Abstract
Hourly temperature profile sequences from a time series of radiometric soundings over Amundsen Gulf in Canada's western maritime Arctic were used to establish the general shape of heat flux convergence (heat accumulation) profiles attributable to mesoscale sensible heat flux from the unconsolidated sea-ice surface. This sensible heat flux signature profile was then used to identify 520 similar heat flux convergence profiles in the January–April 2008 period. Only hours with clear skies were included to eliminate heat flux convergence resulting from enhanced downwelling longwave radiation, which often accompanies cloud cover, and heating caused by the phase change of water. The median heat flux convergence profile attributed to sensible heat flux decreased logarithmically with height. The sensible heat flux ranged from near 0 to 183 W m−2; the median was 11.4 W m−2; the average was 21.1 W m−2; the mode was 0.1 W m−2. These sensible heat flux estimates are reliable because they are based on the fundamental principle of heat conservation and measured temperature profiles. A relationship best described by a fourth-order polynomial was found between the magnitude of the sensible heat flux and the vertical extent of the heat flux convergence (h) which indicated a nearly linear increase in h from 50 to 350 m as the sensible heat flux increased from near 0 to 28 W m−2 and a plateau in h at about 500 m for sensible heat flux above 80 W m−2.
RÉSUMÉ [Traduit par la rédaction] Nous avons utilisé des séquences de profils de température horaire d'une série chronologique de sondages radiométriques au-dessus du golfe d'Amundsen dans l'Arctique maritime de l'ouest du Canada pour établir la forme générale des profils de convergence de flux de chaleur (accumulation de chaleur) attribuable au flux de chaleur sensible à mésoéchelle provenant de la surface non consolidée de la glace de mer. Nous avons ensuite employé ce profil signature de flux de chaleur sensible pour identifier 520 profils de convergence de flux de chaleur semblables au cours de la période de janvier à avril 2008. Nous n'avons pris en compte que les heures de ciel clair pour éliminer la convergence de flux de chaleur résultant du rayonnement accru de grandes longueurs d'onde qui accompagne souvent les ciels couverts de même que le réchauffement dû au changement de phase de l'eau. Le profil médian de convergence de flux de chaleur attribué au flux de chaleur sensible diminuait de façon logarithmique avec la hauteur. Le flux de chaleur sensible variait de près de 0 jusqu’à 183 W m−2; la médiane était de 11.4 W m−2; la moyenne était de 21.1 W m−2; le mode était 0.1 W m−2. Ces estimations de flux de chaleur sensible sont fiables parce qu'elles sont basées sur le principe fondamental de la conservation de la chaleur et sur des profils de température mesurés. Nous avons trouvé une relation, bien décrite par un polynome du quatrième ordre, entre la grandeur du flux de chaleur sensible et l'extension verticale de la convergence de flux de chaleur (h), qui indique un accroissement quasi linéaire de h de 50 à 350 m lorsque le flux de chaleur sensible passe de près de 0 jusqu’à 28 W m−2, et un plateau de h à environ 500 m pour un flux de chaleur sensible supérieur à 80 W m−2.
1 Introduction
During winter in the Arctic, a surface-based inversion is the prevalent temperature profile of an atmospheric boundary layer (ABL) over consolidated sea ice (Overland & Guest, Citation1991). The complex emission, absorption, and re-emission of longwave radiation between neighbouring altitudes results in relatively small heat flux divergence values which generally decrease with height (Drue & Heinemann, Citation2007; Hoch, Calanca, Philipona, & Ohmura, Citation2007; Stull, Citation2000). Though small, persistent radiative heat flux divergence can dramatically cool the ABL. The strength of the radiation inversion is often modulated by advective heat flux divergence (heat depletion) or convergence (heat accumulation). Overland and Guest (Citation1991) termed the depth to which these two heat divergence mechanisms drive the thermal structure of the Arctic ABL the radiative boundary layer (RBL).
Over unconsolidated sea ice (pack ice with an inter-ice-floe lead network and/or a polynya, a non-linearly shaped area of open water or new and young sea ice ≤0.3 m thick), the RBL may be modified by the convergence of sensible heat flux from the surface. On the synoptic scale, the area-average sensible heat flux over unconsolidated sea ice is usually a relatively small heat source because the local upward flux from the open water and/or through thin ice tends to be balanced by the downward flux to the more expansive surface of the thicker pack ice (Lüpkes, Vihma, Birnbaum, & Wacker, Citation2008; Overland et al., Citation2000). However, at the micro- and the mesoscales, the temperature profile of the RBL can be altered near the surface by sensible heat flux even when the leads and/or polynyas constitute only 1–5% of the total surface area (Andreas & Cash, Citation1999; Andreas et al., Citation2002; Khvorostyanov, Curry, Gultepe, & Strawbridge, Citation2003; Maykut, Citation1978; Raddatz, Galley, & Barber, Citation2012). The convergence of sensible heat near the surface typically generates a thermal internal boundary layer (TIBL) (Arya, Citation2001; Garratt, Citation1990; Overland & Guest, Citation1991). For a tabulation of field observations of sensible heat flux over leads and polynyas, see Gultepe, Isaac, Williams, Marcotte, and Strawbridge (Citation2003). Observations often vary because of differences in surface and/or atmospheric conditions and also because of differing measurement techniques (for example, tower versus aircraft platforms).
In 2008 over Amundsen Gulf in Canada's western maritime Arctic, the median winter ABL had a 0–350 m TIBL that was attributed solely to sensible heat flux from the unconsolidated sea-ice surface, below an elevated inversion or residual RBL to 1000 m (Raddatz, Asplin, Candlish, & Barber, Citation2011). A TIBL, linked to the sea-ice cover of the Gulf, was found on 10 of the 12 days examined from January to March 2008 (Raddatz et al., Citation2012). Fetches ranging from 25 to 82 km (mean of 41.6 km), corresponding to adjustment times of 2.4 to 5.4 h (mean = 3.7 h), indicated that the temperature profile of the lower portion of the ABL was responding to the mesoscale rather than the microscale sensible heat flux.
The temperature profile of the Arctic ABL may also be modified by the release of latent heat associated with condensation and/or deposition of water vapour or its consumption by evaporation and/or sublimation (Clapp, Citation1961; Serreze et al., Citation2007). Cloud cover may also warm the ABL by increasing downwelling longwave radiation. This latter effect may be as large as that resulting from sensible heat flux from the surface (Curry, Rossow, Randall, & Schramm, Citation1996; Persson, Fairall, Andreas, Guest, & Perovich, Citation2002; Stramler, Del Genio, & Rossow, Citation2011).
Microwave radiometric observations of temperature and vapour density profiles during the January through April 2008 portion of the International Polar Year, Circumpolar Flaw Lead (IPY-CFL) system study (Barber et al., Citation2010) provided a unique opportunity to apply the integral profile method to estimate the mesoscale sensible heat flux from the unconsolidated sea-ice surface of Amundsen Gulf. During this period, pack ice with a vast network of inter-ice-floe leads covered most of the Gulf, and a polynya was a persistent feature of the icescape (Raddatz et al., Citation2012). The methodology, although rarely employed because it requires high temporal resolution soundings, is accurate because it is derived from a basic principle—conservation of heat. Hourly average ABL temperature profile sequences were examined to establish the general shape of heat flux convergence (heat accumulation) profiles attributable to mesoscale sensible heat flux from the surface, rather than to warm air advection. The sensible heat flux signature profile was then used to identify all similar heat flux convergence profiles from the dataset of over 2800 usable hourly profiles. For the selected hours (n = 520), vertical integration of the heat flux convergence gave estimates of the sensible heat flux under clear skies.
This analysis of hourly heat flux convergence profiles over Amundsen Gulf will contribute to our understanding of the influence of sensible heat flux on the thermal structure of the Arctic ABL over unconsolidated sea ice, which is a prevalent surface type in much of the Arctic during the annual cycle (Galley, Key, Barber, Hwang, & Ehn, Citation2008). This understanding will improve our characterization and modelling of the Arctic air mass which develops over the western maritime Arctic (Anderson & Neff, Citation2008; Bradley, Keimig, & Diaz, Citation1992; Brummer, Citation1997; Curry, Citation1983; Gultepe et al., Citation2003; Penner, Citation1955; Randall et al., Citation1998). In addition, because the southward movement of the Arctic air mass is a significant feature of the North American climate in winter, more realistic modelling of the thermal character of the Arctic ABL will help to improve weather simulations below the Arctic Circle.
2 Data
The IPY-CFL data-collection campaign saw the CCGS Amundsen overwinter in unconsolidated high concentration sea ice in Amundsen Gulf in Canada's western maritime Arctic. Eastern Amundsen Gulf is closed except for narrow channels between Victoria Island and the continental landmass and between Victoria Island and Banks Island. Western Amundsen Gulf is connected to the Beaufort Sea through a broad outlet bounded to the north by Banks Island and to the south by Cape Bathurst. The ship's median daily location from January through April 2008 is indicated by one dot per day in .
Fig. 1 Amundsen Gulf in Canada's western Arctic with the locations of the CCGS Amundsen for January through April 2008. There is one dot per day indicating the median ship position for that day.
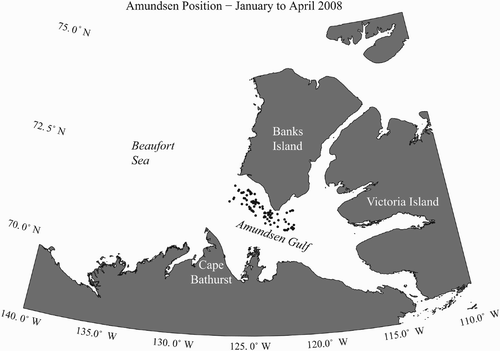
a Mesoscale Sea-Ice Concentrations
Landfast ice usually forms along the north, south, and eastern perimeters of Amundsen Gulf (Galley, Else, Howell, Lukovich, & Barber, Citation2012). Unconsolidated pack ice with a vast network of inter-ice-floe leads generally covers most of the Gulf and often persists from freeze-up in the autumn to break-up in spring. A flaw-lead polynya is a recurrent feature of the icescape (Barber & Hanesiak, Citation2004; Carmack & MacDonald, Citation2002; Galley et al., Citation2008).
For the 2008 winter, a time series of daily sea-ice concentration by type for Amundsen Gulf was obtained from the Canadian Ice Service (CIS) digital ice chart archive (EC, Citation2011). These weekly analyses integrate a number of sources including aerial surveys, satellite remote sensing (National Oceanic and Atmospheric Administration Advanced Very High Resolution Radiometer (NOAA AVHRR), passive microwave products, RADARSAT-1 Synthetic Aperture Radar (SAR), and RADARSAT-2 Advanced Synthetic Aperture Radar (ASAR)), as well as in situ observations. The CIS digital ice charts are created manually by expert ice analysts who derive the partial concentration for each of the standard ice types (EC, Citation2005) and the total sea-ice concentration for the Canadian Arctic. Fractional sea-ice concentrations are ratios, expressed in tenths, which describe the area of the water surface covered by a particular ice type. Total ice concentration is the sum of the partial ice concentrations expressed as a fraction of the total surface area (EC, Citation2005). Thus, ice concentrations are fractional surface areas. Agnew and Howell (Citation2003) showed that CIS digital data are useful for monitoring of sea ice on the mesoscale.
Weekly sea-ice charts between 7 January and 5 May 2008 were used to determine the partial and total sea-ice concentrations (in tenths) in Amundsen Gulf for the standard sea-ice types. The data from each CIS digital file were gridded at 2 × 2 km on a Lambert conformal conic projection. A 4 km2 grid cell was chosen because it was small enough to capture coastlines adequately while still allowing a manageable data volume.
b ABL Temperature Profiles
As part of the instrumentation aboard the CCGS Amundsen during the IPY-CFL field campaign (Barber et al., Citation2010), a Radiometrics MP-3000A© Microwave Radiometer (MWR) measured atmospheric temperature and vapour density profiles (at 50 m intervals from 0 to 500 m, at 100 m intervals from 500 to 2000 m, and at 250 m intervals from 2000 to 10,000 m) at various locations over Amundsen Gulf. The MWR viewed atmospheric radiances from the zenith in 12 channels; seven channels between 51 and 59 GHz provided information on the temperature profile, and five channels between 22 and 30 GHz provided information on the vapour density profile. The radiometric system rejected periods with precipitation when the profiles may have been erroneous because of moisture on the radome. Profiles were derived using the manufacturer's neural network retrieval and radiation transfer model that had been trained using measurements from the nearest operational upper-air station at Inuvik, Canada (68.30°N; 133.47°W). Explanations of radiometric profiling of temperature and moisture are available in Solheim et al. (Citation1998), Guldner and Spankuch (Citation2001), Ware et al. (Citation2003), Gaffard et al. (Citation2008) and elsewhere so no further explanation is provided herein.
A significant benefit of microwave radiometers over radiosondes is their high temporal resolution (approximately 1 minute). The IPY-CFL MWR soundings were averaged to generate hourly temperature profiles for January through April 2008. The intent of this processing was to obtain a manageable dataset while increasing the signal-to-noise ratio by smoothing out random errors.
Microwave radiometric profilers have been tested and found to have acceptable accuracy at a variety of locations (Cadeddu, Peckham, & Gaffard, Citation2002; Gaffard et al., Citation2008; Guldner & Spankuch, Citation2001; Knupp et al., Citation2009; Liljegren, Lesht, Kato, & Clothiaux, Citation2001). Throughout the IPY-CFL and during a subsequent field campaign in the Beaufort Sea, ArcticNet2009, weather balloons carrying Vaisala RS92-SGPD radiosondes were launched from the CCGS Amundsen to validate the MWR profiles. Salient details of the comparison between the MWR profiles and the radiosonde observations, available in Candlish, Raddatz, Asplin, and Barber (Citation2012), are summarized in Section 4c of this paper with potential sources of error discussed. The hourly IPY-CFL MWR profiles have previously been used to characterize the ABL over Amundsen Gulf (Raddatz et al., Citation2011) and to link the winter through early summer ABL profiles over the Gulf to its sea-ice cover (Raddatz et al., Citation2012).
c Cloud
A Vaisala CT25K© Ceilometer aboard the CCGS Amundsen measured cloud-base height continuously using laser backscatter caused by water droplets and ice crystals. Fog, precipitation, and diamond dust (ice crystals without cloud) between the ship and the cloud base may attenuate the cloud-base signal. Attenuation is taken into account by a normalizing process which identifies return signals originating from a cloud base (Vaisala, Citation2002). The ceilometer has a vertical resolution of 15 m (lowest possible cloud base is 15 m above the ship's deck) to approximately 7.6 km. The instrument was positioned to ensure a clear view of the sky.
The microwave radiometric profiler uses a zenith-pointing infrared radiometer (9.6–11.5 μm) to measure cloud-base temperature (if clouds are present). Cloud-base height is estimated by comparing the cloud-base temperature to the temperature profile (Ware et al., Citation2003).
3 Methodology
The Eulerian finite difference form of the heat continuity or thermodynamic energy equation (Eq. (1)) gives the rate of change of the mean temperature, (ΔTm (z )/Δt) of a layer of the atmosphere (Δz) as a result of the depletion (flux divergence, ΔQ(z)Flux) or accumulation (flux convergence, −ΔQ(z)Flux) of heat, plus the heating/cooling rate resulting from the phase change of water (ΔQ(z)Latent) within the layer (Arya, Citation2001; Brummer, Citation1997; Oke, Citation1987; Stull, Citation2000):
Equation (2) equates the heat flux divergence, ΔQ(z)Flux, for a layer of the ABL to the sum of ΔQ(z) R , the radiation heat flux divergence, ΔQ(z) Wm , the divergence of heat transported by the horizontal and vertical components of the mean wind (the former is termed advection and the latter subsidence or ascent), and ΔQ(z) Wt , the heat flux divergence due to the turbulent component of the wind. The last term reduces to the vertical exchange of sensible heat at the surface. Entrainment or the turbulent exchange of heat between the ABL and the free atmosphere is not a factor during the Arctic winter because the ABL is generally stable. High temporal resolution MWR soundings were averaged to generate hourly temperature profiles. Thus, it was assumed that the transfer of heat by the turbulent component of the horizontal wind, including heat transfer by sea-ice-edge turbulence, was included in the advection term. Possible warming (cooling) resulting from large-scale subsidence (ascent) was not considered a significant factor for the ABL.
By re-arranging Eq. (1), the heating/cooling rate for each layer of the ABL can be expressed as
By restricting the focus of this study to the near-surface layers of the ABL (0–1000 m) and to cloud-free hours when warming could be attributed to sensible heat flux from the surface, all the heating terms, except ΔQ(z) Wt , could be ignored. For these hours, sensible heat flux at the surface was obtained by integrating the simplified form of Eq. (3) from the surface, z = 0, to the top of the TIBL, z = h, defined as the level where the heat flux convergence dropped to ≤0 or began to increase with height (Arya, Citation2001). In finite difference form, the integral profile equation for the sensible heat flux at the surface (Q) becomes
In summary, ABL temperature profiles at close intervals (1 h averages) were used to determine near-surface sensible heat flux convergence profiles attributable to sensible heat flux from the surface (Arya, Citation2001). For these hours, the integration of the heat flux convergence through the near-surface layers, Eq. (4), gave estimates of the sensible heat flux from the unconsolidated sea-ice surface of Amundsen Gulf. The frequency distribution of sensible heat flux values was then plotted. A relationship between the sensible heat flux and the depth of the near-surface layer that it warmed (TIBL) was also determined.
4 Results and discussion
a Sea-Ice Concentrations
From 7 January to 5 May 2008, Amundsen Gulf was nearly, but not completely, ice covered. The total sea-ice concentration was generally 9.7/10, meaning that the combined fractional surface area of all types of ice was approximately 97%. This indicated that the pack ice remained unconsolidated with a network of inter-ice-floe leads and/or a polynya covering approximately 3% of the surface area. The fractional surface area of total sea ice is approximate because the numerical value of 9.7/10 is assigned to all sea-ice concentrations between 9/10 (unconsolidated pack ice consisting of very close ice floes), and 10/10 (pack ice composed of immobile consolidated ice) (EC, Citation2005).
The fractional surface area of the lead/polynya network in Amundsen Gulf was defined as the percentage of grid cells with open water, and new or young sea ice (<0.3 m thick) which contribute to the mesoscale sensible heat flux from the surface (Maykut, Citation1978). From 7 January to 5 May, the contributing fractional surface area ranged from near 0 to greater than 25% ().
b Surface Heat Flux
A sensible heat flux signature profile for heat flux convergence (heat accumulation) attributable to sensible heat flux from the unconsolidated sea-ice surface of Amundsen Gulf was derived from physical reasoning (Arya, Citation2001; Oke, Citation1987), an examination of selected sequences of hourly ABL temperature profiles, and the heat flux divergence/convergence profiles associated with the hour-to-hour changes in the temperature profiles. The sensible heat flux signature profile had the following features:
1. | Heat flux convergence (heat accumulation), rather than heat flux divergence (heat depletion), present in the surface layer (0–50 m). | ||||
2. | Heat flux convergence decreased with height from a maximum in the surface layer—the layer nearest to the heat source. | ||||
3. | Heat flux convergence dropped to zero or reversed sign in or below the 800–900 m layer, or the heat accumulation was restricted to the surface layer. | ||||
4. | Both the ceilometer and the MWR profiler recorded clear skies. |
On 14 February, changes in the ABL temperature profiles from 0500 to 0600 utc and from 0600 to 0700 utc are examples of warming caused by sensible heat flux from the unconsolidated sea-ice surface of Amundsen Gulf (a). The temperature of the ABL from the surface to about 400 m increased from 0500 to 0600 utc, and again from 0600 to 0700 utc. This near-surface portion of the ABL was stable (temperature increased with height) at 0500 utc, and it remained stable. Very small decreases in temperatures occurred in the inversion layer from 400 to 1000 m. These ABL temperature profiles with a weakly stable TIBL below an elevated inversion or residual RBL are commonly found over the unconsolidated sea-ice surface in Amundsen Gulf (Raddatz et al., Citation2011, Citation2012). Heat flux convergence (heat accumulation) for each 100 m layer from the surface to 1000 m was plotted at the mid-point of the layer (b). For both hours, the maximum heat flux convergence occurred in the near-surface layer, and the heat flux convergence decreased with height; it reversed sign above the 350–400 m layer for 0500–0600 utc, and above the 450–500 m layer for 0600–0700 utc. The sensible heat flux was 13.3 W m−2 for the first hour and 26.3 W m−2 for the second hour. These hours, and all hours with similar heat flux convergence profiles, were selected from the dataset.
Fig. 3 (a) ABL (0–1000 m) microwave radiometric temperature profiles for 0500, 0600, and 0700 utc, 14 February 2008. (b) Heat flux convergence profiles for 100 m layers (positive values indicate heat accumulation), 14 February 2008. From 0500 to 0600 utc, the mesoscale sensible heat flux = 13.3 W m−2, and the heat flux convergence reached 350 m. From 0600 to 0700 utc, the mesoscale surface heat flux = 26.3 W m−2, and the heat flux convergence reached 450 m.
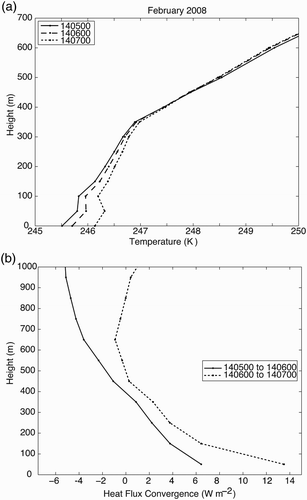
An example of warm air advection occurred on 14 April from 0700 to 0900 utc. The temperature of the ABL from the surface to 1000 m increased from 0700 to 0800 utc and again from 0800 to 0900 utc (a). The near-surface portion of the ABL (0–400 m) had a near-neutral lapse rate (approximately 0.91 K per 100 m) at 0700 utc, and it remained near neutral. From 400 to 1000 m the ABL was stable throughout the period. Heat flux convergence (heat accumulation) for each 100 m layer from the surface to 1000 m was plotted at the mid-point of the layer (b). The heat flux convergence profile for the first hour increased from the surface to a maximum in the 500–600 m layer, then decreased with height outlining an “advective nose.” The heat flux convergence profile for the second hour had a less pronounced advective nose with a maximum in the 200–300 m layer. These hours, and all hours with similar heat flux convergence profiles, were removed from the dataset.
Fig. 4 (a) ABL (0–1000 m) microwave radiometric temperature profiles for 0700, 0800, and 0900 utc, 14 April 2008. (b) Heat flux convergence profiles for 100 m layers (positive values indicate heat accumulation) from 0700 to 0800 utc and from 0800 to 0900 utc, 14 April 2008.
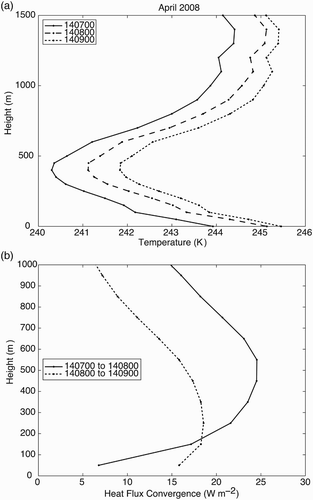
In summary, all heat flux convergence/divergence profiles that did not correspond with the sensible heat flux signature profile were filtered from the dataset. In particular, hourly heat flux convergence profiles that showed a typical warm advective nose (heat flux convergence increasing then decreasing with height forming an elevated maximum) were removed from the dataset. The 2839 heat flux divergence profiles associated with hourly temperature changes in the lower ABL, in the January to April 2008 period, yielded 520 clear-sky profiles that were attributed to sensible heat flux from the unconsolidated sea-ice surface of Amundsen Gulf. Plots of the 10, 50 (median), and 90th percentile values for 100 m layers from the surface to 700 m reveal that the heat flux convergence decreased logarithmically with height (). For the median profile, the best-fit curve was
Fig. 5 Median, 10, and 90th percentile heat flux convergence values attributed to sensible heat flux from the unconsolidated sea-ice surface for 100 m layers from the surface to 700 m. The best-fit curve for the median profile shows that the heat flux convergence decreased logarithmically with height.
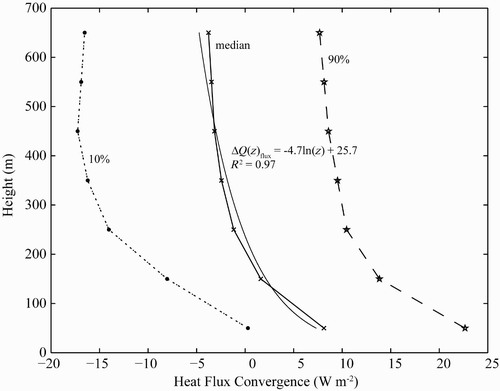
For these 520 hours, the sensible heat flux at the surface was obtained by integrating the heat flux convergence from the surface, z = 0, to the top of the TIBL, z = h, defined as the level where the heat flux convergence dropped to ≤0 or began to increase with height. The frequency distribution of the sensible heat flux estimates was plotted with the vertical axis expressed as the percentage of occurrences (). The mid-point of each class interval (0 < Q ≤10, 10 < Q ≤ 20, 20 < Q ≤ 30 … 180 < Q ≤ 190) is indicated on the horizontal axis. The sensible heat flux ranged from near 0 to 183 W m−2; 98% of the values were below 103 W m−2. The median was 11.4 W m−2; the average was 21.1 W m−2, and the mode was 0.1 W m−2. A mode value near zero is consistent with the modelling results of Lüpkes et al. (Citation2008). The sensible heat flux under clear skies persisted from one to nine hours. The length of these intervals was likely controlled by multiple effects including the movement of the ship and/or the sea ice, a change in the character of the approaching atmospheric flow, and/or the removal of all cloudy hours from the time series.
Fig. 6 Frequency distribution for 520 hourly values of mesoscale sensible heat flux from the unconsolidated sea-ice surface of Amundsen Gulf from January through April 2008.
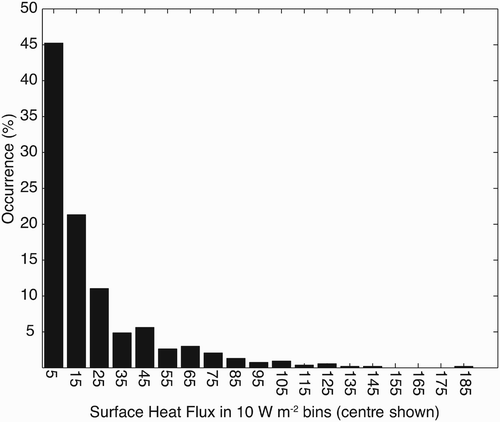
The vertical extent of the ABL layer, z = 0 to z = h, that experienced heat accumulation from sensible heat flux originating from the surface was 50 to 700 m; the median, mean, and mode were 150, 235, and 50 m, respectively. These TIBL heights are somewhat lower than the median height of 350 m found by Raddatz et al. (Citation2011) and the typical height of 400 m suggested by Overland and Guest (Citation1991). A relationship best described by a fourth-order polynomial was found between the magnitude of the sensible heat flux from the surface and the vertical extent of the heat flux convergence (). That is,
Fig. 7 Mesoscale sensible heat flux from the unconsolidated sea-ice surface of Amundsen Gulf versus the depth of heat flux convergence (heat accumulation).
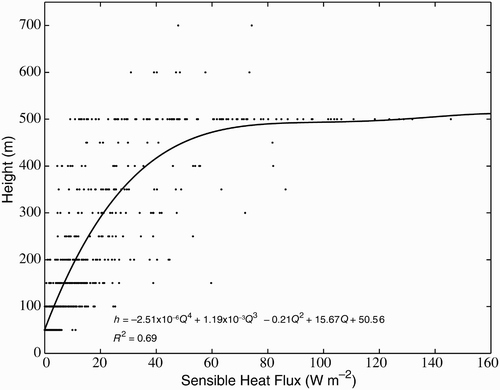
Although the precision of this relationship is limited by the finite difference approach with discrete steps in the heat flux convergence versus height plot, it indicates a nearly linear increase in h from 50 to 350 m as the sensible heat flux increased from near 0 to 28 W m−2. Above 80 W m−2, h plateaus at about 500 m.
c Sources of Error
There are two aspects of this analysis where errors may arise. These include (1) the measurement of the 0–1000 m ABL temperature profiles by the microwave radiometric profiler and (2) the simplifying assumptions applied to the thermodynamic energy equation and the methodology employed to identify the clear-sky heat flux convergence profiles attributable to mesoscale sensible heat flux from the surface.
Because the MWR temperature profiles were derived using the manufacturer's neural network retrieval and radiation transfer model that had been trained using historical radiosonde soundings from Inuvik, Canada, Candlish et al. (Citation2012) applied the Mann-Whitney two-sample difference test to five years (2002–06) of Inuvik radiosonde observations, and to the radiosondes launched from the CCGS Amundsen during the IPY-CFL and ArcticNet2009 field campaigns. This comparison showed that the latter were statistically similar to the historical Inuvik soundings when Amundsen Gulf had high concentrations of sea ice, as was the case during our study period. Candlish et al. (Citation2012) also compared the hourly average profiles from the MWR mounted on board the CCGS Amundsen with the hourly profiles from the radiometer operating at Barrow, Alaska. They found that the shipboard and land-based radiometers had similar accuracy statistics.
The average root mean square error (rmse) and bias of the CCGS Amundsen's MWR profiles from the surface to 1500 m (rmse = 1.5 K; bias = 0.7 K), using the radiosonde profiles (Vaisala RS92-SGPD) as the standard (Candlish et al., Citation2012; Raddatz et al., Citation2011), were consistent with earlier studies (e.g., Gaffard et al., Citation2008; Guldner & Spankuch, Citation2001; Liljegren et al., Citation2001). The estimated vertical resolution of the MWR profiler was 125 m up to 400 m (Cadeddu et al., Citation2002; Ware et al., Citation2003). Raddatz et al. (Citation2011) and Candlish et al. (Citation2012) concluded from the verification statistics, and from the ABL structure apparent in the radiometric soundings, that the MWR profiler was capable of accurately delineating the vertical profile of the ABL. In this study, potential errors resulting from inaccuracies in the MWR temperature profiles have been minimized by considering only the surface to 1000 m portion of the ABL and by using differences in the hourly temperature profiles, rather than the profiles themselves.
The thermodynamic energy equation used to determine the heat flux divergence profiles for the near-surface layers of the ABL is reliable because it is based on the fundamental principle of heat conservation (Arya, Citation2001) and measured temperature profiles. The integral profile method of determining the sensible heat flux from the surface avoids the empiricism of bulk flux relationships; however, some precision is lost by the use of a finite difference approach. The ABL temperatures, T(z), are often converted to potential temperatures, θ(z); however, with height as the vertical coordinate (θ(z) = T(z) + Γ dz, where Γ d is the dry adiabatic lapse rate (Stull, Citation2000)), this conversion was not necessary because the difference between the initial and the final values at a specified height, z, are the same for both temperature and potential temperature.
A close examination of the shape of each heat flux convergence profile effectively eliminated all cases of warm air advection. The retention of only heat flux convergence profiles under clear skies eliminated hours when cloud cover may have enhanced downwelling longwave radiation which could have contributed to warming of the near-surface layers and hours when the phase change of water could have been a factor (Curry et al., Citation1996). The influence of “diamond dust” or ice crystals on longwave radiation under clear skies, which was not recorded as a cloud base by either the ceilometers or the radiometer, was not considered. Although RFD may have enhanced or reduced the observed warming of the lower ABL by a few tenths of a degree per hour (Hoch et al., Citation2007; Stull, Citation2000), it was assumed that sensible heat flux was the driver of the observed changes in the near-surface temperature profiles because others have shown that RFD tends to lag the temperature tendency (Hoch et al., Citation2007).
5 Summary and conclusions
A January–April 2008 clear-sky time series of hourly radiometric ABL temperature profiles was used to establish the general shape or signature profile of heat flux convergence (heat accumulation) attributable to mesoscale sensible heat flux from the unconsolidated sea-ice surface of Amundsen Gulf. During this period, the fractional surface area that was open water or covered by thin ice (both surfaces contribute to the sensible heat flux from the surface) ranged from near 0 to greater than 25%. The sensible heat flux signature profile was then used to identify all heat flux convergence profiles attributable to sensible heat flux from the surface and to eliminate all the heat flux convergence profiles attributed to warm air advection. Only hours with clear skies were analyzed to eliminate heat flux convergence resulting from enhanced downwelling longwave radiation that often accompanies cloud cover and heating caused by the phase change of water. For the 520 clear-sky hours when the heat flux convergence profiles could be attributed to heat flux from the unconsolidated surface of Amundsen Gulf, plots of the 10, 50 (median), and 90th percentile values for 100 m layers from the surface to 700 m reveal that the median heat flux convergence profile decreased logarithmically with height. The sensible heat flux ranged from near 0 to 183 W m−2; 98% were below 103 W m−2. The median was 11.4 W m−2; the average was 21.1 W m−2, and the mode was 0.1 W m−2. This range of sensible heat flux values is consistent with that obtained from a literature search by Gultepe et al. (Citation2003). The vertical extent of the ABL that experienced heat accumulation from sensible heat flux originating from the surface was 50 to 700 m; h or TIBL median, mean, and mode depths were 150, 235, and 50 m, respectively. A relationship best described by a fourth-order polynomial was found between the magnitude of the sensible heat flux and the vertical extent of the heat flux convergence which indicated a nearly linear increase in h from 50 to 350 m as the sensible heat flux increased from near 0 to 28 W m−2, with a plateau in h at about 500 m for the sensible heat flux above 80 W m−2.
It was concluded that under clear skies (1) the median heat flux convergence profile resulting from mesoscale sensible heat flux from sea ice with near 0 to 25% open water and new or young ice (an unconsolidated sea-ice surface) decreased logarithmically with height, (2) although the mesoscale sensible heat flux from an unconsolidated sea-ice surface may exceed 100 W m−2, the most common value is <1 W m−2, and (3) the greater the mesoscale sensible heat flux from the unconsolidated sea-ice surface, the greater the depth of the TIBL (maximum of 700 m, but most frequently the warming resulting from sensible heat flux was restricted to the lowest 50 m). These conclusions, derived from field data collected during the IPY-CFL campaign, will contribute to our understanding of the thermal structure of the Arctic ABL over unconsolidated sea ice. This is a requirement for improving the characterization and modelling of the thermal character of the Arctic air mass that develops over the western maritime Arctic—an air mass that is a significant feature of the North American climate in winter.
References
- Agnew , T. A. and Howell , S. 2003 . The use of operational ice charts for evaluating passive microwave ice concentration data . Atmosphere-Ocean , 41 : 317 – 331 . (doi:10.3137/ao.410405)
- Anderson , P. S. and Neff , W. D. 2008 . Boundary layer physics over snow and ice . Atmospheric Chemistry and Physics , 8 : 3563 – 3582 . (doi:10.5194/acp-8-3563-2008)
- Andreas , E. L. and Cash , B. A. 1999 . Convective heat transfer over wintertime leads and polynyas . Journal of Geophysical Research , 104 : 25721 – 25734 . (doi:10.1029/1999JC900241)
- Andreas , E. L. , Guest , P. S. , Persson , P. O. G. , Christopher , C. W. , Horst , T. W. , Moritz , R. E. and Semmer , S. R. 2002 . Near-surface water vapor over polar sea ice is always near ice saturation . Journal of Geophysical Research , 107 ( C10 ) : 1 – 15 . doi:10.1029/2000JC000411
- Arya , S. P. 2001 . Introduction to micrometeorology , 2 , San Diego , CA : Academic Press .
- Barber , D. G. , Asplin , M. G. , Gratton , Y. , Lukovich , J. V. , Galley , R. J. , Raddatz , R. L. and Leitch , D. 2010 . The International Polar Year (IPY) Circumpolar Flaw Lead (CFL) system study: Overview and the physical system . Atmosphere-Ocean , 48 : 225 – 243 .
- Barber , D. G. and Hanesiak , J. M. 2004 . Meteorological forcing of sea ice concentrations in the southern Beaufort Sea over the period 1979 to 2000 . Journal of Geophysical Research , 109 : C6014 doi:10.1029/2003JC002027
- Bradley , R. S. , Keimig , F. T. and Diaz , H. F. 1992 . Climatology of surface-based inversions in the North American Arctic . Journal of Geophysical Research , 97 : 15699 – 15712 . (doi:10.1029/92JD01451)
- Brummer , B. 1997 . Boundary layer mass, water, and heat budgets in wintertime cold-air outbreaks from the Arctic sea ice . Monthly Weather Review , 125 : 1824 – 1837 . (doi:10.1175/1520-0493(1997)125<1824:BLMWAH>2.0.CO;2)
- Cadeddu , M. , Peckham , G. and Gaffard , C. 2002 . The vertical resolution of ground-based microwave radiometers analyzed through a multi-resolution wavelet technique . Geoscience and Remote Sensing, IEEE Transactions , 40 : 531 – 540 . (doi:10.1109/TGRS.2002.1000313)
- Candlish , L. M. , Raddatz , R. , Asplin , M. and Barber , D. G. 2012 . Atmospheric temperature and absolute humidity profiles over the Beaufort Sea and Amundsen Gulf from a microwave radiometer . Journal of Atmospheric and Oceanic Technology , 29 : 1182 – 1201 . doi:10.1175/JTECH-D-10-05050.1
- Carmack , E. C. and MacDonald , R. W. 2002 . Oceanography of the Canadian shelf of the Beaufort Sea: A setting for marine life . Arctic , 55 : 29 – 45 .
- Clapp , P. F. 1961 . Normal heat sources and sinks in the lower troposphere in winter . Monthly Weather Review , 89 : 147 – 162 . (doi:10.1175/1520-0493(1961)089<0147:NHSASI>2.0.CO;2)
- Curry , J. 1983 . On the formation of continental polar air . Journal of Atmospheric Science , 40 : 2278 – 2292 . (doi:10.1175/1520-0469(1983)040<2278:OTFOCP>2.0.CO;2)
- Curry , J. A. , Rossow , W. B. , Randall , D. and Schramm , J. L. 1996 . Overview of Arctic cloud and radiation characteristics . Journal of Climate , 9 : 1731 – 1764 . (doi:10.1175/1520-0442(1996)009<1731:OOACAR>2.0.CO;2)
- Drue , C. and Heinemann , G. 2007 . Characteristics of intermittent turbulence in the upper stable boundary layer over Greenland . Boundary Layer Meteorology , 124 : 361 – 381 . doi:10.1007/s10546-007-9175-8
- EC (Environment Canada) . 2005 . Manual of standard procedures for observing and reporting ice conditions , 9 , Ottawa , , Canada : Canadian Ice Service, Environment Canada . Retrieved from http://www.ec.gc.ca/glaces-ice/default.asp?lang=En&n=4FF82CBD-1
- EC (Environment Canada). (2011). The Canadian Ice Service Archive (CISA) [Data]. Retrieved from the Canadian Ice Service website: http://www.ec.gc.ca/glaces-ice/default.asp?lang=En&n=0A70E5EB-1
- Gaffard , C. , Nash , J. , Walker , E. , Hewison , T. J. , Jones , J. and Norton , E. G. 2008 . High time resolution boundary layer description using combined remote sensing instruments . Annales Geophysicae , 26 : 2597 – 2612 . (doi:10.5194/angeo-26-2597-2008)
- Galley , R. J. , Else , B. G. T. , Howell , S. E. L. , Lukovich , J. V. and Barber , D. G. 2012 . Landfast sea ice conditions in the Canadian Arctic: 1983–2009 . Arctic , 65 : 133 – 144 .
- Galley , R. J. , Key , E. , Barber , D. G. , Hwang , B. J. and Ehn , J. 2008 . Spatial and temporal variability of sea ice in the southern Beaufort Sea and Amundsen Gulf: 1980–2004 . Journal of Geophysical Research , 113 : C05S95 doi:10.1029/2007JC004553
- Garratt , J. R. 1990 . The internal boundary layer . Boundary Layer Meteorology , 50 : 171 – 203 . (doi:10.1007/BF00120524)
- Guldner , J. and Spankuch , D. 2001 . Remote sensing of the thermodynamic state of the atmospheric boundary layer by ground-based microwave radiometry . Journal of Atmospheric and Oceanic Technology , 19 : 925 – 933 . (doi:10.1175/1520-0426(2001)018<0925:RSOTTS>2.0.CO;2)
- Gultepe , I. , Isaac , G. A. , Williams , A. , Marcotte , D. and Strawbridge , K. B. 2003 . Turbulent heat fluxes over leads and polynyas and their effect on Arctic clouds during FIRE_ACE: Aircraft Observations for April 1998 . Atmosphere-Ocean , 41 : 15 – 34 . (doi:10.3137/ao.410102)
- Hoch , S. W. , Calanca , P. , Philipona , R. and Ohmura , A. 2007 . Year-round observation of long-wave radiative flux divergence in Greenland . Journal of Applied Meteorology , 46 : 1469 – 1479 . doi:10.1175/JAM2542.1
- Khvorostyanov , V. I. , Curry , J. A. , Gultepe , I. and Strawbridge , K. B. 2003 . A springtime cloud over the Beaufort Sea polynya: Three-dimensional simulation with explicit spectral microphysics and comparison with observations . Journal of Geophysical Research , 108 : D94296 doi:10.1029/2001JD001489
- Knupp , K. , Coleman , T. , Phillips , T. , Ware , R. , Cimini , D. , Vandenberghe , F. , Vivekanandan , J. and Westwater , E. 2009 . Ground-based passive microwave profiling during dynamic weather conditions . Journal of Atmospheric and Oceanic Technology , 26 : 1057 – 1073 . (doi:10.1175/2008JTECHA1150.1)
- Lee , S-H. and Kimura , F. 2001 . Comparative studies in the local circulations induced by land-use and by topography . Boundary Layer Meteorology , 101 : 157 – 182 . (doi:10.1023/A:1019219412907)
- Liljegren, J. C., Lesht, B. M., Kato, S., & Clothiaux, E. E. (2001). Evaluation of profiles of temperature, water vapor and cloud liquid water from a new microwave radiometer. 11th Atmospheric Radiation Measurement (ARM) Science Team Meeting, Atlanta, GA, 19–23 March. Retrieved from http://radiometrics.com/pubs.html
- Lüpkes , C. , Vihma , T. , Birnbaum , G. and Wacker , U. 2008 . Influence of leads in sea ice on the temperature of the atmospheric boundary layer during polar night . Geophysical Research Letters , 35 : L03805 doi:10.1029/2007GL032461
- Maykut , G. A. 1978 . Energy exchange over young sea ice in the central Arctic . Journal of Geophysical Research , 83 : 3646 – 3658 . (doi:10.1029/JC083iC07p03646)
- Oke , T. R. 1987 . Boundary layer climates , 2 , London : Methuen .
- Overland , J. E. and Guest , P. S. 1991 . The Arctic snow and air temperature budget over sea ice during winter . Journal of Geophysical Research , 96 : 4651 – 4662 . (doi:10.1029/90JC02264)
- Overland , J. E. , McNutt , S. L. , Groves , J. , Salo , S. , Andreas , E. L. and Persson , O. P. G. 2000 . Regional sensible and radiative heat flux estimates for winter Arctic during the Surface Heat Budget of the Arctic Ocean (SHEBA) experiment . Journal of Geophysical Research , 105 : 14093 – 14102 . (doi:10.1029/1999JC000010)
- Penner , C. M. 1955 . A three-front model for synoptic analyses . Quarterly Journal of the Royal Meteorological Society , 81 : 89 – 91 . (doi:10.1002/qj.49708134710)
- Persson , O. P. G. , Fairall , C. W. , Andreas , E. L. , Guest , P. S. and Perovich , D. K. 2002 . Measurements near the atmospheric surface flux group tower at SHEBA: Near-surface conditions and surface energy budget . Journal of Geophysical Research , 107 ( C10 ) : 8045 doi:10.1029/2000JC000705
- Raddatz , R. L. , Asplin , M. G. , Candlish , L. and Barber , D. G. 2011 . General characteristics of the atmospheric boundary layer in a flaw lead polynya region for winter and spring . Boundary Layer Meteorology , 138 : 321 – 335 . doi:10.1007/s10546-010-9557-1
- Raddatz , R. L. , Galley , R. J. and Barber , D. G. 2012 . Linking the atmospheric boundary layer to the Amundsen Gulf sea-ice cover: A mesoscale to synoptic-scale perspective from winter to summer 2008 . Boundary Layer Meteorology , 142 : 123 – 148 . doi:10.1007/s10546-011-9669-2
- Randall , D. , Curry , J. , Battisti , D. , Flato , G. , Grumbine , R. , Hakkinen , D. , Matrinson , R. , Preller , R. , Walsh , J. and Weatherly , J. 1998 . Status and outlook for large scale modeling of atmosphere-ice-ocean interactions in the Arctic . Bulletin of the American Meteorological Society , 79 : 197 – 219 . (doi:10.1175/1520-0477(1998)079<0197:SOAOFL>2.0.CO;2)
- Serreze , M. C. , Barrett , A. P. , Slater , A. G. , Steele , M. , Zhang , J. and Trenberth , K. E. 2007 . The large-scale energy budget of the Arctic . Journal of Geophysical Research , 112 : D11122 doi:10.1029/2006JD008230
- Solheim , F. , Godwin , J. R. , Westwater , E. R. , Han , Y. , Keihm , S. J. , March , K. and Ware , R. 1998 . Radiometric profiling of temperature, water vapor, and cloud liquid water using various inversion methods . Radio Science , 33 : 393 – 404 . (doi:10.1029/97RS03656)
- Stramler , K. , Del Genio , A. D. and Rossow , W. B. 2011 . Synoptic driven Arctic winter states . Journal of Climate , 24 : 1747 – 1762 . doi:10.1175/2010JCL13817.1
- Stull , R. B. 2000 . Meteorology for scientists and engineers , 2 , Pacific Grove , CA : Brooks/Cole .
- Vaisala . 2002 . Ceilometer CT25K user's guide , Helsinki , , Finland : Vaisala . M210345en-A edition. Retrieved from ftp://ftp.cmdl.noaa.gov/pub/Albee/_Arctic%20%20VALUABLE%20instrumention%20documentation/Vaisala/Ceilometer%20CT25K%20Users%20guide.pdf
- Ware , R. , Carpender , R. , Guldner , J. , Liljegren , J. , Nehrkorn , T. , Solhelm , F. and Vandenberghe , F. 2003 . A multichannel profiler of temperature, humidity, and cloud liquid . Radio Science , 38 : 8079 doi:10.1029/2002RS002856