Abstract
Damping-off disease can cause severe stand loss and reduce seedling vigour of kabuli chickpea (Cicer arietinum L.). While Pythium spp. are thought to be the primary cause of damping-off in chickpea, diversity and pathogenicity of other genera have not been widely explored. In surveys of three Montana fields of chickpea affected by damping-off, Fusarium spp. were isolated from 83% of the seeds tested, and were more common than the other taxa recovered, namely Pythium and Rhizopus. Fusarium spp. were identified to species using morphological characters and sequence analysis of two loci, the internal transcribed spacer (ITS) region of ribosomal DNA and the translation elongation factor (TEF) 1-α gene. Consistently identified species were F. oxysporum Schltdl. and F. redolens Wollenw. While discrepancies between identification methods made the identity of other isolates unclear, Fusaria infecting chickpea were diverse and varied across sites. The pathogenicity of eight isolates to kabuli chickpea was tested across a gradient of soil moisture and initial propagule density. All isolates were able to cause disease on kabuli chickpea seeds and seedlings. Effects of soil moisture levels on disease incidence varied among isolates, even isolates whose TEF 1-α sequences were 100% identical. This indicates that strains within a species can vary in pathogenicity. Additionally, most isolates caused more severe disease symptoms on cotyledons than roots.
Résumé
La fonte des semis peut causer de lourdes pertes au champ et réduire la vigueur des semis des pois chiches Kabuli (Cicer arietinum L.). Bien que Pythium spp. semblent être la cause première de cette maladie chez le pois chiche, la diversité et la pathogénicité d'autres genres n'ont pas été évaluées extensivement. Dans des études menées dans trois champs de pois chiches du Montana touchés par la fonte des semis, on a isolé Fusarium spp. de 83 % des semences testées et ceux-ci étaient plus courants que les autres taxons décelés, entre autres, Pythium et Rhizopus. Fusarium spp. ont été identifiés à des espèces qui utilisent les caractères morphologiques et l'analyse de séquence de deux locus, la région de l'espaceur transcrit interne (ETI) de l'ADN-r et le gène 1-α du facteur d'élongation de la traduction (FET). Les espèces qui y étaient identifiées invariablement incluaient F. oxysporum Schltdl. et F. redolens Wollenw. Tandis que des différences entre les méthodes d'identification rendaient l'identité des autres isolats incertaine, les fusariums infectant les pois chiches étaient différents et variaient d'un site à un autre. La pathogénicité de huit isolats de pois chiches Kabuli a été testée le long d'un gradient d'humidité de sol et de densité initiale de propagules. Tous les isolats ont pu transmettre la maladie aux semences et aux semis de pois chiches. Les effets des taux d'humidité du sol sur l'incidence de la maladie variaient chez les isolats, même chez ceux dont les séquences du gène 1-α du FET étaient identiques à 100 %. Ceci indique que des souches appartenant à une espèce peuvent varier quant à la pathogénicité. De plus, la plupart des isolats ont provoqué des symptômes plus graves sur les cotylédons que sur les racines.
Introduction
In North America, chickpea is grown primarily in California, Idaho, Montana, North Dakota, Oregon, South Dakota, and Washington, in the provinces Alberta and Saskatchewan, as well as parts of Mexico. Seed rot, pre-emergence damping-off and post-emergence damping-off (collectively called ‘damping-off’) can cause substantial stand loss and decreased plant vigor (Haware, Citation1998) and yields (Kaiser & Hannan, Citation1983; Trapero-Casas & Jiménez-Diaz, 1986; Trapero-Casas et al., Citation1990). This disease is characterized primarily by pre-emergence seed decay and cotyledon degradation, as well as discoloration and necrosis of roots (Kaiser & Hannan, Citation1983). Damping-off of chickpea has been primarily attributed to Pythium spp., in particular Pythium ultimum Trow (Kaiser & Hannan, Citation1983; Trapero-Casas et al., Citation1990; Haware, Citation1998), although Fusarium solani Mart. has also been reported to be associated with kabuli chickpea damping-off (Kaiser & Hannan, Citation1983; Brick et al., Citation1998; Haware, Citation1998; Hammon & Berrada, Citation2001; Chen et al., Citation2004). As with many seedling diseases, chickpea damping-off appears to be a function of environmental conditions (cool, wet weather) and inoculum density of the pathogens. Although studies testing seed treatments for damping-off in field settings have been conducted in the Great Plains states of the USA (e.g. Hammon & Berrada, Citation2001; Chen et al., Citation2004; Leisso et al., Citation2009), little published work exists addressing the identity of pathogens that cause damping-off in chickpea.
Both Fusarium spp. and Pythium spp. were isolated from kabuli chickpea affected by damping-off in Montana field trials at three locations in 2007 (Leisso et al., Citation2009). While root rot in chickpea can be caused by F. solani and F. oxysporum Schltdl. (Trapero-Casas & Jiménez-Díaz, Citation1985; Nene & Reddy, Citation1987; Nene & Sheila, Citation1996; Chen, Citation2008; Hasanzade et al., Citation2008), little is known about the ability of Fusarium spp. to cause damping-off of chickpea, with the exception of F. solani (Kaiser & Hannan, Citation1983; Chen et al., Citation2004) and F. equiseti (Corda) Sacc. (Goswami et al., Citation2008). Past research has indicated Fusarium spp. are most pathogenic in dry soils, and are frequently reported as important disease agents in dry conditions (Stover, Citation1953; Cook & Papendick, Citation1972; Cook, Citation1981).
The objectives of this study were to assess which pathogens were associated with damping-off of kabuli chickpea in Montana, determine the pathogenicity of the most commonly isolated Fusarium spp., and to determine how soil moisture and Fusarium spp. inoculum density affect disease incidence and severity.
Materials and methods
Recovery and identification of Fusarium spp. isolates
To assess the incidence and diversity of fungi associated with damping-off in chickpea, decaying kabuli chickpea (Cicer arietinum ‘Sierra’) seeds and seedlings were collected three to four weeks after planting from control field plots where no fungicide seed treatment was applied. These plots were part of a study of seed treatments to prevent damping-off (Leisso et al., Citation2009). Diseased seeds and seedlings were collected from three locations in Montana – Bozeman, Huntley, and Sidney – on 16 May, 17 May and 23 May 2007, respectively. During the three weeks following planting and prior to seed and seedling collection, Bozeman soil temperatures averaged 14.1 °C and plots received 42 mm of precipitation. At Huntley, the average soil temperature during the three weeks following planting was 15.4 °C and plots received 7 mm of precipitation. At Sidney, mean soil temperature was 14.2 °C, and plots received 49 mm of precipitation. Two rotting seeds or seedlings were collected from each of the six control treatment plots for a total of 12 seeds per location; seedlings showed evidence of germination such as elongation of the radical. Seeds or seedlings were collected from row sections showing low seedling emergence. Each seed or seedling was washed to remove excess soil, surface disinfested in 0.013% NaOCl for 30 s, rinsed three times with sterile de-ionized water, and divided into eight pieces; four pieces were plated on potato dextrose agar (PDA) amended with 50 ppm streptomycin and PARP (17 g corn meal agar, 5 ppm pimaricin, 250 ppm ampicillin, 10 ppm rifampin and 100 ppm pentachloronitrobenzene L−1). Each dish contained two pieces from each of the two cotyledons. Hyphal tips were subcultured to PDA and initially identified using morphological criteria (Van der Plaats-Niterink, Citation1981; Nelson et al., Citation1983; Barnett & Hunter, Citation1998; Leslie & Summerell, Citation2006).
For common genera of fungi/oomycetes (defined here as isolated from more than 10% of seeds per site), rates of infection and coinfection were calculated based on morphological identifications. Fusarium species were sequenced (see below) and identified with a BLAST search. To determine the composition of Fusarium species within each site, morphologically distinct isolates were identified using BLAST based on the internal transcribed spacer region (ITS) rDNA sequences. The number of unique isolates (with different ITS sequence) in each seed was used to calculate the richness and evenness of Fusarium species for each site using Simpson's index of diversity (Simpson, Citation1949). A sub-set of BLAST searches returned multiple species that matched the sequence to the same degree. These samples could represent one or several species. Community compositions of each site were compared using the later assumption. Differences among sites in frequency of genera/ITS isolates were tested using chi-square statistics.
Molecular identification
Eight Fusarium isolates were selected for characterization as representing the most commonly isolated morphological type from each site. Single spores of isolates were obtained by performing serial dilution plating of spores and transferring a single germinating spore to PDA. DNA was extracted from fungal mycelia using the E.Z.N.A. Fungal DNA kit (Omega Bio-tek, Doraville, GA) according to manufacturer's instructions. Approximately 250 mg of mycelia were aseptically removed from a one-wk-old colony of each isolate grown on PDA. Mycelia were placed in a sterile mortar, frozen with liquid nitrogen, and ground into fine powder. Approximately 100 mg of the pulverized mycelia was used for DNA extraction.
Primers ITS1F (Gardes & Bruns, Citation1993) and ITS4 (White et al., Citation1990) were used to amplify the rDNA ITS region. The 20 μL reaction mixture consisted of 1X GoTaq Master mix (Promega Corporation, Madison, WI) 1.25 μM primer ITS 1F, 1.25 μM primer ITS 4, and 85 ± 20 ng of extracted DNA. PCR conditions were denaturation at 95 °C for 5 min, followed by 35 cycles of denaturation at 95 °C for 30 s, annealing at 55 °C for 30 s, and extension at 72 °C for 1 min with a final extension step at 72 °C for 7 min. The amplification was performed using an iCycler Thermal Cycler (Bio-Rad Laboratories Ltd, Hemel Hempstead, UK). Amplified DNA was purified using QIAquick PCR purification kit (Qiagen Sciences Inc., Germantown, MD) and directly sequenced by Functional BioSciences of Madison, WI, using forward primer ITS1F.
The translation elongation factor (TEF) 1-α gene, which has the highest phylogenetic utility for identification of Fusarium spp. was also amplified and sequenced. Primers used were EF1 and EF2 (Geiser et al., Citation2004) and the reaction conditions were the same as described for amplification of the ITS region, with the exception of an annealing temperature of 53 °C. Amplified DNA was purified using QIAquick PCR purification kit (Qiagen Sciences Inc., Germantown, MD) and sequenced by Functional BioSciences of Madison, WI using forward primer EF1.
ITS region and TEF 1-α sequences were compared to sequence collections on GenBank (Benson et al., Citation2005) using BLAST (Altschul et al., Citation1990) algorithm ‘blastn.’. Translation elongation factor sequences were queried to the FUSARIUM-ID server at http://fusarium.cbio.psu.edu (Geiser et al., Citation2004) which contains a BLAST search tool. Sequences generated in this study have been deposited in the GenBank database under accession numbers listed in .
Effects of soil moisture and inoculum density of Fusarium spp. on disease incidence and severity
Eight Fusarium isolates were tested for pathogenicity to chickpea seeds across a range of soil moisture and inoculum densities in a growth chamber experiment. A factorial treatment design was used with four moisture levels (10, 20, 30 and 40%, v/v [volume water to volume sand]) and five inoculum levels (0, 10, 100, 1000 and 10 000 propagules g−1 of sand) for a total of 20 treatments. Published data relating soil volumetric water content and water/matric potential (Blank & Young, Citation1992) was used to parameterize the van Genuchten model (Citation1980) and estimate the matric potential of our moisture treatments. The 0.4, 0.3, 0.2 and 0.1 volumetric water content treatments were estimated to have matrix potentials of 0, −0.00038, −0.00157, and −0.00677 MPa. Moisture and inoculum levels were chosen based on results of preliminary experiments and previous legume studies (Kraft, Citation1975; Kaiser & Hannan, Citation1983; Trapero-Casas & Jiménez-Díaz, Citation1985). Sand (silica sand 20/30, Lane Mountain Co., Valley, WA) was chosen as the growth medium due to its neutral pH and inert properties (Dhingra & Sinclair, Citation1985).
Fusarium inoculum was produced in 25% potato dextrose broth (PDB, one-quarter of manufacturer's recommendations for g L−1, EMD Chemicals USA, Rockland, MA) by inoculating with three 7-mm diameter circular agar plugs cut from the edges of actively growing three to five day-old-cultures and filtering broth cultures through four layers of sterile cheesecloth after 15–20 days of growth. Conidia, chlamydospores, and hyphal fragments in solution were quantified using a haemacytometer (Hausser Scientific, Horsham, PA). Prior to sand inoculation, 250 μL of propagule solution was plated onto PDA and the percentage of conidia, chlamydospores, or hyphal fragments that were germinating and actively growing was quantified after 12 h incubation at 25 °C in the dark. Volumes of propagule solutions added to sand were adjusted by adding sterile tap water so that the inoculum potential was proportional to the number of viable propagules.
Sand moisture levels and inoculum concentration were achieved by aseptically mixing 70 g autoclaved sand (autoclaved twice, 24 h between each cycle) in individual sterile Petri dishes with sterile distilled water containing the appropriate quantity of inoculum using a flame-sterilized glass rod. Ten seeds of kabuli chickpea ‘Sierra’ with low resistance to seed-seedling disease were placed in each dish after treatments had been established. Seeds were surface-disinfested in 0.013% NaOCl for 3 min, rinsed for 30 s in 70% ethanol, and then rinsed thrice in sterilized de-ionized water and dried overnight (at least 10 h at room temperature ∼23 °C) on autoclaved paper towels in a laminar flow hood prior to use. Dishes were sealed with Parafilm to prevent moisture loss. No additional water was added to the dishes during the experiment. Dishes were placed in a growth chamber set at 15 °C/10 °C day/night regime, an optimal temperature range for chickpea germination (Ellis et al., Citation1986), and a 14 h photoperiod. Three replicates of the 20 treatments were arranged in a completely randomized block design with one replicate per block. The experiment was performed twice for each isolate.
Disease incidence and severity was measured 10 days after planting. The number of diseased seeds or seedlings was recorded, and cotyledon and root disease severity assessed visually according to percentage of necrotic cotyledon, epicotyl and hypocotyl tissue on a 1–5 scale modified from rating scales for legume seed and root diseases (Kraft, Citation1975; Trapero-Casas & Jiménez-Díaz, Citation1985; Peters & Grau, Citation2002) ().
Table 1. Cotyledon and root disease severity rating scale used in evaluating disease symptoms of kabuli chickpea seeds and seedlings infected by Fusarium spp
To complete Koch's postulates, five surface-disinfested, diseased seeds from each trial were plated on PDA for the re-isolation and identification of the inoculated-Fusarium spp.
Statistical analyses
Data analysis was performed using Statistical Analysis System (version 9.1, SAS Institute, Cary, NC). Residuals for each experimental parameter were examined for normality and homogeneity of variances. Assumptions of normality were examined using the Shapiro-Wilk's test (Shapiro & Wilk, Citation1965) performed with PROC UNIVARIATE. Homogeneity of variance between isolates and between experiments was examined using Hartley's F-max test (Hartley, Citation1950). Data were log transformed for analysis as necessary. Orthogonal polynomial statements were constructed in PROC GLM to compare disease incidence levels within the levels of soil moisture and inoculum density as well as to determine linear and quadratic trends in disease incidence as affected by soil moisture and propagule density. Multilinear regression using PROC REG was used to examine the linear relationship between moisture combined with inoculum density on disease incidence. Cotyledon and root disease severity data were analyzed using analysis of variance in PROC GLM. Letter groupings delineating equal means were generated by comparing results of the lsmeans pdiff option, which performs pair-wise comparisons of least squares means. Pearson's correlation coefficients were generated by comparing least square means of affected isolates of disease incidence, cotyledon disease severity and root disease severity. For all analyses, P < 0.05 was considered significant.
Results
Pathogen isolation and Fusarium spp. identification
Genera commonly isolated from kabuli chickpea seeds affected by damping-off were Fusarium, Pythium and Rhizopus. Multiple genera were isolated from 67% of seeds. Fusarium was the most common genus recovered, occurring on 83% of all seeds tested (). Pythium spp. and Rhizopus spp. were found on 50% and 47% of all seeds, respectively. Co-infection of Fusarium and Pythium was most common (28%), followed by Fusarium and Rhizopus (22%) and all three genera (14%). Interestingly, Pythium and Rhizopus were only isolated from the same seed once. Among seeds where a single genus was isolated, Fusarium was the most common (58%) while Pythium was less frequent (17%). Frequency of Fusarium recovery was consistently high across sites (χ2 = 1.2 w/2 d.f., P > 0.5). However, incidence of genera in the Huntley site, where Pythium was not isolated from any seeds and Rhizopus was isolated from twice as many seeds compared with the other sites, differed significantly (χ2 = 22.65 w/12 d.f., P < 0.05) from the other two sites, Bozeman and Sidney, where compositions of genera were similar (χ2 = 2.26 w/5 d.f., P > 0.1).
Table 2. Pathogens isolated from kabuli chickpea affected by damping-off in Montana field trials. Pathogens were isolated from 12 diseased seeds at each location
Communities of Fusarium species were similarly diverse across sites but differences in composition followed the same pattern seen in the analysis of common genera. Seven to eleven ITS species were isolated in each site and evenness (a measure of the degree to which communities are dominated by a single species) was similarly high across sites (). Distribution of Fusarium ITS differed significantly among sites (χ2 = 48.3 w/28 d.f., P < 0.001). Site differences in distribution are driven by, in order of statistical importance: (1) F. equiseti was commonly isolated in Huntley but not from the other two sites, (2) F. redolens Wollenw. was isolated only from Sidney and (3) F. oxysporum was absent in Huntley. Distribution was similar between the Bozeman and Sidney isolates (χ2 = 19.82 w/14 d.f., P = 0.071).
Table 3. Diversity of Fusarium isolates across three sites in Montana
Of the Fusarium isolates obtained from each location, several morpho-species were associated with damping-off at high frequency, and from these species, a subset of eight isolates was selected for pathogenicity testing (). The morphological, ITS and TEF identifications agreed to the species level for isolates 2.6 and 3.5 only. The morphological and TEF/Fusarium-ID identifications agreed for isolates 1.7, 2.2 and 3.2. Accession numbers and closest identities to Fusarium spp. of the isolates according to sequence comparison of the ITS rDNA region and TEF 1-α gene sequence to GenBank using BLAST as well as the Fusarium-ID database are listed in .
Table 4. Morphological and sequence-based identification of Fusarium spp. isolated from kabuli chickpea affected by damping-off in Montana
Effects of soil moisture and inoculum density of Fusarium spp. on disease incidence and severity
All Fusarium spp. isolates tested infected kabuli chickpea seeds and seedlings. Disease occurred at all moisture and inoculum density levels (). Low inoculum concentrations (10 propagules g−1 of sand) caused disease for all of the isolates, except for isolate 3.5 (F. oxysporum) whose disease incidence was not different from zero at P < 0.05 (). Disease incidence varied among isolates. Maximum incidence ranged from 100% for isolates 1.3 and 3.2, approximately 80% for isolates 1.7, 2.2 and 4.6, and 40–50% for isolates 2.6, 3.5 and D.I.
Fig. 1. Effects of moisture, inoculum density on disease incidence in chickpea seedlings across eight Fusarium spp. isolates under controlled conditions in growth chamber experiments. Inoculum densities are in propagules per gram of dry sand.
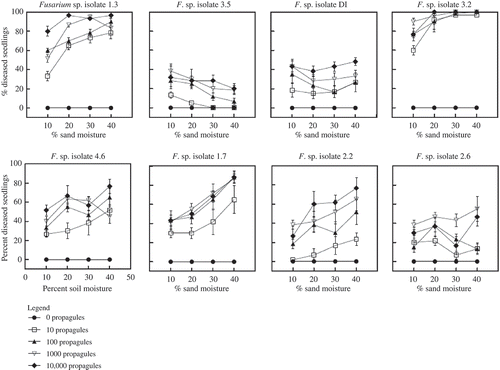
With few exceptions, high levels of disease incidence were observed by Fusarium isolates at relatively low inoculum density (< 100 propagules g−1 of sand). All of the isolates showed linear relationships between log propagule density and disease incidence at low inoculum densities (< 100 propagules g−1 of sand) but at higher inoculum densities, disease incidence was unaffected or reduced (quadratic term, P < 0.05) for six of the eight isolates ( and ).
Table 5. Analysis of variance and significance of contrasts for linear and quadratic trends of damping-off incidence of kabuli chickpea cause by Fusarium spp. in relation to moisture and inoculum density in growth chamber trials
Moisture and inoculum density had highly significant and independent (interaction, P > 0.05) effects on disease incidence for most isolates (). The relationship between soil moisture and disease incidence varied among isolates. Five of the eight isolates (1.3, 1.7, 2.2, 3.2 and 4.6) showed an increase in disease incidence with increasing soil moisture, while isolate 3.5 had an inverse relationship, and isolates DI and 2.6 exhibited a neutral relationship ( and ).
Cotyledons had higher severity ratings than roots for six of the eight isolates for data pooled over all moisture and inoculum levels (). Isolate 3.2 caused the most severe disease on cotyledons, followed by isolate DI. Isolate 3.2 caused the most severe disease on roots, followed by isolate 1.7.
Table 6. Disease severity of cotyledons and roots infected by Fusarium spp. isolates and correlation between disease incidence and severity of damping-off of kabuli chickpea caused by Fusarium spp. in growth chamber trials
Across treatments, conditions that facilitated infection were associated with increases in disease severity. Pearson's correlation coefficients indicated a positive relationship between disease incidence and severity for cotyledons and roots for all isolates except isolate 2.6 for cotyledons and isolate 2.6 and 4.6 for roots ().
Discussion
A range of Fusarium species appear to be common seed and seedling pathogens on chickpea. Diversity and incidence of infection was consistently high across sites, suggesting that disease was not affected by the range in abiotic conditions observed in this study. Furthermore, Fusarium was the most commonly isolated genus from seeds where only one genus was recovered. Rhizopus, generally considered as a secondary or opportunistic pathogen, was present in low numbers and usually (in 14 of 17 isolations) found in association with Fusarium. The pathogenicity of Rhizopus was not tested in this study.
Soil temperature and growing season precipitation may affect community composition of seed/seedling pathogens of chickpea. Huntley, which is warmer and drier than the other two sites that were wet and cool, showed a different composition of recovered genera and of Fusarium species. We also did not isolate Pythium from chickpeas at Huntley, probably due to the prevailing dry conditions, which is not conducive to Pythium infection (Agrios, Citation1997).
Disease severity was highest for isolates identified based on morphology as F. tricinctum (Corda) Sacc. and F. solani. This is consistent with previous reports of F. solani as a pathogen of chickpea (Kaiser & Hannan, Citation1983; Brick et al., Citation1998; Haware, Citation1998; Hammon & Berrada, Citation2001; Chen et al., Citation2004). Isolates obtained from Huntley had ITS sequences consistent with F. equisiti, which has been reported as a pathogen of several legume species including chickpea, where it was shown to cause seed decay and seedling disease (Goswami et al., Citation2008). Other studies have confirmed that diverse Fusarium species can infect chickpea seeds and seedlings. Bayraktar and Dolar (Citation2009) isolated several of the same morphological species as in this study, and F. redolens and F. acuminatum Ellis & Everh. have been reported to infect chickpea (Gambhir et al., Citation2010). Furthermore, the positive relationship, exhibited by most but not all isolates, between disease incidence and soil moisture, suggests that optimal conditions for infection differ among Fusarium spp. Past research has indicated that Fusarium spp. are favoured by dry soils, and are frequently reported as important pathogens under dry conditions (Stover, Citation1953; Cook & Papendick, Citation1972; Cook, Citation1981). However, our results indicate that for more than half of the isolates, disease incidence increased with increasing soil moisture. Three of these isolates were identified as F. solani according to morphological characters (1.3, 1.7 and 2.2). Other isolates were non-responsive (DI and 2.6; F. sambucinum Fuckel and F. redolens) or inversely affected by soil moisture (3.5; F. oxysporum).
Extrapolating the results of the pathogenicity experiment to field conditions is difficult. However, biological activity in the soil is often a function of water potential rather than soil water content. This suggests that while similar relationships between soil moisture and pathogenicity are expected in field soils, observed levels of pathogenicity are predicted to occur at higher soil volumetric water contents in field soils compared with our sand moisture treatments since increasing loam and clay content results in higher matric potentials for a given soil water content.
The increase in disease incidence with soil moisture may be due to effects of soil moisture on seed exudates. Soil moisture increases the quantity of seed exudates released from the seed during germination. As seed moisture increases, chickpeas likely exude higher quantities of compounds such as carbohydrates and amino acids, similar to peas (Cook & Flentje, Citation1967). These nutrient-rich exudates may stimulate pathogen propagule germination (Schroth & Snyder, Citation1961) and serve as a food source for both Fusarium spp. and other microorganisms (Singh & Merhota, Citation1980; Nelson, Citation1990). This process may also explain the increased disease severity on cotyledons compared with roots. Since the cotyledons represent the greatest volume of seed tissues, they would be the primary tissue releasing exudates and the first site of infection for Fusarium. In this study, cotyledon disease symptoms were more severe than root symptoms, and disease severity on cotyledons increased with moisture increase, while it did not increase on roots.
Kabuli chickpeas are predisposed to infection during the early seedling growth stage due to low seed defences (Kumar et al., Citation1991), a juvenile tissue-based susceptibility in the first 48 h of germination (Kaiser & Hannan, Citation1983), and the nature of their seed exudates, which, as a nutrient source, can increase pathogen inoculum potential. High soil moisture levels, which stimulate high seed exudate release, combined with low soil temperatures at planting (Ellis et al., Citation1986), likely increase the length of the juvenile tissue susceptibility (Kaiser & Hannan, Citation1983). These factors create a favourable environment for the initiation of damping-off disease.
While results from morphological studies, molecular identification, pathogenicity, and response to soil moisture suggest that multiple Fusarium species can cause seed and seedling disease in chickpea, further research is still needed to determine the diversity and identity of the Fusarium species associated with damping-off in chickpea. The Fusarium spp. tested for pathogenicity in this study were identified as F. solani, F. oxysporum, F. tricinctum, F. culmorum, F. sambucinum and F. redolens using morphological characters. Molecular identification was attempted with two commonly used sequences, the ITS region of rDNA and the TEF gene. However, with the exception of F. oxysporum and F. redolens, identification was inconsistent with the different methods and sequences were submitted to GenBank as ‘Fusarium spp.’ due to this lack of consistency. Furthermore, strain diversity within species appears to be present. For example, isolates with identical TEF 1-α gene sequences (isolates 4.6 and DI; isolates 1.7 and 2.2) responded differently in the pathogenicity assay. This is consistent with results from Hasanzade et al. (Citation2008) who tested 20 isolates of F. solani f. sp. pisi W.C. Snyder & H.N. Hansen on a susceptible cultivar of chickpea and found pathogenicity varied significantly between isolates.
Results of seed treatment studies in Montana in 2007 demonstrated that mefenoxam (marketed as Apron XL LS), which targets Pythium, was highly effective in controlling damping-off on chickpea, while seed treatments containing fluidoxonil (Maxim), targeting other fungi, were less effective (Leisso et al., Citation2009). This suggests that Pythium spp. (Kaiser & Hannan, Citation1983; Trapero-Casas et al., Citation1990) may be more important in causing damping-off in Montana than pathogens such as Fusarium spp. or that fludioxonil was not effective in controlling Fusarium. The interaction between Fusarium spp. and Pythium spp. in causing damping-off disease on chickpea was not explored in this study, but previous work has indicated that simultaneous infection by more one than one pathogen can cause higher levels of disease severity than either organism alone (Peters & Grau, Citation2002). We therefore recommend growers use a mix of fungicides to target both Pythium and Fusarium on chickpea.
Acknowledgements
The authors would like to extend their appreciation to O. Neher, E. Grimme, J. Holmes, M. Burgess, S. King, J. Eckhoff and B. Schaff for their helpful input and technical assistance. This work was supported by Interregional Project – 4 Biopesticide Grant No. 407-1409.
References
- Agrios , G.N. 1997 . Plant pathology , 4th , San Diego, CA : Academic Press .
- Altschul , S.F. , Gish , W. , Miller , W. , Myers , E.W. and Lipman , D.J. 1990 . Basic local alignment search tool . J. Mol. Biol , 215 : 403 – 410 .
- Barnett , H.L. and Hunter , B.B. 1998 . Illustrated genera of imperfect fungi , 4th , St. Paul, MN : APS Press .
- Bayraktar , H. and Dolar , F.S. 2009 . Genetic diversity of wilt and root rot pathogens of chickpea, as assessed by RAPD and ISSR . Turk. J. Agric. for , 33 : 1 – 10 .
- Benson , D.A. , Karsch-Misrachi , I. , Lipman , D.J. , Ostell , J. and Wheeler , D.L. 2005 . GenBank . Nucleic Acids Res , 36 : D23 – D26 .
- Blank , R. and Young , J. 1992 . Influence of matric potential and substrate characteristics on germination of Nezpar Indian Ricegrass . J. Range Manag , 45 : 205 – 209 .
- Brick , M.A. , Berrada , A. , Schwartz , H.F. and Krall , J. 1998 . Technical Bulletin TB 98-2: Garbanzo bean production trials in Colorado and Wyoming , Denver, CO : Colorado Agricultural Experiment Station and Wyoming Agricultural Experiment Station, Colorado Department of Education .
- Chen , W. 2008 . “ Chickpea diseases: ecology and control ” . In Encyclopedia of pest management , Edited by: Pimentel , D. Vol. 1 , 1 – 5 . London : Taylor & Francis .
- Chen , W. , Paulitz , T.C. , McPhee , K.E. and Muehlbauer , F.J. 2004 . Field evaluation of seed treatment fungicides for control of root rot and damping-off of chickpea, 2003 . Fungicide Nematicide Tests , 59 : ST017
- Cook , R.J. 1981 . “ Water relations in the biology of Fusarium ” . In Fusarium: diseases, biology, and taxonomy , Edited by: Nelson , P.E. , Toussoun , T.A. and Cook , R.J. 236 – 244 . University Park, PA : The Pennsylvania State University Press .
- Cook , R.J. and Flentje , N.T. 1967 . Chlamydospore germination and germling survival of Fusarium solani f. sp. pisi in soil as affected by soil water and pea seed exudation . Phytopathology , 57 : 178 – 182 .
- Cook , R.J. and Papendick , R.I. 1972 . Influence of water potential of soils and plants on root disease . Annu. Rev. Phytopathol , 10 : 349 – 374 .
- Dhingra , O.D. and Sinclair , J.B. 1985 . Basic plant pathology methods , Boca Raton, FL : CRC Press .
- Ellis , R.H. , Covell , S. , Roberts , E.H. and Summerfield , R.J. 1986 . The influence of temperature on seed germination rate in grain legumes II. Intraspecific variation in chickpea (Cicer arietinum L.) at constant temperatures . J. Exp. Bot , 37 : 1503 – 1515 .
- Gambhir , A. , Lamppa , R.S. , Rasmussen , J.B. and Goswami , R.S. 2010 . Evaluation of aggressiveness and host range of Fusarium acuminatum and Fusarium redolens associated with root rot of dry beans . Phytopathology , 100 : S185
- Gardes , M. and Bruns , T.D. 1993 . ITS primers with enhanced specificity for basidiomycetes – application to the identification of mycorrhizae and rusts . Mol. Ecol , 2 : 133 – 118 .
- Geiser , D.M. , Del Mar Jimenez-Gasco , M. , Kang , S. , Makalowska , I. , Veeraraghaven , N. , Ward , T.J. , Zhang , N. , Kuldau , G.A. and O'Donnell , K. 2004 . FUSARIUM-ID v. 1.0: A DNA sequence database for identifying Fusarium . Eur. J. Plant Pathol , 110 : 473 – 479 .
- Goswami , R.S. , Dong , Y. and Punja , Z.K. 2008 . Host range and mycotoxin production by Fusarium equiseti isolates originating from ginseng fields . Can. J. Plant Pathol , 30 : 155 – 160 .
- Hammon , R. and Berrada , A. 2001 . “ Evaluation of Kodiak® biological seed treatment to control seedling diseases of chickpea (summary) ” . In Western Colorado Research Center, 2001 Research Report. Tech. Rep. TR01-13 , Edited by: Caspari , H.R. , Godin , R.W. , Hammon , R. , Larsen , H.J. , Calvin , C.H. and Zimmerman , R. J. 16 Fort Collins, CO : Agricultural Experiment Station, Cooperative Extension, Western Colorado Research Centre, Colorado State University .
- Hartley , H.O. 1950 . The maximum F-ratio as a shortcut for heterogeneity of variance . Biometrika , 37 : 308 – 312 .
- Hasanzade , F. , Falahati Rastegar , M. , Jafarpour , B. and Kermani , M. 2008 . Identification of Fusarium solani f. sp. pisi, the cause of root rot in chickpea, and assessment of its genetic diversity using AFLP in Northeastern Iran . Res. J. Biol. Sci , 3 : 737 – 741 .
- Haware , M.P. 1998 . “ Diseases of chickpea ” . In The pathology of food and pasture legumes , Edited by: Allen , D. and Lenne , J. 473 – 516 . New York, NY : CAB International .
- Kaiser , W. and Hannan , R. 1983 . Etiology and control of seed decay and preemergence damping-off of chickpea by Pythium ultimum . Plant Dis. , 67 : 77 – 81 .
- Kraft , J.M. 1975 . A rapid technique for evaluating pea lines for resistance to Fusarium root rot . Plant Dis. Rep , 59 : 1007 – 1011 .
- Kumar , J. , Kaiser , W.J. and Hannan , R.M. 1991 . Damping off resistance in chickpeas . Plant Dis , 75 : 1244 – 1245 .
- Leisso , R.L. , Miller , P.M. and Burrows , M.E. 2009 . The influence of biological and fungicidal seed treatments on chickpea (Cicer arietinum L.) damping-off . Can. J. Plant Pathol , 31 : 38 – 46 .
- Leslie , J.F. and Summerell , B.A. 2006 . The Fusarium laboratory manual , Ames, IO : Blackwell Publishing .
- Nelson , E.N. 1990 . Exudate molecules initiating fungal responses to seeds and roots . Plant Soil , 129 : 61 – 73 .
- Nelson , P.E. , Toussoun , T.A. and Marasas , W.F.O. 1983 . Fusarium species: an illustrated manual for identification , University Park, PA : The Pennyslvania University Press .
- Nene , Y.L. and Reddy , M.V. 1987 . “ Chickpea diseases and their control ” . In The chickpea , Edited by: Saxena , M.C. and Singh , K.B . 233 – 270 . Wallingford, UK : CAB International .
- Nene, Y.L., & Sheila, V.K. (1996). Common names of plant diseases: diseases of chickpea (Cicer arietinum L.). Minneapolis, MN: The American Phytopathological Society. http://www.apsnet.org/publications/commonnames/Pages/Chickpea.aspx (http://www.apsnet.org/publications/commonnames/Pages/Chickpea.aspx) (Accessed: 6 April 2011 ).
- Peters , R.D. and Grau , C.R. 2002 . Inoculation with nonpathogenic Fusarium solani increases severity of pea root rot caused by Aphanomyces euteiches . Plant Dis , 86 : 411 – 414 .
- Schroth , M.N. and Snyder , W.C. 1961 . Effect of host exudates on chlamydospore germination of the bean root rot fungus, Fusarium solani f. phaseoli . Phytopathology , 51 : 389 – 393 .
- Shapiro , S.S. and Wilk , M.B. 1965 . An analysis of variance test for normality (complete samples) . Biometrika , 52 : 591 – 611 .
- Simpson , E.H. 1949 . Measurement of diversity . Nature , 163 : 688
- Singh , P.J. and Merhotra , R.S. 1980 . Relation between seed exudate and host susceptibility in gram (Cicer arietinum L.) to Rhizoctonia bataticola . Plant Soil , 56 : 265 – 271 .
- Stover , R.H. 1953 . The effect of soil moisture on Fusarium species . Can. J. Bot. , 31 : 693 – 697 .
- Summerell , B.A. , Salleh , B. and Leslie , J.F. 2003 . A utilitarian approach to Fusarium identification . Plant Dis. , 87 : 117 – 128 .
- Trapero-Casas , A. and Jiménez-Diaz , R.M. 1985 . Fungal wilt and root rot diseases of chickpea in southern Spain . Phytopathology , 17 : 1146 – 1151 .
- Trapero-Casas , A. , Kaiser , W.J. and Ingram , D.M. 1990 . Control of Pythium seed rot and preemergence damping-off of chickpea in the U.S. Pacific Northwest and Spain . Plant Dis. , 74 : 563 – 569 .
- Van Der Plaats-Niterink , A.J. 1981 . Monograph of the genus Pythium , Baarn, , the Netherlands : Centraalbureau Voor Schimmelcultures .
- Van Genuchten , M. 1980 . A closed-form equation for predicting the hydraulic conductivity of unsaturated soils . Soil Sci. Soc. Amer. J. , 44 : 892 – 898 .
- White , T.J. , Bruns , T. , Lee , S. and Taylor , J.W. 1990 . “ Amplification and direct sequencing of fungal ribosomal RNA genes for phylogenetics ” . In PCR protocols: a guide to methods and applications , Edited by: Innis , M.A. , Gelfand , D.H. , Sninsky , J.J. and White , T.J. 315 – 322 . New York, NY : Academic Press .