South Asian monsoon by orogrophic insulation versus plateau heating . Nature , 463 : 218 – 222 . (doi:10.1038/nature08707)
- Chen, L. X., & Li, W. L. (1982). The structure of monthly atmosphere heat sources over the Asian monsoon region. Proceedings of National Seminar on Tropical Summer Monsoon (II). pp. 246–255. Yunnan People's Publishing House, Kunming (in Chinese).
- Chen , L. X. and Li , W. L. 1985 . The atmospheric heat budget in summer over Asia monsoon area . Advances in Atmospheric Sciences , 2 : 487 – 497 . (doi:10.1007/BF02678747)
- Flohn , H. 1953 . Hochgebirge und allgemeine Zirkulation, II: Die Gebirge als Wärmequellen . Meteorology and Atmospheric Physics , 5 : 265 – 279 .
- Flohn, H. (1968). Contributions to a meteorology of the Tibetan highlands. Atmospheric Science Paper 130, pp. 120. Colorado State University, Fort Collins, Colorado.
- Frauenfeld , O. W. , Zhang , T. and Serreze , M. C. 2005 . Climate change and variability using European Centre for Medium-Range Weather Forecasts reanalysis (ERA-40) temperatures on the Tibetan Plateau . Journal of Geophysical Research , 110 : D2101 doi:10.1029/2004JD005230
- Gibson, J. K., Kallberg, P., Uppala, S., Hernandez, A., Nomura, A., & Serrano, E. (1997). ERA description, ECMWF reanalysis project report series 1, ECMWF. Reading.
- Huffman , G. J. , Adler , R. F. , Arkin , P. , Chang , A. , Ferraro , R. , Gruber , A. , Janowiak , J. , McNab , A. , Rudolf , B. and Schneider , U. 1997 . The Global Precipitation Climatology Project (GPCP) combined precipitation datasets . Bulletin of the American Meteorological Society , 78 : 5 – 20 . (doi:10.1175/1520-0477(1997)078<0005:TGPCPG>2.0.CO;2)
- Kalnay , E. , Kanamitsu , M. , Kistler , R. , Collins , W. , Deaven , D. , Gandin , L. , Iredell , M. , Saha , S. , White , G. , Woollen , J. , Zhu , Y. , Leetmaa , A. , Reynolds , R. , Chelliah , M. , Ebisuzaki , W. , Higgins , W. , Janowiak , J. , Mo , K. C. , Ropelewski , C. , Wang , J. , Jenne , R. and Joseph , D. 1996 . The NCEP/NCAR 40-year reanalysis project . Bulletin of the American Meteorological Society , 77 ( 3 ) : 437 – 471 . (doi:10.1175/1520-0477(1996)077<0437:TNYRP>2.0.CO;2)
- Kanamitsu , M. , Ebisuzaki , W. , Woollen , J. , Yang , S.-K. , Hnilo , J. J. , Fiorino , M. and Potter , G. L. 2002 . NCEP-DOE AMIP-II reanalysis (R-2) . Bulletin of the American Meteorological Society , 83 : 1631 – 1645 . (doi:10.1175/BAMS-83-11-1631)
- Kistler , R. , Kalnay , E. , Collins , W. , Saha , S. , White , G. , Woollen , J. , Chelliah , M. , Ebisuzaki , W. , Kanamitsu , M. , Kousky , V. , van den Dool , H. , Jenne , R. and Fiorino , M. 2001 . The NCEP-NCAR 50-year reanalysis: Monthly means CD-ROM and documentation . Bulletin of the American Meteorological Society , 82 ( 2 ) : 247 – 267 . (doi:10.1175/1520-0477(2001)082<0247:TNNYRM>2.3.CO;2)
- Kummerow , C. , Barnes , W. , Kozu , T. , Shiue , J. and Simpson , J. 1998 . The Tropical Rainfall Measuring Mission (TRMM) sensor package . Journal of Atmospheric and Oceanic Technology , 15 : 808 – 816 . (doi:10.1175/1520-0426(1998)015<0809:TTRMMT>2.0.CO;2)
- Lan , G. D. , Wen , Z. P. and He , H. Y. 2005 . Comparison of the atmospheric heat sources obtained from ERA and those from NCEP-II and study of the variations in the nature of heating over the global atmosphere . Chinese Journal of Atmospheric Sciences , 29 ( 1 ) : 154 – 163 .
- Li , C. F. and Yanai , M. 1996 . The onset and interannual variability of the Asian summer monsoon in relation to land-sea thermal contrast . Journal of Climate , 9 : 358 – 375 . (doi:10.1175/1520-0442(1996)009<0358:TOAIVO>2.0.CO;2)
- Li , C. , Zhang , T. J. and Chen , J. 2004 . Climatic change of Qinghai-Xizang Plateau region in recent 40-year reanalysis and surface observation data-contrast of observational data and NCEP, ECMWF surface air temperature and precipitation . Plateau Meteorology , 23 ( suppl ) : 97 – 103 . (in Chinese)
- Li , D. L. , Zhang , J. J. and Wu , H. B. 1996 . A study on the effects of climatic feature of OLR over the Qinghai-Xizang Plateau on general circulation in northern hemisphere . Plateau Meteorology , 15 ( 3 ) : 259 – 268 . (in Chinese)
- Liebmann , B. and Smith , C. A. 1996 . Description of a complete (interpolated) outgoing longwave radiation dataset . Bulletin of the American Meteorological Society , 77 : 1275 – 1277 .
- Lin , H. and Derome , J. 1996 . On the modification of the high and low-frequency eddies associated with the PNA anomaly: An observational study . Tellus , 49A : 87 – 99 .
- Lin , H. and Wu , Z. 2011 . Contribution of the autumn Tibetan Plateau snow cover to seasonal prediction of North American winter temperature . Journal of Climate , 24 : 2801 – 2813 . (doi:10.1175/2010JCLI3889.1)
- Lin , H. and Wu , Z. 2012 . Contribution of Tibetan Plateau snow cover to the extreme winter condition of 2009–2010 . Atmosphere-Ocean , 50 ( 1 ) : 86 – 94 . (doi:10.1080/07055900.2011.649036)
- Liu , Y. M. , Wu , G. X. and Liu , H. 1999 . The effect of spatially nonuniform heating on the formation and variation of subtropical high part III: Condensation heating and South Asia High and Western Pacific Subtropical High . Acta Meteorologica Sinica , 57 ( 5 ) : 525 – 538 . (in Chinese)
- Luo , H. and Yanai , M. 1984 . The large-scale circulation and heat sources over the Tibetan Plateau and surrounding areas during the early summer of 1979, Part II: Heat and moisture budgets . Monthly Weather Review , 112 : 966 – 989 . (doi:10.1175/1520-0493(1984)112<0966:TLSCAH>2.0.CO;2)
- New , M. , Hulme , M. and Jones , P. 1999 . Representing twentieth-century spacetime climate variability, Part II: Development of 1901–96 monthly grids of terrestrial surface climate . Journal of Climate , 13 : 2217 – 2238 . (doi:10.1175/1520-0442(2000)013<2217:RTCSTC>2.0.CO;2)
- Newman , M. , Sardeshmukh , P. D. and Bergman , J. W. 2000 . An assessment of the NCEP, NASA, and ECMWF reanalysis over the tropical west Pacific warm pool . Bulletin of the American Meteorological Society , 81 : 41 – 48 . (doi:10.1175/1520-0477(2000)081<0041:AAOTNN>2.3.CO;2)
- Simpson , J. , Adler , R. F. and North , G. R. 1988 . A proposed Tropical Rainfall Measuring Mission (TRMM) satellite . Bulletin of the American Meteorological Society , 69 : 278 – 295 . (doi:10.1175/1520-0477(1988)069<0278:APTRMM>2.0.CO;2)
- Trenberth , K. E. 1995 . Atmospheric circulation climate changes . Climatic Change , 31 : 427 – 453 . (doi:10.1007/BF01095156)
- Uppala, S. (2002). ECMWF reanalysis, 1957–2001, ERA-40. ERA-40 Project Report Series, 3, pp. 1–10.
- Wang , B. , Bao , Q. , Hoskins , B. , Wu , G. and Liu , Y. 2008 . Tibetan Plateau warming and precipitation change in East Asia . Geophysical Research Letters , 35 : L14702 doi:10.1029/2008GL034330
- Wu , R. , Kinter , J. L. and Kirtman , B. P. 2005 . Discrepancy of interdecadal changes in the Asian region among the NCEP-NCAR reanalysis, objective analyses, and observations . Journal of Climate , 15 : 3048 – 3067 . (doi:10.1175/JCLI3465.1)
- Wu , Z. , Li , J. , Jiang , Z. and Ma , T. 2012a . Modulation of the Tibetan Plateau snow cover on the ENSO teleconnections: From the East Asian summer monsoon perspective . Journal of Climate , 25 : 2481 – 2489 . (doi:10.1175/JCLI-D-11-00135.1)
- Wu , Z. , Li , J. , Jiang , Z. , He , J. and Zhu , X. 2012b . Possible effects of the North Atlantic Oscillation on the strengthening relationship between the East Asian summer monsoon and ENSO . International Journal of Climatology , 32 : 794 – 800 . (doi:10.1002/joc.2309)
- Wu , Z. , Wang , B. , Li , J. and Jin , F. F. 2009 . An empirical seasonal prediction model of the East Asian summer monsoon using ENSO and NAO . Journal of Geophysical Research , 114 : D18120 doi:10.1029/2009JD011733
- Xu , Y. , Ding , Y. H. and Zhao , Z. C. 2001 . Confidence analysis of NCEP/NCAR 50-year global reanalyzed data in climate change research in China . Quarterly Journal of Applied Meteorology , 12 ( 3 ) : 337 – 347 . (in Chinese)
- Yanai , M. , Li , C. F. and Song , Z. S. 1992 . Seasonal heating of the Tibetan Plateau and its effects on the evolution of the Asian summer monsoon . Journal of the Meteorological Society of Japan , 70 : 319 – 351 .
- Yanai , M. and Tomita , T. 1998 . Seasonal and interannual variability of atmospheric heat sources and moisture sinks as determined from NCEP–NCAR reanalysis . Journal of Climate , 11 : 463 – 482 . (doi:10.1175/1520-0442(1998)011<0463:SAIVOA>2.0.CO;2)
- Yang , X. F. and Jiang , S. C. 1995 . Characteristics of the seasonal variation in OLR field over the Tibetan Plateau . Quarterly Journal of Applied Meteorology , 6 ( 4 ) : 414 – 421 . (in Chinese)
- Ye , D. 1981 . Some characteristics of the summer circulation over the Qinghai-Xizang (Tibet) Plateau and its neighborhood . Bulletin of the American Meteorological Society , 62 : 14 – 19 . (doi:10.1175/1520-0477(1981)062<0014:SCOTSC>2.0.CO;2)
- Ye, D. Z., & Gao, Y. X. (1979). The meteorology of the Qinghai-Xizang (Tibet) Plateau (pp. 278). Beijing: Science Press (in Chinese).
- Ye , D. Z. , Luo , S. W. and Zhu , B. Z. 1957 . Structure of the circulation fields over Tibetan Plateau and surrounding areas and thermal balance of the tropospheric atmosphere . Acta Meteorologica Sinica , 28 ( 2 ) : 108 – 121 . (in Chinese)
- Zhang , B. , Chen , L. X. , He , J. H. , Zhu , C. W. and Li , W. 2009 . Characteristics of atmospheric heat sources over Asia in summer: Comparison of results calculated using multiple reanalysis datasets . Acta Meteorologica Sinica , 23 ( 5 ) : 585 – 597 .
- Zhang , Y. , Li , T. and Wang , B. 2004 . Decadal change of the spring snow depth over the Tibetan Plateau: The associated circulation and influence on the East Asian summer monsoon . Journal of Climate , 17 : 2780 – 2793 . (doi:10.1175/1520-0442(2004)017<2780:DCOTSS>2.0.CO;2)
- Zhao , T. B. and Fu , C. B. 2008 . Intercomparison of the summertime subtropical high from the ERA-40 and NCEP/NCAR over east Eurasia and the western north Pacific . Advances in Atmospheric Sciences , 26 ( 1 ) : 119 – 131 . (doi:10.1007/s00376-009-0119-8)