Abstract
A phytotoxin isolated from Calonectria ilicicola, the causal agent of soybean red crown rot, was identified as PF1070A, a cyclic peptide consisting of four amino acids. This compound is known as an antitumour antibiotic and to induce synthesis of metallothionein in mouse Ltk- cells. We tested PF1070A against three soybean cultivars that differ in susceptibility to C. ilicicola; however, there was no significant difference among their responses to the toxin. We determined the amount of PF1070A produced by 17 isolates of C. ilicicola, and found that there was a strong positive correlation between virulence and PF1070A production. Together, these results suggest that the phytotoxin PF1070A has a role in the virulence of C. ilicicola.
Résumé
Une phytotoxine isolée chez Calonectria ilicicola, agent causal de la pourriture du collet chez le soja, a été identifiée en tant que PF1070A, un peptide cyclique constitué de quatre acides aminés. Ce composé est connu comme antibiotique antitumoral et il induit la synthèse de la métallothionéine dans les cellules Ltk- chez la souris. Nous avons testé PF1070A sur trois cultivars de soja de sensibilité différente à C. ilicicola. Toutefois, il n'y a pas eu de différence significative entre les réactions à la toxine. Nous avons déterminé la quantité de PF1070A produite par 17 isolats de C. ilicicola et nous avons trouvé qu'il y avait une forte corrélation positive entre la virulence et la production de PF1070A. L'ensemble de ces résultats suggère que la phytotoxine PF1070A joue un rôle dans la virulence de C. ilicicola.
Introduction
Red crown rot of soybean (Glycine max L. Merrill) is caused by a soilborne fungal pathogen, Calonectria ilicicola Boedijn & Reitsma (anamorph: Cylindrocladium parasiticum Crous, M.J. Wingf. and Alfenas). The first report of this disease was in Chiba, Japan, in 1968 (Nishi, Citation1989). The disease was subsequently reported in the USA in 1972 (Rowe et al., Citation1973), South Korea in 1976 (Sung, Citation1980), Cameroon in 1978 (Bernaux, Citation1979), and China in 1992 (Ma et al., Citation2004). Soybean plants are killed in severe cases of the disease. Plants that survive the disease show earlier maturity, and consequently, decreased pod number and poor growth of seed. Roy et al. (Citation1989) reported the occurrence of this disease in Mississippi, and estimated yield losses at 25–30% in infested fields.
In spite of the severity of this disease, its pathogenic mechanism has not been studied in detail. The symptoms include root disruption and leaf chlorosis. The pathogen is easily isolated from diseased roots, but not from symptomatic leaf tissues. This suggests that a toxic metabolite of the fungus may function in disease development. Application of culture filtrates of C. ilicicola resulted in leaf wilt (Kim et al., Citation2001), but no active compounds have been identified from this pathogen to date. Several researchers have speculated that toxic metabolites produced by C. ilicicola are involved in pathogenesis of black root rot of peanut, because necrosis was visible on hypocotyls (Johnston & Beute, Citation1975) and tap roots (Harris & Beute, Citation1982) prior to fungal invasion. The symptoms of sudden death syndrome (SDS) of soybean caused by Fusarium solani (Mart.) Sacc. f. sp. glycines are very similar to those of red crown rot. In the case of SDS, a single protein purified from culture filtrates caused browning of soybean calli and necrosis on detached soybean cotyledons and leaves (Jin et al., Citation1996).
There are many other plant diseases in which phytotoxins have roles in pathogenesis (Meehan & Murphy, Citation1947; Ueno et al., Citation1975a ; Kawai et al., Citation1983; Lawrence et al., Citation1990). In this study, therefore, we aimed to isolate and identify a phytotoxin from C. ilicicola and analyze its role in the pathogenic mechanism of red crown rot.
Materials and methods
Fungal isolates
The C. ilicicola isolate UH2-1 was used for isolation and identification of the phytotoxin, and 17 isolates including UH2-1 were used for analysis of the role of the phytotoxin in red crown rot pathogenesis (). All isolates were originally obtained from soybean roots, and were preserved by the method described previously (Ochi & Nakagawa, Citation2010).
Table 1. Calonectria ilicicola isolates analyzed in this study and their pathogenicity and phytotoxin production
Isolation of the phytotoxic metabolite and bioassay
The UH2-1 strain was cultivated on potato-dextrose agar (PDA) (Difco, Detroit, MI, USA) at 25 °C for five days. For liquid cultures, a mycelial plug was inoculated into 1 L potato-dextrose broth (PDB) (Difco, Detroit, MI, USA) in a 3 L flask and incubated at 25 °C for two weeks in the dark without shaking. Twelve flasks were prepared and so the culture material became 12 L in total. The cultured material was mixed with 2 L acetone for each flask and incubated at 25 °C for one day. The mixture was filtered to clear the mycelial culture and was evaporated to remove acetone by using a rotary evaporator. The aqueous residue was extracted three times with 333 mL ethyl acetate for each flask. The ethyl acetate extract was purified as shown in . Final preparative high-performance liquid chromatography (HPLC) conditions are as follows: column ODS-UG-5 (250 × 20 mm) (Nomura Chemical, Aichi, Japan); eluent, 70% aqueous CH3CN; flow rate, 10 mL min−1; detection, UV at 200 nm.
Fig 1. Purification procedure for phytotoxin from Calonectria ilicicola and ODS-HPLC chromatogram. aNecessary quantity of solvent was divided into seven fractions equally.
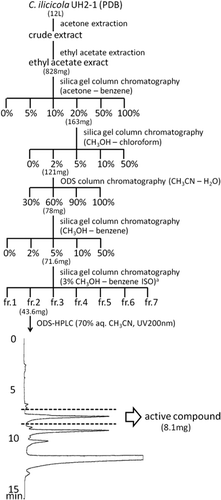
After fractionation, the activity of the extract was examined using alfalfa as the test plant. We selected alfalfa because it is in the same family as soybean (Leguminosae), it is susceptible to infection by C. ilicicola, and it is small enough for simple tests. Seeds were surface-sterilized in a solution of 1% NaOCl for 1 min, then rinsed twice in sterile distilled water before germination on sterile, moist filter paper for one day. A portion of the fraction equivalent to 100 mL cultured material dissolved in CH3OH and CH3OH as control were placed in glass culture tubes (3-cm diameter), then the CH3OH was removed by air-drying and the residue was resuspended in 1 mL sterile distilled water. Five uniform seedlings were placed in each glass culture tube containing the fractionated extract, and were grown at 25 °C for five days under room light conditions. Shoot and root lengths were measured, maximum and minimum values in each tube were excluded and average values were calculated. Each experiment comprised three replicates.
Analysis of isolated phytotoxin
The molecular weight of the phytotoxin was determined by liquid chromatography-time of flight-mass spectrometry (LC-TOF-MS), JMS-T100LC (JEOL, Tokyo, Japan); column Develosil ODS-UG-5 (150 × 2 mm); eluent 55% aqueous CH3CN; flow rate 0.2 mL min−1; column oven temperature 40 °C; ionization ESI-positive. The structure of the phytotoxin was analyzed by NMR in CDCl3 using an Avance 800 system (Bruker BioSpin, Karlsruhe, Germany) using residual CHCl3 (δH 7.24) and CDCl3 (δC 77.0) as internal standards.
Virulence test of the isolated phytotoxin
To confirm its role in virulence, the isolated compound was applied to roots and leaves of the soybean cultivar ‘Tachinagaha’. For root growth inhibition tests, soybean seeds were rinsed in 70% ethanol for 30 s and surface-sterilized with a solution of 1% NaOCl for 8 min. Seeds were then rinsed twice in sterile distilled water and germinated on sterile, moist filter paper for one day. Aliquots of the isolated compound (50, 100, 200 or 500 μg in CH3OH) and CH3OH as control were placed in glass culture tubes (3-cm diameter), CH3OH was removed by air drying, and then the compound was resuspended in 1 mL sterile distilled water. Three uniform seedlings were placed in each glass culture tube and were grown at 25 °C for four days under room light conditions. Fresh roots were weighed and the ratio of weight of treated fresh roots to control calculated. Each experiment comprised three replicates.
For the leaf chlorosis test, aliquots of the isolated compound (10, 40 or 80 μg dissolved in CH3OH) and CH3OH as control were placed in glass culture tubes (3-cm diameter). The CH3OH was removed by air-drying and the compound was resuspended in 1 mL sterile distilled water. A soybean leaf (first true leaf) was placed in the tube at 25 °C for one week under ambient room light conditions to allow the compound to absorb into the petiole. Each experiment comprised three replicates.
Reaction of various cultivars of soybean towards phytotoxin
The susceptibility to C. ilicicola differs among soybean cultivars (Nakagawa et al., Citation1990; Nakajima et al., Citation1994; Kim et al., Citation1998). Therefore, we determined whether there were differences among cultivar's responses to the phytotoxin. We selected the following cultivars for root growth inhibition assays as described above: ‘Fukuyutaka’ (low susceptibility), ‘Enrei’ (moderately susceptible) and ‘Harosoy’ (highly susceptible).
Correlation between pathogenicity and phytotoxin production in C. ilicicola isolates
We used 17 C. ilicicola isolates for pathogenicity tests. Each isolate was inoculated into wheat bran-vermiculite medium (wheat bran/vermiculite/water 1:1:3, w/w) and cultured for one month at 25 °C in the dark. The cultured material of each isolate was then added to autoclaved agricultural soil (Kureha, Tokyo, Japan) at a rate of 1% (w/w). Plastic pots (9-cm diameter) were filled with infested soil, and three soybean seeds of ‘Tachinagaha’ were sown in each pot. Uninoculated wheat bran-vermiculite medium was similarly added to soil as the control. This experiment was conducted in a 25 °C greenhouse under natural light conditions. Each treatment consisted of three replicates. After three weeks, the disease index was evaluated using the following scale: 0, healthy; 1, roots discoloured; 2, a few lateral roots lost; 3, many lateral roots lost; 4, damping off. We used the following formula to calculate disease severity: Disease severity = Σ (number of plants in each category × disease index) × 100/(total number of plants × 4).
For the phytotoxin production test, we used the same 17 isolates as those used in the pathogenicity test. Each isolate was incubated on PDA at 25 °C for five days. A mycelial plug of each isolate was inoculated into a 300 mL flask containing 100 mL PDB and incubated at 25 °C for one month in the dark without shaking. The purification process was effectively modified, so each culture was then extracted with 200 mL acetone at 15 °C for one day. The mixture was filtered to clear the mycelial culture and was evaporated to remove acetone by using a rotary evaporator. The aqueous residue was extracted three times with 33 mL ethyl acetate. The ethyl acetate extract was partially purified by silica gel column chromatography (acetone-benzene) and then C18 column chromatography (CH3CN-H2O). Both chromatography steps were carried out using Sep-Pak Vac (500 mg) columns (Waters, Milford, MA, USA). After that, the amount of phytotoxin produced by each isolate was quantified by ODS-HPLC analysis (column, Develosil ODS-UG-5 150 × 2.0 mm; eluent, 55% aqueous CH3CN; flow rate, 0.2 mL min−1; detection, UV at 200 nm). The amount of phytotoxin in each sample was quantified by comparing the compound's peak area to a PF1070A standard curve.
Results
Identification of phytotoxin produced by C. ilicicola
The procedure for purifying the phytotoxic compound is shown in . The ethyl acetate extract was fractionated by silica gel column chromatography. In the growth inhibition test, the 20% acetone-benzene fraction showed the strongest activity against alfalfa seedlings, and so this fraction was rechromatographed in three different solvent systems (). The active fraction was then purified by ODS-HPLC yielding a single bioactive peak that eluted at 8.1 min (). The yield of the phytotoxin was 8.1 mg from 12 L culture liquid.
LC-TOF-MS of the phytotoxic compound showed a cationized molecule at m/z 569 (M + H)+. The molecular formula was determined to be C31H44N4O6 based on results of high-resolution LC-TOF-MS (m/z 569.31031, calculated 569.33391).
The 13C NMR spectrum of the phytotoxic compound showed five carbonyl signals, four of which were at ester or amide carbonyl region (170.23–174.20 ppm), and one of which was at ketone region (207.57 ppm) (). The 1H NMR spectrum showed four α-proton signals at 4.50–4.86 ppm (). These facts suggested the phytotoxin to be a peptide composed of four amino acids. Structure of the side chains of the amino acids were elucidated by tracing correlations on DQF-COSY (Double Quantum Filtered-Correlated Spectroscopy), TOCSY (Total Correlation Spectroscopy), HSQC (Heteronuclear Single Quantum Correlation) and HMBC (Heteronuclear Multiple Bond Correlation), and the component amino acids were determined to be phenylalanine (Phe), isoleucine (Ile), piperidine-2-carboxylic acid (Pip), and 2-amino-9,10-epoxi-8-oxodecanoic acid (Aoe). Sequence of the amino acids was confirmed by HMBC correlations between α-protons and carbonyls, and the phytotoxic compound was identified as PF1070A (cyclo (Ile-Pip-Aoe-Phe)) (). The 1H and 13C NMR spectral data of the phytotoxic compound coincided with reported data for PF1070A (). PF1070A was previously isolated from Humicola sp. as an antitumour antibiotic (Yoshida et al., Citation1992) and then it was shown to be a potent inducer of the metallothionein synthesis in mouse (Asahi et al., Citation1999).
Table 2. 1H and 13C NMR data for PF1070A in CDCl3
In soybean ‘Tachinagaha’, PF1070A inhibited root growth in a concentration-dependent manner (), and the symptom was reproduced on leaves by a leaf chlorosis test (). This confirmed that PF1070A was the phytotoxin. This is the first report on phytotoxicity of PF1070A.
Fig 4. Responses of soybean cultivar ‘Tachinagaha’ and three soybean cultivars to PF1070A. PF1070A (50, 100, 200 or 500 μg) was suspended in 1 mL sterile distilled water in glass culture tubes (3-cm diameter). Three soybean seedlings were placed in each glass and were grown at 25 °C under room light conditions. Fresh roots were weighed after four days of treatment. Each experiment comprised three replicates.
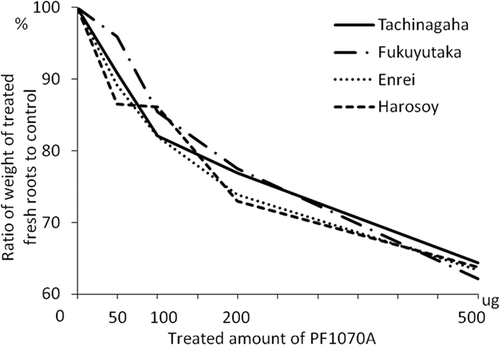
Fig 5. Foliar symptoms after PF1070A treatment. a, Control. b, 10 μg PF1070A treatment. c, 40 μg PF1070A treatment. d, 80 μg PF1070A treatment. PF1070A was suspended in 1 mL sterile distilled water in glass culture tubes (3-cm diameter). A soybean leaf (first true leaf) was place in the tube at 25 °C for one week under ambient room light to allow the compound to absorb into the petiole. Each experiment comprised three replicates.
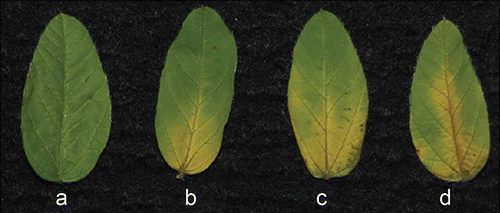
Responses of soybean cultivars to PF1070A
Fresh roots were weighed after four days of treatment (). Root growth was inhibited by PF1070A in a concentration-dependent manner on ‘Tachinagaha’. The IC50 values of PF1070A in the three soybean cultivars were not significantly different (721 μg mL−1 in ‘Fukuyutaka’, 741 μg mL−1 in ‘Enrei’, and 754 μg mL−1 in ‘Harosoy’), in spite of the fact that there are differences among three cultivars in susceptibility.
Correlation between virulence and phytotoxin production in C. ilicicola isolates
The disease severity of 17 isolates ranged from 2.8 to 89.3, while PF1070A production in cultures ranged from 0.01 to 4.46 mg 100 mL−1 (). The disease severity was plotted against PF1070A production (); the Spearman's rank correlation coefficient for the two values was 0.69 (P < 0.01). Thus, there is a significant positive correlation between pathogenicity and PF1070A production. In addition, simple linear regression analysis showed that the regression line is significant and PF1070A production can be used to predict an isolate's virulence.
Discussion
In this study, we identified a phytotoxin from C. ilicicola as PF1070A, which is a cyclic peptide consisting of four amino acids. Yoshida et al. (Citation1993) reported that PF1070A has antitumour antibiotic properties, and later, it was reported that this compound has an important role in the induction of metallothionein synthesis in mouse (Asahi et al., Citation1999). This is the first report on its phytotoxicity to soybean.
Kim et al. (Citation2001) reported that the cultivar differences of foliar sensitivity to red crown rot in bioassays were not correlated well with those of susceptibility of plants in the greenhouse. As the most important phenomenon in red crown rot was disruption of roots, we evaluated the sensitivity to culture filtrates using a root growth inhibition test and compared it with the susceptibility to C. ilicicola. PF1070A showed a similar growth inhibition among three cultivars even though their susceptibility to C. ilicicola was different. We presume that this result indicates the difference in susceptibility is not from the biological activity of the phytotoxin.
Kim et al. (Citation2001) also reported that virulence of C. ilicicola isolates was not correlated with the toxicity of culture filtrates of each isolate in a trifoliate assay. They concluded that toxic metabolites produced by C. ilicicola were secondary determinants of virulence, and were not critical for disease development. However, in the present study, virulence of C. ilicicola isolates was positively correlated with PF1070A production, suggesting that PF1070A is associated with some aspect of virulence. We observed that root disease severity is not necessarily associated with extent of foliar symptoms in field tests, and we presume that some factor other than the amount of toxin probably affects symptom development.
Several four amino acid cyclic peptides have been reported to have phytotoxic effects, including the HC-toxin (cyclo(L-Ala-D-Ala-L-Aoe-D-Pro)) isolated from Cochliobolus carbonum Nelson (the causal agent of northern leaf spot of maize) (Kawai et al., Citation1983) and Cyl-1 (cyclo(L-Aoe-D-O-MeTyr-L-Ile-L-Pro)) and Cyl-2 (cyclo(L-Aoe-D-O-MeTyr-L-Ile-L-Pip)), isolated from C. kyotensis (Hirota et al., Citation1973). Like PF1070A, these three toxins contain Aoe. In addition, the AM-toxin has been isolated from Alternaria mali Roberts, the causal agent of Alternaria blotch of apple (Ueno et al., Citation1975a , Citation1975b ). The HC- and AM-toxins play key roles in the pathogenic mechanisms of their respective pathogens (Scheffer & Ullstrup, Citation1965; Ueno et al., Citation1975a ). HC-toxin acts as a histone deacetylase (HDAC) inhibitor, causing cytostatis and apoptosis, and is associated with pathogenesis in northern leaf spot of maize. Maize HDACs were inhibited by HC-toxin in vivo, as demonstrated by the accumulation of hyperacetylated forms of the core histones in both susceptible maize embryos and tissue cultures but not in resistant maize (Ransom & Walton, Citation1997). Yoshida and Sugita (Citation1992) reported that trapoxin A (cyclo(L-Phe-L-Phe-D-Pip-L-Aoe)), a compound closely related to HC-toxin and PF1070A, also has the same function as HC-toxin. On the other hand, reaction to C. carbonum and to HC-toxin is controlled in maize by the Hm locus (Meeley & Walton, Citation1991). This locus encodes HC-toxin reductase (Johal & Briggs, Citation1992), which detoxifies HC-toxin by reducing the 8-carbonyl group of Aoe (Meeley et al., Citation1992), so it is thought that Aoe is an active site for HDAC. As PF1070A is also a cyclic peptide consisting of four amino acids including Aoe, it is expected to show similar functions to that of HC-toxin. Further studies are required to confirm this.
In this study, we showed that the phytotoxin of C. ilicicola associated with disease severity of red crown rot was PF1070A. Rudolph (Citation1976) pointed out that a toxin would very seldom act alone but rather in combination with other products of the pathogen (metabolites and enzymes). In future research, we are going to study the relationship between susceptibility of soybean and phytotoxin by investigating the biochemical behaviour of soybean to the phytotoxin.
Acknowledgement
We thank Dr Ikuko Maeda of the National Food Research Institute for NMR analysis.
References
- Asahi , I. , Miura , N. , Yamabe , Y. , Toyoda , H. , Imura , N. , Koyama , M. and Naganuma , A. 1999 . PF1070A, a novel and potent inducer of the synthesis of metallothionein . Biochemistry , 38 : 10415 – 10423 .
- Bernaux , P. 1979 . Identification of some diseases of soybean in Cameroon. Agron . Trop. , 34 : 301 – 304 .
- Harris , N.E. and Beute , M.K. 1982 . Histological responses of peanut germplasm resistant and susceptible to Cylindrocladium crotalariae in relationship to inoculum density . Phytopathology , 72 : 1250 – 1256 .
- Hirota , A. , Suzuki , A. , Suzuki , H. and Tamura , S. 1973 . Isolation and biological activity of Cyl-2, a metabolite of Cylindrocladium scoparium. Agric. Biol . Chem. , 37 : 643 – 647 .
- Jin , H. , Hartman , G.L. , Nickell , C.D. and Widholm , J.M. 1996 . Characterization and purification of a phytotoxin produced by Fusarium solani, the causal agent of soybean sudden death syndrome . Phytopathology , 86 : 277 – 282 .
- Johal , G.S. and Briggs , S.P. 1992 . Reductase activity encoded by the HM1 disease resistance gene in maize . Science , 258 : 985 – 987 .
- Johnston , S.A. and Beute , M.K. 1975 . Histopathology of Cylindrocladium black rot of peanut . Phytopathology , 64 : 649 – 653 .
- Kawai , M. , Rich , D.H. and Walton , J.D. 1983 . The structure and conformation of HC-toxin . Biochem. Biophys. Res. Commun. , 111 : 398 – 403 .
- Kim , K.D. , Russin , J.S. and Snow , J.P. 1998 . Susceptibility to Calonectria ilicicola in soybean grown in greenhouse and field . Korean J. Crop Sci. , 43 : 239 – 244 .
- Kim , K.D. , Russin , J.S. , Snow , J.P. and Damann , K.E. Jr. 2001 . Toxic culture filtrates produced by Calonectria ilicicola, causal agent of red crown rot of soybean . Phytoparasitica , 29 : 115 – 123 .
- Lawrence , C.H. , Clark , M.C. and King , R.R. 1990 . Induction of common scab symptoms in aseptically cultured potato tubers by vivotoxin, Thaxtomin . Phytopathology , 80 : 606 – 608 .
- Ma , Z.H. , Zhang , Z.D. , Wang , Y.X. and Yang , X.B. 2004 . Cylindrocladium crotalariae causing red crown rot of soybean in China . Plant Pathol. , 53 : 537
- Meehan , F. and Murphy , H.C. 1947 . Differential phytotoxicity of metabolic by-products of Helminthosporium victoriae . Science , 106 : 270 – 271 .
- Meeley , R.B. and Walton , J.D. 1991 . Enzymatic detoxification of HC-toxin, the host-selective cyclic peptide from Cochliobolus carbonum . Plant Physiol. , 97 : 1080 – 1086 .
- Meeley , R.B. , Johal , G.S. , Briggs , S.P. and Walton , J.D. 1992 . A biochemical phenotype for a disease resistance gene of maize . Plant Cell , 4 : 71 – 77 .
- Nakagawa , A. , Shimada , S. and Yamaguchi , T. 1990 . Studies on soybean root necrosis caused by Calonectria crotalariae 3. Varietal differences of growth and yield component of soybean under pathogen inoculated condition (in Japanese with English summary) . Ann. Rept. Kansai Plant Prot. , 32 : 1 – 8 .
- Nakajima , T. , Sakai , S. , Gomi , T. and Kikuchi , A. 1994 . Development of methods for assessing resistance to black root rot caused by Calonectria crotalariae in soybean and screening for resistant germplasm (in Japanese with English summary) . Bull. Tohoku Nat. Agric. Exp. Stn. , 88 : 39 – 56 .
- Nishi , K. 1989 . Present situation on Calonectria root rot of soybean (in Japanese) . J. Agric. Sci. (Japan) , 44 : 70 – 75 .
- Ochi , S. and Nakagawa , A. 2010 . A simple method for long-term cryopreservation of Calonectria ilicicola on barley grains . J. Gen. Plant Pathol. , 76 : 112 – 115 .
- Ransom , R.F. and Walton , J.D. 1997 . Histone hyperacetylation in maize in response to treatment with HC-Toxin or infection by the filamentous fungus Cochliobolus carbonum . Plant Physiol. , 115 : 1021 – 1027 .
- Rowe , R.C. , Beute , M.K. and Wells , J.C. 1973 . Cylindrocladium black root rot of peanuts in North Carolina – 1972 . Plant Dis. Rep. , 57 : 387 – 389 .
- Roy , K.W. , McLean , K.S. , Lawrence , G.W. , Patel , M.V. and Moore , W.F. 1989 . First report of red crown rot of soybeans in Mississippi . Plant Dis. , 73 : 273
- Rudolph , K. 1976 . “ Non-specific toxins ” . In Physiological plant pathology , Edited by: Heitefuss , R. and Williams , P.H. 270 – 315 . Berlin : Springer Verlag .
- Scheffer , R.P. and Ullstrup , A.J. 1965 . A host-specific toxic metabolite from Helminthosporium carbonum . Phytopathology , 55 : 1037 – 1038 .
- Sung , J.M. 1980 . An investigation of undescribed black root rot disease of soybean caused by Cylindrocladium (Calonectria) crotalariae in Korea . Kor. J. Mycol. , 8 : 53 – 57 .
- Ueno , T. , Hayashi , Y. , Nakashima , T. , Fukami , H. , Nishimura , S. , Kohmoto , K. and Sekiguchi , A. 1975a . Isolation of AM-toxin I, new phytotoxic metabolite from . Alternaria mali. Phytopathology , 65 : 82 – 83 .
- Ueno , T. , Nakashima , T. , Hayashi , Y. and Fukami , H. 1975b . Structures of AM-toxin I and II, host specific phytotoxic metabolites produced by Alternaria mali . Agric. Biol. Chem. , 39 : 1115 – 1122 .
- Yoshida , H. and Sugita , K. 1992 . A novel tetracyclic peptide, trapoxin, induces phenotypic change from transformed to normal in sis-oncogene-transformed NIH3T3 cells. Japn. J . Cancer Res. , 83 : 324 – 328 .
- Yoshida , S. , Yoshioka , M. , Yaguchi , T. , Nagasawa , M. and Koyama , M. 1993 . PF1070A and B, new antitumour antibiotics produced by Humicola sp. Sci. Rep . Meiji Seika Kaisha , 32 : 58 – 63 .