Abstract
Sudden death syndrome of soybean, caused by Fusarium virguliforme, is one of the most damaging diseases affecting soybean production in the Midwestern region of the USA. To date, there has been very low genetic variation detected in F. virguliforme populations, although isolates can exhibit differing aggressiveness on soybean. To further investigate the genetics behind this variation in aggressiveness, multiple fingerprint analyses were conducted on a collection of F. virguliforme isolates from Iowa and neighbouring states, and their aggressiveness was tested using greenhouse and culture filtrate assays. Twelve RAPD primers identified 13 genotypes, while hybridization of the (CAT)5 probe to Pst I-restricted genomic DNA yielded eight genotypes. Fingerprint patterns obtained by RFLP analysis of mitochondrial DNA with the enzyme HaeIII were identical for all F. virguliforme isolates. Combined analysis of the RAPD and (CAT)5 binary data identified 25 genotypes within F. virguliforme, indicating a greater amount of genetic diversity than was previously known. Disease severity and plant growth varied significantly among isolates, but isolate aggressiveness was not associated with genetic variation. This study provides evidence for the existence of genetic variation in F. virguliforme but suggests that minor quantitative traits and environmental interactions are primarily responsible for the variation in aggressiveness found among isolates within the species.
Résumé
Le syndrome de la mort subite du soja, causé par Fusarium virguliforme, est une des maladies du soja qui provoque le plus de dommages dans le Midwest américain. À ce jour, très peu de variations génétiques ont été détectées dans les populations de F. virguliforme, bien que des isolats puissent afficher des degrés variables de virulence à l'égard du soja. Pour étudier la génétique à l'origine de cette variation, de multiples analyses de l'empreinte génétique ont été faites sur une collection d'isolats de F. virguliforme provenant de l'Iowa et des États voisins. Leur virulence a été testée au moyen de filtrats de serre et de culture. Douze sondes RAPD ont identifié 13 génotypes tandis que l'hybridation entre la sonde (CAT)5 et l'ADN génomique restreint par Pst-I en a produit huit. Les arrangements d'empreintes obtenus par analyse RFLP de l'ADN mitochondrial avec l'enzyme HaeIII étaient identiques pour tous les isolats de F. virguliforme. L'analyse combinée des données binaires de la RAPD et de la sonde (CAT)5 a permis d'identifier 25 génotypes chez F. virguliforme, ce qui représente une plus grande diversité génétique que celle connue à ce jour. La gravité de la maladie et la croissance des plantes variaient significativement selon les isolats, mais la virulence des isolats n'était pas toujours associée à la variation génétique. Cette étude démontre l'existence de la variation génétique chez F. virguliforme, mais suggère que des traits quantitatifs mineurs et des interactions environnementales sont principalement responsables de la variation sur le plan de la virulence chez les isolats de l'espèce.
Introduction
Sudden death syndrome (SDS) of soybean (Glycine max L.) is an economically important disease that causes major yield losses in North and South America (Roy et al., Citation1997). The disease is characterized by root rot, foliar interveinal chlorosis and necrosis, and premature defoliation (Roy et al., Citation1997; Aoki et al., Citation2005). In the USA, the disease was first detected in Arkansas in 1971 (Hirrel, Citation1983), later spreading northwards along the Mississippi river valley and being reported in Indiana and Illinois in 1986 (Rupe et al., Citation1989), Iowa in 1993 (Yang & Lundeen, Citation1997), Minnesota in 2002 (Kurle et al., Citation2003), Wisconsin in 2007 (Bernstein et al., Citation2007), and Michigan in 2010 (Chilvers et al., Citation2010). SDS is currently found in almost all soybean-producing regions in the USA (Roy et al., Citation1997). Yield losses typically range between 5 and 15%, with up to 80% loss in individual fields (Roy et al., Citation1997). Annual losses of 0.3 to 2.0 million tons were estimated for the period between 1997 and 2007 (Wrather & Koenning, Citation2009). Worldwide, SDS disease has also been reported in Canada (Anderson & Tenuta, Citation1998) and parts of South America, including Argentina (Ploper, Citation1993), Brazil (Yorinori et al., Citation1993), Bolivia, Paraguay (Yorinori, Citation1999) and Uruguay (Ploper et al., Citation2003).
The soilborne fungus Fusarium virguliforme O'Donnell & T. Aoki (formerly F. solani (Mart.) Sacc. f. sp. glycines) is the only causal agent of SDS in North America, whereas four Fusarium species, namely F. virguliforme, F. tucumaniae T. Aoki, O'Donnell, Yos. Homma & Lattanzi, F. brasiliense T. Aoki & O'Donnell and F. crassistipitatum T. Aoki, M. M. Scandiani & O'Donnell cause the disease in South America (Aoki et al., Citation2005; O'Donnell et al., Citation2010; Aoki et al., Citation2011). Various molecular techniques have been used to decipher the species-limits and infer the evolutionary relationships among SDS-causing isolates and other members of the F. solani species complex. These studies have included sequence analyses of the mitochondrial small subunit rDNA (Li et al., Citation2000) and nuclear rDNA (O'Donnell & Gray, Citation1995), as well as restriction fragment length polymorphism (RFLP) analysis of the mitochondrial genome and internal transcribed spacer of the rDNA (Rupe et al., Citation2001; Arruda et al., Citation2005; Malvick & Bussey, Citation2008). Results from these studies showed a nearly homogeneous population of F. virguliforme exists in North America. A multiloci genetic assay based on nucleotide polymorphism within the nuclear ribosomal intergenic spacer rDNA and two anonymous intergenic regions differentiated the soybean SDS pathogen from two closely related pathogens, F. cuneirostrum and F. phaseoli, which cause root rot on Phaseolus and mung beans (O'Donnell et al., Citation2010).
Despite the observed genetic homogeneity (Achenbach & Patrick, Citation1996; Achenbach et al., Citation1997; Aoki et al., Citation2003), many studies have shown considerable variation in aggressiveness among F. virguliforme isolates in both greenhouse and field studies (Roy et al., Citation1989; Malvick & Bussey, Citation2008). The existence of races of F. virguliforme has also been suggested based on differences in aggressiveness (Lim & Jin, Citation1991), although there are no established soybean differentials for race determination. While SDS development and severity is highly dependent on environmental conditions (Scherm et al., Citation1998), this variation in aggressiveness is surprising given the clonal reproductive nature of the pathogen (Scherm & Yang, Citation1996). We hypothesized that differences in aggressiveness found among F. virguliforme isolates may be caused by minor genetic variation within the pathogen population, namely, many quantitative genetic traits.
Some evidence exists for limited genetic variability in F. virguliforme. In one study, random amplified polymorphic DNA (RAPD) analysis of 55 F. virguliforme isolates from nine states in the USA showed the existence of two groups, which the authors called amplitypes (Achenbach & Patrick, Citation1996). Chromosome length polymorphisms and two distinct karyotypic patterns have also been reported from preliminary studies with F. virguliforme isolates (Mansouri, Citation2009). There is a need to further investigate the genetic variation within the F. virguliforme genome and determine if it is associated with variations in isolate aggressiveness. In a recent study, isolates showed a range of aggressiveness levels, but no genetic variation was found through sequence analysis of the ITS, IGS and EF-1 alpha regions (Malvick & Bussey, Citation2008). DNA fingerprint analyses using RAPDs and microsatellite probes provide better discrimination among genotypes due to the large number of characterized fragments from the genome (Taylor et al., Citation1999). These methods have been used to detect previously unknown genetic variations in fungal pathogens such as Ceratocystis cacaofunesta Engelbrecht & Harrington, Eutypa lata (Pers : Fr.) Tul. & C. Tul, and Cadophora gregata Harrington & McNew (Harrington et al., Citation2003; Péros & Berger, Citation2003; Engelbrecht et al., Citation2007). The objective of this study was to employ several molecular markers to characterize the intraspecific genetic variation in 72 F. virguliforme isolates from Iowa and to test whether genetic variation was associated with variation in aggressiveness among isolates.
Materials and methods
Fungal isolates
A total of 72 isolates of F. virguliforme collected from diseased soybean roots () were used in this study. Fifty-eight of these isolates were collected from 16 different counties in Iowa. Thirty-three of the 58 isolates were collected from fields surveyed in 2006–2007 and are represented by the LL code, while the remaining 25 isolates were obtained from a state-wide survey that was conducted in 1994 and 1995 (Yang & Lundeen, Citation1997) and are represented by the Fsg-ISU code. During the survey of 2006–2007, F. virguliforme was isolated from roots using a modified Nash and Snyder medium (MNSM) (Rupe et al., Citation1997) and transferred onto potato dextrose agar (PDA) for identification based on published morphological characteristics (Aoki et al., Citation2003). All the isolates were single-spored, and identification was further confirmed by PCR using primers Fsg 1 and Fsg 2 designed from the mitochondrial small subunit rDNA and by sequence analysis of the EF-1α gene (Li et al., Citation2000; Aoki et al., Citation2003). Also included in this study were 10 isolates of F. virguliforme from Minnesota, three isolates from Illinois and one isolate from Arkansas, which were similarly single-spored and characterized by DNA sequencing ().
Table 1. Origin, year of collection, genotype classes obtained from analysis of RAPD and (CAT)5 markers, and genetic subgroups of Fusarium virguliforme isolates used in this study
DNA extraction
Isolates were grown in malt yeast extract liquid medium (3 g malt extract, 3 g yeast extract, 5 g peptone, 20 g dextrose, 2 g ammonium sulphate in 1 L of water) (O'Donnell, Citation1992) at room temperature (20–35 °C) for 4 days on a rotary shaker (115 rpm). Preliminary experiments showed that this medium minimized the production of polysaccharides by the fungus during growth and yielded the best quality of DNA for all analyses. Mycelial mats were collected by vacuum filtration on Whatman #54 filter paper (Whatman International Ltd, Maidstone, England) and freeze-dried overnight. Total genomic DNA was extracted using the method described by DeScenzo and Harrington (Citation1994) and quantified using a TKO-100 minifluorometer (Hoefer Scientific Instruments, San Francisco, CA).
DNA fingerprint analysis
Four molecular techniques were used to generate fingerprints for analysis of genetic variation: mitochondrial restriction fragment length polymorphisms (RFLP), minisatellite- and microsatellite-primed PCR, nuclear DNA analysis using microsatellite probes (CAT)5 and (CAC)5, and random amplification of polymorphic DNA (RAPD). Each of the four fingerprinting methods was repeated at least once for each isolate, and only bands that were consistently visible and can be unambiguously scored in all replicate gels were analyzed.
Mitochondrial DNA RFLP
For generating the mitochondrial RFLP patterns, total genomic DNA was digested with the restriction enzymes HaeIII, CfoI and MspI (Promega, Madison, WI), which recognize the restriction sites GGCC, GCGC and CCGG, respectively (Wingfield et al., Citation1996). For each isolate, 30 μg of DNA were restricted overnight at 37 °C, with 5 U of each enzyme per μg of DNA in a 500 μL total reaction volume. The digested DNA was concentrated by precipitation with 2 volumes of ethanol and 0.2 M NaCl. The pellet obtained was washed in 70% ethanol and dried in a speed vacuum for 45 min. The DNA pellet was then dissolved in TE buffer (10 mM Tris HCL, 1 mM EDTA) to a concentration of 0.67 μg μL−1, and 15 μL of the restricted product was used in electrophoresis. The restricted samples were separated on a 1.2 % agarose gel at 100 V for 16 h in 1.25× TBE and visualized under UV light after staining with ethidium bromide.
Minisatellite and microsatellite analyses
Four DNA fingerprint markers were used to generate binary data for analysis. The minisatellite primers M13 and T3B, and the microsatellite or intersimple sequence repeat (ISSR) primers (GACA)4 and (GTG)5 were used in 50 μL PCR amplification reactions (McDonald, Citation1997). PCR amplifications were performed in a 50 μL volume consisting of 2.5 mM MgCl2, 0.2 mM deoxyribonucleotide triphosphates, 0.5 μM of each primer, 1× PCR buffer, 0.05 U of Biolase Taq DNA polymerase (Bioline USA Inc. Randolph, MA), and 25 ng of template DNA. The reaction conditions were an initial denaturation at 94 °C for 5 min, 40 cycles of 95 °C for 1 min, 55 °C for 90 s and 72 °C for 2 min. The final extension was set at 72 °C for 5 min. At least two PCR reactions were performed for each isolate–primer combination to assess the consistency of amplification. Twenty μL of each PCR product were mixed with 5 μL of bromophenol blue and separated in 1% agarose gels. Electrophoresis was carried out in 0.5× TBE (Tris-Borate EDTA) buffer run for 6 h at 80 V. The gels were visualized in UV light after staining with ethidium bromide.
Nuclear DNA analysis using microsatellite probes (CAT)5 and (CAC)5
The oligonucleotides (CAT)5 and (CAC)5 were end-labelled with 32P-dCTP using polymerase terminal deoxynucleotidyl transferase (Invitrogen, Carlsbad, CA) and purified through a Sephadex G-25 column. Total genomic DNA obtained as described above was digested with the restriction enzyme PstI following the procedures described by DeScenzo and Harrington (Citation1994). The digested products were resolved on a 1.2% agarose gel (Bio-Rad laboratories, Hercules, CA) in 1.25 × TBE. The gels were run in a system provided with buffer recirculation at 100 V for 16 h. Once the gels were examined under UV light, they were dried with a gel drier for 45 min at room temperature, sealed in a plastic wrap and stored at 4 °C for further use. Hybridization and washing conditions were as previously described (DeScenzo & Harrington, Citation1994). After hybridization, the gels were exposed to an imaging screen (Molecular imager Fx, Biorad, Hercules, CA) for 2 days and scanned with a Pharos Fx Plus molecular imager (Biorad, Hercules, CA). Each isolate was fingerprinted in at least two gel runs, and only the bands present in both runs were scored.
RAPD analysis
Nineteen random amplified polymorphic DNA (RAPD) primers (Achenbach & Patrick, Citation1996; Achenbach et al., Citation1997) were individually screened for the generation of polymorphic loci. Twelve of these primers, namely OPA-01, OPA-02, OPA-04, OPA-13, OPA-19, OPB-05, OPF-01, OPF-13, OPL-12, OPN-13, PM02 and PM04 were selected for further analysis on the basis of reproducibility and the number of polymorphic loci generated. Each PCR reaction mix was set up as described above, except that 100 ng of DNA template and only one primer was used per reaction. PCR reactions were run for 40 cycles with the following steps: 94 °C for 3 min, 40 cycles of 94 °C for 1 min, 36 °C for 1 min and 72 °C for 2 min. The amplified products were run on 1.2% agarose gel in 1.25% TBE buffer for 6 h at 80 V and visualized in UV after staining with ethidium bromide.
Assessment of isolate aggressiveness
Thirty isolates of F. virguliforme were chosen from among the genotypic groups identified with the fingerprinting analysis and tested for aggressiveness on the soybean cultivars ‘Spencer’ and ‘92M91’ using soil inoculation and culture filtrate assays. ‘Spencer’ belongs to maturity group IV and is susceptible to F. virguliforme (Wilcox et al., Citation1989), while ‘92M91’ (Pioneer Hi-Bred, USA) belongs to maturity group II and is moderately resistant to F. virguliforme (Schmidt et al., Citation2008, Citation2009).
Greenhouse assays
Inoculum was prepared following the procedure described in Munkvold and O'Mara (Citation2002). A mixture of sand (1900 mL), cornmeal (380 mL) and water (110 mL) was autoclaved in 20 cm × 30 cm plastic autoclave bags (Fisher Scientific, Pittsburgh, PA) for 1 h at 121 °C on two consecutive days. Each bag then received 2 mL of a 1 × 106 spores mL−1 spore suspension. The bags were incubated in the dark at room temperature (24 ± 2 °C ) for 10 days, and the inoculum was thoroughly mixed on a daily basis. The inoculum of each isolate was mixed with sterile sand : soil (1 : 1) at a ratio of 1 part inoculum to 5 parts soil mix. Sterile sand : cornmeal mix was added at the same ratio for non-inoculated controls. Sterilized 21-cm tall and 4-cm diameter PVC cones were filled with the inoculum : soil mix and a single seed, pre-germinated for 3 days, was planted in each cone. The plants were maintained on a greenhouse bench at 26 ± 4 °C and 24 ± 4 °C with a 14-h photoperiod in June and September, respectively. The relative humidity of the greenhouse during this period varied between 20% in the evening and 70% in morning. The experiment was set up in a completely randomized factorial design, with 10 replicate plants per isolate by cultivar combination, and was conducted twice (trial 1 in June and trial 2 in September, 2009). Foliar disease severity defined as the per cent of total leaf area per plant showing typical SDS chlorosis or necrosis, was rated 10 days after planting and at 5-day intervals thereafter by visual assessments. Plants were destructively sampled 30 days after planting and visually assessed for root rot severity (the per cent root area showing brown to black discolouration), shoot fresh weight, shoot height and root dry weight. F. virguliforme density in the soil was quantified before planting (trial 2 only) and at the end of the experiment (both trials) on three randomly chosen conetainers per isolate using quantitative real-time PCR (Li et al., Citation2008).
Culture filtrate assays
Cell-free culture filtrates of F. virguliforme were prepared as described by Li et al. (Citation1999). Seeds of the soybean cultivars ‘Spencer’ and ‘91M92’ were grown in a growth chamber set to 16 h light at 23 °C and 8 h dark at 16 °C . Fifteen-day-old soybean seedlings were uprooted, and the roots were washed thoroughly in running tap water to remove soil, disinfested in 10% NaOCl for 1 min, then rinsed in sterile water and blotted dry. Single seedlings were placed in 50 mL falcon tubes containing 40 mL of the culture filtrate diluted with sterile water at a ratio of 1 : 5 for the first trial and 1 : 25 for the second trial of the experiment. Control plants were immersed in sterile culture media and water. The experiment was established as a completely randomized design with 10 replicate plants per isolate in trial 1 and six replicate plants per isolate in trial 2. The plants were rated visually for severity of SDS foliar symptoms 5 days after immersion in the culture filtrates and every 4 days thereafter, until 30 days after immersion. Trials 1 and 2 of the experiment were conducted in May and June, 2010, respectively.
Data analysis
For all fingerprinting methods, bands were scored as present or absent to generate a binary matrix. Each band in a profile was considered a single locus with two alleles, present or absent. Each unique combination of polymorphic bands was considered a genotype. Data were transformed into a matrix to calculate genetic distances among isolates. Cluster analysis was performed by UPGMA with Jaccard's similarity coefficient (Sneath & Sokal, Citation1973), and the goodness of fit of the phenogram for the similarity matrix was measured by cophenetic correlation (r) analysis using the NTSYSpc2.0 program (Exeter Software, Setauket, NY). Bootstrap values (1000 replicates) for each branch were calculated using the Phylogenetic Analysis Using Parsimony package (PAUP, ver. 4.0b10; Sinauer Associates). Congruency between the (CAT)5 and RAPD datasets was tested using partition-homogeneity (P-H) test implemented in PAUP. Additional tests (Kishino–Hasegawa: KH, Templeton test: TT, and the Winning-sites test: WS) were performed to assess whether the conflicting dendrogram topologies were significantly different from each other before combining the two datasets for further analysis. Population structure and differentiation were estimated using total genetic variation partitioned among geographic origin. The isolates were partitioned into three subpopulations in which recent and old isolations from Iowa formed two separate groups and the rest of the isolates from other states were combined to form the third subpopulation. All population analysis coefficients were computed using the program PopGen 1.31 (Yeh et al., Citation1999).
Disease and plant growth data from isolate aggressiveness tests were subjected to analysis of variance using the generalized linear mixed model (PROC MIXED) of SAS (SAS Institute, Cary, NC). A nested data structure was used considering isolates nested within genotype and genotypes nested within subgroup. Soybean cultivar and isolate subgroup were treated as fixed effects, while trial, genotype and isolate were considered random effects. The area under disease progress curves (AUDPC) for foliar severity was calculated using the trapezoidal integration method (Campbell & Madden, Citation1990). For root rot, Pearson correlation coefficients and statistical significance were calculated using the PROC CORR procedure of SAS (SAS Institute, Cary, NC) to determine associations between foliar severity AUDPC, root rot severity, root dry weight, shoot height and shoot weight, and inoculum density (log transformed), as well as to test the correlation between greenhouse and culture filtrate assays for foliar symptoms.
Results
RFLP, minisatellite and microsatellite analyses
In the RFLP analysis, the restriction enzyme HaeIII generated identical mitochondrial fingerprint patterns for all F. virguliforme isolates tested in this study. The fingerprinting patterns obtained with the restriction enzymes Cfo 1 and Msp 1 were not repeatable due to incomplete restriction reactions. The two minisatellite primers M13 and T3B, the microsatellite primers (GACA)4 and (GTG)5, and in-gel hybridization of genomic DNA with the (CAC)5 probe, also generated fingerprint patterns that were identical among all isolates of F. virguliforme. However, hybridization with the (CAT)5 probe revealed 30 fingerprint bands, six of which were polymorphic and confined to the high molecular weight DNA fragments (4, 9, 9.4, 15, 23 and 24 kb). No polymorphic bands were observed within the lower molecular weight fragments of the (CAT)5 hybridization. Analysis of the distribution of the six polymorphic bands generated a total of eight unique fingerprint profiles among the 72 F. virguliforme isolates from Iowa, Minnesota, Illinois and Arkansas (). The majority of the isolates collected in 2006 and 2007 from Iowa (LL-coded isolates) had the same fingerprint profile (C1). The 10 isolates of F. virguliforme from Minnesota were grouped in genotype C1, genotype C6 that included the two isolates from Illinois (Mont 1, and ILMont-1A), and genotype C7 that was unique to Minnesota (). The isolate from Arkansas (Rupe) belonged to genotype C4.
RAPD analysis
Two of the 12 RAPD primers generated identical fingerprints among the primers tested for all the F. virguliforme isolates. The primers OPB-05 and OPA-13 were the most polymorphic, generating four polymorphic bands each (data not shown). Seven of the 12 primers identified two genotypes among the 72 isolates of F. virguliforme, two primers identified three genotypes, and one primer (PM-04) identified four genotypes. Analysis of all 12 RAPD primers resulted in 13 genotypes among the 72 isolates of F. virguliforme (). The most common genotype (R1) contained more than 70% of the isolates. Among the most recent collection of isolates from Iowa, 23 isolates belonged to the R1 genotype, while 10 isolates were distributed among six genotypes other than R1 (). Of the 25 isolates collected prior to 2001, nine belonged to genotypes other than R1. All 10 isolates obtained from Minnesota had the R1 genotype. Isolate Mont-1 from Illinois belonged to genotype R8.
Combined fingerprint analysis
The dendrogram generated with the RAPD binary data conflicted to some degree with that generated with the (CAT)5 dataset. More genotypes were resolved with the RAPD dendrogram than within the (CAT)5 dendrogram (). The P-value for the P-H test to determine if there were any differences between the two dendrograms was 0.08 (data not shown). The three additional non-parametric tests for combinability of datasets yielded different results depending on the comparison being made. When the (CAT)5 dataset was compared with the RAPD dendrogram, results of all three tests showed that both RAPD and (CAT)5 dendrogram topologies were equally well supported by the (CAT)5 dataset (P = 0.0901 for KH, P = 0.0679 for TT test, P = 0.1250 for WS test). On the other hand, when the (CAT)5 dendrogram was compared with the RAPD dataset, the dendrogram was not supported by the RAPD dataset (P ≤ 0.0001 for KH test, P = 0.0006 for TT test, P ≤ 0.0001 for WS test). Based on results of the P-H test alone, the datasets were combined and analysis resulted in a total of 25 genotypes among the 72 isolates of F. virguliforme. Four possible subgroups could be distinguished within the dendrogram ().
Fig. 1. Dendrogram showing relatedness of genotypes of Fusarium virguliforme isolates from Iowa, Minnesota, and Illinois based on combined (CAT)5 and RAPD binary data and cluster analysis (unweighted paired group method with arithmetic means, UPGMA). Roman numerals at branch ends indicate the different genotypes (I to XXV), and numbers within parentheses represent the number of isolates within each genotype. The numbers on the branches represent bootstrap support values greater than 50%.
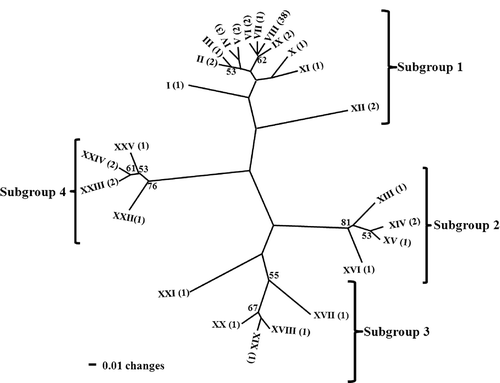
In the combined analysis, genotype VIII within subgroup 1 was geographically widely distributed and was found in 12 of the 16 counties from which samples were collected and comprised 43 isolates (). However, no isolates belonging to genotype VIII were identified in the Iowa counties of Henry, Jasper, Clinton and Fayette. Among the 1993 to 2000 Iowa isolates, 11 (44%) belonged to genotype VIII, while the rest were distributed among 11 genotypes. Out of the 33 isolates that made up the 2006 and 2007 Iowa isolates, 21 (63%) belonged to genotype VIII, while the remaining 12 isolates were distributed among 11 genotypes. Six isolates from Minnesota belonged to genotype VIII, two isolates to genotype IX, and two isolates to genotype IV. The Mont-1 and Fsg-ISU-19 isolates obtained from Illinois belonged to genotypes XIV and XXI, respectively, while the single isolate from Arkansas belonged to genotype XVIII. The cluster pattern showed no association between genotype and geographic origin.
Gene diversities in the total population and within three subpopulations (old Iowa isolates, new Iowa isolates and isolates from other states) were similar (h < 0.200). The extent of differentiation was low (Gst < 0.06) and the level of gene flow was relatively high (Nm = 8.431). There were 16 single-isolate genotypes distributed unequally among the four subgroups. The genetic distance between the recent and old isolates from Iowa was larger than between the old Iowa isolates and isolates from the other states (0.014 and 0.034, respectively). The genetic identities between the pairs of these three populations were very similar (0.986, 0.990 and 0.967).
Isolate aggressiveness
Greenhouse assays
Overall analysis of variance showed a significant effect (P < 0.0001) of trial and trial × isolate interaction on foliar severity (AUDPC) and root rot severity; therefore, data were analyzed separately for each trial. Interveinal chlorotic symptoms on leaves appeared 10 days after planting. In both trials of greenhouse experiments, foliar severity and root rot severity differed significantly (P ≤ 0.0004) among isolates, but no differences in these symptoms were observed (P > 0.05) among genotypes and among subgroups (). The main effect of cultivar was significant (P = 0.0483) for root rot severity in trial 1 and for foliar severity (P = 0.0004) in trial 2, and there was a significant cultivar × isolate interaction in trial 2 (). When data were analyzed separately by cultivar within each trial, the effects of isolate on root rot severity and foliar severity were always significant (P < 0.001), but there were no differences among genotype and subgroup, except for a significant effect (P = 0.019) of subgroup on foliar severity for cultivar ‘Spencer’ in trial 1.
Table 2. Analysis of variance on root rot severity and area under disease progress curve (AUDPC) for foliar symptom severity of soybean sudden death syndrome on plants grown in infested greenhouse soil or exposed to culture filtrates of Fusarium virguliforme in two experimental trials
Both soybean cultivars developed typical SDS symptoms in response to infection by F. virguliforme, but symptom severity was greater in trial 1 than in trial 2. Foliar severity at the final assessment (30 days post-inoculation) ranged from 50 to 82% and 0 to 77% in trial 1 and 2, respectively, on the susceptible cultivar ‘Spencer’, and from 47 to 82% and 0 to 48% in trial 1 and 2, respectively, on the moderately resistant cultivar 92M91. Non-inoculated control plants did not develop foliar symptoms in either trial. Foliar severity varied greatly among isolates and within each genetic group, with isolates ranging from high to low aggressiveness within genotypes and subgroups (). However, the relative aggressiveness of the isolates was not consistent between experimental trials. For example, the isolates LL0001, LL0091, Fsg-ISU 15-5 and Fsg-ISU 22G2 (numbered 11, 6, 17 and 27, respectively) were among the most aggressive in trial 1 but were among the least aggressive in trial 2 on both hosts. In contrast, isolates LL0130, LL0009, LL0105 and Fsg-ISU 6 (numbered 10, 12, 20 and 28, respectively) were consistently among the most aggressive, and isolates Fsg-ISU 17 and Fsg-ISU 22B1 (numbered 26 and 29, respectively) were consistently among the least aggressive isolates, in both trials and on both cultivars. The Mont-1 isolate (numbered 19) ranked among the moderately aggressive isolates in trial 1 and was among the most aggressive isolates in trial 2. Root rot severity also varied among isolates and within genetic groups, with a greater range in severity values observed in trial 2 (). Root rot severity was generally greater than 80% for most isolates in trial 1 and greater than 60% in trial 2 (). Isolates Fsg-ISU 17 and Fsg-ISU 22B1 (numbered 26 and 29, respectively) were the least aggressive in terms of causing root rot in both trials (). Root rot severity rating on non-inoculated controls plants was 0% in trial 1 and 18% in trial 2.
Fig. 2. Area under the disease progress curve (AUDPC) for foliar symptom severity on soybean ‘Spencer’ inoculated with 30 Fusarium virguliforme isolates and incubated for 30 days under greenhouse conditions in (a) trial 1 and (b) trial 2.
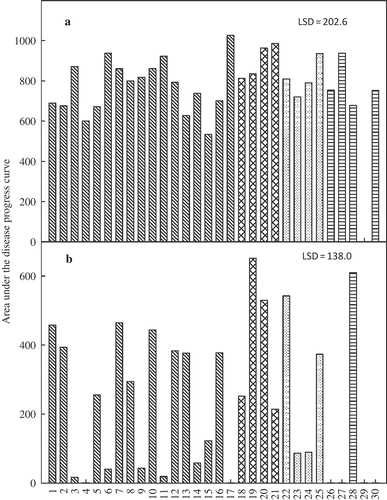
Fig. 3. Root rot severity on soybean plants ‘Spencer’ inoculated with 30 Fusarium virguliforme isolates and incubated for 30 days under greenhouse conditions in (a) trial 1 and (b) trial 2. Per cent root rot was evaluated at the end of the experiment and was calculated as the percentage of necrotic root area.
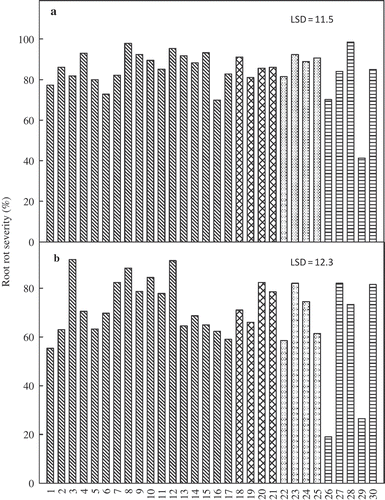
Analysis of variance on root dry weight, shoot dry weight and shoot height showed significant differences among isolates (P < 0.05) on both cultivars in both trials (data not shown). No differences in plant growth variables were found among genotypes or subgroups, except on cultivar 92M91, where subgroup had an effect on shoot height (P = 0.0074) in trial 2, and on shoot weight (P = 0.04) in trial 1. The pathogen density in soil at the end of the experiment varied among isolates (P = 0.002), and was 1000-fold greater in trial 1 compared with trial 2 (data not shown).
Significant correlations were found between disease and plant growth parameters in plants from the greenhouse assay. Foliar disease severity was negatively correlated with root dry weight, shoot height and shoot weight for both cultivars in both trials, but was only correlated with root rot severity for cultivar ‘Spencer’ in trial 1 (). Root rot severity was negatively correlated with root and shoot dry weight, and shoot height in trial 1, but was only correlated with shoot weight in trial 2 (). In trial 1, final inoculum density was significantly correlated with the AUDPC for foliar symptoms only on ‘Spencer’, and was correlated with other measured disease and plant growth parameters on both cultivars (). In trial 2, the final inoculum density was not correlated with any disease or growth parameters.
Table 3. Pearson's correlation coefficient values and significance for Fusarium virguliforme inoculum density in potted mix at the end of the experiment, foliar severity symptoms (area under disease progress curve, AUDPC), root dry weight, shoot length, shoot weight and root rot severity ratings of soybean ‘Spencer’ in trials 1 and 2 of the experiment
Culture filtrate assays
Overall analysis of variance showed a significant effect of trial (P = 0.0001) and trial × isolate interaction (P < 0.0001) on foliar symptoms caused by culture filtrates, so the main effects of cultivars, subgroup, genotype and isolate were tested separately for each trial. The main effect of cultivar was significant in both trials of the experiment, and there was a significant cultivar × isolate interaction in trial 2 (). In trial 1, genotypes had a significant effect on foliar symptoms, but there was no effect of isolate or subgroup. In trial 2, isolate and subgroup were significant, but genotype was not (). When data were analyzed separately by cultivar within each trial, there were significant effects of isolate (P = 0.0004 on ‘Spencer’ and P = 0.0001 on ‘91M92’) and subgroup (P = 0.02 on ‘Spencer’ and P = 0.0007 on 92M91) in trial 2, but there were no effects of isolate, genotype or subgroup in trial 1.
Both soybean cultivars developed typical SDS foliar symptoms (chlorosis and necrosis) four days after the roots were dipped in culture filtrates of F. virguliforme isolates. Foliar severity at the final assessment time ranged from 3 to 31% and 4 to 38% in trial 1 and 2, respectively, on susceptible ‘Spencer’, and from 2 to 57% and 4 to 28% in trial 1 and 2, respectively, on 92M91. Foliar severity varied greatly among isolates and within genetic subgroups on both trials (). The isolates LL0039, LL0038 and LL0019 (numbered 1, 8 and 21, respectively) were among the most aggressive on both cultivars, while isolates Fsg-ISU 19 and Fsg-ISU 17 (numbered 25 and 26, respectively) were among the least aggressive (). Some control plants showed a uniform leaf chlorosis that was very distinct from the characteristic foliar symptoms of SDS. This type of chlorosis was 2 and 19% in trial 1 and 2, respectively, on ‘Spencer’ and 0 and 16% in trial 1 and 2, respectively, on 92M91.
Correlations of isolate aggressiveness between assays
Based on foliar AUDPC values, there was no significant correlation (P > 0.05) between the two experimental trials of the greenhouse assay and between the two experimental trials of the culture filtrate assay for either cultivar. However, trial 1 of the greenhouse assay was correlated with trial 1 of the culture filtrate assay (P = 0.0007, r = 0.60) on cultivar ‘Spencer’, and with both trials of the culture filtrate assay (P = 0.006, r = 0.5 and P = 0.007, r = 0.49 for trials 1 and 2, respectively) on cultivar 92M91. No significant correlations were found between trials 1 and 2 of the greenhouse assay for root dry weight, shoot dry weight, shoot height and root rot severity, except for root rot severity on cultivar ‘Spencer’ (P = 0.0001, r = 0.64).
Discussion
Despite the genetic homogeneity observed within F. virguliforme in earlier studies (Achenbach & Patrick, Citation1996; Achenbach et al., Citation1997; Aoki et al., Citation2003, 2005; Arruda et al., Citation2005), this study revealed the existence of intraspecific genetic variation using RAPD and the (CAT)5 markers. However, no associations were found between the genetic markers and differences in aggressiveness detected among isolates in greenhouse inoculation and culture filtrate assays. The identification of a major genotype among the F. virguliforme isolates from Iowa and Minnesota suggests that a single genotype may have been introduced into the region, and that minor genotypes may have arisen from mutations.
RAPD and microsatellite markers have been successfully used to detect intraspecific genetic variations in other fungi (Wingfield et al., Citation1996; Doherty et al., Citation2003; Harrington et al., Citation2003; Engelbrecht et al., Citation2007). An earlier study of genetic variability in F. virguliforme using RAPDs identified only two genotypes among 55 isolates from nine states, including one isolate from Iowa (Achenbach et al., Citation1997). One of the genotypes was represented by only five isolates, including the reference isolate Mont 1 used in our study. The RAPD markers used in the present study produced the most polymorphisms, with a total of 13 genotypes identified among the 72 isolates of F. virguliforme analyzed, while the (CAT)5 probe generated a total of eight genotypes. Despite being more conservative than the RAPD markers, the (CAT)5 probe placed the 10 isolates from Minnesota into three genotypes, although they all belonged to the same genotype based on the RAPD analysis. It is plausible that a larger sample from Minnesota might show a more varied population than could be detected with the relatively small number of isolates analyzed.
Both the separate and combined analysis of the RAPD and (CAT)5 datasets identified a major genotype within F. virguliforme. Genotype VIII was found in 12 of the 16 Iowa counties, and was dominant among both older and more recent isolates from Iowa. This genotype was also dominant among the isolates from Minnesota. Genotype VIII may, therefore, have dominated the original population introduced into Iowa in 1993 (Yang & Lundeen, Citation1997), with minor genotypes arising through mutations. In most studies in which genetic markers such as (CAT)5 have been successfully used to distinguish indigenous pathogen populations from introduced ones (Engelbrecht et al., Citation2007), low levels of genetic variation have been attributed to a recent introduction of the pathogen into an area. Often, one or two genotypes are dominant in the introduced populations and a limited number of mutations explain the minor variation among genotypes. For example, low levels of genetic variation centred around two genotypes of differing aggressiveness (race A and race M, respectively) were observed among isolates of the introduced soybean pathogen, Cadophora gregata f. sp. sojae, using the microsatellite probes (CAT)5 and (CAC)5 (Harrington et al., Citation2003). In the case of F. virguliforme, a larger study including isolates from other US states would be needed to conclude if genotype VIII is dominant in other regions and if it might have been the main genotype introduced into the US from South America (Aoki et al., Citation2005).
Although some genetic variation was observed, differences in fingerprint profiles were generally represented by one to three unique fragments, indicating a very closely related population of isolates. The similar population structure and high homogeneity was further suggested by the low (< 50%) bootstrap support value between two of the subgroups and the similar gene diversity values for all subgroups. The low level of genetic variation observed within the F. virguliforme population further suggests a recently introduced pathogen (Engelbrecht et al., Citation2007).
Our study is the first to analyze the relationship between variation in genetic composition and aggressiveness in F. virguliforme. Variation in isolate aggressiveness has been previously reported in F. virguliforme (Lim & Jin, Citation1991; Achenbach et al., Citation1997; Li et al., Citation2000, Citation2009; Aoki et al., Citation2005; Malvick & Bussey, Citation2008), but the genetic background of the isolates was not determined (Lim & Jin, Citation1991; Roy et al., Citation1997; Li et al., Citation2000, Citation2009), or no genetic variation was detected among the isolates studied (Malvick & Bussey, Citation2008). Isolates from Iowa varied greatly in their aggressiveness on two soybean cultivars, as indicated by the ability of some isolates to cause severe root and foliar symptoms and significant reductions in plant growth, while other isolates caused only mild symptoms. However, no association was found between genetic variation in F. virguliforme and variation in isolate aggressiveness as assessed in greenhouse inoculations and by culture filtrate assays. The virulence of the isolates was very similar on the two soybean cultivars, suggesting that the inoculum densities used in both assays were sufficient to cause disease even in the moderately resistant cultivar ‘92M91’. These results raise important questions about soybean cultivar resistance and inoculum thresholds in the field.
Several explanations are possible for the lack of association between isolate aggressiveness and genetic markers. First, the genetic markers used may not be linked to aggressiveness traits, as has been reported in other fungal pathogens (Mishra et al., Citation2003; Trouillas & Gubler, Citation2010). According to Pariaud et al. (Citation2009), aggressiveness is determined by the combination of numerous quantitative traits, and there can be significant differences in aggressiveness among isolates that belong to the same genetic group as defined by neutral markers. In a study on Ceratocystis fimbriata (Harrington et al., Citation2011), for example, a large variation in aggressiveness was found within and among pathogen populations from different hosts, but aggressiveness was not correlated with genetic markers. They also reported nearly as much variation in aggressiveness within lineages and among lineages of C. fimbriata on some hosts (Harrington et al., Citation2011). Similarly, variation in F. virguliforme aggressiveness was not associated with genetic markers in our study, and variation in aggressiveness was greater among isolates than among genotypes.
Secondly, the genetic markers used in our study may have been linked only to a small subset of the minor genes that define aggressiveness, making it difficult to associate a definite phenotype to the genotypes. Aggressiveness components of F. virguliforme include sporulation rate, infection efficiency (Luo et al., Citation1999), ability to colonize the xylem (Navi & Yang, Citation2008) and ability to produce toxins that cause foliar symptoms (Li et al., Citation2009). Since aggressiveness components are defined by quantitative traits, variations in several of these components will occur in natural populations through mutations (Pariaud et al., Citation2009). These mutations may have reduced aggressiveness by affecting any of these components, thereby causing variations within the genotypes as defined by the markers used.
Finally, a large portion of variation in aggressiveness may have resulted from an isolate by environment interaction, which poses a major constraint to the reliability of aggressiveness tests (Pariaud et al., Citation2009). The interaction of the environment on aggressiveness may have been particularly significant in this study since the SDS pathosystem is well-known for its dependence on environmental factors, such as temperature and soil moisture (Scherm & Yang, Citation1996; Roy et al., Citation1997). The lack of correlation between trials of the greenhouse and culture filtrate assays, resulting from the inconsistency in aggressiveness ranking among isolates further illustrates the challenges in phenotyping soybean responses to infection by F. virguliforme (Torto et al., Citation1996; Njiti et al., Citation2001; Hashmi et al., Citation2005). Despite best efforts to incubate plants under similar conditions, greenhouse temperatures ranged from 22 to 30 °C in trial 1 and 20 to 28 °C in trial 2 and natural fluctuations in light intensity also occurred. These variations may have affected pathogen growth and sporulation, and consequently the relative aggressiveness of isolates. The marked difference in final inoculum density in soil between the two trials may also have contributed to the lack of consistent aggressiveness ratings between greenhouse experiments.
In the SDS pathosystem, the environment may affect several components of aggressiveness (Scherm & Yang, Citation1996; Roy et al., Citation1997). Measures of disease severity generally correlated between experimental trials of the greenhouse study, except for root rot severity, which was not well correlated with the other parameters. In related studies (Gongora & Leandro, Citation2007), ratings of root rot before maximum severity was reached were better correlated with foliar severity than when root rot was rated at the end of the experiment. In the present study, the fact that some plants showed significant root rot, but escaped the development of foliar symptoms, demonstrates the complexity of a disease where root rot is caused by pathogen colonization, whereas foliar symptoms result from pathogen toxins translocated to the leaves (Roy et al., Citation1997). It has been proposed that some isolates may be good root colonizers but poor toxin producers (Li et al., Citation2009).
The findings of this study do not support the hypothesis that variation in aggressiveness is associated with intraspecific genetic variation. However, the data suggest that minor quantitative traits that determine aggressiveness vary greatly within the F. virguliforme population, and that genetic fingerprinting markers do not strongly correlate with the measures of aggressiveness. In addition, variation in aggressiveness may result from significant interactions between isolate and the environment. Since aggressiveness in the SDS pathosystem is very difficult to characterize, it is possible that experimental variation may have masked any associations with genotype or subgroup, highlighting the importance of controlling experimental conditions in SDS studies. Continued work to understand the sources of variation in isolate aggressiveness are needed to understand pathogen biology and help increase the reliability of SDS resistance screening assays.
Acknowledgements
This work was supported by funding from the Iowa Soybean Association. We thank Dean Malvick for providing isolates from Minnesota.
References
- Achenbach , L.A. and Patrick , J.A. 1996 . Use of RAPD markers as a diagnostic tool for the identification of Fusarium solani isolates that cause soybean sudden death syndrome . Plant Dis , 80 : 1228 – 1232 .
- Achenbach , L.A. , Patrick , J.A. and Gray , L.E. 1997 . Genetic homogeneity among isolates of Fusarium solani that cause soybean sudden death syndrome . Theor. Appl. Genet , 95 : 474 – 478 .
- Anderson , T.R. and Tenuta , A.U. 1998 . First report of Fusarium solani f. sp. glycines causing sudden death syndrome of soybean in Canada . Plant Dis , 82 : 448
- Aoki , T. , O'donnell , K. and Scandiani , M.M. 2005 . Sudden death syndrome of soybean in South America is caused by four species of Fusarium: Fusarium brasiliense sp. nov., F. cuneirostrum sp. nov . F. tucumaniae, and F. virguliforme. Mycoscience , 46 : 162 – 183 .
- Aoki , T. , O'donnell , K. , Homma , Y. and Lattanzi , A.R. 2003 . Sudden death of soybean is caused by two morphologically and phylogenetically distinct species within the Fusarium solani species complex – F. virguliforme in North America and F. tucumaniae in South America . Mycologia , 95 : 660 – 684 .
- Aoki , T. , Scandiani , M.M. and O'donnell , K. 2011 . Phenotypic, molecular phylogenetic, and pathogenetic characterization of Fusarium crassistipitatum sp. nov., a novel soybean sudden death syndrome pathogen from Argentina and Brazil . Mycoscience , doi: doi 10.1007/s10267-011-0150-3
- Arruda , G.M.T. , Miller , R.N.G. , Ferreira , M.A.S.V. and Cafe-Filho , A.C. 2005 . Morphological and molecular characterization of the sudden-death syndrome pathogen of soybean in Brazil . Plant Pathol , 54 : 53 – 65 .
- Bernstein , E.R. , Atallah , Z.K. , Koval , N.C. , Hudelson , B.D. and Grau , C.R. 2007 . First report of sudden death syndrome of soybean in Wisconsin . Plant Dis , 91 : 1201
- Campbell , C.L. and Madden , L.V. 1990 . Introduction to plant disease epidemiology , New York : John Wiley & Sons .
- Chilvers , M.I. and Brown-Rytlewski , D.E. 2010 . First report and confirmed distribution of soybean sudden death syndrome caused by Fusarium virguliforme in Southern Michigan . Plant Dis , 94 : 164
- Descenzo , R.A. and Harrington , T.C. 1994 . Use of (CAT)5 as a DNA fingerprint probe for fungi . Phytopathology , 84 : 534 – 539 .
- Doherty , K.R. , Zweifel , E.W. , Elde , N.C. , Mckone , M.J. and Zweifel , S.G. 2003 . Random amplified polymorphic DNA markers reveal variation in the symbiotic fungus of leaf-cutting ants . Mycologia , 95 : 19 – 23 .
- Engelbrecht , C.J.B. , Harrington , T.C. , Alfenas , A.C. and Suarez , C. 2007 . Genetic variation in populations of the cacao wilt pathogen . Ceratocystis cacaofunesta. Plant Pathol , 56 : 923 – 933 .
- Gongora , C. and Leandro , L. 2007 . Effect of inoculum level of Fusarium virguliforme on timing of foliar and root symptom expression of soybean sudden death syndrome . Phytopathology, 97 , : S41
- Harrington , T.C. , Steimel , J. , Workneh , F. and Yang , X.B. 2003 . Characterization of two races of Phialophora gregata in North-Central United States . Phytopathology , 93 : 901 – 912 .
- Harrington , T.C. , Thorpe , D.J. and Alfenas , A.C. 2011 . Genetic variation in aggressiveness to native and exotic hosts among Brazilian populations of . Ceratocystis fimbriata. Phytopathology , 101 : 555 – 566 .
- Hashmi , R.Y. , Bond , J.P. , Schmidt , M.E. and Klein , J.H. 2005 . A temperature-controlled water bath method for evaluating soybean reaction to sudden death syndrome (SDS) . Plant Health Progress , doi: doi 10.1094/PHP-2005-0906-01-RS
- Hirrel , M.C. 1983 . Sudden death syndrome of soybean – a disease of unknown etiology . Phytopathology , 77 ( Suppl ) : 1689
- Kurle , J.E. , Gould , S.L. and Lewandowski , S.M. 2003 . First report of sudden death syndrome (Fusarium solani f. sp. glycines) of soybean in Minnesota . Plant Dis , 87 : 449
- Li , S. , Hartman , G.L. and Chen , Y. 2009 . Evaluation of aggressiveness of Fusarium virguliforme isolates that cause soybean sudden death syndrome . J. Plant Pathol , 91 : 77 – 86 .
- Li , S. , Hartman , G.L. and Widhom , J.M. 1999 . Viability staining of soybean suspension-cultured cells and seedling stem cutting assay to evaluate phytotoxicity of Fusarium solani f. sp. glycines culture filtrates . Plant Cell Reports , 18 : 375 – 380 .
- Li , S. , Hartman , G.L. , Domier , L.L. and Boykin , D. 2008 . Quantification of Fusarium solani f. sp. glycines isolates in soybean roots by colony-forming unit assays and real-time quantitative PCR . Theor. Appl. Genet , 117 : 343 – 352 .
- Li , S , Tam , Y.K. and Hartman , G.L. 2000 . Molecular differentiation of Fusarium solani f. sp. glycines from other F. solani based on mitochondrial small subunit rDNA sequences . Phytopathology , 90 : 491 – 497 .
- Lim , S.M. and Jin , H. 1991 . Pathogenic variability in Fusarium solani isolated from soybeans with sudden death syndrome symptoms . Phytopathology, 81 , : S1236
- Luo , Y. , Myers , O. , Lightfoot , D.A. and Schmidt , M.E. 1999 . Root colonization of soybean cultivars in the field by Fusarium solani f. sp . glycines. Plant Dis , 83 : 1155 – 1159 .
- Malvick , D.K. and Bussey , K.E. 2008 . Comparative analysis and characterization of the soybean sudden death syndrome pathogen Fusarium virguliforme in the northern United States . Can. J. Plant Pathol , 30 : 467 – 476 .
- Mansouri , S. 2009 . Chromosomal polymorphisms in Fusarium virguliforme, the causal agent of sudden death syndrome in soybean . Phytopathology , 99 : S79
- Mcdonald , B.A. 1997 . The population genetics of fungi: tools and techniques . Phytopathology , 87 : 448 – 453 .
- Mishra , P.K. , Fox , R.T.V. and Culham , A. 2003 . Inter-simple sequence repeat and aggressiveness analyses revealed high genetic diversity, recombination and long-range dispersal in Fusarium culmorum . Ann. Appl. Biol , 143 : 291 – 301 .
- Munkvold , G.P. and O'mara , J.K. 2002 . Laboratory and growth chamber evaluation of fungicidal seed treatments for maize seedling blight caused by Fusarium species . Plant Dis , 86 : 143 – 150 .
- Navi , S.S. and Yang , X.B. 2008 . Foliar symptom expression in association with early infection and xylem colonization by Fusarium virguliforme (formerly F. solani f. sp. glycines), the causal agent of soybean sudden death syndrome . Plant Health Progress , doi: doi 10.1094/PHP-2008-0222-01-RS
- Njiti , V.N. , Johnson , J.E. , Torto , T.A. , Gray , L.E. and Lightfoot , D.A. 2001 . Inoculum rate influences selection for field resistance to soybean sudden death syndrome in the greenhouse . Crop Sci , 41 : 1726 – 1731 .
- O'donnell , K. 1992 . Ribosomal DNA internal transcribed spacers are highly divergent in the phytopathogenic ascomycete Fusarium sambucinum (Gibberella pulicaris) . Current Genet , 22 : 213 – 220 .
- O'donnell , K. and Gray , L.E. 1995 . Phylogenetic relationships of the soybean sudden death syndrome pathogen Fusarium solani f. sp. phaseoli inferred from rDNA sequence data and PCR primers for its identification . Mol. Plant-Microbe Interact , 8 : 709 – 716 .
- O'donnell , K. , Sink , S. , Scandiani , M.M. , Luque , A. , Colletto , A. Biasoli , M. 2010 . Soybean sudden death syndrome species diversity within North and South America revealed by multilocus genotyping . Phytopathology , 100 : 58 – 71 .
- Pariaud , B. , Ravigne , V. , Halkett , F. , Goyeau , H. , Carlier , J. and Lannou , C. 2009 . Aggressiveness and its role in the adaptation of plant pathogens . Plant Pathol , 58 : 409 – 424 .
- Peros , J.-P. and Berger , G. 2003 . Genetic structure and variation in aggressiveness in European and Australian populations of the grapevine dieback fungus . Eutypa lata. Eur. J. Plant Pathol. , 109 : 909 – 919 .
- Ploper , L.D. 1993 . Síndrome de la muerte súbita: Nueva enfermedad de la soja en el noroeste argentino . Avance Agroindustrial , 54 : 5 – 9 .
- Ploper , L.D. , Yorinori , J.T. and Morel , P.W. August 26–29 2003 . “ Importancia de las enfermedades de la soja en el Mercosur (in Spanish) ” . In Actas Simposio Internacional sobre Soja, XI Congreso de AAPRESID , Edited by: Tomo , II . August 26–29 , 163 – 174 . Rosario : Argentina .
- Roy , K.W. , Hershman , D.E. , Rupe , J.C. and Abney , T.S. 1997 . Sudden death syndrome of soybean . Plant Dis , 81 : 1100 – 1111 .
- Roy , K.W. , Lawrence , G.W. , Hodges , H.H. , Mclean , K.S. and Kilelbrew , J.F. 1989 . Sudden-death syndrome of soybean: Fusarium solani as incitant and relation of heterodera glycines to disease severity . Phytopathology , 79 : 191 – 197 .
- Rupe , J.C. , Correll , J.C. , Guerber , J.C. , Becton , C.M. , Gbur , E.E. , Cummings , M.S. Jr. and Yount , P.A. 2001 . Differentiation of the sudden death syndrome pathogen of soybean, Fusarium solani f. sp. glycines, from other isolates of F. solani based on mitochondrial DNA restriction fragment length polymorphisms . Can. J. Bot , 79 : 829 – 835 .
- Rupe , J.C. , Hirrel , M.C. and Hershman , D.E. 1989 . “ Sudden death syndrome ” . In Compendium of soybean diseases , Edited by: Sinclair , J.B. and Backman , P.A. 84 – 85 . St. Paul , MN : American Phytopathological Society .
- Rupe , J.C. , Robbins , R.T. and Gbur , E.E. JR. 1997 . Effect of crop rotation on soil densities of Fusarium solani and Heterodera glycines and on development of sudden death syndrome of soybean . Crop Prot , 18 : 575 – 580 .
- Scherm , H. and Yang , X.B. 1996 . Development of sudden death syndrome of soybean in relation to soil temperature and soil water matric potential . Phytopathology , 86 : 642 – 649 .
- Scherm , H. , Yang , X.B. and Lundeen , P. 1998 . Soil variables associated with sudden death syndrome in soybean fields in Iowa . Plant Dis , 82 : 1152 – 1157 .
- Schmidt, C., Shenaut, M., & Bond, J. (2009). Soybean sudden death syndrome commercial variety test results. http://soybeandiseases.info/ (http://soybeandiseases.info/) (Accessed: 25 October 2011 ).
- Schmidt, C., Shenaut, M., Herzog, C., Clark, D., & Bond, J. (2008). Soybean sudden death syndrome commercial variety test results. http://soybeandiseases.info/ (http://soybeandiseases.info/) (Accessed: 25 October 2011 ).
- Sneath , P.H.A. and Sokal , R.R. 1973 . Numerical taxonomy , San Francisco , CA : W.H. Freeman .
- Taylor , J.W. , Jacobsen , D.J. and Fisher , M.C. 1999 . The evolution of asexual fungi: reproduction, speciation and classification . Annu. Rev. Phytopathol , 37 : 197 – 246 .
- Torto , T.A. , Njiti , V. and Lightfoot , D.A. 1996 . Loci underlying resistance to sudden death syndrome and Fusarium solani in field and greenhouse assays do not correspond . Soybean Genet. Newsl. , 23 : 163 – 166 .
- Trouillas , F.P. and Gubler , W.D. 2010 . Host range, biological variation, and phylogenetic diversity of Eutypa lata in California . Phytopathology , 100 : 1048 – 1056 .
- Wilcox , J.R. , Roach , M.T. and Abney , T.S. 1989 . Registration of ‘Spencer’ soybean . Crop Sci. , 29 : 830 – 831 .
- Wingfield , B.D. , Harrington , T.C. and Steimel , J. 1996 . A simple method for detection of mitochondrial DNA polymorphisms . Fung. Genet. Newsl , 43 : 56 – 60 .
- Wrather , J.A. and Koenning , S.R. 2009 . Effects of disease on soybean yields in the United States 1996 to 2007 . Plant Health Progr. , doi: doi 10.1094/PHP-2009-0401-01-RS
- Yang , X.B. and Lundeen , P. 1997 . Occurrence and distribution of soybean sudden death syndrome in Iowa . Plant Dis , 81 : 719 – 722 .
- Yeh , F.C. , Rongcai , Y. and Boyle , T. 1999 . PopGene version 1.31 , Alberta , , Canada : University of Alberta .
- Yorinori , J.T. 1999 . “ Situação atual das enfermidades de soja na Bolivia, Brasil e Paraguai (in Portuguese) ” . In Proceedings Mercosoja 99, 21–25 June 1999, Rosario, Argentina 40 – 43 .
- Yorinori , J.T. , Charchar , M.J.A. , Naser , L.C.B. and Henning , A.A. 1993 . “ Doenças da soja e seu controle ” . In Cultura da Soja nos Cerrados Edited by: Arantes , N.E. and Souza , P.I.M. 333 – 397 . Potafos, Piracicaba, SP , , Brazil