Abstract
Four hundred and seventeen single-uredinial isolates of Puccinia triticina collected from wheat in seven regions of Russia in 2007 were tested for virulence with 24 near-isogenic wheat differential lines and molecular variation with six RAPD and one UP-PCR DNA markers. Seventy-nine virulence phenotypes and 71 molecular genotypes were identified. The P. triticina isolates varied for virulence on resistance genes Lr1, Lr2a, Lr2b, Lr2с, Lr3a, Lr3bg, Lr3ka, Lr14a, Lr14b, Lr15, Lr16, Lr17, Lr18, Lr19, Lr20, Lr21, Lr24, Lr26, Lr28 and LrB. All isolates were virulent on Lr10, Lr11 and Lr30, and avirulent on Lr9. THTTTJ was the predominant phenotype in all regions with frequency ranging from 25 to 63%. Diversity analysis of the regional collections demonstrated inconsistency of results obtained with virulence and molecular markers. According to the virulence data, the North Caucasian and Central collections of P. triticina were the most distant from all the other regional populations. The most similar were collections from West Siberian and Ural regions, which may be a result of growing genetically similar wheat cultivars. According to the molecular marker data, the Central, Central Black Earth, and Ural populations clustered distinctly from the North Western, North Caucasian, and West Siberian collections.
Résumé
En 2007, 417 isolats mono-urédiniaux de Puccinia triticina, collectés sur du blé provenant de 7 régions de Russie, ont été testés, avec 6 marqueurs RAPD et 1 UP-PCR, pour leur virulence à l'égard de 24 lignées différentielles quasi-isogéniques et leur variation moléculaire. Soixante-dix-neuf phénotypes de virulence et 71 génotypes moléculaires ont été identifiés. Sur le plan de la virulence, les isolats de P. triticina variaient à l'égard des gènes de résistance Lr1, Lr2a, Lr2b, Lr2c, Lr3a, Lr3bg, Lr3ka, Lr14a, Lr14b, Lr15, Lr16, Lr17, Lr18, Lr19, Lr20, Lr21, Lr24, Lr26, Lr28 et LrB. Tous les isolats étaient virulents à l'égard de Lr10, Lr11 et Lr30, et non virulents à l'égard de Lr9. Le phénotype THTTTJ était le plus courant dans toutes les régions avec une fréquence variant de 25 à 63 %. L'analyse de la diversité des collections régionales a démontré la non-cohérence des résultats obtenus avec les marqueurs de virulence et les marqueurs moléculaires. Selon les données de virulence, les collections de P. triticina provenant du nord du Caucase et du Centre étaient les plus éloignées de toutes les autres populations régionales. Les plus semblables étaient les collections de l'ouest de la Sibérie et de l'Oural, ce qui peut découler du fait que, dans ces régions, on utilise des cultivars de blé similaires. Selon les données obtenues avec les marqueurs moléculaires, les populations du Centre, du Centre-Terres noires et de l'Oural forment un groupe distinct de celles du Nord-Ouest, du nord du Caucase et de l'ouest de la Sibérie.
Introduction
Wheat leaf rust, caused by Puccinia triticina Eriks., is an important disease which affects production in many agroclimatic regions of the Russian Federation. The major grain production in Russia (about 75% of the grain-growing area) is concentrated in the North Caucasian, Central Black Earth, Central, Volga, Ural and Volga-Vyatka regions (). During the last 20 years, severe epidemics in the North Caucasian region were reported from 2 to 3 times within a decade and resulted in yield losses of 30–35%. In the Central and Volga regions, epidemics were registered from 5 to 7 times in a decade with estimated losses of 20–30%. In the Central Black Earth region, epiphytotics have occurred in up to 4 years out of 10, causing crop losses of 15–20%. In the Volgo-Vyatka and Ural regions, severe disease development was observed every two years, and crop losses were estimated at 15–20% (Sanin et al., Citation2004).
Fig. 1. Map of Russian agroecological regions. NW – Northwestern, NC – North Caucasian, CBE – Central Black Earth, C – Central, LV - Low Volga, U – Ural, WS – West Siberian, MV – Middle Volga, VV – Volgo-Vyatka.
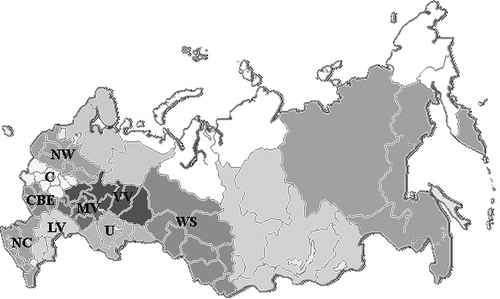
Annual virulence surveys of P. triticina in different regions of Russia and the former USSR were conducted by the All Russian Institute of Plant Protection (St. Petersburg) since 1980. Differentiation among the Caucasian, European, and West Siberian populations was established on the basis of virulence analysis on the local differential set of two ‘Thatcher’ lines (Lr1 and Lr2a) and seven cultivars. The West Asian population differs from the European one, while typical ‘European’ and ‘Asian’ phenotypes were observed in the Volga region (Mikhailova, Citation1996; Mikhailova & Gultyaeva, Citation1997). The ‘Thatcher’ near-isogenic lines as differentials and the North-American nomenclature for encoding virulence phenotypes (Long & Kolmer, Citation1989) have been used since 2002 to characterize P. triticina races in Russia. Results obtained with the 16 ‘Thatcher’ differentials supported the findings of Mikhailova (Citation1996) concerning the structure of Russian leaf rust population (Gultyaeva, Citation2007).
Variation in virulence of P. triticina might depend on selection pressure of resistance genes deployed in wheat cultivars. In contrast, molecular markers are generally assumed to be neutral to host selection. Therefore, they may provide more accurate tools for analysing genetic variation of plant pathogens with gene-for-gene relationships within a host–pathogen system.
Analyses of genetic variability using molecular markers began in the mid 1990s and have provided new insight into the population biology of P. triticina. The first studies on wheat leaf rust used random amplified polymorphic DNA (RAPD) markers (Kolmer et al., Citation1995) and amplified fragment length polymorphism (AFLP) markers (Kolmer, Citation2001), which revealed distinct groups of P. triticina genotypes in Canada. Development of simple sequence repeat (SSR) DNA markers for P. triticina has greatly facilitated and improved analysis of the population structure of the pathogen (Duan et al., Citation2003; Szabo & Kolmer, Citation2007). The neutral molecular markers (RAPD, AFLP, SSR) provide stable instruments for differentiation among groups of isolates of P. triticina (Ordoñez & Kolmer, Citation2009). Virulence and molecular markers were utilized to study wheat leaf rust in Western Europe (Park et al., Citation2000), North America and South America (Ordoñez et al., Citation2010), Central Asia and Caucasus (Kolmer & Ordoñez, Citation2007), Ethiopia (Mebrate et al., Citation2006) and Italy (Mantovani et al., Citation2010). Although wheat leaf rust has been characterized with molecular markers in many regions all over the world, the current study is the first analysis of P. triticina in Russia using molecular markers (RAPDs).
The major objective of this study was to examine virulence and molecular variability in isolate collections of P. triticina from the main wheat growing areas of Russia. Virulence and molecular diversity within and among regional populations of P. triticina in Russia were analysed on the basis of the leaf rust survey of 2007.
Materials and methods
P. triticina isolates
The annual 2007 survey of wheat leaf rust was conducted in the period of extensive spread of the fungus in the summer. Samples were taken from widely cultivated commercial cultivars on regional plant protection stations located in different agroclimatic zones () and from susceptible cultivars grown in plant breeding nurseries. All samples consisted of several leaves with uredia. The leaves were air-dried and stored at 4 °C until multiplication and analysis. Multiplication of single urediniospore isolates for virulence tests and DNA extraction was performed by the method of detached leaf segments preserved in water–benzimidazol solution (40 mg L−1) (Mikhailova & Gultyaeva, Citation1997; Lind & Gultyaeva, Citation2007). Individual pustules that developed on the leaf segments were transferred by a sterile needle onto new leaf segments of the susceptible cultivar ‘Leningradka’. They are regarded to be single uredia progenies. The leaf segments were incubated at 100% relative humidity and 17–22 °C in darkness for 18 h, followed by 20 °C at 18 h of light. Using the same procedure, progenies of pustules and single spores were multiplied on leaf segments and subsequently on isolated seedling plants to get necessary amount of spores for inoculation of wheat tester lines and DNA extraction for virulence and molecular marker analyses, respectively.
Determination of virulence phenotypes
Infection types were assessed after 7 days of incubation using a 0–4 scale (McIntosh et al., Citation1995). Isolates with infection types 0–2 were assumed to be avirulent, whereas isolates with infection types 3–4 were considered to be virulent.
Virulence of leaf rust isolates was determined on 24 ‘Thatcher’ near-isogenic lines, which were divided into six ordered subsets of four differentials. The subsets consisted of lines with resistance genes Lr1, Lr2a, Lr2c and Lr3a; Lr9, Lr16, Lr24 and Lr26; Lr3ka, Lr11, Lr17 and Lr30; LrB, Lr10, Lr14a and Lr18; Lr2b, Lr3bg, Lr14b and Lr15; and Lr19, Lr20, Lr21 and Lr28. ‘Thatcher’ was included as a susceptible control. Each isolate was given a six-letter race designation, adapted from the nomenclature developed by Long & Kolmer (Citation1989). Each letter corresponds to a specific combination of low or high reaction on the four differentials from the corresponding set.
Determination of molecular genotypes
DNA was extracted according to Justesen et al. (Citation2002) from 10 to 20 mg of fresh urediniospores of each isolate. Spores were ground by shaking with 20 mg of glass beads (G9268, Sigma) in a FastPrep24 shaker (MP Biomedicals, USA) for 50 s. PCR reactions were performed in a C1000™ Thermal Cycler (BioRad) programmed at 94 °С for 3 min; followed by 40 cycles of 30 s at 92 °С, 1 min at 36 °С and 1 min at 72 °С. This was followed by 10 min at 72 °С for RAPD and 94 °С for 3 min; followed by 30 cycles of 35 s at 92 °С, 70 s at 53 °С, and 30 s at 72 °С, with a final 10 min at 72 °С for UP-PCR. Each reaction comprised 2 μL 10× Tag polymerase reaction buffer (Mg free), 1.2 μL of 25 mM MgCl2, for RAPD or 1.6 μL for UP-PCR, 1.6 μL of a 2.5 mM stock of each of dATP, dCTP, dGTP and dTTP, 0.2 μL of Taq polymerase (Dialat; 5 units μL−1), 2.0 μL of template DNA, 1 μL of primer (10 pМ for RAPD and 20 pМ for UP-PCR), and 12 μL of sterile double-distilled water for RAPD or 11.6 for UP-PCR. Amplification products were separated by electrophoresis in 1.5% agarose gels prepared in 1× TBE buffer and containing 0.5 μg mL−1 of ethidium bromide, for 2 to 3 h at 70V.
Twenty RAPD primers (402, 450, 489, 517, 521, 531, 538, 556, 180.01, 270.01, 270.09, 280.02, 270.09, 280.02, 360.04, 370.02, 370.09, 480.04, OPQ9 and OPR) previously used by Kolmer et al. (Citation1995) and Park et al. (Citation2000) were also utilized in the current study. Arbitrary decamer primers were obtained from the Eurogen company (Moscow, http://www.evrogen.ru). Selection of polymorphic primers was performed with 16 and 19 isolates from the North Western and Low Volga regions, respectively. In addition, five universal primers (L45, 3–2, 15–19, AS15inv and AS4) related to the UP-PCR technique (Bulat et al., Citation1998) were also included in molecular analysis. This technique uses longer primers, relatively higher annealing temperatures, and faster ramping in order to produce more reproducible band patterns. Only one UP-PCR primer AS4 (TGTGGGCGCTCGACAC) and six RAPD primers (450 (CGGAGAGCCC), 490 (AGTCGACCTT), 538 (TGACCTCTCC), 280.02 (CGACGCGTGC), 270.09 (GCTCTCACCG) and 480.04 (CGCCACGAGC)) were chosen due to their polymorphism and ability to produce consistent banding patterns with major band differences between the isolates.
Data analysis
Descriptive analysis of populations with virulence and molecular data included calculation of the following parameters: virulence frequency on each differential line, phenotype/genotype frequencies, average virulence complexity (number of virulent reactions per isolate), simple richness (SR, number of phenotypes/genotypes per isolate), and evenness (E, Sheldon index). Pathogenicity data for each isolate were converted to ‘1’ (virulent) or ‘0’ (avirulent), and major reproducible RAPD and UP-PCR bands were scored as ‘1’ (present) or ‘0’ (absent). Dissimilarity between virulence patterns of isolates was measured with the simple mismatch coefficient (m), while for the molecular data pairwise comparison of isolates was made using the simple mismatch (m) and Jaccard (j) dissimilarities according to Kosman & Leonard (Citation2005).
Diversity within and between regional collections of isolates was assessed with three types of indices: genotypic (Shannon normalized diversity and Rogers' distance, based on phenotype/genotype frequencies), gene (Nei's diversity and distance, based on virulence/allele frequencies), and genetic (Kosman's diversity [KWm and KWj ] and distance [KBm and KBj ] with regard to the simple mismatch (m) and Jaccard (j) dissimilarity, which take into account both the phenotype/genotype structure of populations and measure of resemblance between different phenotypes/genotypes). Detailed description of the mentioned diversity and distance measures can be found in Kosman & Leonard (Citation2007). All diversity and distance parameters and their statistics based on bootstrapping across isolates (200 random samples of isolates drawn with replacement from the original collections) were calculated with the VAT software (Kosman et al., Citation2008).
The Mantel test (Mantel, Citation1967) was employed to measure the degree of relationship (correlation) between any two relevant distance matrices. The genotypic, gene and genetic distances between the wheat leaf rust populations for each type of data, as well as genetic distances (KBm ) obtained with the virulence and molecular markers, were compared. The corresponding calculations were performed with the MXCOMP program of NTSYSpc package, version 2.1 (Exeter Software, Setauket, NY).
The UPGMA dendrograms for the wheat leaf rust populations with regard to the Rogers, Nei, and Kosman distances between them were derived using the SAHN program of NTSYSpc package, version 2.1 (Exeter Software, Setauket, NY). For each dendrogram, the cophenetic correlation was calculated (COPH and MXCOMP programs of NTSYSpc) to test goodness of fit for clustering with dendrograms (assessment of matching the dendrogram structure with the original distance matrix upon which the clustering was based).
Results
Populations of wheat leaf rust from seven agroecological regions of Russia () were analysed for virulence and molecular variability. In total, 417 single-uredinial isolates were tested. The number of isolates in the regional collections varied from 19 in the Low Volga area to 138 in the Northwestern region (). Further reported differences among diversities within or distances between populations were statistically significant (the bootstrap-based standard errors of all diversity and distance estimates did not exceed 0.001).
Table 1. Descriptive parameters of Russian regional populations of P. triticina in 2007 obtained with virulence phenotyping
Virulence study
There were 79 virulence phenotypes of P. triticina distinguished on the set of 24 near-isogenic wheat differential lines. The number of different virulence phenotypes in the regional populations varied from four in the Volga collection to 35 in the Northwestern region (). Fourteen phenotypes were detected in two or more regions (), while only one (THTTTJ) appeared in all seven regions. THTTTJ was predominant everywhere except the Central Black Earth region, with frequency ranging from 25% to 63%. The second most common phenotype (TGTTTJ) was found in six regions and was less frequent (2–35%) than THTTTJ, but was predominant in the Central Black Earth region (). The average virulence complexity of isolates was high in all populations (). Phenotype richness of the North Caucasian population was the highest (SR = 0.349), and the corresponding phenotypes were the most evenly distributed (E = 0.803). The minimum richness and evenness were established in the Low Volga (SR = 0.211) and Central (E = 0.635) regions, respectively.
Table 2. Frequency of common virulence phenotypes of P. triticina in seven agroecological regions of Russia (%)
The P. triticina isolates varied for virulence on genes Lr1, Lr2a, Lr2b, Lr2с, Lr3, Lr3bg, Lr3ka, Lr14a, Lr14b, Lr15, Lr16, Lr17, Lr18, Lr19, Lr20, Lr21, Lr24, Lr26, Lr28 and LrB (). All isolates were virulent on lines with Lr10, Lr11 and Lr30. No virulence on Lr9 was detected. A few isolates were virulent on Lr24, Lr19 and Lr28, while the virulence frequency was usually very high on all other differentials. Relatively strong regional variation in virulence frequency was observed on Lr1, Lr2a, Lr26, Lr15 and Lr20, whereas little variability in virulence was found on all other differentials. The most variable virulence reaction was detected on Lr26 with minimum (30%) in the Central Black Earth region and maximum (100%) in the Central region.
Table 3. Virulence frequency (%) within the Russian regional populations of P. triticina in 2007
Rank order of diversity estimates within the regional collections of isolates was generally inconsistent for the gene (Nei), genotypic (Shannon normalized) and genetic (Kosman) diversity measures (data not shown). Therefore, Kosman's diversity index (KWm ) was used for comparison of the regions (). Virulence diversity within the Northwestern and North Caucasian regions was significantly higher (KWm = 0.122 and 0.110, respectively) than that within the Central, Central Black Earth, West Siberian, Ural, and Low Volga regions (KWm = 0.069, 0.066, 0.083, 0.074 and 0.035, respectively).
Fig. 2. Virulence (a) and molecular (b) diversity within P. triticina populations from seven agroecological regions of Russia according to the Kosman's (KWm ) diversity index. Region abbreviations: NW – Northwestern, NC – North Caucasian, CBE – Central Black Earth, C – Central, LV - Low Volga, U – Ural, WS – West Siberian.
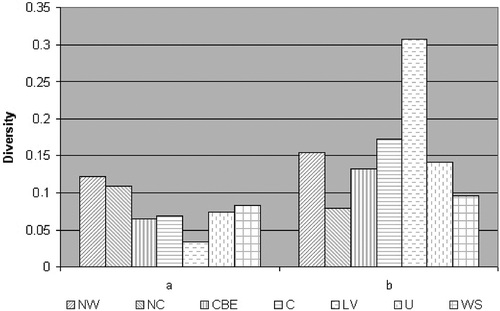
Comparison of different types of distances between the regional collections of leaf rust with the Mantel test (Mantel, Citation1967) revealed low levels of relationships between them (correlations of the genetic KBm distance with the genotypic and gene distances were 0.773 and 0.461, respectively). This may explain the structural differences in the UPGMA dendrograms built with the corresponding distance matrices (data not shown). Due to this discrepancy, analysis of the relationship between the populations was performed with KBm distance. Differences between all populations were small (KBm values varied from 0.027 to 0.073), but statistically significant.
Confidence in results obtained for the regional collection from Low Volga was low because a relatively small number of isolates obtained from a single variety was tested in this region. Therefore, the Low Volga collection was not included in analysis of relationship among the regional populations of wheat leaf rust by UPGMA dendrograms for virulence and molecular data. The UPGMA dendrogram with regard to the genetic distance KBm ( a) conveys nearly ‘actual’ relationships between the populations, because its degree of fit to the corresponding KBm distance matrix was very good (cophenetic correlation = 0.901). Thus, the North Caucasian and Central collections of P. triticina were the most distant from all the other regional populations according to the virulence data ( a). The most similar were collections from Western Siberia and Ural, which together with the Central Black Earth region formed a group of closely related populations. The Northwestern population was closer to this group than to the isolates from the Central and North Caucasian regions ( a).
Fig. 3. UPGMA dendrograms of the Russian regional populations of P. triticina based on virulence (a) and molecular (b) markers data with regard to the Kosman's (KBm ) genetic distance. Region abbreviations: NW – Northwestern, NC – North Caucasian, CBE – Central Black Earth, C – Central, U – Ural, WS – West Siberian.
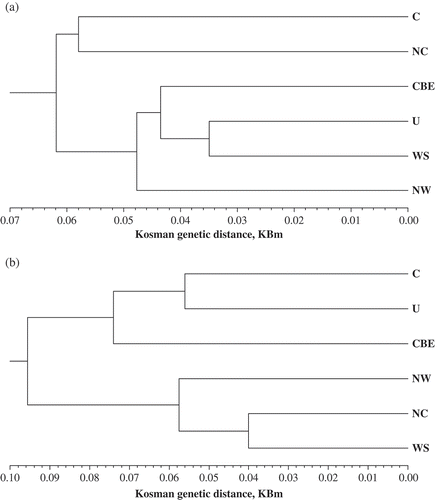
Molecular study
Six RAPD and one UP-PCR polymorphic primers were selected to perform molecular markers analysis of P. triticina isolates. Five RAPD primers (450, 538, 280.02, 270.09 and 480.09) distinguished two polymorphic fragments each; another one (490) detected three fragments; and UP-PCR primer AS4 generated one polymorphic locus. Altogether, 14 polymorphic binary loci with two ‘alleles’ (0 and 1) were utilized to obtain profiles of isolates with molecular markers.
There were 71 molecular genotypes distinguished among 417 P. triticina isolates. The number of different genotypes in the regional populations varied from 11 to 37 in the North Caucasian and Northwestern collections, respectively (). About 50% of all the genotypes found in each population (except the Low Volga region) were represented by two or more isolates. Frequencies of predominant genotypes varied from 10% to 53% in the Low Volga and North Caucasian populations, respectively (). The genotype richness in the Low Volga region was the highest (SR = 0.842; ), and the corresponding genotypes were the most evenly distributed (E = 0.983). The minimum richness and evenness were established in the Ural (SR = 0.250) and North Caucasian (E = 0.694) populations, respectively ().
Table 4. Descriptive parameters of Russian regional populations of P. triticina in 2007 obtained with molecular markers
Only one molecular genotype was detected in all the collections, with frequencies ranging from 5% in the Central Black Earth and Volga regions to 54% in the North Caucasian region. Common leaf rust genotypes were identified in different regions () – the Northwestern and North Caucasian regions shared 11 genotypes (maximum value), while only two genotypes (minimum) were shared by the Low Volga and West Siberian regions.
Table 5. Number of common molecular genotypes of P. triticina in Russian regional populations
Qualitatively different estimates of molecular diversity within the populations were obtained with the gene (Nei), genotypic (Shannon normalized) and genetic (Kosman) diversity measures (data not shown). Since KWm and KWj indices ranked the populations identically (KWj data not shown) and their values were strongly correlated, we further refer only to the KWm genetic diversity measure (). Molecular diversity within the Low Volga population of P. triticina was considerably higher (KWm = 0.308) than that within all other regions, while the lowest molecular diversity was within the North Caucasian collection (KWm = 0.080).
Comparison of different types of distances between the regional collections of P. triticina with the Mantel test (Mantel, Citation1967) established rather good correspondence between them. The genetic distances KBm and KBj were very strongly correlated (0.998), while the correlations of the genetic KBm distance with the genotypic and gene distances were 0.926 and 0.830, respectively. This may explain the qualitative identity of topology of the UPGMA dendrograms based on the corresponding distance matrices (data not shown). Thus, only the UPGMA dendrogram with regard to the genetic distance KBm is presented in b to describe relationships between the regional leaf rust populations derived from the molecular marker data. This dendrogram conveys nearly ‘actual’ relationships between the populations because its degree of fit to the corresponding KBm distance matrix was very good (cophenetic correlation = 0.917). The differences between all populations measured with the KBm distance were statistically significant. The most similar were collections from North Caucasus and Western Siberia, which together with the Northwestern one formed a group of closely related populations of P. triticina. Another group consisted of the Central, Ural, and Central Black Earth populations ( b).
No association was found between the virulence data and the molecular data. A negative correlation (−0.213; Mantel test) was ascertained between the KBm distances among populations for virulence and molecular markers. Relationships between the leaf rust regional collections, as expressed by the corresponding UPGMA dendrograms (), were also inconsistent.
Discussion
Virulence analysis of the Russian leaf rust populations revealed low differentiating ability when using the international set of differentials. Isolates from all populations were virulent on about 19 differentials on average. The extremely high frequencies of virulence to most resistance genes tested (Lr2b, Lr2c, Lr3а, Lr3bg, Lr3ka, Lr10, Lr11, Lr14a, Lr14b, Lr16, Lr17, Lr18, Lr21, Lr30 and LrB) could be explained by the wide distribution of these genes in Russian commercial wheat varieties for a long time, which makes the differential lines inefficient for virulence analysis of Russian P. triticina populations. According to McIntosh et al. (Citation1995), about 26 genes had been identified in wheat germplasm from 1946 to 1980 and most of them were utilized by Russian wheat breeders. Zhemchuzhina et al. (Citation1992) found 11 Lr-genes (Lr1, Lr2b, Lr2c, Lr3a, Lr10, Lr14a, Lr16, Lr17, Lr20, Lr23, Lr26) in 47 of 60 wheat varieties released in the North Caucuses before 1990. Some of the most successful winter wheat varieties with ineffective genes have been widely utilized in modern breeding in Russia. These include ‘Mironovskaya 808’, ‘Tarasovskaya 29’, ‘Bezostaya 1’ and ‘Skorospelka 3’ (Lr3а); ‘Zernogradka 6’, ‘Zernogradka 31’ and ‘Krasnodarskaya 70’ (Lr2b, Lr17); ‘Labinka’ and ‘Odesskaya krasnokolosaya’ (Lr14a); ‘Obriy’, ‘Zirka’ and ‘Olviya’ (Lr17 Lr23); and ‘Albatros odesskiy’ (Lr30) (Zhemchuzhina et al., Citation1992). A limited number of molecular markers are known for identification of Lr genes, thus the estimation of actual distribution of Lr genes in Russian varieties is unknown. Distribution of the ineffective Lr10 and Lr26 genes in modern Russian varieties (above 30% and 15% of varieties, respectively) was established by Gultyaeva et al. (Citation2009). High frequencies of virulence to some Lr genes also may be the ‘hitchhiking’ phenomenon, when unnecessary virulence is present. For instance, the presence of Lr21 was not found in Russian varieties with molecular markers (Gultyaeva et al., Citation2009), but virulence to Lr21 was high in 2007 and in previous years (Lind & Gultyaeva, Citation2007). Finally, it is possible that erroneous virulence assessments occurred, due to the limited reliability of the detached leaf method for several Lr genes (Felsenstein et al., Citation1998). However, weak effectiveness of most seedling Lr genes in Russian regions in 2006–2008 was also reported by Zhemchuzhina & Kurkova (Citation2010).
Only five differentials (Lr1, Lr2а, Lr15, Lr20 and Lr26) provided variable patterns of virulence frequency (30–100%) across the regional populations. Virulence to Lr26 was the most variable among the regional populations in 2007. Long-term analysis of Russian populations revealed fluctuations in virulence (Lind & Gultyaeva, Citation2007) that might be caused by broad range of cultivation of varieties with Lr26 gene and their uneven proportion in the regions in different years. The increase in the frequency of virulence to Lr1 during the last decade is likely due to the massive cultivation of wheat varieties with Lr1 gene (e.g. ‘Moskovskaya 35’, ‘Deya’, ‘TAU’) (Gultyaeva et al., Citation2009). Cultivars with Lr9 and Lr19 were introduced into Russia in the late 1980s and early 1990s, respectively. Lr9 is still effective, while Lr19 is effective in the Central, North Caucasus and Northwestern regions where cultivars with this gene are not grown. In the Volga region, resistance to Lr19 was defeated since the end of the 1990s (Sibikeev & Krupnov, Citation2007). In the current virulence survey, we did not detect virulence to Lr19 in the Low Volga region probably because a relatively small number of isolates obtained from a single variety was tested in this region. Virulence to Lr24 was detected for the first time in Russia (the Central and Central Black Earth regions) in the present survey.
Very high levels of virulence on Lr16, Lr18 and Lr21 were established in Russia, compared with some other regions of the world. Thus, virulence frequencies on these genes vary from very low in Canada and USA (McCallum & Seto-Goh, Citation2008; Kolmer et al., Citation2009) to much higher in Europe and Middle East (Manisterski et al., Citation2000; Mesterházy et al., Citation2000; McVey et al., Citation2004; Lind & Gultyaeva, Citation2007; Manninger, Citation2009; Hanzalová et al., Citation2010). There could be some differences in how the infection types are interpreted in different regions. However, it seems that the Russian population of wheat leaf rust is rather similar to the European populations, which are fundamentally more virulent for Lr16, Lr18 and Lr21 genes than the populations in North America.
The virulence pattern of wheat leaf rust in the North Caucasian region was the most distinct because of the large range of winter wheat cultivars grown there, the abundance of wild relatives of wheat, and the ability of the pathogen to survive during winter periods. Collections from Ural and West Siberia regions were closely related, which may be due to the genetically similar wheat cultivars with a uniform set of resistance genes grown in these regions. These populations of P. triticina differ from the Central and North Western regions, where possible migration of the rust spores from the neighbouring European countries may affect the pathogen population composition.
Genetic distances, based on virulence data, between the regional collections of P. triticina in Russia were very small (though statistically significant) despite the considerable geographic distances between the regions (many hundreds or even thousands of kilometres). These relationships among the populations may be explained by the wheat cultivars that have similar compositions of resistance genes, by the variable wind direction in the European and West Siberian parts of Russia, and by the absence of geographical barriers (except the Ural Mountains). Relative uniformity of the Russian population of P. triticina is in contrast to the general structure of wheat leaf rust populations in the USA. Although geographical distances among the Russian regions and among seven areas of the USA are comparable, populations of P. triticina in USA were much more distant from each other (values of KBm varied from 0.06 to 0.390 in USA [ in Kolmer et al., Citation2003] relative to 0.027–0.073 in Russia). Virulence diversities within the regions were also much higher in the USA compared with Russia (see here and in Kolmer et al., Citation2003).
The relationships among the regional collections of P. triticina based on molecular marker data differed from that established with virulence data. The six populations were separated into two distinct clusters, which could be characterized by specific molecular genotypes. One cluster included the Ural, Central Black Earth, and Central regions (four specific RAPD genotypes, 9% of total 417 isolates tested), and the other one consisted of Northwestern, North Caucasian and West Siberian regions (three specific RAPD genotypes, 5% of total number of isolates).
Estimates of diversity within the groups of P. triticina isolates were inconsistent between virulence data and molecular markers. The high virulence diversity within the Northwestern and North Caucasian populations was probably due to the sample collection, which was mainly from breeding stations with a variable spectrum of resistance genes in the host plants. This would support the paradigm that the main driving force of virulence evolution of wheat leaf rust in Russia is selection pressure from resistance genes in the host plants. Existence of common molecular genotypes in geographically distant regions might result from long-distance migration of P. triticina urediniospores in Russia. However, the molecular markers used in this study did not allow for comprehensive analyses of the genetic variation.
No reliable region-specific combinations of virulence and avirulence were detected in the Russian populations, unlike the results obtained in wheat leaf rust surveys in Canada and Europe (Kolmer et al., Citation1995; Park et al., Citation2000). In Europe, nearly all detected virulence phenotypes could be separated into two groups on the basis of virulence or avirulence to the genes Lr3a, Lr3bg, Lr3ka and Lr30 (Park et al., Citation2000). However, this division was not so apparent using molecular marker (RAPD) data, indicating that phenotypes within the two groups may not be as closely related as the virulence data would suggest. All Russian isolates were virulent on Lr30, and only a few were avirulent on Lr3a, Lr3bg and Lr3ka. The latter isolates were sporadically detected in North Caucasian, Ural and West Siberian regions and just like the European P. triticina populations, their molecular genotypes were not identical. Moreover, the isolates avirulent on Lr3a, Lr3bg and Lr3ka shared three common, widely distributed molecular genotypes with many other virulence phenotypes. This situation was contrary to that reported in Canada, where two major geographically separated groups of P. triticina had certain virulence and molecular (RAPD and SSR) genotypes (Kolmer et al., Citation1995). One group consisted of virulence phenotypes that were either virulent or avirulent on both Lr2a and Lr2c, whereas phenotypes from the other group were avirulent on Lr2a and virulent on Lr2c (Kolmer et al., Citation1995). The majority of the Russian isolates were virulent on both Lr2a and Lr2c, but 13% of the isolates from the Northwestern and North Caucasian regions were avirulent on Lr2a and virulent on Lr2c. Molecular genotypes were not unique for isolates from these groups, while some isolates from the different groups had identical molecular patterns.
Methodological issues of data analysis in plant pathology require attention, because inferences from diversity analysis of populations can be driven by techniques of diversity and distance assessments and not only by the data. Commonly used measures of diversity within or between populations consider either phenotype (genotype) frequencies (e.g. Shannon normalized diversity index or Rogers' distance) or virulence (allele) frequencies (e.g. Nei diversity or Nei gene distance). Kosman's diversity (KWm ) and distance (KBm ) measures are more suitable for populations with an asexual or mixed mode of reproduction, because they take into account not only the phenotype (genotype) frequencies but also the genetic similarity between virulence or banding patterns of isolates (Kosman, Citation1996; Kosman & Leonard, Citation2007). Qualitative differences in diversity and distance assessments with the gene, genotypic and genetic measures, including discrepancy in topology of the corresponding UPGMA dendrograms, were reported here. Such disparity may lead to false interpretations of relationship between populations. Therefore, a uniform diversity analysis is an essential condition for valid comparison of outcomes and inferences obtained in different studies.
Acknowledgements
The studies were conducted with financial support from the Russian Foundation for Basic Researches (Grant No. 07-04-01455а). Collaboration between Russian and Israeli researchers was initiated thanks to the travel grant from Israeli Academy of Sciences and Humanities. We thank Prof. A. Dinoor (Hebrew University of Jerusalem) and anonymous reviewers for critical reading of the manuscript and helpful comments and suggestions.
References
- Bulat , S. , Lubeck , M. , Mironenko , N. , Jensen , D. and Lubeck , P. 1998 . UP-PCR analysis and ITS1 ribotyping of Trichoderma and Gliocladium fungi . Mycol. Res , 102 : 933 – 943 .
- Hanzalová , A. , Huszár , J. , Herzová , E. and Bartoš , P. 2010 . Physiologic specialization of wheat leaf rust (Puccinia triticina Eriks.) in the Slovak Republic in 2005, 2006 and 2008 . Czech J. Genet. Plant Breed. , 46 : 114 – 121 .
- Duan , X. , Enjalbert , J. , Vautrin , D. , Solignac , C. and Giraud , T. 2003 . Isolation of 12 microsatellite loci, using an enrichment protocol, in the phytopathogenic fungus Puccinia triticina . Mol. Ecol Notes , 3 : 65 – 67 .
- Felsenstein , F.G. , Park , R.F. and Zeller , F.J. 1998 . The use of detached seedling leaves of Triticum aestivum to study pathogenicity in Puccinia recondita f. sp. tritici . J. Phytopathol. , 146 : 115 – 121 .
- Gultyaeva , E. I. Sept 16–21, 2007 2007 . “ Wheat leaf rust survey in Russia in 2001–2006 ” . In Proceedings of the XV Congress of European Mycologists Sept 16–21, 2007 , 252 Saint Petersburg , , Russia
- Gultyaeva , E. , Baranova , O. , Alpatyeva , N. and Krämer , I. Oct. 13–16, 2009 2009 . “ Occurrence of leaf rust resistance genes in Russian wheat varieties and their influence on virulence frequencies in the pathogen population ” . In Proceedings of the 12 International Cereal Rusts and Powdery Mildews Conference Oct. 13–16, 2009 , 89 Antalya , , Turkey
- Justesen , A.F. , Ridout , C.J. and Hovmøller , M.S. 2002 . The recent history of Puccinia striiformis f.sp. tritici in Denmark as revealed by disease incidence and AFLP markers . Plant Pathol. , 51 : 13 – 23 .
- Kolmer , J.A. 2001 . Molecular polymorphism and virulence phenotypes of the wheat leaf rust fungus Puccinia triticina in Canada . Can. J. Bot. , 79 : 917 – 926 .
- Kolmer , J.A. , Liu , J.Q. and Sies , M. 1995 . Virulence and molecular polymorphism in Puccinia recondita f. sp. tritici in Canada . Phytopathology , 85 : 276 – 285 .
- Kolmer , J.A. , Long , D.L. and Hughes , M.E. 2009 . Physiologic specialization of Puccinia triticina on wheat in the United States in 2007 . Plant Dis. , 93 : 538 – 544 .
- Kolmer , J.A. , Long , D.L. , Kosman , E. and Hughes , M.E. 2003 . Physiologic specialization of Puccinia triticina on wheat in the United States in 2001 . Plant Dis. , 87 : 859 – 866 .
- Kolmer , J.A. and Ordoñez , M.E. 2007 . Genetic differentiation of Puccinia triticina populations in Central Asia and the Caucasus . Phytopathology , 97 : 1141 – 1149 .
- Kosman , E. 1996 . Difference and diversity of plant pathogen populations. A new approach for measuring . Phytopathology , 86 : 1152 – 1155 .
- Kosman, E., Dinoor, A., Herrmann, A., & Schachtel G.A. (2008). Virulence Analysis Tool (VAT). User Manual. http://www.tau.ac.il/lifesci/departments/plant_s/members/kosman/VAT.html (http://http://www.tau.ac.il/lifesci/departments/plant_s/members/kosman/VAT.html)
- Kosman , E. and Leonard , K.J. 2005 . Similarity coefficients for molecular markers in studies of genetic relationships between individuals for haploid, diploid, and polyploid species . Mol. Ecol. , 14 : 415 – 424 .
- Kosman , E. and Leonard , K.J. 2007 . Conceptual analysis of methods applied to assessment of diversity within and distance between populations with asexual or mixed mode of reproduction . New Phytol. , 174 : 683 – 696 .
- Lind , V. and Gultyaeva , E. 2007 . Virulence of Puccinia triticina on winter wheat in Germany and the European regions of Russian Federation . J. Phytopathol. , 155 : 13 – 21 .
- Long , D.L. and Kolmer , J.A. 1989 . A North American system of nomenclature for Puccinia recondita f.sp. tritici . Phytopathology , 79 : 525 – 529 .
- Manisterski , J. , Eyal , Z. , Ben-Yehuda , P. and Kosman , E. 2000 . Comparative analysis of indices in the study of virulence diversity between and within populations of Puccinia recondita f. sp. tritici in Israel . Phytopathology , 90 : 601 – 607 .
- Manninger , K. Oct. 13–16, 2009 2009 . “ Occurrence and virulence changes of leaf rust in Hungary during 1999–2008 ” . In Proceedings of the 12 International Cereal Rusts and Powdery Mildews Conference Oct. 13–16, 2009 , 37 Antalya , , Turkey
- Mantel , N.A. 1967 . The detection of disease clustering and a generalized regression approach . Cancer Res. , 27 : 209 – 220 .
- Mantovani , P. , Maccaferri , M. and Tuberosa , R. 2010 . Virulence phenotypes and molecular genotypes in collections of Puccinia triticina from Italy . Plant Dis. , 94 : 420 – 424 .
- McCallum , B. D. and Seto-Goh , P. 2008 . Physiologic specialization of Puccinia triticina in Canada in 2005 . Can. J. Plant Pathol. , 30 : 124 – 132 .
- McIntosh , R.A. , Welling , C.R. and Park , R.F. 1995 . Wheat Rusts: an Atlas of Resistance Genes , 200 Dordrecht : CSIRO, Australia, and Kluwer Academic Publishers .
- McVey , D.V. , Nazim , M. , Leonard , K.J. and Long , D.L. 2004 . Patterns of virulence diversity in Puccinia triticina on wheat in Egypt and the United States in 1998–2000 . Plant. Dis. , 88 : 271 – 279 .
- Mebrate , S.A. , Dehne , H.W. , Pillen , K. and Oerke , E.C. 2006 . Molecular diversity in Puccinia triticina isolates from Ethiopia and Germany . Phytopathology , 154 : 701 – 710 .
- Mesterházy , Á. , Bartoš , P. , Goyeau , G. , Niks , R. and al , et . 2000 . European virulence survey for leaf rust in wheat . Agronomie , 20 : 793 – 804 .
- Mikhailova , L.A. 1996 . The population structure of the causal agent of wheat leaf rust Puccinia recondita f.sp. tritici Rob.ex Desm. V. The areals and paths of spore migration . Mikologia i Fitopatologia , 30 : 84 – 90 . (In Russian)
- Mikhailova , L.A. and Gultyaeva , E.I. Nov. 11–13, 1997 1997 . “ Puccinia recondita Rob.ex Desm. f.sp. tritici populations in the European and Asian parts of CIS (former USSR) ” . In Proceedings of the conference ‘Approaches to Improving Disease Resistance to Meet Future Needs: Airborne Pathogens of Wheat and Barley. Nov. 11–13, 1997 , 134 – 137 . Praha , , Czech Republic
- Ordonez , M.E. , German , S.E. and Kolmer , J.A. 2010 . Genetic differentiation within the Puccinia triticina population in South America and comparison with the North American population suggests common ancestry and intercontinental migration . Phytopathology , 100 : 376 – 383 .
- Ordoñez , M.E. and Kolmer , J.A. 2009 . Differentiation of molecular genotypes and virulence phenotypes of Puccinia triticina from common wheat in North America . Phytopathology , 99 : 750 – 758 .
- Park , R.F. , Jahoor , A. and Felsenstein , F.G. 2000 . Population structure of Puccinia recondita in Western Europe during 1995 as assessed by variability in pathogenicity and molecular markers . J. Phytopathol. , 148 : 169 – 179 .
- Sanin, S.S., Nazarova, L.N., & Ibragimov, T.Z. (2004). The modern epidemiological situation of cereal rusts in European Russia. In Proceedings of the 11th International Cereal Rusts and Powdery Mildews Conference. Aug. 22–27, 2004, Norwich, England (p. 2.25) www.crpmb.org/icrpmc11/abstracts.htm (http://www.crpmb.org/icrpmc11/abstracts.htm)
- Sibikeev , S.N. and Krupnov , V.A. 2007 . Evolution of leaf rust and protection against it of bread wheat in the Volga region . Bulletin Saratov State Agrarian University in Honor of N.I. Vavilov , : 92 – 94 . (In Russian)
- Szabo , L.S. and Kolmer , J.A. 2007 . Development of simple sequence repeat markers for the plant pathogenic rust fungus Puccinia triticina . Mol. Ecol. Notes , 7 : 708 – 710 .
- Zhemchuzhina , A. and Kurkova , N. May 30–31, 2010 2010 . “ Structure of population of Puccinia triticina in various regions of Russia in 2006–2008 ” . In Proceedings of the BGRI Technical Workshop May 30–31, 2010 , 27 St. Petersburg , , Russia
- Zhemchuzhina , A.I. , Nazarova , L.N. and Dumchenko , A.M. 1992 . Resistance of winter wheat to leaf rust . Selekciya i semenovodstvo, 1 , 10 : 6 – 11 . (in Russian)