Abstract
Fusarium head blight (FHB) is an important disease of wheat and causes significant economic and yield losses. The principal pathogen associated with FHB in Manitoba is Gibberella zeae (Schwein.) Petch (anamorph = Fusarium graminearum Schwabe). Trichoderma harzianum Rifai is a well-known biocontrol agent of many plant pathogens. The extracts from 11 T. harzianum isolates and cell- free filtrates were screened for antifungal activity against perithecial development. Isolates T472 and T22 (RootShield™ positive control) significantly reduced perithecia numbers by more than 70 %. It was found that isolates T472 and T22 had similar chemical composition. Through the use of cluster analysis, ordination and regression methods, several compounds (C18, C22, C27 and C42) were unique to T472 and T22 and may be responsible for the reduction in perithecia. A multivariate weighted average (WA) regression approach demonstrated that the use of chemical signatures is an effective method in predicting which isolates and compounds maybe be involved in reducing perithecial numbers. The R2 for the WA analysis was 0.745.
Résumé
La fusariose de l'épi (FE) est une maladie grave du blé qui cause d'importantes pertes tant sur le plan financier que sur celui des rendements. Au Manitoba, le principal agent pathogène associé à la FE est Gibberella zeae (Schwein.) Petch (forme imparfaite de Fusarium graminearum Schwabe). Trichoderma harzianum est un agent de lutte biologique courant, utilisé pour combattre plusieurs agents pathogènes chez les végétaux. Les extraits de 11 isolats et de filtrats sans cellules de T. harzianum ont été évalués en fonction de leur activité antifongique relativement au développement des périthèces. Les isolats T472 et T22 (Rootshield™ action positive) ont significativement réduit le nombre de périthèces (de plus de 70 %). Il a été démontré que les isolats T472 et T22 avaient une composition chimique semblable. Grâce à l'analyse des grappes et aux méthodes d'ordination et de régression, certains composés (C18, C22, C27 et C42) se sont avérés spécifiques de T472 et T22, et il est possible qu'ils soient responsables de la réduction du nombre de périthèces. Une approche faisant appel à une régression multivariée des moyennes pondérées (MP) a démontré que l'utilisation des signatures chimiques permet de prédire efficacement quels sont les isolats et les composés responsables de la réduction du nombre de périthèces. Le R2 découlant de l'analyse MP était 0.745.
Introduction
Members of the genus Trichoderma are well-known biocontrol agents and have been shown to control many plant pathogens, including Rhizoctonia spp., Botrytis spp., Sclerotinia spp. (Harman et al., Citation1993), Pythium spp. (Benhamou & Chet, Citation1997), Fusarium graminearum Schwabe (Fernandez, Citation1992; Inch & Gilbert, Citation2007), and Cochliobolus sativus (S. Ito & Kurib.) Drechsler ex Dastur (Fernandez, Citation1992). Commercial formulations of T. harzianum Rifai are available, including isolate T39 (TRIDODEX 20SP ™, Makhteshim Chemical Works, Israel) which is used for biological control of Botrytis cinerea (De Bary) Whetzel (Elad, Citation2000), and T22 (RootShield ™), registered for the biocontrol of various phytopathogens in greenhouse crops (Harman et al., Citation2004). Trichoderma species have a number of characteristics that are amenable to their usefulness in biological control, including being easily isolated from soil, plants and decaying wood, having a rapid growth rate, sporulating profusely and colonizing substrates very quickly (Howell, Citation2003).
Modes of action in biocontrol depend on the species and their interactions with target hosts. Rhizosphere competition by T. harzianum and induced localized or systemic resistance have been demonstrated to provide the plant with enhanced resistance to abiotic stresses, improved nutrient uptake efficiency, and protection from various plant pathogens (Harman et al., Citation2004). Other mechanisms of biocontrol include a combination of mycoparasitism, competition, and antibiosis (Howell, Citation2003; Harman, Citation2006). Trichoderma produces various cell wall-degrading enzymes (CWDEs), including proteases (Elad & Kapat, Citation1999), ß-1,3-glucanases (Cruz et al., Citation1995), and chitinases (Baek et al., Citation1999). These enzymes have been found to be essential for penetration and colonization of the host and ultimately cause cell death (Baek et al., Citation1999). They appear to be synergistic in their activity as greater biocontrol is achieved when a mixture of the CWDEs are used (Elad, Citation1996). The genus Trichoderma also produces a wide variety of volatile and non-volatile compounds that are effective in suppressing plant pathogens (Lorito et al., Citation1996). These compounds include alkyl pyrones (Claydon et al., Citation1987), isonitriles (Fujiwara et al., Citation1982), polyketides (Ordentlich et al., Citation1992), trichorzianines (Rebuffat et al., Citation1989), trichokindins (Iida et al., Citation1994), azaphilone, 1-hydroxy-3-methyl-anthraquinone, 1,8-dihydroxy-3-methyl-anthraquinone, butenolide, harzianolide and harzianpyridone (Vinale et al., Citation2009). The exact mode of action for most of these compounds is not known. Trichoderma species and related genera are also known to produce various types of antibiotic peptides, referred to as peptaibols. Over 200 peptaibols from various fungal species have been identified, sequenced and compiled in a database at http://www.cryst.bbk.ac.uk/peptaibol (Whitmore & Wallace, Citation2004).
The complex multivariate inter-relationships of compounds involved in biocontrol are not well understood and there are no published examples that attempt to predict possible bioactivity from chemical signatures. Cell-free filtrates of T. harzianum were able to reduce perithecial numbers when applied before or co-inoculated with G. zeae (Inch & Gilbert, Citation2007). This suggests that the cell-free filtrates have antimicrobial compounds that are effective in reducing perithecial development and are potential biopesticides. It has been demonstrated that metabolite production by T. harzianum is species- and strain-specific (Vinale et al., Citation2006).
High throughput screening is commonly used in the pharmaceutical industry when screening for new bioactive compounds. Isolation and characterization of new potential biocontrol strains can be expensive and time-consuming. Using reverse phase high performance liquid chromatography (RP-HPLC) and regression analysis may provide a method for rapid screening and identification of useful isolates. Thrane et al. (Citation2001) classified 44 Trichoderma strains using image analysis of complete chromatographic matrices obtained from HPLC. However, recent studies have focused on integration of descriptive methods with models that can predict the relationships between spectral patterns observed and sample composition (Nord et al., Citation2004). Zhang et al. (Citation2004) compared NIR spectral datasets from green tea to look at overall composition of peaks similar to HPLC spectra. This method could be implemented in biological control to predict the potential performance of a biocontrol agent based on spectral data, such as HPLC, prior to field trials. There has been little use of such approaches to develop predictive models of biological control from HPLC data.
The objectives of the study were to investigate the effect of secondary metabolites, produced in cell-free filtrates of T. harzianum, on the perithecial production of G. zeae and to identify unique biochemical signatures among isolates of Trichoderma that predict their bioactivity in inhibiting or reducing perithecia. This was addressed by: (1) identifying chemical signatures within isolates of Trichoderma that reduced perithecial production using bracketed alkylphenone RIs; (2) determining the multivariate associations among isolates of T. harzianum and similarities among their chemical composition based on RIs; (3) determining the relationship between chemical composition based on RIs and known levels of biocontrol activity; and (4) by developing a model to predict biocontrol efficacy from RIs.
Materials and methods
Isolates used
Ten T. harzianum isolates used in this study were obtained from the Canadian Collection of Fungal Cultures, Ottawa, and included T030 (DAOM 198030), T472 (DAOM 216472), T130 (DAOM 222130), T151 (DAOM 222151), T183 (DAOM 222183), T083 (DAOM 199083), T088 (DAOM 167088), T926 (DAOM 175926), T136 (DAOM 222136) and T137 (DAOM 222137). All isolates were identified and confirmed from ITS ribosomal sequence data. An additional isolate T-22 (strain KRL-AG2), which is registered in Canada as a biological control agent, RootShield™ (Bioworks, New York), was included as a positive control. The G. zeae isolate used was 1H (DAOM 192132) and was chosen based on aggressiveness and ability to produce perithecia on wheat straw under laboratory and field conditions. All cultures were grown on potato dextrose agar (PDA) (39 g Difco PDA, 1 L distilled water) for five to seven days under fluorescent white light at 20 °C. Cultures were then stored at 5 °C until needed.
Inoculum production
A macroconidial suspension of G. zeae was prepared using five to eight-day-old fungal cultures grown on PDA. The cultures were cut into 1 cm2 sections and added to streptomycin-amended CMC liquid medium (carboxymethyl cellulose 15 g, NH4NO3 1 g, KH2PO4 1 g, MgSO4·7H20 0.5 g, yeast extract 1 g, distilled water 1 L, streptomycin sulfate 0.2 g added to the cooled medium). The flask was attached to a vacuum line and air was bubbled gently through the medium to promote macroconidial production. After seven days at room temperature, macroconidia were counted using a hemacytometer and the suspension adjusted to a final concentration of 5.0 × 104 macroconidia mL−1 by adding sterile distilled water. A surfactant (Tween 20™) was added just prior to inoculation (0.2 mL per 100 mL inoculum).
Cell-free filtrates
Cell-free filtrates were prepared by inoculating 500 mL of liquid organic medium (glucose 10 g, peptone 1 g, yeast extract 0.1 g, KH2PO4 1 g, MgSO4·7H20 0.3 g, distilled water 1 L) with a 200 μL spore suspension (5.0 × 105 spores/mL) of T. harzianum. The flasks were placed on a rotary shaker set at 100 rpm under cool white fluorescent light (Sylvania cool white 30W) at room temperature for 10 days. The cultures were filtered through a Whatman #1 filter paper (Maidstone, England) and then through a 0.22 μm polyethersulfone, sterilizing, low-protein binding membrane (Corning, NY). Non-inoculated organic medium (as above) was also filtered and served as a control.
Extraction of secondary metabolites from cell-free filtrate
Organic compounds were extracted from the cell-free filtrates using diethyl ether and hexane. The cell-free filtrates were placed into a 1 L separatory funnel and ether was added (1/10 volume, i.e. 50 mL ether to 500 mL filtrate), and inverted several times to extract compounds. The aqueous phase was drained off and the organic phase containing the compounds was collected. This step was repeated three times to ensure that all organic compounds were extracted from the filtrate. Following this, 10 mL of hexane was added to the aqueous phase and inverted several times. Magnesium sulfate (MgSO4) was added to remove any remaining water and then was filtered to remove the residual MgSO4. The ether was evaporated using a rotovap, which left an oily residue on the bottom of the flask. This residue was re-suspended in 4 mL ether and transferred to 4 mL vials with Teflon-lined caps. Nitrogen gas was used to further concentrate the samples to 1 mL and the samples were then stored at −5 °C until needed.
Bioassay
The extracts were screened for antifungal activity by immersing pieces (1 cm long) of autoclaved wheat straw in 1 mL of concentrated ether extract for approximately one hour. Once all the ether was evaporated, 500 μL of a macroconidial spore suspension (5.0 x 104 spores mL−1) of G. zeae was applied. Treated pieces of straw were placed on moistened vermiculite and incubated at 20 °C under fluorescent white light. Numbers of mature perithecia were counted three weeks after inoculation. Treated straw inoculated with G. zeae only, and ether followed by G. zeae, were included as controls. Each treatment was replicated four times and the entire experiment using a randomized design was repeated once.
Reverse phase high performance liquid chromatography
Reverse phase high performance liquid chromatography (RP-HPLC) was performed on extracts of T. harzianum on a Waters 2690 separation module with autosampler and Waters 996 photodiode array detector. This was fitted with a 5 μm LiChrospher® 100 RP-18 guard column (LiChroCART® 4-4) and a reverse-phase 5 μm 250-4 LiChrospher® 100 RP column (LiChroCART® 4-4). Results were analyzed using Empower Software version 2.0. The column was eluted at a flow of 1 mL min−1 with a gradient using a solvent system composed of A: 0.1% H3PO4 - acidified water and B: HPLC grade acetonitrile. The gradient used for the analysis was: (time [min]/A[%]/B[%]) = 0/100/0, 5/95/5, 10/95/5, 14/90/10, 20/80/20, 23/80/20, 30/65/35, 35/65/35, 43/50/50, 48/25/75, 55/0/100, 60/0/100. Injection volumes were 50 μL per sample and each injection was replicated three times.
Calculation of retention index
Chromatographic peaks were monitored at 225 nm and characterized by their UV-VIS spectrum and alkylphenone RI. A series of internal alkylphenone standards (Frisvad & Thrane, Citation1987) were included: acetophenone, butyrophenone, decanophenone, hexaphenone, octophenone, propiophenone, valerophenone. The bracketed alkylphenone RIs were calculated from the following equation:
Determination of compounds from RIs
The compounds were labelled for analysis based on Thrane et al. (Citation2001). Each peak in the HPLC was identified and numbered. After all peak signatures were labelled, the compounds were aggregated based on similar RIs. The choice to merge or maintain a particular label was based on a visual assessment of the chromatogram and by preliminary statistical and multivariate analyses. Different RIs were assumed to represent unique compounds.
Data file structure and datasets
The data were analyzed as binary (presence or absence) and rank-order (abundance) data. For the binary data analysis, RI-based chemicals were considered as being ‘present’ (coded as 1) or ‘not present’ (coded as 0) (Thrane et al., Citation2001). For the binary data analysis, the influence of concentration of a particular compound was ignored and trace presence or abundance of a compound were both counted as ‘1’ or present (Legendre & Legendre, Citation1998).
The concentration of compounds was also analyzed using rank orders. The relative height of each peak on the chromatogram was used to rank the concentration of the compounds. The ranks were recorded in reverse order such that the largest value was associated with the highest peak for an isolate. The number of compounds identified within an isolate thus influenced the ‘size’ of largest rank value. Using a reverse rank order for concentration reflects the relationship in magnitude of the concentration without using absolute values.
Multivariate data analysis and software
Cluster analysis of the binary HPLC data was performed using the Jaccard coefficient measure of association (to form a distance matrix) followed by the unweighted pair group method with arithmetic mean (UPGMA). The Jaccard coefficient ignores shared zeros when calculating distances in a binary matrix which is preferred when abundant mutual absences are present in the dataset (Legendre & Legendre, Citation1998). Choice of UPGMA has the advantage of reducing the chaining effects in data where group structure may be weak (Grondona et al., Citation1997; Thrane et al., Citation2001). Ordination of these data was performed using principal coordinates analysis (PCoA, metric multidimensional scaling) using the Jaccard measure of association. PCoA is similar to principal components analysis (PCA) except that it can account for a variety of distance-based association measures (Legendre & Legendre, Citation1998) and differs from PCA in the fact that it recovers coordinates for the objects without retaining information about correlations among the variables.
Cluster analysis of the rank-order dataset was performed using a chord distance metric and UPGMA as the clustering algorithm (Grondona et al., Citation1997; Thrane et al., Citation2001; Nord et al., Citation2004). The chord distance is a standardized form of the Euclidean distance that scales object vectors to unit length (Legendre & Legendre, Citation1998). This method preserves relative magnitude but not absolute magnitude. Therefore, it is appropriate for data containing shared zeros and semi-metric data. Correspondence analysis (CA) was performed to ordinate the ranked data. CA is frequently used with rank-order data in social psychology and marketing and is commonly known as perceptual mapping or correspondence factor analysis (Torres & Greenacre, Citation2002). This method has been applied to fungal HPLC data (Sonjak et al., Citation2005) and can work with a variety of matrices consisting of positive values (Legendre & Legendre, Citation1998) or rank-order data (Faust & Wasserman, Citation1993).
To determine the relationship between perithecial reduction and chemical composition of the isolates, a weighted averaging calibration (WA) was performed. The WA predicts which chemical or combination of chemicals (weighted by the rank of the concentration) is effective in the reduction of perithecial production. To determine which compounds may be responsible for the decrease, tolerance down-weighting was used to eliminate the influence of compounds shared by all isolates, since these are unlikely to contribute to the reduction in numbers of perithecia.
All statistical analyses were performed using CRAN-R and the contributed packages in the CRAN repository (R Development Core Team Citation2009, see http://cran.r-project.org/). Contributed packages Analogue and Paltran were used to perform WA, CA was used to perform correspondence analysis, the package Vegan was used for computing associations for chord and Jaccard metrics, and Ade4 was used for PCoA.
Results
The RP-HPLC chromatographs revealed differences in the chemical signatures among isolates and secondary metabolites (chromatographs not shown). The efficacy of the 11 isolates tested is summarized in (observed perithecial inhibition). The initial data matrix consisted of 11 isolates by 107 RI compounds, of which 66 unique secondary metabolites were identified after aggregation of similar RIs. All analyses proceeded on 11 (isolates) × 66 (RI compounds).
Table 1. Statistics for the weighted averaging calibration (WA) analysis of perithecial reduction (%) and the observed and predicted values for perithecial reduction (%) of Gibberella zeae on straw treated with cell-free filtrate extracts of isolates of Trichoderma harzianum
The unweighted paired-group dendrogram of isolates using a Jaccard association matrix of binary relationships is presented in . Branch lengths indicated distance (differences) in the chemical composition of isolates. In this dendrogram, isolates with the highest reduction in perithecial development (T472 and T22) formed a tight cluster on the basis of having similar presence/absence patterns in peak concentrations as identified by HPLC. There was some evidence of chaining as observed in the tendency for groups to ‘grow’ through the addition of single isolates. For example, T926, T036, T030 and T188 are sequentially added to form the large group containing those members. This is often a side-effect of data containing large numbers of zeros that can affect multivariate data structure (Legendre & Legendre, Citation1998). The first two axes of the principal coordinates analysis of the 11 isolates based on the Jaccard association matrix is presented in . The first axis accounted for 25% of the dispersion in the distance matrix and clearly separated isolates T472 and T22 from the other isolates. Isolates associated with low-medium reduction in perithecial production showed no definite pattern on the ordination plot, similar to the results from the cluster analysis.
Fig. 1. Unweighted paired-group dendrogram of isolates using a Jaccard association matrix of binary relationships (presence-absence) of compounds detected in HPLC. Branch lengths indicate distance (differences) in the chemical composition of isolates.
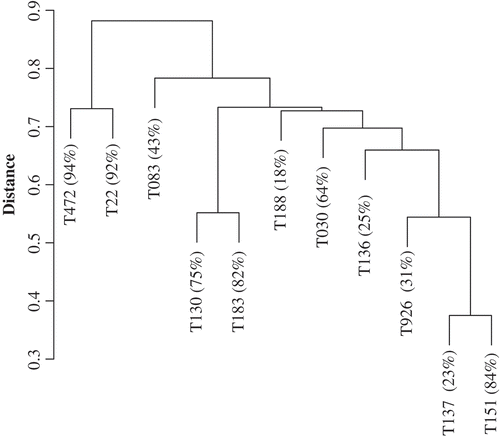
Fig. 2. The first two axes of the principal coordinates analysis of the 11 isolates based on a Jaccard association matrix calculated from the binary data. Percentage of the overall dispersion in the data matrix accounted for by each component is provided.
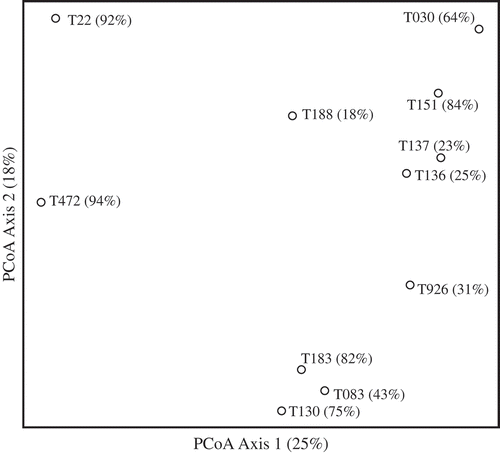
Unweighted paired-group dendrogram of isolates using a chord distance association matrix of concentration relationships (reverse rank-order) showed a three-group structure (). In , the mean percentage reduction in perithecia is also presented for each group. The between-group trends are clearer than they were in the analysis of the binary dataset. Typically, the level of reduction within each group was consistently either high, medium or low. Two of the three isolates in the leftmost class had the highest inhibition; the exception was T188. This isolate had the fewest number of compounds of all isolates tested, but of those, shared several with T472 and T22. For a similar reason – but to the opposite effect, T151 with 84% perithecial reduction was placed in a group that otherwise performed poorly in this regard (rightmost group).
Fig. 3. Unweighted paired-group dendrogram of isolates using a chord distance association matrix of concentration relationships (reverse rank-order) of compounds detected in HPLC. Branch lengths indicate distance (differences) in the chemical composition of isolates. The mean percentage of perithecial reduction is provided at the three group level and annotated on the dendrogram. Group 1 is reported with and without T188.
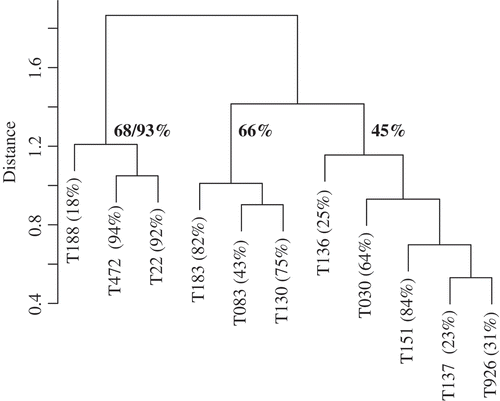
The correspondence between compound rank-order and the isolates tested in this study is provided in . Like the PCoA analysis, the first axis of the correspondence analysis extracted 25% of the total information in the dataset and separated isolates T472 and T22. Unlike the previous ordination, CA also provided an ordinate for each of the RI defined compounds in the study. Compounds C18, C22, C27 and C42 showed highest affinities to these two isolates, and occurred in both isolates (raw rank-order dataset, not published). However, they were not present in T188. As the RI labels were applied in order of polarity and size, these compounds eluted early indicating greater polarity and smaller size.
Fig. 4. Correspondence analysis of the rank-order concentration data. Isolate scores are indicated by a black dot and labelled appropriately. Compound scores are triangles, most compounds with large absolute scores are labelled for the purposes of discussion. Percentage of total inertia accounted for by each axis is provided.
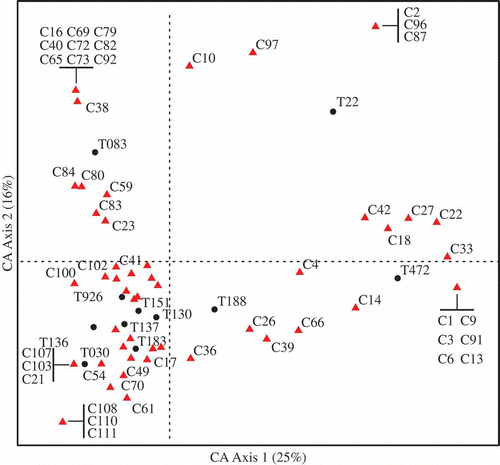
Results of the weighted averaging calibration model are presented in . The R2 for the model relationship was good at 0.745 with a root mean square error (RMSE) of 14% and a mean residual of zero. Isolates with the highest predicted perithecial reduction were T472 and T22 (). The WA plot of observed and fitted perithecial inhibition has relatively balanced residuals, but there is some potential for curvilinear relationship that is not incorporated into this model.
Discussion
The 11 Trichoderma isolates evaluated in this study showed a wide range of different secondary metabolite production and concentrations. The positive control, T22 (RootShield™) and T188 produced several similar compounds; however, the isolates differed greatly in their ability to reduce perithecial production. This may have been due to the fact that either the bioactive compound was not present in T188 or that the relative concentration of compounds was only approximately half that of compounds present in T22 and too low for reducing perithecia. Generally, isolates with similar chemical composition and ability to reduce perithecia clustered together, indicating that certain chemical combinations may be responsible for inhibition. However, caution must be used when screening potential biocontrol agents based on the metabolites produced. Development of resistance is a potential problem that may result in lower efficacy of control. Ajouz et al. (Citation2010) demonstrated that B. cinerea became resistant to pyrrolnitrin in five generations when exposed to high concentration of the chemical. Elad (Citation2000) demonstrated that Trichoderma isolates which produced various CWDEs were not always effective in biocontrol. In his study, all five isolates tested were shown to produce endochitinase, chitobiosidase and N-acetyl-B-D-glucosaminidase. However, only one isolate (a known biocontrol agent, T39) produced high concentrations of these enzymes. The other four isolates produced the enzymes but in lower quantities.
None of the compounds produced by the 11 isolates was able to be accurately identified using GC-MS chromatography with our existing chemical database. However, some generalizations can be made regarding the polarity and general composition of the chemicals. Based on the column and solvent used, small polar molecules would have eluted first from the column. These compounds were probably polar compounds, such as peptaibols. The compounds that eluted in the middle may have been larger aromatic hydrocarbons, similar to many polyketide-derived antibiotics, including harzianopyridone (Vinale et al., Citation2009), trichorzianines (Rebuffat et al., Citation1989), and 6-pentyl-α-pyrone (Claydon et al., Citation1987). These are commonly produced by T. harzianum and have antimicrobial properties. The compounds with the highest retention times could potentially be long chain, non-polar fatty acids, which may include ergosterol, a common component of fungal plasma membrane.
In RP-HPLC analysis, bracketed alkylphenone retention indices (RIs) may be used to indicate presence of specific compounds (secondary metabolites) or groups of compounds that share similar polarity and structural properties. Slight differences in retention times of a peak and RIs can result from compounds that are closely related. The chromatogram UV spectrum is used to determine whether compounds with similar RIs are the same or related compounds (Thrane et al., Citation2001). Peaks on the chromatograms that have similar shape and retention time are considered the same compound. When comparing several chromatograms from different RP-HPLC runs, the magnitude and shape of the chromatogram are sensitive to experimental conditions and sample concentration. In addition the concentration and types of secondary metabolites produced by an isolate can vary depending on growth medium, temperature, light and extraction method (Vinale et al., Citation2009). Therefore the protocols must be standardized to ensure consistency of the peaks in the chromatogram when comparing multiple runs. The use of RIs to determine presence or absence of specific peaks avoids problems associated either with magnitude or absolute concentration. The pattern and complexity of peaks in a chromatogram is a reflection of the presence of different secondary metabolites and these chemical signatures can be used for identification and classification through multivariate analysis (Cserhati, Citation2008). Image analysis of HPLC chromatograms has been shown to be effective at identifying Trichoderma isolates. Thrane et al. (Citation2001) concluded that secondary metabolite profiles are different enough to elucidate isolate differences. Compounds with similar retention times, RI values and UV spectrum can be considered the same compound. Nielsen & Smedsgaard (Citation2003) screened 474 mycotoxins and calculated the RI values for these compounds. However, the problem with using their RI values to classify unidentified compounds is the difficulty in reproducing exactly the HPLC methods they used. Temperature, solvent and column differences can all result in different retention times. Even multiple runs on the same column can result in differences due to drifting of the retention times. To avoid errors in the binary matrix, the RI values in this study were calculated based on the average retention time for three replicates. If a compound was identified in only one or two of the replicates, it was excluded from the analysis. Also to prevent cross-contamination between sample injections, a wash cycle was run each time between samples. The WA calibration model was able to predict biocontrol efficacy based on secondary metabolite composition spectrum. Several isolates of T. harzianum show promise for biocontrol. It was found that T. harzianum isolates that reduced perithecial development to the same degree had secondary metabolites of similar chemical composition and concentration. These compounds may be responsible for biocontrol. Inch and Gilbert (Citation2011) demonstrated that the main mechanisms for biocontrol of G. zeae is mostly through the production of secondary metabolites and not mycoparasitism. Compounds produced by T. harzianum appear to inhibit the development of mature perithecia. This study demonstrated that using chemical signatures in combination with multivariate analysis can limit errors due to subjective visual analysis of the chromatographic data (Thrane et al., Citation2001; Nielsen & Smedsgaard, Citation2003). Many regression and regression-like modelling approaches have been suggested, one of which is the application of multivariate calibration such as partial least squares (PLS) of the absorbance signals in chemometrics (Hassel et al., Citation2002; El-Gindy et al., Citation2004; Parisotto et al., Citation2007). The use of PLS in combination with spectrophotometric approaches has been shown to be effective in quantifying compounds in a multivitamin mixture. This method is cheaper and had fewer time consuming steps than using HPLC alone (El-Gindy et al., Citation2004). PLS regression analysis was also used to quantify organic and amino acids in beer using 1H NMR spectroscopy data (El Gindy et al., Citation2004). However, PLS is a linear model and many systems do not exhibit strict linearity (Hassel et al., Citation2002). When dealing with non-linear data, weighted averaging partial least squares (WA-PLS) (ter Braak & Juggins, Citation1993) or weighted averaging calibration can be used (ter Braak & van Dam, Citation1989). WA and WA-PLS have only recently been applied in chemometrics and in bioassay approaches. In conclusion, with further testing and development, the WA calibration prediction model approach may prove useful in the rapid screening of culture filtrates of T. harzianum isolates. The method used a regression model to predict the environment from species responses to the environment, suggesting that bioactivity is a direct function of secondary metabolites. The practical value of WA or WA-PLS approaches is that models could predict the performance of isolates prior to field studies. Furthermore, as suggested by El Gindy et al. (Citation2004), spectrophotometric analysis using WA-PLS performed well, compared with HPLC, at lower cost and fewer time-consuming steps. The model presented in this paper requires further validation using a larger set of T. harzianum isolates.
Acknowledgements
This work was funded by the Natural Sciences and Engineering Research Council of Canada (NSERC) and DOW AgroSciences. A special thanks to Lorne Adam and Abel el Hadrami for their technical assistance.
References
- Ajouz , S. , Nicot , P.C. and Bardin , M. 2010 . Adaptation to pyrrolnitrin in Botrytis cinerea and the cost of resistance . Plant Pathol. , 59 : 556 – 566 .
- Baek , J.M. , Howell , C.R. and Kenerly , C.M. 1999 . The role of extracellular chitinase from Trichoderma virens Gv29-8 in biocontrol of Rhizoctonia solani . Curr. Genet. , 35 : 41 – 50 .
- Benhamou , N. and Chet , I. 1997 . Cellular and molecular mechanisms involved in the interaction between Trichoderma harzianum and Pythium ultimum . App. Environ. Microbiol. , 63 : 2095 – 2099 .
- Claydon , N. , Allan , M. , Hanson , J.R. and Avent , A.G. 1987 . Antifungal alkyl pyrones of Trichoderma harzianum . Trans. Br. Mycol. Soc. , 88 : 503 – 513 .
- Cruz , J. , Pintor-Toro , J.A. , Benitez , T. and Llobell , A. 1995 . Purification and characterization of an endo-ß-1,6-glucanase from Trichoderma harzianum that is related to its mycoparasitism . J. Bacteriol. , 177 : 1864 – 1871 .
- Cserhati , T. 2008 . Multivariate methods in chromatography , John Wiley & Sons .
- Elad , Y. 1996 . Mechanisms involved in the biological control of Botrytis cinerea incited diseases . Eur. J. Plant Pathol. , 102 : 719 – 732 .
- Elad , Y. 2000 . Biological control of foliar pathogens by means of Trichoderma harzianum and potential modes of action . Crop Prot. , 19 : 709 – 714 .
- Elad , Y. and Kapat , A. 1999 . Role of Trichoderma harzianum protease in the biocontrol of Botrytis cinerea . Eur. J. Plant Pathol. , 105 : 177 – 189 .
- El-Gindy , A. , El-Yazby , F. , Mostafa , A. and Maher , M.M. 2004 . HPLC and chemometric methods for the simultaneous determination of cyproheptadine hydrochloride, multivitamins, and sorbic acid . J. Pharm. Biomedical Analysis , 35 : 703 – 713 .
- Faust , K. and Wasserman , S. 1993 . Correlation and association models for studying measurements on ordinal relations . Sociological Methodol. , 23 : 177 – 215 .
- Fernandez , M.R. 1992 . The effect of Trichoderma harzianum on fungal pathogens infesting wheat and black oat straw . Soil Biol. Biochem. , 10 : 1031 – 1034 .
- Frisvad , J.C. and Thrane , U. 1987 . Standardized high-performance liquid chromatography of 182 mycotoxins and other fungal metabolites based on alkylphenone retention indices and UV-vis spectra (diode array detection) . J. Chromatog. , 404 : 195 – 214 .
- Fujiwara , A. , Okuda , T. , Masuda , S. , Shiomi , Y. , Miyamoto , C. , Sekine , Y. , Tazoe , M. and Fujiwara , M. 1982 . Fermentation, isolation and characterization of isonitrile antibiotics . Agric. Biol. Chem. , 46 : 1803 – 1809 .
- Grondona , I. , Hermosa , R. , Tejada , M. , Gomis , M.D. , Mateos , P.F. , Bridge , P.D. , Monte , E. and Garcia-Acha , I. 1997 . Physiological and biochemical characterization of Trichoderma harzianum, a biological control agent against soil-borne fungal plant pathogens . Appl. Environ. Microbiol. , 63 : 3189 – 3198 .
- Harman , G.E. 2006 . Overview of mechanisms and uses of Trichoderma spp . Phytopathology , 96 : 190 – 194 .
- Harman , G.E. , Hayes , C.K. , Lorito , M. , Brodway , R.M. , Di Pietro , A. , Peterbauer , C. and Tronsmo , A. 1993 . Chitinolytic enzymes of Trichoderma harzianum: purification of chitobiosidase and endochitinase . Phytopathology , 83 : 313 – 318 .
- Harman , G.E. , Howell , C.R. , Viterbo , A. , Chet , I. and Lorito , M. 2004 . Trichoderma species–opportunistic, avirulent plant symbionts . Nature Rev. Microbiol. , 2 : 43 – 56 .
- Hassel , P.A. , Martin , E.B. and Morris , J. 2002 . Non-linear partial least squares. Estimation of the weight vector . J. Chemometrics , 16 : 419 – 426 .
- Howell , C.R. 2003 . Mechanisms employed by Trichoderma species in the biological control of plant diseases: the history and evolution of current concepts . Plant Dis. , 87 : 4 – 10 .
- Iida , A. , Sanekata , M. , Fujita , T. , Tanaka , H. , Enoki , A. , Fuse , G. , Kanai , M. , Rudewicz , P.J. and Tachikawa , E. 1994 . Fungal metabolites. XVI. Structures of new peptaibols, trichokindins I–VII, from the fungus Trichoderma harzianum . Chem. Pharm. Bull. , 42 : 1070 – 1075 .
- Inch , S. and Gilbert , J. 2007 . Effect of Trichoderma harzianum on perithecial production of Gibberella zeae on wheat straw . Biocont. Sci. Technol. , 17 : 635 – 646 .
- Inch , S and Gilbert , J. 2011 . Scanning electron microscopy observations of the interaction between Trichoderma harzianum and perithecia of Gibberella zeae . Mycologia , 103 : 1 – 9 .
- Legendre , P. and Legendre , L. 1998 . Numerical ecology , 2nd , Amsterdam : Elsevier Science .
- Lorito , M. , Woo , S.L. , D'ambrosio , M. , Harman , G.E. , Hayes , C.K. , Kubicek , C.P. and Scala , F. 1996 . Synergistic interactions between cell wall degrading enzymes and membrane-affecting compounds . Mol. Plant-Microbe Interact. , 9 : 206 – 213 .
- Nielsen , K.E. and Smedsgaard , J. 2003 . Fungal metabolite screening: database of 474 mycotoxins and fungal metabolites for de-replication by standardised liquid chromatography–UV–mass spectrometry methodology . Chromatogr. A , 1002 : 111 – 136 .
- Nord , L.I. , Vaag , P. and Duus , J.Ø. 2004 . Quantification of organic and amino acids in beer by 1H NMR spectroscopy . Anal. Chem. , 76 : 4790 – 4798 .
- Ordentlich , A. , Wiesman , Z. , Gottleb , H.E. , Cojocaru , M. and Chet , I. 1992 . Inhibitor furanone produced by the biocontrol agent Trichoderma harzianum . Phytochemistry , 31 : 485 – 486 .
- Parisotto , G. , Flores Ferrão , M. , Furtado , J.C. and Molz , R.F. 2007 . Determination of amoxicillin content in powdered pharmaceutical formulations using DRIFTS and PLS . Braz. J. Pharmaceut. Sci. , 43 : 89 – 96 .
- R Development Core Team . 2009 . R: A language and environment for statistical computing , Vienna : R Foundation for Statistical Computing .
- Rebuffat , S. , Mohamed , E.H. , Hennig , P. , Devoust , D. and Bodo , B. 1989 . Isolation, sequence, and conformation of seven trichorzianines B from Trichoderma harzianum . Int. J. Peptide Protein Res. , 34 : 200 – 210 .
- Sonjak , S. , Frisvad , J.C. and Gunde-Cimerman , N. 2005 . Comparison of secondary metabolite production by Penicillium crustosum strains, isolated from Arctic and other various ecological niches . FEMS Microbiol. Ecol. , 53 : 51 – 60 .
- Ter Braak , C.J.F. and Van Dam , H. 1989 . Inferring pH from diatoms: a comparison of old and new calibration methods . Hydrobiologia , 178 : 209 – 223 .
- Ter Braak , C.J.F. and Juggins , S. 1993 . Weighted averaging partial least squares regression (WA-PLS): an improved method for reconstructing environmental variables from species assemblages . Hydrobiologia , 269/270 : 485 – 502 .
- Thrane , U. , Poulsen , S.B. , Nirenberg , H.I. and Lieckfeldt , E. 2001 . Identification of Trichoderma strains by image analysis of HPLC chromatograms . FEMS Microbiol. Lett. , 203 : 249 – 255 .
- Torres , A. and Greenacre , M. 2002 . Dual scaling and correspondence analysis of preferences, paired comparisons and ratings . Int. J. Res. Marketing , 19 : 401 – 405 .
- Vinale , F. , Ghisalberti , E.L. , Sivasithamparam , K. , Marra , R. , Ritieni , A. , Ferracane , R. , Woo , S. and Lorito , M. 2006 . Major secondary metabolites produced by two commercial Trichoderma strains active against different phytopathogens . Lett. Appl. Microbiol. , 43 : 143 – 148 .
- Vinale , F. , Ghisalberti , E.L. , Sivasithamparam , K. , Marra , R. , Ritieni , R. , Ferracane , R. , Woo , S. and Lorito , M. 2009 . Factors affecting the production of Trichoderma harzianum secondary metabolites during the interaction with different plant pathogens . Lett. Appl. Microbiol. , 48 : 705 – 711 .
- Whitmore , L. and Wallace , B.A. 2004 . The Peptaibol Database: a database for sequences and structures of naturally occurring peptaibols . Nucleic Acids Res. , 32 : D593 – D594 . Database issue
- Zhang , M.H. , Xu , Q.S. and Massart , D.L. 2004 . Averaged and weighted average partial least squares . Analytica Chimica Acta , 504 : 279 – 289 .