Abstract
Fusarium head blight (FHB) is a devastating disease of wheat (Triticum aestivum L.). This study investigated a ‘Frontana/Remus’ doubled haploid population (n = 210 lines) to map and validate the ‘Frontana’ resistance quantitative trait loci (QTL) focusing on Fusarium-damaged kernels (FDK). The plant material was evaluated in six epidemic situations for Fusarium resistance in inoculated field experiments, with either Fusarium graminearum or F. culmorum. Other studies have focused on FHB QTL, but it is important to evaluate how far these QTL determine FDK values. The data show that the genetic regulation of FHB resistance is more complex than earlier proposed. FHB resistance QTL were identified on chromosomes 3A, 4A and 6B. Markers showed association with FDK resistance on chromosomes 3D and at the marker Xs12m15_4. QTL on 2B, 4B, 5A and 7B chromosomes were responsible for both FHB and FDK resistance; in this case, the same QTL influenced both traits and possibly other traits during disease development. These QTL are very important, because they can be considered to be real Fusarium resistance QTL. The use of markers in breeding programmes, which are associated only with FHB or FDK resistance may be questionable and require further research. Heading date QTL were detected on chromosomes 1A, 2D and 7B overlapping with neither FHB nor FDK resistance QTL. The QTL identified in the ‘Frontana/Remus’ population were in good agreement with earlier results from the literature.
Résumé
La fusariose des panicules (FP) est une maladie dévastatrice qui atteint le blé (Triticum aestivum L). Cette étude a été porté sur une population haploïde doublée, le ‘Frontana/Remus’ (n = 210 lignées), afin d'organiser et valider les loci des traits qualitatifs (LTQ) de résistance du ‘Frontana’ en se focalisant sur les grains fusariés (GF). La matière végétale a été évaluée pour sa résistance à la fusariose dans six situations épidémiques au moyen d'expériences d'inoculation sur le terrain, soit avec de la Fusarium graminearum ou de la F. culmorum. D'autres études sont axées sur le FP LTQ, mais cela est important d'évaluer comment ces LTQ peuvent déterminer la quantité les GF. Les données montrent que la régulation génétique de la résistance à la FP est plus complexe qu'on a le proposé anvant. Des FP LTQ ont été identifiés sur les chromosomes 3A, 4A et 6B. Les marqueurs montrent un lien de résistance aux GF au niveau de chromosome 3D et de la marqueur Xs12m15_4. Les LTQ sur les chromosomes 2B, 4B, 5A et 7B se trouvent être responsables de résistance à la FP et aux GF, dans ce cas, le même LTQ influe les deux traits de la même manière et vraisemblablement les autres traits au cours de l'évolution de la maladie. A notre niveau, ces LTQ sont très importants parce que pouvant être considères comme de vrais LTQ de résistance à la fusariose. L'utilisation des marqueurs dans les programmes d'amélioration qui sont associées uniquement de résistance à la FP ou aux GF est un procédé douteux et mérite une recherche approfondie. Les LTQ de la date d'épiaison ont été détectés au niveau des chromosomes 1A, 2D et 7B, étant à cheval ni avec les LTQ de resistance à la FP ou aux GF. Les LTQ identifiés dans la population ‘Frontana/Remus’ correspondent aux résultats enregistrés par les études antérieures.
Introduction
Fusarium head blight (FHB) is one of the most important cereal diseases causing concerns for food and feed safety, yield and quality. The use of resistant cultivars, together with proper crop management practices, are the most effective strategies to control FHB (Bai & Shaner, Citation1994; McMullen et al., Citation1997). However, progress in disease control has been much slower than initially supposed 15–20 years ago (Brown-Guedira et al., Citation2008). Identifying effective resistant stocks and understanding the complex genetic structure of FHB resistance would enhance effective breeding for this trait. Buerstmayr et al. (Citation2009) summarized 22 quantitative trait loci (QTL) for FHB resistance, mapped to all wheat chromosomes – except 7D – in different mapping populations. The most important QTL have been found on chromosomes 2D, 3BS, 3BSc, 4B, 5AS and 6BS (McCartney et al., Citation2004; Yu et al., Citation2006; Liu et al., Citation2007), and have been validated in independent mapping populations.
The best-characterized QTL are Fhb1 on chromosome 3BS and Fhb2 on chromosome 6B, both coding Type 2 resistance, and both derived from the highly resistant ‘Sumai 3’ or its close relatives (Waldron et al., Citation1999; Anderson et al., Citation2001; Buerstmayr et al., Citation2002, Citation2003; Somers et al., Citation2003; Lemmens et al., Citation2005; Cuthbert et al., Citation2006). Their intensive use in breeding did not produce the expected results, as the wheat lines inherited many deleterious traits from the adapted germplasm; only sporadic success was recorded in variety development.
The moderately resistant Brazilian spring wheat cultivar ‘Frontana’ originates from the cross of ‘Fronteira/Mentana’ (Schroeder & Christensen, Citation1963; Van Ginkel et al., Citation1996). The genetic background of its FHB resistance was analysed by several research groups. Singh et al. (Citation1995) declared that the resistance of ‘Frontana’ is controlled by the additive interaction of a minimum of three minor genes. Steiner et al. (Citation2004) investigated the ‘Frontana/Remus’ DH population (210 lines) and found Type I Fusarium resistance QTL on chromosomes 3A, 5A, 2B, 4B and 6B in ‘Frontana’, and on chromosomes 1B, 2A and 3B in ‘Remus’. Mardi et al. (Citation2006) confirmed the 3AL QTL of ‘Frontana’ in the ‘Frontana/Falat’ population and identified two additional QTL on chromosomes 1BL and 7AS. Srinivasachary et al. (Citation2008) found ‘Frontana’ FHB resistance QTL on chromosomes 1B, 2B, 3A, 6A, 6B, 7A and 7D in a RIL population from a cross between RL4137 (FHB-resistant line derived from ‘Frontana’) and the moderately FHB-resistant variety ‘Timgalen’. Beyond these, QTL for plant height on 2B, 4A and 5B, as well as for ‘awns’ on 2B were identified. These authors (Srinivasachary et al., Citation2008) analysed only FHB symptoms, while other traits of Fusarium resistance were not considered. The moderate resistance of ‘Frontana’ was confirmed also by Burlakoti et al. (Citation2010) who compared the genetic background of ‘Alsen’ (developed from ‘Sumai 3’), ‘Frontana’ and the susceptible W9207 line. Notably, ‘Frontana’ showed the highest level of resistance to initial infection among the three parents. ‘Alsen’ showed the highest level of resistance with respect to FHB spread, Fusarium-damaged kernels (FDK) and deoxynivalenol (DON) content. This result may be explained by the differences in mechanism of Type I resistance (initial infection) and Type II (FHB spread) resistance and the different effectiveness of the QTL. Yang et al. (Citation2006) stated that ‘Frontana’ does not have QTL in common with ‘Sumai 3’ on chromosomes 3B and 6B, which confirms the importance of ‘Frontana’ as a source of resistance.
FHB severity is influenced by different morphological traits, such as plant height and heading date (Mesterhazy, 1987, 1995; Parry et al., Citation1995). In order to avoid false QTL detection, it is essential to screen wheat lines in different environments. This has been confirmed by Draeger et al. (Citation2007) through the identification of QTL for FHB resistance in the winter wheat variety ‘Arina’ and by Lu et al. (Citation2011) through the investigation of the effect of Rht-D1b plant height allele compared with FHB resistance QTL in a DH population in three countries, across six years. In spite of all the published studies, during phenotyping mapping populations, usually just one F. graminearum and/or F. culmorum isolate/inoculum (when mixed) was used in 2–3 study years (Mardi et al., Citation2005, Citation2006; Chen et al., Citation2007; Klahr et al., Citation2007), meaning that just 2–3 epidemic situations gave the phenotypic data, which might not be enough to detect real or medium effective QTL and reduce the genotype-by-environment interaction.
With the low number of epidemic situations – especially under field conditions – the resistance data are less reliable than with a higher number of environments, and might lead to invalid QTL analyses. This is the key activity since the molecular genetic work with current laboratory instrumentation can be easily standardized, while this is not the case for the phenotyping of mapping populations, especially under field conditions (Szabo-Hever et al., Citation2008). In this study, the number of epidemic situations was increased by increasing the number of different aggressive isolates in order to obtain more reliable data in resistance phenotyping and to obtain less interaction with the environment.
FHB resistance is a complex trait as it is controlled by several small, medium or large effective QTL. Marker-assisted selection (MAS) for FHB resistance already exists. In recent years, screening for FHB resistance (Fhb1 and Fhb2) has become particularly important (Gupta et al., Citation2010). Salameh et al. (Citation2010) and von der Ohe et al. (Citation2010) investigated the effect of Qfhs.ifa-5A compared with the effect of Fhb1 on FHB resistance. Among others, their results showed that Qfhs.ifa-5A has a smaller effect on FHB resistance than Fhb1 or both QTL together. Beyond this, no systematic negative effect of the spring wheat-derived QTL on grain yield, thousand grain weight, hectolitre weight and protein content was found (Salameh et al., Citation2010). Wilde et al. (Citation2007) investigated the effect of the 3B and 5A QTL from CM82036 (‘Sumai 3/Thornbird’) and the 3A QTL from ‘Frontana’. In their experiment, plants carrying 3B, 5A and 3A QTL showed the highest level of FHB severity resistance and DON reduction compared with those carrying only the 3B and 5A QTL; however, the phenotypic selection seemed to be the most efficient method in the resistance tests.
The QTL with small and medium effects are most frequently not validated which is a problem in MAS. There are no publications identifying ‘Frontana’-derived FDK resistance QTL, which is important, because FDK has a stronger association with toxin accumulation than the visual scores of incidence or severity (Mesterházy, Citation1995).
The objectives of this study were: (i) to compare the QTL data with the Austrian results using a different inoculation method from that used by Steiner et al. (Citation2004) in Tulln; (ii) to identify FDK QTL in the ‘Frontana/Remus’ population and compare them with the QTL for FHB severity; (iii) to identify QTL which are not Fusarium resistance QTL, but may influence disease development; and (iv) to estimate the practicability of MAS when ‘Frontana’ is used as a donor of FHB resistance.
Materials and methods
Plant materials
The ‘Frontana/Remus’ doubled haploid (DH) population was generated at IFA-Tulln, Austria and was mapped for QTL by Steiner et al. (Citation2004). The population was developed from the cross of ‘Frontana’ by ‘Remus’ and consists of 210 DH lines. ‘Frontana’ (‘Fronteira/Mentana’) is a Brazilian spring wheat cultivar known for its resistance to FHB. ‘Remus’ is a spring wheat cultivar released by the Bavarian State Institute for Agronomy in Freising, Germany. It is well adapted for cultivation in Central Europe, but it is susceptible to FHB.
Field experiments for Fusarium resistance evaluation
The plant material was evaluated in the nursery of Cereal Research Ltd. in Szeged (Hungary) over four seasons: 2002, 2004, 2005 and 2006, using individual F. graminearum and F. culmorum isolates. Seed was sown in all experiments in mid-October, using Wintersteiger Plot Spider planter (Wintersteiger GmbH, Ried, Austria), at the usual sowing time of winter wheat. The plots (i.e. genotypes) were planted in two replications in a randomized complete block design to serve later as main plots of the experiments. No winter damage was observed in these experiments. Each replicate consisted of 1.5 m long rows. The width of the main plots was set according to the number of isolates used for inoculation to place one bunch infected per row: 1-row plots were planted in trials inoculated with 1 isolate, 2-row plots for 2 isolates and 4-row plots for 4 isolates.
Inoculum production and inoculation procedure
Fungal suspensions were made in 10 L heat stable glass flasks filled with 9.4 L liquid Czapek-Dox medium (Mesterházy, Citation1977). They were aerated at room temperature for a week. Conidium concentration was determined using a Bürker haemocytometer (MOM, Budapest, Hungary). The aggressiveness of the isolates was tested in Petri dishes according to the method of Mesterházy (Citation1985). Until used, the inocula were stored at 4 °C in 600 L refrigerators (‘Grönland’ from Rostock, East Germany). Each genotype was inoculated individually with one to four isolates of either F. graminearum or F. culmorum. The details of the inocula of isolates used are shown in . In the experiments altogether, eight isolates were used; however, two isolates in 2006 were not pathogenic enough, so they were omitted from further analysis. The remaining six epidemic situations are still far more than the 2–3 generally applied in the literature.
Table 1. Isolate names and number of conidia ml− 1 used in the field screening in each experiment and the results of the Petri dish test (mean percentage of infected germs of five readings) according to the method of Mesterházy (Citation1985)
At full flowering (Feekes growth stage 10.5.1), bunches of 15–25 spikes were sprayed from all sides with a hand-held-sprayer to inoculate the spikes uniformly, using about 15–20 mL fungal suspension for each sample as described by Mesterházy (Citation1987, 1995). One bunch of spikes per row of each genotype (in a randomized complete block design) was inoculated with a single isolate. Arrangement was combined with the use of isolates that were not randomized within plots, but 30–40 cm was kept between bunches. After inoculation, bunches were covered with a transparent polyethylene bag for 48 hours. After removing the bags, the plants were loosely bound with a label for identification at half plant height to allow the leaves to photosynthesize freely.
FHB and FDK assessment
Observations of FHB disease severity were started on the 10th day after inoculation and were repeated on every fourth day. FHB evaluations were made on a 0–100 linear scale (% of spikelets infected = severity) as long as the control heads were green; this meant four readings per season.
The groups of inoculated heads were harvested manually and stored until threshing in paper bags. The samples were threshed using a stationary thresher (Wintersteiger LD 180, Ried, Austria) at low wind, in order to retain the shrivelled, low thousand kernel weight (TKW) Fusarium-damaged kernels. Chaff was removed using an Ets Plaut-Aubry (41290 Conan-Oucques, France) air separator. After cleaning, FDK were visually rated (visual estimation of scabby grains with definite tombstone, or chalky-white and rose discoloration as a percentage). Shrivelled, but non-discolored grains were not considered Fusarium-damaged; these originated mostly from withered spikelets above the infection point due to wilting (Lehoczki-Krsjak et al., Citation2010).
Other traits
In each year, plant height was measured as the distance from the soil surface to the top of heads excluding awns. The date of heading and anthesis were also recorded as the number of days from 1 January to heading or anthesis.
Genomic DNA extraction
DNA was isolated from seedling leaf tissue according to the CTAB method (Rogers & Bendich, Citation1985). The quality and quantity were measured with NanoDrop 1000 Spectrophotometer (Thermo Scientific Company).
Molecular markers
The 583 marker data, comprising 135 microsatellite markers, 416 AFLP, 32 RFLP markers and the map information of the ‘Frontana/Remus’ population were provided by Steiner et al. (Citation2004). Forty-five SSR markers were tested on the population at Cereal Research Ltd. (Hungary). Most of the primers used in Hungary were selected from the literature (Röder et al., Citation1998; Somers et al., Citation2004; Mardi et al., Citation2006). The PCR reaction mix for SSR markers contained: 0.12 μM forward primer, 0.12 μM reverse primer, 1× PCR Master Mix (Fermentas) and 20 ng template DNA for 10 μl reaction volume per sample. The PCR programme for SSR primers was: 94 °C for 8 min and then 40 cycles of 94 °C for 5 s, 50–60 °C for 5 s, 72 °C for 8 s followed by 72 °C for 5 min. The PCRs were performed on an Eppendorf Mastercycler®ep 96-well thermocycler. The PCR products were separated on 5% polyacrylamide gels using a Sequi-Gen GT Sequencing Cell 30 cm gel apparatus (Bio-Rad) or with the Agilent 2100 Bioanalyzer (Agilent Technologies).
Statistical analysis
Statistical analyses were made by SPSS 15.0 software ‘Descriptive statistics’ function to calculate means, minimum and maximum values, percentiles, standard deviation, to test the normal distribution of the FHB severity and FDK data. The ‘General Linear Model’ (GLM) function was used to perform the two-way ANOVA, investigating the genotype-by-experiment interactions. The FHB severity and FDK data for each fungal isolate from different years were analysed as single experiments (epidemic situations), because there are no races within F. graminearum and host resistance is non-specific for different Fusarium spp. (Mesterházy, Citation1995, Citation2002). Therefore the epidemics caused by either F. graminearum or F. culmorum could be analysed together. The data for all epidemic situations were checked for normal distribution (Weber, Citation1972). Broad-sense heritability was estimated across experiments according to Nyquist (Citation1991) with the following formulas: H2 across experiments = 1−(MSGxE/MSG). (MSGxE: mean square genotype × experiment; MSG: mean square genotype).
QTL analysis
Linkage groups were constructed using JoinMap® 3.0 (Van Ooijen & Voorrips, Citation2001) and interval mapping was done using MapQTL® 5 (Van Ooijen, Citation2004). Linkage groups were established by using a maximum recombination fraction of 0.45. The permutation tests (determined by 1000 iterations) indicated minimum LOD scores between 1.2 and 2.1 at a P = 0.05 significance level. Interval mapping (IM) was made with the phenotypic data of single experiments, with the mean values of F. graminearum and F. culmorum inoculations and overall means for FHB severity and FDK rates of all the selected isolates, as well as with lodging, plant height and heading date.
Results
Fusarium resistance
The means of the parental lines and the DH lines, the population ranges and standard deviation for FHB severity and FDK rate reported by isolates and across the different environments is shown in . In the experiments, the FHB-resistant parent ‘Frontana’ and the susceptible ‘Remus’ showed Fusarium infection symptoms according to their resistance level. Nevertheless, the isolates and the different environmental conditions among years caused differences in FHB severity and FDK rate means between the parental and the DH lines. The distribution of FHB severity and FDK rate means of the selected experiments is presented in and
Table 2. Means, ranges and standard deviations for fusarium head blight (FHB) severity and Fusariu m-damaged kernels (FDK) rate caused by isolates of Fusarium graminearum and Fusarium culmorum over six environments (experiments) in a ‘Frontana/Remus’ population. Plant height (cm) and heading date (days) are mean values from the investigated experiments
Fig. 1. Histogram of fusarium head blight (FHB) means of 210 doubled-haploid lines in a ‘Frontana/Remus’ population over six environments.
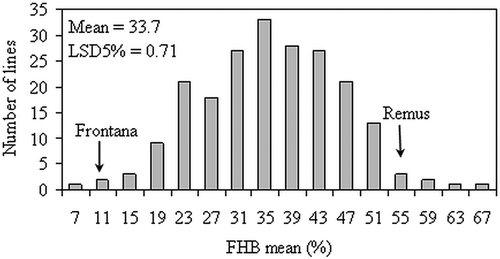
Fig. 2. Histogram of Fusarium-damaged kernels (FDK) levels of 210 doubled-haploid lines in a ‘Frontana/Remus’ population over six environments.
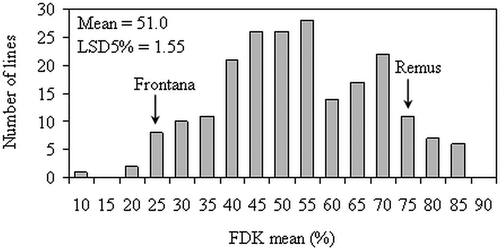
The large differences for FHB severity and FDK rate between parental genotypes accounted for significant genetic variation in the mapping populations. ANOVA was calculated for FHB severity and FDK rate across the six epidemic situations (). The FDK data were usually significantly more severe than FHB severity. The genotype effects differ significantly from the genotype-by-experiment interaction, indicating that the basic ranking is similar. The heritability estimates (0.64 for FHB and 0.74 for FDK) indicate a good reproducibility of the results among tests for both traits.
Table 3. Analysis of variance for fusarium head blight (FHB) severity and levels of Fusariu m-damaged kernels (FDK)
Correlations were calculated among each experiment and means for the two Fusarium species, means for FHB severity, FDK levels, lodging, plant height and heading date across the experiments (). The correlations were somewhat closer for FDK values, indicating a better reproducibility. Means, calculated for the two Fusarium species used in the experiments, showed good correlation within FHB or FDK, while only moderate correlation was detected among the two traits. The correlations between individual experiments to the F. culmorum means were closer than those of the F. graminearum means which can be explained by the different number of epidemic situations used for calculating means for the two species. Plant height and lodging did not seriously influence FHB data (in dry seasons, lodging was rare); however, a later heading date increased FHB values through the different weather conditions during the initial fungal growth. Lodging and plant height influenced FDK values at a low level; however, the later heading genotypes decreased FDK severity. Lodging was moderately correlated with plant height, and heading date was later for taller genotypes.
Table 4. Correlations between fusarium head blight (FHB) severity, Fusarium-damaged kernels (FDK) levels in each experiment (1–6), with mean values, lodging, plant height and heading date (n = 210). (** = correlation is significant at the 0.01 level; * = correlation is significant at the 0.05 level)
Molecular linkage map
In the linkage analysis, 583 markers were mapped to 44 linkage groups, covering a genetic distance of 1643 cM. Partial maps were obtained for all wheat chromosomes. The QTL analyses were made with the means across epidemic situations (experiments) and with each situation separately. The latter is not shown, as the use of means across experiments did not significantly change the results. All the QTL regions detected on the different chromosomes in the different experiments are shown in . There was no case where a QTL would be significant in all epidemic situations. However, the QTL analyses gave significantly better results with mean data (). With some exceptions, the F. culmorum gave higher LOD values than F. graminearum, which can be explained by the lower number of epidemic situations with this latter Fusarium species. The genetic map of chromosomes containing marker intervals linked to FHB and/or FDK resistance is shown in
Fig. 3. Genetic map of QTL associated with fusarium head blight severity (FHB, dashed lines) and/or Fusarium-damaged kernels levels (FDK, dotted lines) over six experiments. Solid lines are the significant LOD level of a given linkage group.
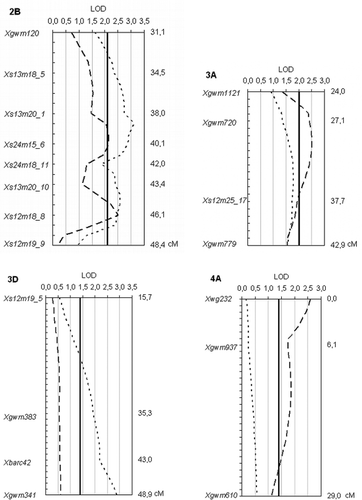
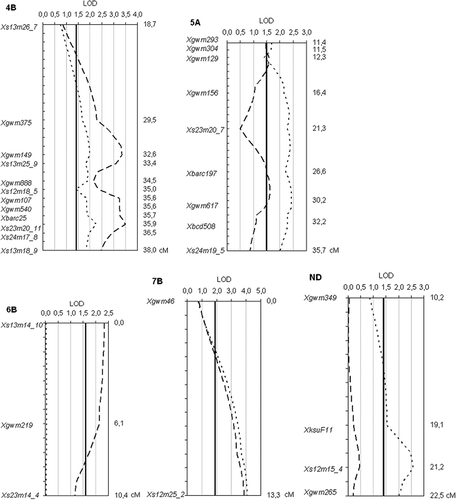
Table 5. Locations of the QTL detected and chromosome regions with LOD values for fusarium head blight (FHB) severity and Fusariu m-damaged kernels (FDK) levels in each experiment. The highlighted values are significant according to the genome-wide permutation tests
Table 6. Locations of the QTL detected and chromosome regions with LOD values, percentage of explained phenotypic variance (VE) for fusarium head blight (FHB) severity, Fusarium-damaged kernels (FDK) levels () and heading date (HD) (). The highlighted values mean LOD > 2.0, others are significant according to the genome-wide permutation tests
FDK : Chromosome regions on 2B (Xgwm120-Xs12m19_9 and Xgwm526), 3D (Xs12m19_5-Xgwm341), 4B (Xs13m26_7-Xs13m18_9), 5A (Xgwm293-Xs24m19_5), 7B (Xs12m25_2) and one unassigned linkage group (Xs12m15_4) were significant for FDK as a measure for resistance. A QTL on chromosome 7B (Xs12m25_2) showed the highest LOD value (4.05), accounted for 10.9% of the phenotypic variance (r2 or VE) and was found in the same position as the QTL for FHB severity. Other QTL coincidental with FHB resistance were identified on 2B (Xgwm120-Xs12m19 and Xgwm526), 4B (Xs13m26_7-Xs13m18_9) and 5A (Xgwm293-Xs24m19_5).
FHB severity: Interval mapping analysis with MapQTL revealed Fusarium resistance QTL on chromosomes 2B (Xgwm120-Xs12m19_9 and Xgwm526), 3A (Xgwm1121-Xgwm779), 4A (Xwg232), 4B (Xs13m26_7-Xs13m18_9), 5A (Xgwm293-Xs24m19_5), 6B (Xs13m14_10-Xs23m14_4) and 7B (Xs12m25_2), all originated from ‘Frontana’. The highest LOD value (3.88) was measured on chromosome 7B explaining 10.6% of the phenotypic variance (r2 or VE). The chromosome region Xgwm293-Xs24m19_5 on 5A had the smallest, although still significant, effect. Markers did not show the same association with both traits. Five of the 10 QTL influenced significantly both traits (FHB severity and FDK) simultaneously. Three had an effect only on FHB, and another two determined only FDK resistance.
Other traits : Among the other phenotypic traits examined, QTL for heading date were identified on chromosomes 1A (Xs13m14_5a-Xwg983) and 2D (Xs25m19_16-Xgwm608), as well as on 7B (Xgwm46); none overlapped with FHB severity or FDK QTL.
Discussion
The moderately resistant genotypes might have many QTL with small or medium effects. This group has an increasing significance for breeding, because they are mostly adapted and FHB resistance can be improved with their use. The genetic regulation of FHB resistance is more complicated than previously thought. QTL were found that regulated either FHB or FDK and also QTL were found which conferred resistance to both traits. This means that QTL determining only FHB severity do not provide sufficiently reliable information about potential resistance using MAS. Additionally, we do not know how these QTL determining FHB severity will influence DON contamination. The function of the QTL are also not known, and the genes that are behind them are unknown. Since many traits can influence disease development, many of which are not defence-related traits can be identified as FHB QTL that may have nothing to do with the genetic resistance. We think that the more reliable markers are those which determine more resistance-based traits, because in that case, the probability of having real Fusarium resistance QTL is much higher than in the case of markers which link to QTL determining either FHB or FDK. On the other hand, we should develop mapping populations that are morphologically more homogeneous: plant height and heading date differences are smaller, all lines are awned or awnless, the structure of the head is similar, the plant materials where compact and other head types occur are better exposed to unwanted changes and influences. These many uncertainties lead us to conclude that more epidemic situations are necessary to detect real resistance QTL. Better phenotyping is also important. We found that securing humidity with polyethylene bags instead of misting is more neutral to plant height influence. In the artificially inoculated nursery, the different plant height groups had nearly the same FHB values, but their natural infection severities showed large differences according to plant height classes (Mesterházy, Citation1987).
The situation we report in ‘Frontana’ is not unique. Three mapping populations of the Swiss winter wheat ‘Arina’ were analysed with different susceptible parents (Paillard et al., Citation2004; Draeger et al., Citation2007; Semagn et al., Citation2007). Of the many QTL and markers described in these papers, only one was common in the three tests, the Rht-D1 marker on the chromosome 4DS. Draeger et al. (Citation2007) published eight QTL associated with AUDPC, and only one locus (Qfhs.jic 7a) influenced FDK. DON was, however, influenced by two marker regions which also influenced AUDPC (Qfhs.jic-4d and Qfhs.jic.6b). These observations are similar to the results in the ‘Frontana/Remus’ population.
The importance of type I resistance is increasingly recognized, and the testing methodology has slowly developed to investigate it more precisely. It seems also that the combination of Type I and Type II resistance is highly effective. Cultivar ‘Sumai 3’ proves this clearly where the 3BS and 5A QTL come from two different varieties (‘Funo’ and ‘Taiwanxiaomai’). In China, Mexico (CIMMYT), USA and Canada, breeding has focused on Type II resistance. Nowadays, they consider also Type I resistance and there is a general conviction that spray inoculation is suitable to test the total resistance, while the point inoculation tests only Type II; therefore, spray inoculation can be more suitable for breeding purposes (Mesterházy, Citation1995; Miedaner et al., Citation2003). Applying the best Fusarium testing method is very important for QTL mapping and MAS, as effective Fusarium resistance breeding needs both phenotypic selection and MAS (Wilde et al., Citation2007; Kosová et al., Citation2009; Löffler et al., Citation2009). Our observations suggest that the spraying inoculation covers overall FHB resistance and the original description of Type I (response to spraying inoculation) and Type II (response to point inoculation) is not correct. Variants of the spraying inoculation cover all resistance types, not only these two; as well as kernel resistance, DON resistance and tolerance can be tested with these methodological approaches (Mesterházy, Citation1995).
Plant morphological and developmental traits, such as plant height, spike morphology, and early or late flowering, influence FHB infection. Accordingly, it is possible that some of the putative FHB resistance QTL correspond to genes modulating morphological or developmental traits (disease escape) rather than physiological resistance. Therefore, in QTL mapping studies, other plant traits in addition to FHB severity, such as plant height and flowering date, should be measured in order to check for co-location with FHB resistance QTL (Buerstmayr et al., Citation2009).
In spite of the differences in field testing methodology, the inoculation methods and location, the QTL from the experiment in Szeged showed very good agreement with the results of Steiner et al. (Citation2004). They used both spray inoculation and mist irrigation for testing field resistance and single-floret inoculation testing for resistance to fungal spread along the ear. They identified chromosome regions on the 2B (Xs13m25_8-Xs24m15_6), 3A (Xdupw227-Xgwm720), 4B (Xs13m25_9), 5A (Xgwm129-Xbarc197), 6B (Xs23m14_4) chromosomes for FHB severity and/or FHB incidence, plus another region on 2B (Xs25m15_2-Xs24m19_11) for FHB spread, in agreement with our mapping results for FHB severity. The FHB QTL of ‘Remus’ (on chromosomes 1B, 2A and 3B) could not be supported with the Hungarian data as we detected neither FHB nor FDK resistance QTL deriving from ‘Remus’. A comparison of the QTL regions we identified with those of Steiner et al. (Citation2004) is shown in . Occasionally, the flanking markers of certain validated QTL are different, although these QTL regions are essentially the same in both studies.
Table 7. Chromosomes and markers linked to fusarium head blight (FHB) resistance identified in Szeged and Tulln (severity and/or incidence) in the ‘Frontana/Remus’ DH mapping population based on the general means across years
We confirmed the existence of two Fusarium resistance related QTL on the 2B chromosome: one in the region of the Xgwm526, which was also identified by Schmolke et al. (Citation2005), and another at Xgwm120-Xs12m19_9 that were reported by Steiner et al. (Citation2004) and Srinivasachary et al. (Citation2008) too as a ‘Frontana’ FHB severity and plant height QTL.
A homologous region was identified associating with FHB resistance on chromosome 3A (Xgwm1121-Xgwm779) in Hungary as it was in Austria with the same population by Steiner et al. (Citation2004). In other ‘Frontana’-crossed populations, Mardi et al. (Citation2006), Berzonsky et al. (Citation2007), Wilde et al. (Citation2007) and Srinivasachary et al. (Citation2008) also detected the same chromosome region responsible for Fusarium resistance.
For FHB severity and FDK, we detected a QTL on 4B between the markers Xs13m26_7 and Xs13m18_9 as did Steiner et al. (Citation2004), but they found this region was associated also with plant height. FHB resistance QTL at 5A and 6B were also in agreement between the two studies. We found the 5A chromosome region (Xgwm293-Xs24m19_5) responsible for FHB severity and FDK resistance. This region also was identified in the ‘Frontana/Remus’ population by Steiner et al. (Citation2004), who found this region also related to plant height. Gervais et al. (Citation2003) observed that the FHB resistance QTL on the 5A chromosome is independent from the QTL on the 5A for plant height. We could not verify this last assumption in ‘Frontana’ with the Hungarian testing method, as we did not detect any plant height QTL during the analysis.
The 6B QTL between the markers Xs13m14_10-Xs23m14_4 was detected as a FHB severity QTL. This chromosome region was described by several research groups in Asian wheat lines (Waldron et al., Citation1999; Anderson et al., Citation2001; Shen et al., Citation2003; Yang et al., Citation2003; Somers et al., Citation2006). The QTL we identified does not seem to be related to fhb2 on the 6B chromosome. This view was supported by Yang et al. (Citation2006) who stated that ‘Frontana’ does not have homologous QTL with ‘Sumai 3’ on the 6B chromosome.
In the Szeged analysis, the QTL at the marker Xs12m25_2 on the 7B chromosome had the highest effect on FHB severity and FDK levels. This is the first report of this chromosome region as a ‘Frontana’-derived resistance QTL. Schmolke et al. (Citation2005) identified this region as associated not only with FHB resistance, but also with heading date, a result which may have originated from the heterogeneity problems of a RIL population derived from the cross of the Fusarium-resistant ‘Dream’ and the FHB-susceptible dwarf type ‘Lynx’.
FDK QTL in the ‘Frontana/Remus’ population
In the literature, ‘Frontana’-derived FDK QTL have not yet been reported. In addition to the four FHB resistance QTL on the 3A (Xgwm1121-Xgwm779), 4A (Xwg232) and 6B (Xs13m14_10-Xs23m14_4) regions, four QTL were mapped for FHB severity and also for FDK levels on chromosomes: 2B (Xgwm120-Xs12m19_9 and Xgwm526), 4B (Xs13m26_7-Xs13m18_9), 5A (Xgwm293-Xs24m19_5), 7B (Xs12m25_2) and two additional chromosome regions were identified influencing only FDK rates on 3D (Xs12m19_5-Xgwm341) and one at the marker Xs12m15_4 without chromosome determination.
It might be easier to understand the results of the QTL analysis by following the correlations among the different experiments and different traits. The visual scores are evaluated 3–4 weeks after inoculation, but for harvest 2–3 additional weeks elapse. Heavy rains in this period enhance further disease development that is reflected only in the FDK value. Lodging influences FHB data through plant height as tall genotypes tend to lodge more probably. The effect of lodging on FDK is more frequent because of the time slippage between the evaluations of the two traits. Researchers should be aware, however, that there may be as yet unidentified traits that influence results. Traits that determine physiological resistance should not be identified as FHB QTL. This can be verified only by further research. Difficulties can be eliminated from the analysis of small and medium effective QTL via testing the plant material during at least four or more experimental epidemic situations. It is shown well in our study, where the QTL effects were dissimilar between single (statistically selected) experiments. This can be explained with the different aggressiveness of the isolates and with the phenomena that the phenotypic difference of genotypes in the experiments can be variable, which variation can be balanced by using mean values of different good quality datasets with normal distribution.
QTL linked to heading date
For date of anthesis, Steiner et al. (Citation2004) identified a QTL on 2D, in the same region we detected a QTL for heading date (Xs25m19_16-Xgwm608). This region was mentioned by other research groups as a FHB resistance coding interval of ‘Frontana’ (Mardi et al., Citation2005; Yang et al., Citation2005), but our data do not support this view. However, when the population has a long flowering time (10–14 days), changing weather conditions can cause differences between FHB levels of early or late genotypes and may result in phenotyping errors.
The QTL associated with heading date on chromosomes 1A, 2D and 7B did not overlap with any Fusarium resistance QTL in spite of the significant correlation between the resistance data and the heading date. This confirms the accuracy of the phenotyping method used, as it did not cause false QTL detection.
Regarding the Fusarium resistance QTL originating from ‘Frontana’, the data mostly supported the findings of Steiner et al. (Citation2004). However, with the Hungarian phenotypic data, the presence of QTL on 1B, 2A and 3B derived from ‘Remus’ could not be detected. We concluded therefore that those QTL appeared less stable, and could not be validated from the same population under different environmental conditions. The presence of the 1A and 7A Fusarium resistance QTL of Mardi et al. (Citation2006) could not be confirmed in our mapping population either.
The comparison of the two phenotyping systems of the ‘Frontana/Remus’ population resulted in small differences in the QTL estimates, indicating that both methodologies (used in Tulln or in Szeged) appear to be useful for Fusarium resistance testing and QTL mapping.
Our results indicate the importance of selection not only for FHB, but also for FDK resistance, as we found different genetic backgrounds responsible for the two traits in some cases. It is clear that Fusarium resistance is regulated not only by one or two genes but also by the interaction of several small and moderately effective QTL, which can be associated with other plant morphological traits or environmental factors. It is also important that the phenotypic screening that works well is more effective than MAS alone (Wilde et al., Citation2007) and it will be so in the foreseeable future. The recommended way for Fusarium resistance breeding is to identify and build up the necessary QTL in the parent lines and identify them in the phenotypically selected advanced progenies.
Acknowledgements
This work was supported by the DEAK Co. Ltd., EU-funded FP5 Project FUCOMYR and FP7 project MYCORED.
References
- Anderson , J.A. , Stack , R.W. , Liu , S. , Waldron , B.L. , Fjeld , A.D. Coyne , C. 2001 . DNA markers for fusarium head blight resistance QTLs in two wheat populations . Theor. Appl. Genet. , 102 : 1164 – 1168 .
- Bai , G.H. and Shaner , G.E. 1994 . Scab of wheat: prospects for control . Plant Dis. , 78 : 760 – 766 .
- Berzonsky , W.A. , Gebhard , B.L. , Gamotin , E. , Leach , G.D. and Ali , S. 2007 . A reciprocal backcross monosomic analysis of the scab resistant spring wheat (Triticum aestivum L.) cultivar, ‘Frontana . Plant Breed , 126 : 234 – 239 .
- Brown-Guedira , G. , Griffey , C. , Kolb , F. , McKendry , A. , Murphy , J.P. and Van Sanford , D. 2008 . Breeding Fhb-resistant soft winter wheat: progress and prospects . Cereal Res. Commun , 36 ( Supplementum B ) : 31 – 35 .
- Buerstmayr , H. , Lemmens , M. , Hartl , L. , Doldi , L. , Steiner , B. , Stierschneider , M. and Ruckenbauer , P. 2002 . Molecular mapping of QTLs for fusarium head blight resistance in spring wheat. I. Resistance to fungal spread (type II resistance) . Theor. Appl. Genet. , 104 : 84 – 91 .
- Buerstmayr , H. , Steiner , B. , Hartl , L. , Griesser , M. , Angerer , N. Lengauer , D. 2003 . Molecular mapping of QTLs for fusarium head blight resistance in spring wheat. II. Resistance to fungal penetration and spread . Theor. Appl. Genet. , 107 : 503 – 508 .
- Buerstmayr , H. , Ban , T. and Anderson , J.A. 2009 . QTL mapping and marker-assisted selection for fusarium head blight resistance in wheat: a review . Plant Breed. , 128 : 1 – 26 .
- Burlakoti , R.R. , Mergoum , M. , Kianian , S.F. and Adhikari , T.B. 2010 . Combining different resistance components enhances resistance to fusarium head blight in spring wheat . Euphytica , 172 : 197 – 205 .
- Chen , X.F. , Faris , J.D. , Hu , J.G. , Stack , R.W. , Adhikari , T. Elias , E.M. 2007 . Saturation and comparative mapping of a major fusarium head blight resistance QTL in tetraploid wheat . Mol. Breed. , 19 : 113 – 124 .
- Cuthbert , P.A. , Somers , D.J. , Thomas , J. , Cloutier , S. and Brule-Babel , A. 2006 . Fine mapping Fhb1, a major gene controlling fusarium head blight resistance in bread wheat (Triticum aestivum L.) . Theor. Appl. Genet. , 112 : 1465 – 1472 .
- Draeger , R. , Gosman , N. , Steed , A. , Chandler , E. , Thomsett , M. Srinivasachary . 2007 . Identification of QTLs for resistance to fusarium head blight, DON accumulation and associated traits in the winter wheat variety Arina . Theor. Appl. Genet. , 115 : 617 – 625 .
- Gervais , L. , Dedryver , F. , Morlais , J.Y. , Bodusseau , V. , Negre , S. Bilous , M. 2003 . Mapping of quantitative trait loci for field resistance to fusarium head blight in a European winter wheat . Theor. Appl. Genet. , 106 : 961 – 970 .
- Gupta , P.K. , Langridge , P. and Mir , R.R. 2010 . Marker-assisted wheat breeding: present status and future possibilities . Mol. Breed. , 26 : 145 – 161 .
- Klahr , A. , Zimmermann , G. , Wenzel , G. and Mohler , V. 2007 . Effects of environment, disease progress, plant height and heading date on the detection of QTLs for resistance to fusarium head blight in a European winter wheat cross . Euphytica , 154 : 17 – 28 .
- Kosová , K. , Chrpová , J. and Šíp , V. 2009 . Cereal resistance to fusarium head blight and possibilities of its improvement through breeding . Czech J. Genet Plant Breed. , 45 : 87 – 105 .
- Lehoczki-Krsjak , S. , Szabo-Hever , A. , Toth , B. , Kotai , C. , Bartok , T. Varga , M. 2010 . Prevention of Fusarium mycotoxin contamination by breeding and fungicide application to wheat . Food Addit. Contam. Part a-Chemistry Analysis Control Exposure & Risk Assessment , 27 : 616 – 628 .
- Lemmens , M. , Scholz , U. , Berthiller , F. , Dall'Asta , C. , Koutnik , A. Schuhmacher , R. 2005 . The ability to detoxify the mycotoxin deoxynivalenol colocalizes with a major quantitative trait locus for fusarium head blight resistance in wheat . Mol. Plant-Microbe Inter. , 18 : 1318 – 1324 .
- Liu , S. , Abate , Z.A. , Lu , H. , Musket , T. , Davis , G.L. and McKendry , A.L. 2007 . QTL associated with fusarium head blight resistance in the soft red winter wheat Ernie . Theor. Appl. Genet. , 115 : 417 – 427 .
- Löffler , M. , Schon , C.C. and Miedaner , T. 2009 . Revealing the genetic architecture of FHB resistance in hexaploid wheat (Triticum aestivum L.) by QTL meta-analysis . Mol. Breed. , 23 : 473 – 488 .
- Lu , Q.X. , Szabo-Hever , A. , Bjørnstad, Å., Lillemo , M. , Semagn , K. Mesterhazy , A. 2011 . Two major resistance QTL are required to counteract the increased susceptibility to Fusarium head blight at the Rht-D1b gene in wheat . Crop Sci. , 51 : 2430 – 2438 .
- Mardi , M. , Buerstmayr , H. , Ghareyazie , B. , Lemmens , M. , Mohammadi , S.A. , Nolz , R. and Ruckenbauer , P. 2005 . QTL analysis of resistance to fusarium head blight in wheat using a ‘Wangshuibai’-derived population . Plant Breed. , 124 : 329 – 333 .
- Mardi , M. , Pazouki , L. , Delavar , H. , Kazemi , M.B. , Ghareyazie , B. Steiner , B. 2006 . QTL analysis of resistance to fusarium head blight in wheat using a ‘Frontana’-derived population . Plant Breed. , 125 : 313 – 317 .
- McCartney , C.A. , Somers , D.J. , Fedak , G. and Cao , W. 2004 . Haplotype diversity at fusarium head blight resistance QTLs in wheat . Theor. Appl. Genet. , 109 : 261 – 271 .
- McMullen , M. , Jones , R. and Gallenberg , D. 1997 . Scab of wheat and barley: a re-emerging disease of devastating impact . Plant Dis. , 81 : 1340 – 1348 .
- Mesterházy , Á. 1977 . Reaction of winter wheat varieties to four Fusarium species . Phytopathol. Z. , 90 : 104 – 112 .
- Mesterházy , A. 1985 . Effect of seed production area on the seedling resistance of wheat to fusarium seedling blight . Agronomie , 5 : 491 – 497 .
- Mesterházy , A. 1987 . Selection of head blight resistant wheats through improved seedling resistance . Plant Breed. , 98 : 25 – 36 .
- Mesterházy , A. 1995 . Types and components of resistance to fusarium head blight of wheat . Plant Breed. , 114 : 377 – 386 .
- Mesterházy , A. 2002 . Role of deoxynivalenol in aggressiveness of Fusarium graminearum and F. culmorum and in resistance to fusarium head blight . Eur. J. Plant Pathol. , 108 : 675 – 684 .
- Miedaner , T. , Moldovan , M. and Ittu , M. 2003 . Comparison of spray and point inoculation to assess resistance to fusarium head blight in a multienvironment wheat trial . Phytopathology , 93 : 1068 – 1072 .
- Nyquist , W.E. 1991 . Estimation of heritability and prediction of selection response in plant-populations . Crit. Rev. Plant Sci. , 10 : 235 – 322 .
- Paillard , S. , Schnurbusch , T. , Tiwari , R. , Messmer , M. , Winzeler , M. , Keller , B. and Schachermayr , G. 2004 . QTL analysis of resistance to fusarium head blight in Swiss winter wheat (Triticum aestivum L.) . Theor. Appl. Genet. , 109 : 323 – 332 .
- Parry , D.W. , Jenkinson , P. and McLeod , L. 1995 . Fusarium ear blight (scab) in small grain cereals – a review . Plant Pathol. , 44 : 207 – 238 .
- Röder , M.S. , Korzun , V. , Wendehake , K. , Plaschke , J. , Tixier , M.H. , Leroy , P. and Ganal , M.W. 1998 . A microsatellite map of wheat . Genetics , 149 : 2007 – 2023 .
- Rogers , S.O. and Bendich , A.J. 1985 . Extraction of DNA from milligram amounts of fresh, herbarium and mummified plant-tissues . Plant Mol. Biol. , 5 : 69 – 76 .
- Salameh , A. , Buerstmayr , M. , Steiner , B. , Neumayer , A. , Lemmens , M. and Buerstmayr , H. 2010 . Effects of introgression of two QTL for fusarium head blight resistance from Asian spring wheat by marker-assisted backcrossing into European winter wheat on fusarium head blight resistance, yield and quality traits . Mol. Breed. , 28 : 485 – 494 .
- Schmolke , M. , Zimmermann , G. , Buerstmayr , H. , Schweizer , G. , Miedaner , T. , Korzun , V. and Hartl , L. 2005 . Molecular mapping of fusarium head blight resistance in the winter wheat population Dream/Lynx . Theor. Appl. Genet. , 111 : 747 – 756 .
- Schroeder , H.W. and Christensen , J.J. 1963 . Factors affecting resistance of wheat to scab caused by . Gibberella zeae. Phytopathology , 53 : 831 – 838 .
- Semagn , K. , Skinnes , H. , Bjornstad , A. , Maroy , A.G. and Tarkegne , Y. 2007 . Quantitative trait loci controlling fusarium head blight resistance and low deoxynivalenol content in hexaploid wheat population from ‘Arina’ and NK93604 . Crop Sci. , 47 : 294 – 303 .
- Shen , X. , Zhou , M. , Lu , W. and Ohm , H. 2003 . Detection of fusarium head blight resistance QTL in a wheat population using bulked segregant analysis . Theor. Appl. Genet. , 106 : 1041 – 1047 .
- Singh , R.P. , Ma , H. and Rajaram , S. 1995 . Genetic-analysis of resistance to scab in spring wheat cultivar Frontana . Plant Dis. , 79 : 238 – 240 .
- Somers , D.J. , Fedak , G. and Savard , M. 2003 . Molecular mapping of novel genes controlling fusarium head blight resistance and deoxynivalenol accumulation in spring wheat . Genome , 46 : 555 – 564 .
- Somers , D.J. , Isaac , P. and Edwards , K. 2004 . A high-density microsatellite consensus map for bread wheat (Triticum aestivum L.) . Theor. Appl. Genet. , 109 : 1105 – 1114 .
- Somers , D.J. , Fedak , G. , Clarke , J. and Cao , W.G. 2006 . Mapping of FHB resistance QTLs in tetraploid wheat . Genome , 49 : 1586 – 1593 .
- Srinivasachary , Gosman , N. , Steed , A. , Faure , S. , Bayles , R. , Jennings , P. and Nicholson , P. 2008 . Mapping of QTL associated with fusarium head blight in spring wheat RL4137 . Czech J. Genet. Plant , 44 : 147 – 159 .
- Steiner , B. , Lemmens , M. , Griesser , M. , Scholz , U. , Schondelmaier , J. and Buerstmayr , H. 2004 . Molecular mapping of resistance to fusarium head blight in the spring wheat cultivar Frontana . Theor. Appl. Genet. , 109 : 215 – 224 .
- Szabo-Hever , A. , Toth , B. , Lehoczki-Krsjak , S. and Mesterhazy , A. 2008 . Mapping of FHB resistance QTLs in the Mini Mano/Frontana and Frontana/Remus DH populations . Cereal Res. Commun , 36 : 271 – 275 . Supplement. B
- Van Ginkel , M. , VanderSchaar , W. , Yang , Z.P. and Rajaram , S. 1996 . Inheritance of resistance to scab in two wheat cultivars from Brazil and China . Plant Dis. , 80 : 863 – 867 .
- Van Ooijen , J.W. 2004 . MapQTL Version 5: Software for the mapping of quantitative trait loci in experimental populations , Wageningen , , 57 pages : Plant Research International .
- Van Ooijen , J.W. and Voorrips , R.E. 2001 . JoinMap Version 3.0: Software for the calculation of genetic linkage maps , Wageningen , , 51 pages : Plant Research International .
- Von der Ohe , C. , Ebmeyer , E. , Korzun , V. and Miedaner , T. 2010 . Agronomic and quality performance of winter wheat backcross populations carrying non-adapted fusarium head blight resistance QTL . Crop Sci. , 50 : 2283 – 2290 .
- Waldron , B.L. , Moreno-Sevilla , B. , Anderson , J.A. , Stack , R.W. and Frohberg , R.C. 1999 . RFLP mapping of QTL for fusarium head blight resistance in wheat . Crop Sci. , 39 : 805 – 811 .
- Weber , E. 1972 . Grundriss der biologischen Statistik , Jena : VEB Gustav Fischer Verlag . 706 pages
- Wilde , F. , Korzun , V. , Ebmeyer , E. , Geiger , H.H. and Miedaner , T. 2007 . Comparison of phenotypic and marker-based selection for fusarium head blight resistance and DON content in spring wheat . Mol. Breed. , 19 : 357 – 370 .
- Yang , Z.P. , Gilbert , J. , Somers , D.J. , Fedak , G. , Procunier , J.D. and McKenzie , I.H. 2003 . Marker assisted selection of fusarium head blight resistance genes in two doubled haploid populations of wheat . Mol. Breed. , 12 : 309 – 317 .
- Yang , Z.P. , Gilbert , J. , Fedak , G. and Somers , D.J. 2005 . Genetic characterization of QTL associated with resistance to fusarium head blight in a doubled-haploid spring wheat population . Genome , 48 : 187 – 196 .
- Yang , Z.P. , Gilbert , J. and Procunier , J.D. 2006 . Genetic diversity of resistance genes controlling fusarium head blight with simple sequence repeat markers in thirty-six wheat accessions from east Asian origin . Euphytica , 148 : 345 – 352 .
- Yu , J.B. , Bai , G.H. , Cai , S.B. and Ban , T. 2006 . Marker-assisted characterization of Asian wheat lines for resistance to fusarium head blight . Theor. Appl. Genet. , 113 : 308 – 320 .