Abstract
A previously undescribed spotting of tomato fruit was observed in the packinghouse and storage facility of a commercial organic grower in the Fraser Valley of British Columbia during 2009–2011. Symptoms initially appeared as small black spots on the tomato fruit, barely visible after harvest. The black spots expanded to form larger grey-black lesions with evidence of mycelial growth in the centre after 5–10 days of storage at 12 °C or after tomatoes were shipped to retail outlets. Isolations from symptomatic skin and pericarp tissues from early and expanded lesions primarily yielded species of Penicillium. Healthy tomato fruit inoculated with conidia of Penicillium isolates developed black spots which progressed to form larger grey lesions, typical of symptoms observed in the commercial greenhouse. Several Penicillium species were identified using morphological characteristics and DNA sequencing of the ITS region and partial β-tubulin gene. Isolates identified as P. olsonii produced the characteristic spotting of fruit followed by expanding lesions, while other isolates identified as P. solitum and P. polonicum caused a rapid soft rot and decay. Ripe fruit inoculated with P. olsonii had a significantly higher disease severity index compared with those that were inoculated at the breaker or flush stages. Inoculations on the tomato shoulder resulted in significantly higher disease severity than inoculations at the middle or base of the fruit. Swabs of tomato fruit surfaces and calyx tissues taken while fruit were still on the vine followed by plating onto PDA revealed that high populations (>40 CFU) of resident P. olsonii were present on the fruit. Similar populations were detected when calyx tissues were plated directly onto PDA. The mode of entry of P. olsonii is likely through naturally occurring cracks or russeting of the fruit cuticle from resident populations. Wounding of the skin did not significantly enhance disease severity compared with unwounded treatments. Disease severity increased significantly when tomatoes were incubated at 12 °C compared with 22 °C following inoculation. This appears to be the first report of a fruit spotting and post-harvest decay of tomato fruit caused by P. olsonii.
Résumé
Une tache à ce jour non décrite a été observée, de 2009 à 2011, sur les tomates dans la station fruitière et l'entrepôt d'un producteur biologique de la vallée du Fraser, en Colombie-Britannique. Les symptômes étaient caractérisés initialement par de petites taches noires, à peine visibles après la récolte, apparaissant sur les tomates. Les taches noires s'étendaient pour former de plus grandes lésions gris noir affichant une certaine croissance mycélienne en leur centre après de 5 à 10 jours d'entreposage à 12 °C, ou après que les tomates avaient été expédiées aux détaillants. L'isolement de peaux symptomatiques et de tissus du péricarpe issus de lésions fraîches et étendues a surtout produit des espèces de Penicillium. Des tomates saines, inoculées avec des conidies d'isolats de Penicillium ont développé des taches noires qui ont formé de plus grandes lésions grises, typiques des symptômes observés dans les serres commerciales. Plusieurs espèces de Penicillium ont été identifiées en se référant aux caractéristiques morphologiques et par séquençage de la région de l'espaceur transcrit interne de l'ADN et séquençage partiel du gène β-tubuline. Les isolats identifiés en tant que P. olsonii ont produit les taches caractéristiques sur les fruits, suivies des lésions étendues, tandis que d'autres isolats, identifiés en tant que P. solitum et P. polonicum, ont rapidement causé de la pourriture molle et de la décomposition. Les fruits mûrs inoculés avec P. olsonii affichaient un indice de gravité de la maladie significativement plus élevé que ceux qui étaient inoculés au début du rougissement ou au stade de la pousse. Les inoculations de la couronne du fruit ont engendré une gravité significativement plus intense de la maladie que celles appliquées au milieu ou à la base du fruit. Des prélèvements effectués sur la surface des tomates et sur les tissus du calice alors que les fruits étaient encore sur la grappe, suivis d'une mise en culture sur gélose dextrosée à la pomme de terre, ont révélé de fortes populations résidentes de P. olsonii (>40 CFU) sur les fruits. Des taux de population semblables ont été détectés quand les tissus des calices ont été directement cultivés sur gélose dextrosée à la pomme de terre. P. olsonii semble pénétrer dans le fruit par les fissures naturelles de celui-ci et la rugosité de la cuticule causée par les populations résidentes. Le fait de meurtrir la peau des fruits n'a pas significativement accru la gravité de la maladie comparativement aux fruits intacts. La gravité de la maladie s'est accrue significativement lorsque les tomates étaient incubées à 12 °C après inoculation, comparativement à 22 °C. Cette étude semble être la première qui traite de cette tache sur la tomate, causée par P. olsonii, et de la décomposition qui s'en suit après la récolte.
Introduction
Fruit spotting symptoms were sporadically observed on several cultivars of harvested fresh market on-the-vine (TOV) tomatoes from a commercial organically managed greenhouse in the Fraser Valley of British Columbia (BC) in April–October of 2009 and 2010. Affected fruit had small grey to black spots, 1–3 mm in diameter, that progressively expanded into larger grey or yellow lesions over time in storage. Initial spotting was seen on the fruit shoulder, close to the calyx, which is often the site of russeting or occurrence of miniature cracks in the cuticle (Dorais, Citation2004). Freshly harvested fruit did not display visible symptoms, which began to become apparent in the packinghouse coolers, where tomatoes were stored at 10–12 °C for periods of several days to weeks. Following shipment of mildly affected fruit, reports of more severe lesions and fruit decay were reported from storage locations of retail outlets. The presence of the fruit discolouration significantly reduced fruit appearance and market price of the high-valued organic tomato crop. Frequently, the fruit were returned to the grower and had to be discarded. The symptoms observed did not match those of common tomato fruit spots or post-harvest diseases reported in the literature (Jones et al., Citation1991; Howard et al., Citation1994), therefore the objectives of this study were to identify the causal agent of the disease and to investigate the effects of temperature and wounding on disease development.
Materials and methods
Isolation of causal agent
Tomatoes displaying a range of symptoms from small lesions to large expanding lesions () were obtained from the storage facility (12 °C) located at a commercial greenhouse in Delta, BC. Tissue pieces (∼0.25 mm2) were cut from symptomatic fruit and dipped for 30 s in 70% ethanol followed by immersion in 0.5% NaOCl for 60 s, and rinsed three times with sterile distilled water (SDW). Excess moisture was blotted on sterile filter paper and tissue pieces were placed on different agar media, including V8 juice agar, water agar and potato dextrose agar. Petri dishes were incubated at room temperature (21–23 °C) until fungal growth was observed, at which time transfers were made to V8 agar. Cultures were stored on this medium at 4 °C.
Fig. 1. Symptoms of spotting observed on tomato fruit collected from the packinghouse of a commercial greenhouse in the Fraser Valley of BC. (A–C) Black spots can be seen 2–3 days after harvest, and progress to yellow (D) or grey (E) lesions after 7–10 days in storage. (F). Penicillium-like conidiophores visible on clear tape lifts taken from sporulating lesions. (G) Hyphae and necrosis associated with cuticle cracks from black spots compared with cuticle cracks of healthy tissues (H) (400 × magnification, bar = 20 μm).
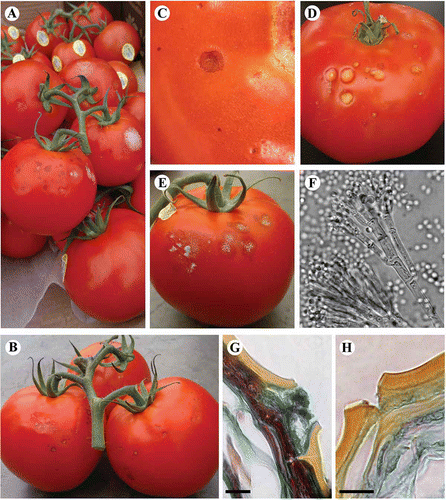
Microscopic observations
Tissue sections from young lesions were prepared for light microscopy to observe the progression of lesions. Pieces c. 2 mm2 were fixed in FAA (ethanol (77%), water (7.9%), acetic acid (7.6%), formaldehyde (5.8%) and, methanol (1.7%)), dehydrated in an ethanol series (70 to 100%), embedded in paraffin, cross-sectioned (5 μm), and stained with Periodate-Schiff or Gram stains to visualize the presence of hyphae or bacteria, respectively, in symptomatic tissues. Control healthy tissues were also obtained, fixed and sectioned for comparison.
Pathogen identification
Most colonies isolated from lesions were initially identified as Penicillium species based on morphological characteristics of single-spore isolates on malt extract agar (MEA) and Czapek yeast agar (CYA) (Frisvad & Samson, Citation2004). Isolates were subsequently identified to species from DNA sequences following amplification of the β-tubulin gene using primer pairs Bt2a (5′ GGTAACCAAATCGGTGCTGCTTTC 3′) and Bt2b (5′ ACCCTCAGTGTAGTGACCCTTG-GC 3′), and the ITS region using primers ITS1 (5′ TCCGTAGGTGAACCTGCGG 3′) and ITS4 (5′ TCCTCCGCTTATTGATATGC-3′), respectively (Glass & Donaldson, Citation1995; O'Donnell & Cigelnik, Citation1997; Samson et al., Citation2004). If sequences derived from these two primer sets were inconclusive for species identification, an additional primer pair, PenF1 (5′ GACAAGAAA-GGTGATTTTTATCTT 3′) and AspR1 (5′ GGTAATGATAATAATAATAATACAGCT 3′) that amplified the cytochrome-oxidase gene, was used (Seifert et al., Citation2007). Purified PCR-samples were sent to MCLAB (San Francisco, CA) for sequencing. Sequences were edited using BioEdit v7.0.9. software, and compared with GenBank using BLASTn.
Proof of pathogenicity
Penicillium isolates consistently isolated from tomato lesions were tested for their ability to produce symptoms on tomato fruit to fulfill Koch's postulates. Healthy tomatoes were obtained from the commercial organic greenhouse, with cultivar type dependent on what was being harvested at the time (May–October 2010). Whole tomatoes were surface-sterilized in 70% ethanol and 0.5% NaOCl prior to inoculation. To prepare fungal inoculum, isolates were grown on V8 agar for 2 weeks at room temperature. Conidia, collected by scraping a small area of the culture (∼1 cm2) with a sterile scalpel, were suspended in SDW with 0.1% Tween 20. Conidia concentration was determined using a haemocytometer and adjusted to provide 106 conidia mL−1, as necessary.
Ten μL droplets of the conidial suspension were pipetted onto the tomato shoulder for a total of 10 separate inoculation points on each fruit. The skin was pierced gently with a sterile insect mounting pin through the inoculum droplet at each of five inoculation points to determine if wounding of the tomato skin was required for infection, while the other five inoculation points on a fruit remained unwounded. Nine fungal isolates () were tested for their ability to cause lesions with or without wounding. A control treatment, either using SDW + Tween 20 or a non-Penicillium species (Cladosporium sp.) as inoculum, was included in all trials. Tomatoes, inoculated with individual isolates, were placed inside separate plastic boxes lined with moistened paper towels which were sealed and placed at room temperature for 7–14 days to monitor for disease development. Treatments were arranged using a randomized design. Each isolate was inoculated separately onto three tomatoes, and the experiment was repeated twice. The number of resulting lesions was counted and scored according to lesion severity as follows: 0 = no lesion; 1 = small black spots contained within the area of the inoculation droplet; 2 = black spots spreading past the inoculation point; and 3 = large coalescing lesions. A disease severity index (DSI) was then calculated as follows: DSI = [(X1*1) + (X2*2) + (X3*3)]/total number of inoculated drops, where X1, X2 and X3 were the number of droplets with lesion severity of 1, 2 or 3, respectively. Lesions that developed were excised, surface-sterilized and plated onto V8 agar as described previously to confirm infection by the fungus inoculated onto the site.
Table 1. Isolate number, species identification and symptoms produced on tomato fruit by fungi recovered from symptomatic greenhouse tomatoes, and a listing of which isolates were used in particular experiments
Influence of temperature on disease severity
The ability of Penicillium isolates to cause lesions at 12 °C compared with room temperature (21 +/−2 °C) was tested. Unwounded tomato fruit were inoculated as above with a total of eight isolates () plus a control treatment. After inoculation, fruit were incubated in chambers which were maintained at 12 °C or room temperature. Each isolate was inoculated onto six replicate tomatoes, with three fruit per isolate incubated at each temperature. The experiment was repeated twice. Disease severity was assessed as described above. The temperature requirements for radial growth of the isolates were also determined by growing them on CYA medium and incubating at 5, 12, 15, 20, 25, 28, 30 and 35 °C. Colony diameter was measured after 14 days of growth. Each isolate was replicated three times and the experiment was repeated twice.
Influence of fruit ripening stage and inoculation position on disease severity
Tomato fruit at three different stages – breaker (stage 2), pink (stage 4) and red ripe (stage 6) – were obtained to determine which ripening stages are most predisposed to infection. Fruit were surface sterilized before inoculation as described previously. Spore suspensions were prepared from four Penicillium isolates, as well as a Cladosporium isolate that was recovered previously from tomatoes (). An additional treatment of SDW + Tween 20 was included as a control. Inoculum droplets (106 conidia mL−1) were placed at 3-cm intervals from calyx to blossom end of the fruit at each ripening stage, for a total of three inoculation positions over the same tomato fruit (shoulder, middle and base). Tomatoes were propped up on a moistened cheesecloth wedge to prevent the base inoculation from touching the containers, and were inoculated in place in their respective containers in the incubator to prevent movement of tomatoes after inoculations had occurred. Once tomatoes were secured in place, six inoculation droplets were placed at each of the three positions on the exposed surface of the fruit. Treatments were arranged in humid boxes in the incubator using a randomized design. Each treatment combination (isolate × ripening stage) was replicated three times, and the experiment was repeated once. Disease severity was calculated after incubation at 12 °C for 14 days as before.
Sampling for inoculum presence
Swabs were taken of breaker-stage fruit using a sterile cotton swab to determine the presence of Penicillium and other fungi on the fruit surface. Two replicate fruit on each of five plants were gently swabbed and the cotton swabs were streaked across the surface of PDA in 60 × 15 mm Petri dishes. Sampling was conducted at five locations within the greenhouse. Swabs were also taken of calyx tissues on the same fruit. The sampling procedure was repeated at the same locations within the greenhouse at weekly intervals during the period July–August 2011. Petri dishes were placed on the laboratory bench under ambient conditions and the number of fungal colonies, in particular Penicillium, was recorded after 7 days. Presumptive identification of Penicillium colonies was based on colony morphology. Random samples (4–5) of leaf prunings that had been discarded on the greenhouse floor were also taken at various times during the season and small pieces were plated onto PDA to determine the types of fungi present.
Statistical analysis
Data from individual trials were combined for analysis when F-tests indicated that variances of the data did not differ significantly. Analysis of variance was performed using the proc glm statement to determine significance of main treatment effects (isolate) and the interaction of treatment with other experimental effects (wounding, temperature, ripening stage and position) using the Statistical Analysis System software (version 9.1; SAS Institute, Inc., Cary, NC). For all experiments, significant differences among treatment means were separated using Tukey's HSD, when ANOVA results indicated there were significant differences among isolates and treatment factors. The Type 1 error rate (α) was set at 0.05 for all statistical tests.
Results
Progression of disease symptoms
Symptoms were initially observed as small grey to black spots, 1–2 mm in diameter, on the surface of ripe fruit (, B, C). Lesions appeared superficial and when the affected tomato skin was gently removed, there was no discolouration visible in the underlying pericarp. Three to four days after initial symptom development, the black spots enlarged to give rise to slightly sunken grey and yellow lesions (5 mm in diameter), that could not be separated from the underlying pericarp (). At advanced stages, approximately 7–10 days after appearance of the black spots, there was visible mycelial growth and sporulation, which appeared as whitish to velvety grey/green areas, in the centre of the lesions (). At this stage, lesions extended into the pericarp, and in severe cases, the entire lesioned area was softened. Clear tape lifts from these areas viewed under the compound microscope revealed the presence of conidiophores with a Penicillium-like structure ().
Microscopic observations of thin sections made through developing lesions showed that there were fungal masses present within the spots, and that the underlying pericarp was necrotic (). The hyphal masses were usually associated with cuticular cracks, suggesting a possible entry point for initial infection (). By comparison, sections made through healthy tissues revealed the presence of cuticular cracks without the fungal mass or necrosis present ().
Pathogen identification
Penicillium colonies were recovered from fruit lesions, almost exclusively, at both early and late stages of the infection, over the sampling period of 2 years. Periodically, Cladosporium and Rhizopus colonies grew from tomato lesions, but always from lesions that were at advanced stages of decay. There were at least three morphologically distinct types of Penicillium colonies recovered (–C). Isolates with differing morphology were cultured on MEA and CYA to further record colony characteristics. The most commonly isolated colonies (from ∼90% of symptomatic tomatoes) were greyish-green in colour on the surface, yellow to cream colour on the underside, and did not produce any exudates or soluble pigments on CYA at 25 °C (). The second most abundant colony type was dark green/blue in colour on the surface, becoming greyer in older colonies, yellow or light brown in colour on the underside, with clear exudates, and no pigmentation of the agar (). The third colony type was blue-grey in colour on the surface, and cream and yellow on the underside (). This colony type readily produced clear exudates, and produced a diffusible brown/beige pigment in the agar in older cultures.
Fig. 2. (A–C) Penicillium isolates from black spots on tomato fruit collected from a commercial packinghouse. (A) P. olsonii; (B) P. solitum; and (C) P. polonicum. All isolates were grown on CYA for 7 days at room temperature. (D–I) Symptoms on tomato fruit. Inoculation was conducted with (D, E, F) P. olsonii, (G) Cladosporium spp., (H) P. solitum, and (I) P. polonicum. Tomato fruit were inoculated at the (D) breaker or (E–I) ripe stage with a 10 uL droplet of conidia at 10 individual points and incubated in humid chambers at (E) room temperature or (D, F–I) at 12 °C for 14 days.
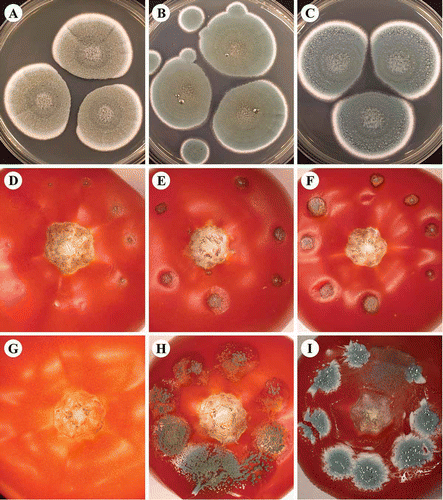
Sequencing of partial β-tubulin gene and ITS regions indicated that the first two morphological types above (, B) belonged to two species of Penicillium, namely P. olsonii and P. solitum, respectively (100% identity). The third morphological type, isolate 510T5 (), was identified as P. polonicum. Sequencing results from the β-tubulin region indicated that this colony type was P. polonicum (98% identity), while the results from the ITS region indicated it to be an unknown Penicillium spp. or P. polonicum with equal per cent identity (99%). Sequencing results from a region of the cytochrome oxidase gene showed isolate 510T5 to be either P. tricolor or P. camemberti with equal likelihood (100%). Combining morphology and molecular data, this isolate () is considered most likely to be P. polonicum. Since P. tricolor has a distinct orange-border on the colony edge when grown on CYA, while P. camemberti occurs exclusively on soft white cheeses (Frisvad & Samson, Citation2004), they are unlikely to be recovered from tomato fruit. Penicillium polonicum is often reported as a contaminant of processed meats products (Aranda et al., Citation2002); however, there are no previous reports indicating that P. polonicum is an airborne constituent in greenhouses or identified as a plant pathogen. Identification of the three recovered Penicillium species described here was confirmed by the National Fungal Identification Services (Agriculture and Agri-Food Canada, Ottawa, ON).
Proof of pathogenicity
Isolates identified as P. olsonii consistently produced typical symptoms corresponding to those observed on greenhouse tomatoes when inoculated onto healthy tomato fruit. Lesions started as small grey to black spots that progressively increased in size at inoculation points (–F). The isolate identified as P. solitum initially produced similar symptoms, but lesions developed at a much faster rate, and often resulted in a rotting of the fruit with extensive green sporulation present (). Disease symptoms caused by the P. polonicum isolate always resulted in rapid and extensive decay of the tomato fruit accompanied by abundant blue sporulation on the fruit surface (). Since this latter isolate caused symptoms of severe rot that did not correspond to typical symptoms found in the greenhouse, it was not included in further experiments.
Analysis of variance indicated that wounding did not significantly affect lesion development compared with unwounded treatments (P = 0.0664), and there was no significant interaction between isolate and wounding treatment (P = 0.5611). Isolate type had a significant effect on the severity of symptoms (P < 0.0001). However, statistical means separation of isolates was not undertaken because symptoms differed between the three species and only one isolate each of P. polonicum and P. solitum was tested compared with seven isolates of P. olsonii. In general, the isolate of P. polonicum (510T5) was the most aggressive pathogen with regard to fruit decay, followed by P. solitum (520T9), whereas the P. olsonii isolates caused moderate symptoms on tomato fruit (). The disease symptoms caused by isolates of P. olsonii were identical to those observed on diseased tomatoes from the greenhouse.
Fig. 3. Disease severity index of symptoms caused by Penicillium species inoculated onto healthy ripe tomato fruit. Tomatoes were either unwounded or wounded with a sterile insect mounting pin at each inoculation point. The shoulder area of red ripe fruit were inoculated with 10 μL droplets of conidia at each inoculation point and incubated for 14 days at room temperature. An isolate of Cladosporium spp. (520T3) recovered from tomatoes served as the control. Isolate abbreviations: Po = P. olsonii, Ps = P. solitum and Pp = P. polonicum.
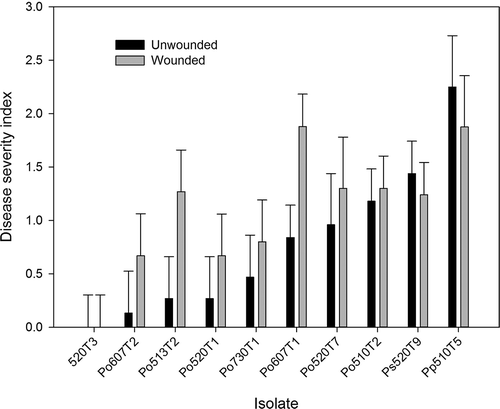
Influence of temperature on disease severity
Temperature and isolate type had a significant effect (P = 0.0047 and 0.0001, respectively) on lesion development caused by Penicillium species. Overall, the disease severity index was higher at 12 °C compared with room temperature, but this difference was not significant for all isolates (). Inoculation with P. solitum at the lower temperature resulted in rapidly coalescing and yellowing lesions, compared with room temperature. Lesions caused by P. olsonii at room temperature remained as small superficial black spots that did not spread beyond the inoculation droplet (). Inoculation with isolates of P. olsonii at 12 °C resulted in lesions that progressed from small black spots to larger yellowing lesions, but lesions often did not spread more than 3–5 mm past the inoculation point after 7 days (). After an extended time of incubation at 12 °C (2–3 weeks), fruit inoculated with P. olsonii had larger lesions but did not decay (data not shown).
Fig. 4. Disease severity index of lesions caused by Penicillium species inoculated onto healthy ripe tomato fruit. Tomatoes were incubated at room temperature (21 +/−2 °C) or at 12 °C in humid chambers for 2 weeks after inoculation. Inoculation was conducted by pipetting 10 uL droplets of conidial suspension onto the unwounded tomato shoulder. Isolate abbreviations: Po = P. olsonii and Ps = P. solitum.
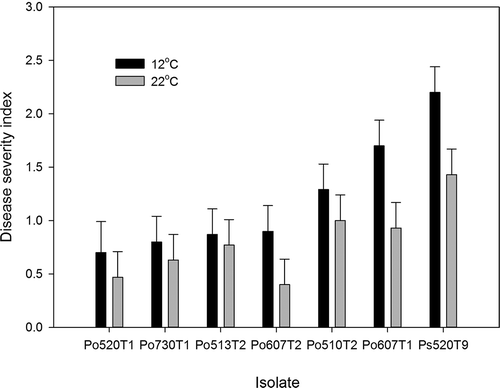
All P. olsonii isolates recovered from tomato fruit displayed similar growth patterns over the range of temperatures tested, so the growth data for P. olsonii isolates was combined for analysis. All isolates showed minimal growth at 5 and 35 °C on CYA (). Mycelial growth occurred at 12 °C, with maximum growth observed between 20–25 °C. In contrast, both P. solitum and P. polonicum grew at 5 °C; at temperatures below 15 °C, these isolates grew much more rapidly than P. olsonii isolates.
Influence of ripening stage and inoculation position on disease severity
The tomato ripening stage and position of inoculation had significant effects on the disease severity index caused by Penicillium species (P < 0.0001 for both). The general trend observed was that disease symptoms were most severe in fully ripened fruit, and when inoculations occurred on the tomato shoulder (). In particular, P. solitum caused the most damage to ripe tomatoes when inoculated at the shoulder, and this disease severity index was significantly higher than that of all other treatment combinations. Lesions developed on fruit that were at both breaker and pink ripening stages when inoculations occurred at the shoulder. Inoculations on the middle of the fruit resulted in development of small lesions, with ripe tomatoes displaying the largest lesions, but severity was generally lower compared with shoulder inoculations (). Inoculations at the base of the tomato rarely resulted in disease development, regardless of the tomato ripening stage (). There was a very low level of disease observed on tomatoes inoculated with an isolate of Cladosporium sp. (isolate 520T3). However, subsequent reisolations from the developing lesions indicated that there was contamination with Penicillium, which was likely P. olsonii based on morphology in culture.
Fig. 6. Disease severity index values for infection caused by Penicillium species on tomato fruit. Fruit were inoculated at the breaker, pink or red ripe stage at three different positions: (a) shoulder, (b) middle region, or (c) base of the fruit, and incubated at 12 °C in humid chambers for 14 days. An isolate of Cladosporium spp. (520T3) recovered from tomatoes served as the control. Isolate abbreviations: Po = P. olsonii and Ps = P. solitum.
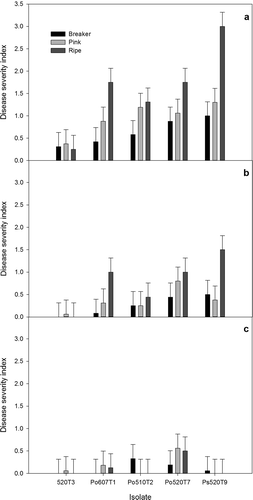
Sampling for inoculum presence
Swab samples taken from the surfaces of ripening tomato fruit while on the vine at various times during the growing season revealed the presence of a large number of fungal colonies recovered on PDA. The most prevalent genus was Penicillium, followed by Rhizopus, Cladosporium, Alternaria and Aspergillus (data not shown). Very few bacteria were recovered. The colony forming units of Penicillium per dish was greater than 40 CFU in many samples. shows colonies of Penicillium species growing on PDA 7 days after recovery from fruit surfaces. From calyx samples, a similarly high recovery of Penicillium colonies was observed. Using morphological criteria, the colonies of Penicillium were identified mostly as P. olsonii. All of the fungal genera recovered from fruit surfaces were also isolated from leaf litter plated onto PDA, with Penicillium being the most predominant (data not shown).
Discussion
The most frequently isolated fungus from diseased tomato fruit in this study was identified as P. olsonii and inoculation of healthy tomato fruit with P. olsonii conidia consistently resulted in the appropriate disease symptoms. Although P. olsonii is not known to cause post-harvest losses during fruit storage, it is a common airborne contaminant in greenhouses and packinghouses (Rodolfi et al., Citation2003; Scott et al., Citation2004; Reboux et al., Citation2009; Li & Lamondia, Citation2010). While P. olsonii has been previously isolated from the surface of mouldy tomato fruit (Frisvad & Samson, Citation2004), there are no published reports showing its ability to cause disease on tomato fruit. This species is also a common contaminant found in the air and on surfaces of pomaceous and citrus fruit within packinghouses. However, previous tests to determine the pathogenicity of P. olsonii on various fruit showed that it only caused mild lesions on apricots (Oliveri et al., Citation2007). Other species of Penicillium, particularly P. expansum, P. solitum and P. digitatum, are commonly associated with postharvest diseases, causing severe losses on apple, pear and citrus fruit (Jones & Aldwinckle, Citation1990). However, Penicillium spp. are not typically associated with post-harvest losses on tomato fruit (Jones et al., Citation1991), although there are reports of P. expansum causing decay of tomato fruit (Miedes & Lorences, Citation2004; Liu et al., Citation2007). Recently, a disease described as blue mould, caused by P. oxalicum, was reported on greenhouse tomato fruit in Korea and Japan (Kwon et al., Citation2008; Umemoto et al., Citation2009). In Japan, the pathogen caused blighting and canker of the shoot system (Umemoto et al., Citation2009), whereas in Korea, P. oxalicum caused a watery rot of mature fruit (Kwon et al., Citation2008). In our study, there were two species of Penicillium isolated from tomato fruit from the packinghouse that had the ability to cause a watery decay and collapse of tomato fruit. The percentage recovery of these isolates, however, was extremely low compared with the abundance of P. olsonii. Occasional fruit decay, similar to that observed in laboratory inoculations of tomato fruit by Penicillium spp., can occur in fruit stored for an extended period of time and is likely caused by species other than P. olsonii.
The initial report of penicillium fruit spot was on organically managed tomatoes, but surveys conducted in 2011 have shown that the disease also occurs on fruit produced conventionally in BC and in the USA (authors, unpublished data). Therefore, this disease is not associated exclusively with organic tomatoes. Our observations have shown that the disease is predominant on vine-ripened (on-the-vine) greenhouse-grown fruit. It is not as common on field-grown tomatoes, and on fruit where the calyx is removed. Samples of calyx tissues were shown to harbour high levels of Penicillium in this study, and this could provide a source of inoculum for disease development during storage or shipping. Further studies are required to determine whether leaf litter contributes to the airborne spore load that may subsequently land on the fruit and calyx surfaces.
There were three main factors that influenced development and severity of lesions caused by P. olsonii on tomato fruit, namely temperature, fruit developmental stage and inoculation position. Lesions expanded and coalesced more rapidly at cooler temperatures compared with room temperature. Chilling injury, which occurs due to exposure to temperatures below 15 °C, is a major factor contributing to predisposition of tomato fruit to post-harvest diseases (Ding et al., Citation2002). After exposure to cool temperatures, tomatoes are more likely to be susceptible to invasion by weakly pathogenic fungi, such as Penicillium spp. (Jones et al., Citation1991). Post-harvest disease development at lower temperatures, such as those found during storage, is further exasperated by russeting of the fruit cuticle. Fractures in the fruit surface act as potential entry points for invading microorganisms, allowing weak pathogens to colonize exposed tissues (Ehret et al., Citation2008). Our results indicated that lesions caused by P. olsonii were more likely to develop and were more severe on the tomato shoulder, which is the principal site of cuticular cracking (Dorais, Citation2004). Micrographs of young lesions verified that fungal hyphal masses were associated with cracks in the cuticle. This avenue of entry and predisposition by cool temperatures which enhance disease development has been described for Alternaria alternata, the causal agent of post-harvest black mould of tomatoes (Jones et al., Citation1991; Cota et al., Citation2007). This pathogen was not recovered from any of the greenhouse tomatoes sampled in this study and is more commonly found on field-grown fresh market tomatoes.
Penicillium fruit spot symptoms were most severe when inoculations were made on ripe fruit, while fruit inoculated at the breaker stage showed the least severe symptoms. When the fruit enters the final stages of ripening, and at optimal growth temperatures, existing defence mechanisms in the plant are likely sufficient to prevent infection and spread of weak pathogens. This resistance can be reduced when fruit are chilled, and those pathogens that are capable of growth at cooler temperatures can continue growth and produce symptoms by colonizing fruit tissues (Sommer, Citation1982). Increased susceptibility of ripening fruit to pathogen invasion can be accentuated by the breakdown of pectin and hemicelluloses that occurs by constitutive cell-wall degrading enzymes (Cantu et al., Citation2008). Spores of P. olsonii detected on the calyx tissues of the developing fruit during growth as well as on ripe fruit surfaces can serve as an inoculum source. Points of contact of the calyx tissue with the fruit surface during storage were frequent points of infection and disease development (unpublished observations). Further investigations are required to determine at what point during growth of the tomato fruit inoculum of P. olsonii is deposited, and whether there is a dormancy period between initial infection and symptom development in the packinghouse. One possibility is that P. olsonii has the ability to infect fruit at the breaker stage, but that there is a delay in symptom development until the fruit reaches the ripe red stage. Understanding the source of inoculum, whether airborne and/or from residual plant debris, and the time of infection and environmental conditions leading up to symptom development in the packinghouse will aid in developing management strategies to reduce penicillium fruit spot and post-harvest decay.
Acknowledgements
We thank the tomato growers, in particular Origin Organic Farms, who provided fruit samples for this study. Technical assistance of Gina Rodriguez and Sean Formby is gratefully acknowledged. Funding for this work was made possible through the Growing Forward Initiative, supported by Agriculture and Agri-Food Canada, the B.C. Greenhouse Growers Association, and the B.C. Ministry of Agriculture and Food, Abbotsford. We thank Keith Seifert at Agriculture and Agri-Food Canada, Ottawa, for confirming the identification of the three Penicillium species used in this study.
References
- Aranda , E. , Rodríguez , M. , Benito , M.J. , Asensio , M.A. and Córdoba , J.J. 2002 . Molecular cloning of verrucosidin-producing Penicillium polonicum genes by differential screening to obtain a DNA probe . Int. J. Food Microbiol. , 76 : 55 – 61 .
- Cantu , D. , Vicente , A.R. , Greve , L.C. , Dewey , F.M. , Bennett , A.B. , Labavitch , J.M. and Powell , A.L.T. 2008 . The intersection between cell wall disassembly, ripening, and fruit susceptibility to Botrytis cinerea . Proc. Natl. Acad. Sci. USA , 105 : 859 – 864 .
- Cota , I.E. , Troncoso-Rojas , R. , Sotelo-Mundo , R. , Sánchez-Estrada , A. and Tiznado-Hernández , M.E. 2007 . Chitinase and [beta]-1,3-glucanase enzymatic activities in response to infection by Alternaria alternata evaluated in two stages of development in different tomato fruit varieties . Sci. Hortic.–Amsterdam , 112 : 42 – 50 .
- Ding , C.K. , Wang , C.Y. , Gross , K.C. and Smith , D.L. 2002 . Jasmonate and salicylate induce the expression of pathogenesis-related-protein genes and increase resistance to chilling injury in tomato fruit . Planta , 214 : 895 – 901 .
- Dorais , M. 2004 . Greenhouse tomato fruit cuticle cracking . Hort. Rev. (Amer. Soc. Hort. Sci.) , 30 : 163 – 184 .
- Ehret , D.L. , Hill , B.D. , Raworth , D.A. and Estergaard , B. 2008 . Artificial neural network modelling to predict cuticle cracking in greenhouse peppers and tomatoes . Comput. Electron. Agric. , 61 : 108 – 116 .
- Frisvad , J.C. and Samson , R.A. 2004 . Polyphasic taxonomy of Penicillium subgenus Penicillium: a guide to identification of food and air-borne terverticillate Penicillia and their mycotoxins . Stud. Mycol. , 49 : 1 – 173 .
- Glass , N.L. and Donaldson , G.C. 1995 . Development of primer sets designed for use with the PCR to amplify conserved genes from filamentous ascomycetes . Appl. Environ. Microbiol. , 61 : 1323 – 1330 .
- Howard , R.J. , Garland , J.A. and Seaman , W.L. 1994 . Diseases and Pests of Vegetable Crops in Canada: An Illustrated Compendium , Ottawa : Canadian Phytopathological Society .
- Jones , A.L. and Aldwinckle , H.S. 1990 . Compendium of Apple and Pear Diseases , St. Paul , MN : American Phytopathological Society Press .
- Jones , J.B. , Stall , R.E. and Zitter , T.A. 1991 . Compendium of Tomato Diseases , St. Paul , MN : American Phytopathological Society Press .
- Kwon , J.-H. , Shen , S.-S. and Jee , H.-J. 2008 . Occurrence of blue mold on tomato caused by Penicilium oxalicum in Korea . Plant Pathol. J. , 24 : 87 – 90 .
- Li , D.W. and Lamondia , J. 2010 . Airborne fungi associated with ornamental plant propagation in greenhouses . Aerobiologia , 26 : 15 – 28 .
- Liu , J. , Tian , S. , Meng , X. and Xu , Y. 2007 . Effects of chitosan on control of postharvest diseases and physiological responses of tomato fruit . Postharvest Biol. Technol. , 44 : 300 – 306 .
- Miedes , E. and Lorences , E.P. 2004 . Apple (Malus domestica) and tomato (Lycopersicum esculentum) fruits cell-wall hemicelluloses and xyloglucan degradation during Penicillium expansum infection . J. Agric. Food Chem. , 52 : 7957 – 7963 .
- O'Donnell , K. and Cigelnik , E. 1997 . Two divergent intragenomic rDNA ITS2 types within a monophyletic lineage of the fungus Fusarium are nonorthologous . Mol. Phylogen. Evol. , 7 : 103 – 116 .
- Oliveri , C. , Campisano , A. , Catara , A. and Cirvilleri , G. 2007 . Characterization and AFLP genotyping of Penicillium strains from postharvest samples and packinghouse environments . J. Plant Pathol. , 89 : 29 – 40 .
- Reboux , G. , Bellanger , A.P. , Roussel , S. , Grenouillet , F. , Sornin , S. , Piarroux , R. , Dalphin , J.C. and Millon , L. 2009 . Indoor mold concentration in Eastern France . Indoor Air , 19 : 446 – 453 .
- Rodolfi , M. , Lorenzi , E. and Picco , A.M. 2003 . Study of the occurrence of greenhouse microfungi in a botanical garden . J. Phytopathol. , 151 : 591 – 599 .
- Samson , R.A. , Seifert , K.A. , Kuijpers , A.F.A. , Houbraken , J.A.M.P. and Frisvad , J.C. 2004 . Phylogenetic analysis of Penicillium subgenus Penicillium using partial β-tubulin sequences . Stud. Mycol. , 49 : 175 – 200 .
- SAS Institute, Inc., Cary, NC, version 9.1.
- Scott , J. , Untereiner , W.A. , Wong , B. , Straus , N.A. and Malloch , D. 2004 . Genotypic variation in Penicillium chrysogenum from indoor environments . Mycologia , 96 : 1095 – 1105 .
- Seifert , K.A. , Samson , R.A. , Dewaard , J.R. , Houbraken , J. , Lévesque , C.A. , Moncalvo , J.M. , Louis-Seize , G. and Hebert , P.D.N. 2007 . Prospects for fungus identification using CO1 DNA barcodes, with Penicillium as a test case . Proc. Natl. Acad. Sci. USA , 104 : 3901 – 3906 .
- Sommer , N.F. 1982 . Postharvest handling practices and postharvest diseases of fruit . Plant Dis. , 66 : 357 – 364 .
- Umemoto , S. , Odake , Y. , Takeuchi , T. , Yoshida , S. , Tsushima , S. and Koitabashi , M. 2009 . Blue mold of tomato caused by Penicillium oxalicum in Japan . J. Gen. Plant Pathol. , 75 : 399 – 400 .