Abstract
Genetic diversity and relatedness in Pyrenophora tritici-repentis, the causal agent of tan spot disease of wheat, were examined in a geographically diverse collection of 80 isolates representing the eight races of the pathogen. Thirty-one simple sequence repeat markers were designed from the P. tritici-repentis genome which amplified 31 different loci in the isolates tested. Data were examined using: (i) Nei's analysis of genetic diversity and genetic distance, (ii) analysis of molecular variance (AMOVA), and (iii) clustering by the unweighted pair group method using arithmetic averages (UPGMA). A significant genetic differentiation (ΦPT = 0.153, P ≤ 0.001) was detected among populations, with isolates from different origins having similar levels of genetic diversity. Nei's analysis of genetic distance revealed that isolates of P. tritici-repentis can be grouped into four distinct populations based on their region of origin. The AMOVA showed that Canadian isolates have less than 9% genetic dissimilarity with isolates from the Caucasus region, while they have up to 32% genetic dissimilarity with isolates from the Fertile Crescent. Isolates were also compared by race, genetic distance and cluster analysis based on pooled allele frequency. Ptr ToxA non-producing isolates clustered together but were distantly related to Ptr ToxA-producing isolates, suggesting that the host-specificity imposed by the different Ptr toxins could lead to differentiation among isolates of P. tritici-repentis.
Résumé
La diversité génétique et la parenté chez Pyrenophora tritici-repentis, agent causal de la tache helminthosporienne du blé, ont été étudiées à partir d'une collection de 80 isolats de différentes provenances géographiques, représentant 8 races de l'agent pathogène. Trente-et-un marqueurs microsatellites ont été conçus à partir du génome de P. tritici-repentis, qui ont amplifié 31 locus différents dans les isolats testés. Les données ont été étudiées par : (i) analyse de Nei de la diversité et de la distance génétiques; (ii) analyse de la variance moléculaire (AMOVA); et (iii) méthode de groupement de paires non pondérées avec moyennes arithmétiques (UPGMA). Une différenciation génétique significative (ΦPT = 0,153, P ≤ 0.001) a été détectée chez les populations, les isolats d'origines différentes affichant des taux similaires de diversité génétique. L'analyse de Nei de la distance génétique a révélé que les isolats de P. tritici-repentis peuvent être regroupés en 4 populations distinctes, selon leur région d'origine. L'AMOVA a montré que les isolats canadiens affichent moins de 9 % de dissimilitude génétique par rapport aux isolats provenant du Caucase, tandis qu'ils en affichent jusqu'à 32 % par rapport à ceux du Croissant fertile. Les isolats ont également été comparés en fonction de la race, de la distance génétique et de l'analyse typologique basée sur la fréquence allélique dans la population. Les isolats non producteurs de Ptr ToxA se sont regroupés, mais étaient vaguement apparentés aux isolats producteurs de Ptr ToxA, ce qui suggère que la spécificité d'hôte imposée par différentes toxines Ptr pourrait induire la différenciation chez des isolats de P. tritici-repentis.
Introduction
Tan spot, caused by the ascomycete Pyrenophora tritici-repentis (Died.) Drechs. (anamorph: Drechslera tritici-repentis (Died.) Shoem.), is a destructive foliar disease of common wheat (Triticum aestivum L.) and durum wheat (Triticum turgidum L.). Yield losses under conditions favourable for tan spot development can be as high as 50% (Hosford, Citation1982; Rees & Platz, Citation1983). In Canada, the disease was first reported in the 1930s (Conners, Citation1934, Citation1939), but did not become a serious problem until the mid-1970s (Tekauz, Citation1976; Lamari et al., Citation2005 a). Increases in the incidence and severity of tan spot have been attributed to changes in agricultural practices, especially the shift to conservation tillage (Rees, Citation1982; Bockus, Citation1998). Wheat is the most widely cultivated crop in the world, with global trade in this commodity exceeding that of all other crops combined (Curtis, Citation2002). As such, yield losses caused by tan spot and other diseases can have a significant negative impact on the economy.
Tan spot is characterized by the development of two distinct symptoms on infected leaves: tan necrosis and extensive chlorosis (Lamari & Bernier, Citation1989). The development of these symptoms is highly specific and results from the interaction between pathogen-produced host-specific toxin(s) and their putative targets or receptors in the host (Strelkov & Lamari, Citation2003; Lamari & Strelkov, Citation2010). Three host-specific toxins are differentially produced by isolates of P. tritici-repentis and are considered to serve as pathogenicity factors for the fungus (Strelkov & Lamari, Citation2003; Lamari & Strelkov, Citation2010). Ptr ToxA is a 13.2 kDa protein that induces necrosis on the leaves of sensitive wheat genotypes, while Ptr ToxB and Ptr ToxC both induce chlorosis but on different wheat genotypes. Ptr ToxB is also a small protein, 6.6 kDa in mass (Strelkov et al., Citation1999), whereas Ptr ToxC appears to be a non-ionic, polar, low-molecular mass molecule (Effertz et al., Citation2002). In wheat, sensitivity to the toxins is controlled by single dominant and independently inherited genes, with one gene for each toxin (Faris et al., Citation1996; Gamba et al., Citation1998; Anderson et al., Citation1999; Effertz et al., Citation2002; Friesen & Faris, Citation2004).
Eight races of P. tritici-repentis have been identified to date, which are defined by their ability to produce the Ptr toxins and thereby cause necrosis or chlorosis on a set of wheat differential genotypes (Lamari et al., Citation1995, Citation1998, 2003). Isolates of the fungus classified as races 2, 3 and 5 produce one toxin each, namely Ptr ToxA, Ptr ToxC or Ptr ToxB, respectively. Isolates classified as races 1, 6 and 7 produce two toxins each (Ptr ToxA + Ptr ToxC, Ptr ToxB + Ptr ToxC, and Ptr ToxA + Ptr ToxB, respectively), while race 8 isolates produce all three toxins. In contrast, race 4 isolates do not produce any known toxins and are non-pathogenic (Strelkov & Lamari, Citation2003; Lamari & Strelkov, Citation2010).
Pyrenophora tritici-repentis is an interesting pathogen for evolutionary studies of populations. In addition to wheat, it infects a wide range of grass species. Of all Pyrenophora species, P. tritici-repentis is believed to have the widest host range and has been reported to infect 26 different species (Shoemaker, Citation1962; Morrall & Howard, Citation1975). It is also able to over-winter on a number of hosts and on infected wheat stubble (Morrall & Howard, Citation1975; Krupinsky, Citation1992). The fungus is a homothallic species in a genus composed primarily of heterothallic species, and occurs worldwide, even in regions where wheat is not cultivated (Strelkov & Lamari, Citation2003).
The greatest diversity with respect to virulence in P. tritici-repentis can be found in countries encompassing portions of the wheat centre of diversity, including Azerbaijan and Syria, with races 1, 2, 5, 7 and 8 identified in the former and races 3, 5, 7 and 8 found in the latter (Lamari et al., Citation2005 b). In contrast, nearly 20 years of surveying in Canada revealed that only races 1 and 2 occur commonly (Strelkov & Lamari, Citation2003). In North Africa (Algeria), races 5 and 6 have been identified (Lamari et al., 1995; Strelkov et al., Citation2002). The worldwide distribution of isolates producing different combinations of toxins, even in regions that are outside of the traditional wheat-growing areas, led to the hypothesis that P. tritici-repentis and its toxins evolved on native grass species local to each region, before it moved to its wheat host (Strelkov & Lamari, Citation2003). Under this scenario, the current race structure arose via toxin loss rather than acquisition and, even if some toxin-encoding genes were acquired by horizontal gene transfer, such an event would have had to occur in geological times (Strelkov & Lamari, Citation2003). Friesen et al. (Citation2006), on the other hand, suggested that P. tritici-repentis acquired the gene encoding Ptr ToxA via horizontal gene transfer as recently as the early 1940s, and that the pathogen was distributed globally in shipments of infected grain.
In order to evaluate the relationship between different isolates of P. tritici-repentis and to determine whether Canadian isolates share a common origin with Old World isolates, a collection of the pathogen from geographically distinct regions was characterized for genetic variation using simple sequence repeat (SSR) (≡ microsatellite) markers. Simple sequence repeats (SSRs) are short sequences of DNA one to six nucleotides in length that are repeated in tandem and randomly distributed in eukaryotic genomes (Karaoglu et al., Citation2005). The number of repeats in SSRs is highly variable, and polymorphisms in length can be detected via PCR amplication using specific primers that flank a repeat. Several studies have shown the utility of SSR markers for the analysis of genetic diversity in fungal plant pathogens (Peever et al., Citation2004; Breuillin et al., Citation2006; Rhaiem et al., Citation2008), and the availability of the complete genome sequence of P. tritici-repentis (http://www.broadinstitute.org) has enabled the rapid development of SSR markers for this fungus.
Materials and methods
Isolates of P. tritici-repentis
A collection of 80 isolates of P. tritici-repentis was used in this study (). This collection was obtained from naturally infected wheat plants sampled from Canada (North America), Azerbaijan (the Caucasus), Algeria (North Africa) and Syria (the Fertile Crescent). For the purpose of this study, isolates from each of the four countries are referred to as populations. The isolates were grown in the dark at room temperature (RT) on V8-potato dextrose agar in 9 cm-diameter Petri dishes (Lamari & Bernier, Citation1989) until they were 4–5 cm in diameter. Five plugs, 1 cm in diameter, were cut from each colony and transferred to 250 mL flasks containing 80 mL of Fries medium (Dhingra & Sinclair, Citation1986). The cultures were incubated in darkness at RT without agitation for three weeks. The mycelial mats were separated from the liquid medium by vacuum filtration through Whatman # 4 filter paper. The mycelial mats were then flash-frozen in liquid nitrogen and lyophilized in a freeze-drier prior to DNA extraction as described below.
Table 1. Isolates of Pyrenophora tritici-repentis analyzed in this study, including isolate name, race designation and geographic origin
DNA extraction
Genomic DNA was extracted from 40 mg of lyophilized mycelium with a Wizard® Genomic DNA Extraction Kit (Promega Corp, Madison, WI), according to the manufacturer's protocol for plant material. This was followed by two extractions with phenol chloroform (1:1 v/v) followed by one extraction with chloroform. The concentration and quality of the DNA was determined spectrophotometrically at A260, and adjusted to a final concentration of 20 ng μL−1 in TE buffer.
Identification of SSR loci
The genome sequence of P. tritici-repentis was downloaded from the intial sequence assembly of March 2007 [Pyrenophora tritici-repentis Sequencing Project, Broad Institute (http://www.broadinstitute.org)] and SSRs were identified using the SSR-finding software ssr.pl (Temnykh et al., Citation2001). A total of 946 putative microsatellites were identified, of which 633 were found to be located in unique single-copy regions. The occurrence and relative abundance of SSR loci in the P. tritici-repentis genome are summarized in .
Table 2. Simple sequence repeat loci identified in the genome of Pyrenophora tritici-repentis (Broad Institute), including motif length and copy number
Primer design and PCR amplification
Using Primer 3 software (Rozen & Skaletsky, Citation2000), 31 primer pairs were designed to amplify different single-copy SSR loci. These primers were selected to amplify a variety of different motifs and repeat numbers, and were located on all chromosomes of the sequenced genome with the exception of chromosome 9. The motif repeat, amplicon size and chromosomal location of the SSR loci used in the study are listed in .
Table 3. Simple sequence repeat loci utilized to assess genetic diversity and relatedness in a global collection of isolates of Pyrenophora tritici-repentis
Polymerase chain reactions were performed in 384-well plates (Applied Biosystems, Carlsbad, CA) in a thermocycler (MJ Research, Waltham, MA) in a 10 μL total volume, containing 20 ng genomic DNA, 1× PCR buffer (Invitrogen, Burlington, ON), 25 μM of each of the four dNTPs, 1.5 mM MgCl2, 0.3 pmol forward primer and 0.2 pmol reverse primer, 1.8 pmol M13-tail primer labelled with FAM, HEX or NED, and 0.875 U Taq DNA polymerase (Invitrogen). Reaction conditions consisted of an initial denaturation step at 95 °C for 2 min, followed by 30 cycles at 94 °C for 1 min, 58 °C for 50 s and 72 °C for 1 min, and a final extension at 72 °C for 5 min. Amplification products were resolved by capillary electrophoresis on an ABI-3100 Genetic Analyzer (Applied Biosystems). The sizes of the amplified SSR loci were determined using Genographer (Benham et al., Citation1999; http://genographer.com/).
Genetic diversity
The isolates of P. tritici-repentis were screened for variation at 31 SSR loci. Variations at these loci were used to determine the population structure of the pathogen. Data were recorded by assigning a letter code to each allele representing a different size amplicon. The percentage of polymorphic loci, gene diversity and allele frequencies for each locus were estimated with POPGENE Version 1.32 (Molecular Biology and Biotechnology Centre, University of Alberta, Edmonton, AB). Genetic diversity for the entire collection and genetic diversity within a population were denoted as H T and H S , respectively. Genetic diversity was calculated as H = (1 − ∑ Pi 2), where i is the frequency of allele i at the locus and averaged across loci (Nei, Citation1973). Genetic diversity values ranged from 0 (when the isolates all shared the same allele frequencies) to 1 (no alleles in common). Nei's unbiased measure of genetic distance within and between populations was also estimated using POPGENE. Pooled allele frequencies within each population were employed to generate a dendrogram based on Nei's genetic distance (D s) using the unweighted pair group method with arithmetic averaging (UPGMA). The consensus tree was displayed using TREEVIEW v. 1.6.6 (Page, Citation1996).
Analysis of molecular variance (AMOVA)
A total of four populations were defined for analysis of molecular variance (AMOVA) based on country of origin (Azerbaijan, Canada, Algeria and Syria). The null hypothesis of a lack of significant differences between isolates from different geographical origins was tested by AMOVA using GenAlEx6 (Peakall & Smouse, Citation2005). The data were converted into a matrix of squared distances between all pairs of the haploid isolates, from which it was possible to obtain an estimate of various variance components, designated as Φ-statistics as described in GenALEx6. The option for binary data without regional structure was used to calculate ΦPT as the proportion of the variance among populations relative to the total variance. ΦPT represents the correlation between individuals within a population, relative to the total:
where VAP is the variance among populations and VWP is the variance within populations. The significance of the variance component was tested using a permutational approach, eliminating the normal distribution assumption that is conventional for analysis of variance but inappropriate for molecular data (Excoffier et al., Citation1992). Race 4 isolate 90-2 from Canada was not included in the analysis of genetic diversity or AMOVA because it was the only non-pathogenic isolate in the collection and exhibited a unique allele size in seven of the 31 SSR loci. Therefore, including 90-2 in the above analyses as part of the Canadian population would have caused a bias in the results. This isolate was, however, included in the phylogenetic analysis in order to infer its relatedness to the other isolates. A second AMOVA test was carried out to test the difference within and between the different races, excluding isolates of races 4 and 6 because the number of isolates examined was insufficient for these races.
Cluster analysis
Cluster analysis based on the distance method UPGMA was used to identify isolates or populations of P. tritici-repentis that may have a common origin, and to determine which isolates are most closely related. All 80 isolates including the non-pathogenic race 4 isolate 90-2 were included in this analysis. A binary data matrix was generated for all loci based on the presence ( = 1) or absence ( = 0) of an amplification product at each locus. Genetic distance was calculated based on the Dice (Citation1945) coefficient of similarity, and clustering was performed by running the similarity matrix on the sequential, agglomerative, hierarchical and nested (SAHN) option (Sneath & Sokal, Citation1973) using UPGMA in the Numerical Taxonomy and Multivariate Analysis System of NTSYS-pc (version 2.1; Exeter Software, Setauket, NY).
Results
Genetic diversity and pathogen populations
A single band was observed for each locus from each isolate after PCR amplification, as expected for a haploid ascomycete fungus. One to 18 alleles were detected per locus among all isolates, with the percentage of loci polymorphic within a population ranging from 58% for the Syrian isolates to 90% for the Azerbaijani isolates (). Genetic diversity at each locus ranged from 0 to 0.9 and was higher in the Azerbaijani and Algerian populations relative to the Canadian and Syrian populations (). Total genetic diversity (H T) for the entire collection of analyzed isolates was estimated to be 0.38.
Table 4. Simple sequence repeat (SSR) polymorphic loci and genetic diversity
Genetic distance
An unrooted tree () was generated based on Nei's genetic distance. The UPGMA tree obtained using the pooled allele frequencies in each population revealed clustering of the four populations into three distinct groups. The first group consisted of the Azerbaijani and Canadian isolates, the second of the Algerian isolates, and the third of the Syrian isolates. The smallest genetic distance (Ds = 0.0603) was observed between the Canadian and Azerbaijani populations, while the highest genetic distance was detected between the Syrian and the Canadian populations (Ds = 0.2370).
Fig 1. Unrooted UPGMA tree based on Nei's genetic distance between populations of Pyrenophora tritici-repentis from Syria (SYR), Algeria (ALG), Canada (CAN) and Azerbaijan (AZ). The scale bar represents 1 substitution per site. UPGMA = unweighted pair group method using arithmetic averages.
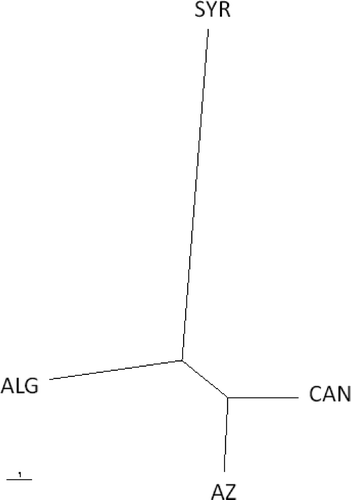
Genetic distance was also calculated in each of the tested races, with the corresponding UPGMA tree shown in . It was found that races 1 and 2 clustered together and were closely related to races 7 and 8, whilst races 3 and 5 clustered together but were distantly related to the other races. Races 4 and 6 were excluded from this analysis because of the small number of isolates analyzed from each of these races.
Fig 2. Unrooted UPGMA tree based on Nei's genetic distance between races 1, 2, 3, 5, 7 and 8 of Pyrenophora tritici-repentis. Races 4 and 6 were excluded from the analysis because only a very limited number of isolates from these races were available. The scale bar represents 1 substitution per site. UPGMA = unweighted pair group method using arithmetic averages.
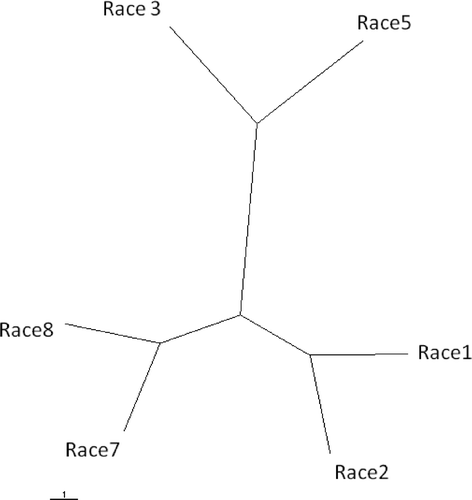
Analysis of Molecular Variance (AMOVA)
Results from AMOVA () revealed significant (P ≤ 0.001) genetic differentiation within and between pathogen populations from Azerbaijan, Canada, Algeria and Syria. Calculations of ΦPT showed that 15% of the variation occurred between populations and 85% of the variation was between isolates within populations. Isolates from Azerbaijan and Canada had the highest degree of similarity; genetic differentiation between these two populations was estimated to be 9% (ΦPT = 0.089, P = 0.001), whereas the highest genetic differentiation (32%) was found between isolates from Canada and Syria (ΦPT = 0.318, P = 0.001). A second AMOVA analysis was carried out by testing the genetic differentiation within and between the different races of the pathogen (). No significant differences (P > 0.001) were found between race 7 and races 2, 3 and 8, or between races 3 and 5. The highest significant genetic differentiation (34%) was found between isolates of races 2 and 3, and between isolates of races 3 and 8.
Table 5. Analysis of molecular variance between populations of Pyrenophora tritici-repentis originating from different countries
Table 6. Analysis of molecular variance in different races of Pyrenophora tritici-repentis
Similarity cluster (UPGMA) analysis
The genetic similarity dendrogram obtained using the Dice coefficient based on the UPGMA analysis is shown in . Two main clusters (C1 and C2) could be distinguished. Cluster C1 included mostly isolates belonging to races 1, 2, 7 and 8, whilst C2 included all race 3 isolates and most of the race 5 isolates. The two clusters shared 53% similarity. Most of the isolates within C1 were from Azerbaijan and Canada and shared more than 57% similarity, while C2 contained all of the Syrian and most of the Algerian isolates, as well as a few isolates from Azerbaijan and Canada (). All but five of the 55 isolates in C1 were Ptr ToxA-producers, while only two of the 24 isolates in C2 produced the toxin. The non-pathogenic isolate 90-2 was distinct from all other isolates and shared 25% similarity with the other isolates ().
Fig 3. Dendrogram obtained by UPGMA analysis showing the genetic similarity of a global collection of isolates of Pyrenophora tritici-repentis. The vertical lines define the two main clusters. Asterisks denote Ptr ToxA-producing isolates of the fungus. UPGMA = unweighted pair group method using arithmetic averages.
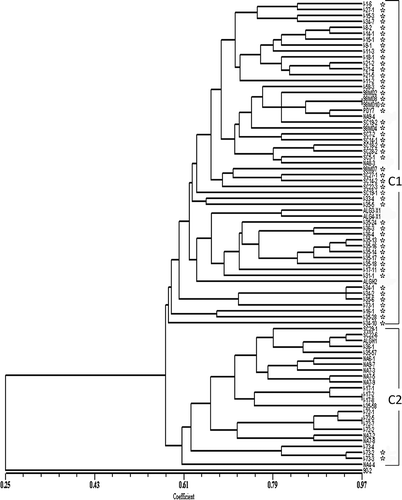
Discussion
Simple sequence repeat markers were used to assess the level of genetic diversity and relatedness within a global collection of P. tritici-repentis. The isolates in this collection were grouped into four distinct groups, which corresponded to their geographical origins, based on Nei's analysis of genetic distance. The Canadian and Azerbaijani populations clustered together in terms of genetic distance, while the greatest distance was found between the Canadian and Syrian populations (). In general, the results of the UPGMA cluster analysis and those of AMOVA were consistent with those of Nei's analysis of genetic distance, in that the Canadian isolates were closest to the Azerbaijani isolates. This finding is consistent with the hypothesis that the North American isolates of P. tritici-repentis were derived from populations introduced into the New World (Friesen et al., 2006). Alternatively, since both Canadian and Azerbaijani isolates produce Ptr ToxA, it is also possible that the clustering reflects the host specificity imposed by the Ptr toxins. While it takes the transfer of only a single toxin gene to alter the race designation of a particular isolate, over time the host specificity imposed by the different toxins could lead to additional genetic differentiation, through reproduction on and adaptation to different host genotypes. Indeed, when calculating the genetic distance, isolates representing races 3 and 5, which do not produce Ptr ToxA, were genetically the most distant from isolates representing races 1, 2, 7 and 8, which do produce this toxin (). In UPGMA cluster analysis (), C2 included all race 3 isolates and most of the race 5 isolates. Most of the isolates in C2 lack the ability to produce Ptr ToxA. The only two Canadian isolates in this cluster are classified as race 3, while the Azerbaijani isolates found in this cluster are classified as race 5.
The current findings are in agreement with previous research by Aung (Citation2001), who utilized random amplified polymorphic DNA (RAPD) markers to examine the relationship between isolates of P. tritici-repentis representing different races of the pathogen. In that analysis, significant differences were observed between necrosis-inducing (Ptr ToxA-producing) race 1 and 2 isolates and non-necrosis inducing (Ptr ToxA non-producing) isolates representing races 3, 5 and 6. Aung (Citation2001) found that Ptr ToxA-producing isolates shared 48% similarity with Ptr ToxA non-producing isolates, and that non-pathogenic isolates shared 26% similarity with pathogenic isolates. In the current study, 53% similarity was found between Ptr ToxA-producing and non-producing isolates, while only 25% similarity was observed between pathogenic isolates and the non-pathogenic isolate 90-2 (). The distinct nature of 90-2 is consistent with an earlier report in which major proteome-level differences were found between pathogenic and non-pathogenic isolates of the fungus (Cao et al., Citation2009).
In contrast, an amplified fragment length polymorphism (AFLP)-based analysis of P. tritici-repentis isolates, conducted in conjunction with sequence analysis of the internal transcribed spacer region of the ribosomal DNA, revealed no relationship between isolate grouping and race or geographic origin (Friesen et al., Citation2005). The isolates included in that study were collected from North and South America and Europe (Friesen et al., Citation2005), while the isolates included in the current study originated from Canada and the wheat centre of diversity. The SSR markers employed were designed to represent each chromosome of the pathogen. In some cases, SSR loci on the same chromosome were expected to be more than 2 Mb apart from each other (). Therefore, the current results likely provide a more representative view of the diversity of the entire genome relative to previous studies.
Nei's analysis of genetic diversity revealed that populations from Syria, Canada, Azerbaijan and Algeria had similar levels of genetic diversity, with slightly higher genetic diversity values found for the isolates from Azerbaijan and Algeria. While variation in population size (i.e. the number of isolates) could influence the calculation of genetic diversity, the overall results did not change when calculations were repeated with reduced numbers of isolates (data not shown). Higher genetic diversity was expected in populations of P. tritici-repentis from Azerbaijan and Algeria, relative to Canadian populations of the pathogen, since Azerbaijan and Algeria are found within regions considered part of the wheat centre of diversity. It has long been known that the centre of diversity of a host may also represent the centre of greatest variability of its pathogens (Vavilov, Citation1951). Based on the virulence phenotypes and race composition of P. tritici-repentis isolates from Syria (Lamari et al., Citation2005 b), greater genetic diversity would also have been expected within the pathogen population from that country. It is possible that the number of Syrian isolates analyzed (eight) was too small to accurately reflect the extent of the genetic diversity within the population.
In summary, analysis of the results obtained with the SSR markers revealed that isolates of P. tritici-repentis grouped according to geographic origin, but that these different populations possessed a similar level of genetic diversity. The considerable genetic differentiation observed between Ptr ToxA non-producing and Ptr ToxA-producing isolates suggests that the host specificity imposed by the Ptr toxins could influence the evolution of P. tritici-repentis and its races.
Acknowledgements
The authors wish to thank Andrzej Walichnowski, Elsa Reimer (Agriculture and Agri-Food Canada) and Ralph Kowatsch (University of Manitoba) for technical assistance. They are also grateful to Travis Banks (Agriculture and Agri-Food Canada) for downloading the P. tritici-repentis genome and searching for possible SSR loci. The help of Xiban Wang (Agriculture and Agri-Food Canada) in data analysis is also gratefully acknowledged. Financial support was provided by the Natural Sciences and Engineering Research Council of Canada, a University of Manitoba Graduate Fellowship, and the A.W. Henry Endowment Fund (University of Alberta).
Notes
†Deceased 11 December 2009.
References
- Anderson , J.A. , Effertz , R.J. , Faris , J.D. , Francl , L.J. , Meinhardt , S.W. and Gill , B.S. 1999 . Genetic analysis of sensitivity to Pyrenophora tritici-repentis necrosis-inducing toxin in durum and common wheat . Phytopathology , 89 : 293 – 297 .
- Aung , T.S.G. 2001 . Molecular polymorphism and virulence in Pyrenophora tritici-repentis , Winnipeg, , Canada : M.Sc. Thesis, University of Manitoba .
- Benham , J. , Jeung , J.-U. , Jasieniuk , M. , Kanazin , V. and Blake , T. 1999 . Genographer: A graphical tool for automated fluorescent AFLP and microsatellite analysis . J. Agric. Genomics , 4 : 3
- Bockus , W.W. 1998 . Control strategies for stubble-borne pathogens of wheat . Can. J. Plant Pathol. , 20 : 371 – 375 .
- Breuillin , F. , Dutch , C. and Robin , C. 2006 . Genetic diversity of the Chestnut blight fungus Cryphonectria parasitica in four French populations assessed by microsatellite markers . Mycol. Res , 110 : 288 – 296 .
- Cao , T. , Kim , Y.M. , Kav , N.N.V. and Strelkov , S.E. 2009 . A proteomic evaluation of Pyrenophora tritici-repentis, causal agent of tan spot of wheat, reveals major differences between virulent and avirulent isolates . Proteomics , 9 : 1177 – 1196 .
- Conners , I.L. 1934 . 14th Annual Report of the Canadian Plant Disease Survey , 100 Ottawa : Canada Department of Agriculture, Experimental Farms Branch .
- Conners , L.L. 1939 . 19th Annual Report of the Canadian Plant Disease Survey , 12 – 14 . Canada Department of Agriculture, Division of Botany and Plant Pathology .
- Curtis , B.C. 2002 . “ Wheat in the world ” . In Bread wheat improvement and production , Edited by: Curtis , B.C. , Rajaram , S. and Gómez Macpherson , H. Vol. 30 , 1 – 17 . Rome, , Italy : FAO Plant Production and Protection Division .
- Dhingra , O.D. and Sinclair , J.B. 1986 . Basic plant pathology methods , 285 – 315 . Boca Raton, FL : CRC Press .
- Dice , L.R. 1945 . Measures of the amount of ecologic association between species . Ecology , 26 : 297 – 302 .
- Effertz , R.J. , Meinhardt , S.W. , Anderson , J.A. , Jordahl , J.G. and Francl , L.J. 2002 . Identification of a chlorosis-inducing toxin from Pyrenophora tritici-repentis and the chromosomal location of an insensitivity locus in wheat . Phytopathology , 92 : 527 – 533 .
- Excoffier , L. , Smouse , P.E. and Quattro , J.M. 1992 . Analyses of molecular variance inferred from metric distances among DNA haployypes: application to human mitochondrial DNA restriction data . Genetics , 131 : 479 – 491 .
- Faris , J.D. , Anderson , J.A. , Francl , L.J. and Jordahl , J.G. 1996 . Chromosomal location of a gene conditioning insensitivity in wheat to a necrosis-inducing culture filtrate from Pyrenophora tritici-repentis . Phytopathology , 86 : 459 – 463 .
- Friesen , T.L. and Faris , J.D. 2004 . Molecular mapping of resistance to Pyrenophora tritici-repentis race 5 and sensitivity to Ptr ToxB in wheat . Theor. Appl. Genet. , 109 : 464 – 471 .
- Friesen , T.L. , Ali , S. , Klein , K.K. and Rasmussen , J.B. 2005 . Population genetic analysis of a global collection of Pyrenophora tritici-repentis, causal agent of tan spot of wheat . Phytopathology , 95 : 1144 – 1150 .
- Friesen , T.L. , Stukenbrock , E.H. , Liu , Z. , Meinhardt , S. , Ling , H. , Faris , J.D. , Rasmussen , J.B. , Solomon , P.S. , McDonald , B.A. and Oliver , R.P. 2006 . Emergence of a new disease as a result of interspecific virulence gene transfer . Nat. Genet , 38 : 953 – 956 .
- Gamba , F.M. , Lamari , L. and Brûlé-babel , A.L. 1998 . Inheritance of race specific necrotic and chlorotic reactions induced by Pyrenophora tritici-repentis in hexaploid wheats . Can. J. Plant Pathol , 20 : 401 – 407 .
- Hosford , R.M. Jr. 1982 . “ Tan spot – developing knowledge 1902–1981, virulent races and wheat differentials, methodology, rating systems, other leaf diseases, literature ” . In Tan spot of wheat and related diseases workshop , Edited by: Hosford , R.M. Jr. 1 – 24 . Fargo, ND : North Dakota Agric. Exp. Station .
- Karaoglu , H. , Lee , C.M.Y. and Meyer , W. 2005 . Survey of simple sequence repeats in completed fungal genomes . Mol. Biol. Evol. , 22 : 639 – 649 .
- Krupinsky , J.M. 1992 . Grass hosts of . Pyrenophora tritici-repentis. Plant Dis. , 76 : 92 – 95 .
- Lamari , L. and Bernier , C.C. 1989 . Evaluations of wheat lines and cultivars to tan spot Pyrenophora tritici-repentis based on lesion type . Can. J. Plant Pathol , 11 : 49 – 56 .
- Lamari , L. , Sayoud , R. , Boulif , M. and Bernier , C.C. 1995 . Identification of a new race of Pyrenophora tritici-repentis: implications for the current pathotype classification system . Can. J. Plant Pathol. , 17 : 312 – 318 .
- Lamari , L. , Gilbert , J. and Tekauz , A. 1998 . Race differentiation in Pyrenophora tritici-repentis and survey of physiologic variation in western . Canada. Can. J. Plant Pathol. , 20 : 396 – 400 .
- Lamari , L. , Strelkov , S.E. , Yahyaoui , A. , Orabi , J. and Smith , R.B. 2003 . The identification of two new races of Pyrenophora tritici-repentis from the host center of diversity confirms a one to-one relationship in tan spot of wheat . Phytopathology , 93 : 391 – 396 .
- Lamari , L. , Mccallum , B.D. and De Pauw , R.M. 2005a . The forensic pathology of Canadian hard red spring wheats: the case of tan spot . Phytopathology , 95 : 144 – 152 .
- Lamari , L. , Strelkov , S.E. , Yahyaoui , A. , Amedov , M. , Saidov , M. , Djunusova , M. and Koichibayev , M. 2005b . Virulence of Pyrenophora tritici-repentis in the countries of the Silk Road . Can. J. Plant. Pathol. , 27 : 383 – 388 .
- Lamari , L. and Strelkov , S.E. 2010 . The wheat/Pyrenophora tritici-repentis interaction: progress towards an understanding of tan spot disease . Can. J. Plant Pathol. , 32 : 4 – 10 .
- Morrall , R.A.A. and Howard , R.J. 1975 . The epidemiology of leaf spot disease in a native prairie. II. Air borne spore populations of Pyrenophora tritici-repentis . Can. J. Bot. , 53 : 2345 – 2353 .
- Nei , M. 1973 . Analysis of gene diversity in subdivided populations . Proc. Natl. Acad. Sci. USA , 70 : 3321 – 3323 .
- Page , R.D.M. 1996 . TreeView: an application to display phylogenetic tree on personal computers . Comput. Appl. Biosci. , 12 : 357 – 358 .
- Peakall , R. and Smouse , P.E. 2005 . “ GenAlEx V6: Genetic Analysis in Excel ” . In Population genetic software for teaching and research , Canberra, , Australia : The Australian National University .
- Peever , T.L. , Salimath , S.S. , Su , G. , Kaiser , W.J. and Muehlbauer , F.J. 2004 . Historical and contemporary multilocus population structure of Ascochyta rabiei (teleomorph: Didymella rabiei) in the Pacific Northwest of the United States . Mol. Ecol. , 13 : 291 – 309 .
- Peakall , R. and Smouse , P.E. 2005 . “ GenAlEx V6: Genetic Analysis in Excel ” . In Population genetic software for teaching and research , Canberra, , Australia : The Australian National University .
- Rees , R.G. and Platz , G.J. 1983 . Effects of yellow spot on wheat: comparison of epidemics at different stages of crop development . Aust. J. Agric. Res. , 34 : 39 – 46 .
- Rhaiem , A. , Cherif , M. , Peever , T.L. and Dyer , P.S. 2008 . Population structure and mating system of Ascochyta rabiei in Tunisia: evidence for the recent introduction of mating type 2 . Plant Pathol. , 57 : 540 – 551 .
- Rozen , S. and Skaletsky , H. 2000 . Primer3 on the for general users and for biologist programmers . Meth. Mol. Biol. , 132 : 365 – 386 .
- Shoemaker , R.A. 1962 . Drechslera Ito . Can. J. Bot. , 40 : 809 – 836 .
- Sneath , P.H.A. and Sokal , R.R. 1973 . Numerical taxonomy: the principles and practice of numerical classification , San Francisco, CA : Freeman Press .
- Strelkov , S.E. and Lamari , L. 2003 . Host-parasite interactions in tan spot Pyrenophora tritici-repentis of wheat . Can. J. Plant Pathol. , 25 : 339 – 349 .
- Strelkov , S.E. , Lamari , L. and Ballance , G.M. 1999 . Characterization of a host-specific protein toxin (Ptr ToxB) from Pyrenophora tritici-repentis . Mol. Plant–Microbe Interact. , 12 : 728 – 732 .
- Strelkov , S.E. , Lamari , L. , Sayoud , R. and Smith , R.B. 2002 . Comparative virulence of chlorosis-inducing races of Pyrenophora tritici-repentis . Can. J. Plant Pathol. , 24 : 29 – 35 .
- Tekauz , A. 1976 . Distribution, severity and relative importance of leaf spot disease of wheat in western Canada in 1974 . Can. Plant Dis. Surv. , 56 : 36 – 40 .
- Temnykh , S. , Declerck , G. , Lukashova , A. , Lipovich , L. , Cartinhour , S. and McCouch , S. 2001 . Computational and experimental analysis of microsatellites in rice (Oryza sativa L.): frequency, length variation, transposon association and genetic marker potential . Genome Res. , 11 : 1441 – 1452 .
- Vavilov , N.I. 1951 . The origin, variation, immunity and breeding of cultivated plants: Selected writings , Waltham, MA : Chronica Botanica Co .