Abstract
Verticillium wilt, caused by the soilborne pathogen Verticillium dahliae (Kleb.), is an important disease affecting more than 400 plant species, including many economic crops. This study explored differential accumulation of phenolic compounds in potato in response to weakly and highly aggressive isolates of this pathogen. After phenolic compounds were extracted from roots, stems and leaves of inoculated plants, those showing differential accumulation were identified using high performance liquid chromatography (HPLC) equipped with a photodiode array detector, a fluorescence detector, and co-elution with commercial standards. Profiling of phenolic compounds during the potato–V. dahliae interaction confirmed previous observations on rutin accumulation in response to infection and showed differential accumulation of hydroxycinnamic acids in different tissues in response to two isolates possessing different levels of aggressiveness. In particular, differential accumulation of caffeic and ferulic acids was recorded in the roots and stems after inoculation, especially with the highly aggressive isolate. In the stems, the accumulation of rutin in response to inoculation was inhibited by the highly aggressive isolate, as compared with the weakly aggressive one.
Résumé
La flétrissure verticillienne, causée par l'agent pathogène terricole Verticillium dahliae (Kleb.), est une maladie importante qui s'attaque à plus de 400 espèces de plantes, y compris de nombreuses cultures de grande importance économique. Cette étude a exploré l'accumulation différentielle de composés phénoliques dans la pomme de terre en réaction à des isolats faiblement et hautement virulents de cet agent pathogène. Après avoir extrait les composés phénoliques des racines, des tiges et des feuilles des plants inoculés, ceux affichant une accumulation différentielle ont été identifiés par chromatographie liquide à haute performance (CLHP) équipée d'un détecteur à photodiodes, d'un détecteur de fluorescence et par coélution avec des standards commerciaux. Le profilage des composés phénoliques durant les interactions pomme de terre-V. dahliae a confirmé les observations antérieures sur l'accumulation de rutine en réaction à l'infection et a démontré l'accumulation différentielle d'acides hydroxycinnamiques dans différents tissus en réaction à deux isolats possédant différents degrés de virulence. Notamment, l'accumulation différentielle d'acide caféique et d'acide férulique a été enregistrée dans les racines et les tiges après inoculation, particulièrement en réponse à l'isolat hautement virulent. Dans les tiges, l'accumulation de rutine en réaction à l'inoculation a été inhibée par l'isolat hautement virulent, en comparaison avec l'isolat faiblement virulent.
Introduction
Verticillium wilt of potato is mainly caused by the soilborne pathogen Verticillium dahliae (Kleb.), which affects a broad range of dicotyledonous annual, perennial and woody plants worldwide (Johnson & Dung, Citation2010). Symptoms include chlorosis, necrosis, vascular discoloration, stunting and wilting (Pegg & Brady, Citation2002), thereby affecting both tuber quality and yield. The disease cycle starts with V. dahliae’s microsclerotia, which can survive in the soil for 10–15 years (Wilhelm, Citation1955). They are stimulated to germinate by root exudates, and develop hyphae, which penetrate and colonize the roots. The hyphae then grow into the xylem vessels and reproduce asexually by conidiospores that move upward in the vascular system (Rowe & Powelson, Citation2002). At the end of the disease cycle, the fungus forms microsclerotia as survival structures.
Verticillium wilt management relies on crop rotation, the use of tolerant cultivars where available (Johnson & Dung, Citation2010), and to a lesser extent on soil fumigation and non-chemical methods including solarization and biological control (Uppal et al., Citation2007, Citation2008). However, in absence of true resistant cultivars, and due to the cost and environmental impact of fumigation and the low efficacy of currently used methods, the development of better management strategies has never been more desirable. Pursuing such a purpose requires a better understanding of the mechanisms underlying infection by the pathogen and the corresponding responses by the host.
Host defences against pathogens can be either constitutive, e.g. structural barriers, phytoanticipins (Osbourn, Citation1996), or induced, e.g. cell wall fortification, tyloses, phytoalexins, reactive oxygen species (ROS) and pathogenesis-related proteins (van Loon et al., Citation2006; Hückelhoven, Citation2007). Many of these reactions are regulated by plant defence signalling involving salicylic, jasmonic and abscisic acids, as well as ethylene and other plant hormones (Vlot et al., Citation2009; Pieterse et al., Citation2009) through a very complex cross-talk pathway (Kunkel & Brooks, Citation2002; Robert-Seilaniantz et al., Citation2011). For example, both salicylic acid (SA) and its glucoside increase in the xylem sap of Brassica napus L. inoculated with V. longisporum (C. Stark) Karapapa, Bainbr. (Ratzinger et al., Citation2009) whereas defence signalling in Arabidopsis thaliana (L.) Heynh. to V. dahliae or one of its toxins involved ethylene (Pantelides et al., Citation2010) and nitric oxide (Shi et al., Citation2009), respectively.
Many plant secondary metabolites have been reported as defence factors against pathogens, including fungi, bacteria and viruses, and many of them are synthesized through the phenylpropanoid pathway. Those involved in plant disease resistance (Arfaoui et al., Citation2007; El Hassni et al., Citation2007) include both phenolics and terpenoids, which were reported to accumulate in cotton seedlings inoculated with V. dahliae (Daayf et al., 1997). Lignin-like phenolics, as well as the sesquiterpenes hemigossypol and desoxyhemigossypol, were also produced in cotton in response to this pathogen (Smit & Dubery, Citation1997; Bianchini et al., Citation1999). In potato, the flavonoid rutin (quercetin-3-O-rhamnoglucoside) also accumulates in response to inoculation with V. dahliae. However, highly aggressive isolates of this pathogen can utilize rutin as a carbon source by cleaving its sugar moiety and metabolizing the remaining flavonol (El Hadrami et al., Citation2009a , Citation2009b ). Comparative analyses using proteomics (El-Bebany et al., Citation2010) and transcriptomics (El-Bebany et al., Citation2011) of the same V. dahliae isolates used in the current study showed that several genes and proteins in the highly aggressive isolate may be involved in overcoming and/or suppressing potato defence mechanisms, indicating a more complex regulation of this interaction than initially considered. The level of aggressiveness of two V. dahliae isolates – Vd1396-9 (highly aggressive) and Vs06-14 (weakly aggressive) – correlated well with the differential gene expression of PAL1, PAL2, PR-1, PR-2 and PR-5 induced in potato (Derksen et al., Citation2013). In light of such data, the objective of this study was to compare the effects of these highly vs weakly aggressive isolates of V. dahliae on the accumulation of phenolic compounds potentially involved in the potato–V. dahliae interaction.
Materials and methods
Potato, V. dahliae and inoculation procedure
Potato tuber pieces of ‘Kennebec’ were planted in a mixture of sand and soil (1:1, v/v) supplemented with 50 g of NPKS fertilizer granules (16:20:0:16) for 5 weeks in a greenhouse (20/16 ± 2 °C day/night, 16 h photoperiod, light intensity of 350 μmol m−2 s−1). In parallel, two V. dahliae isolates of different aggressiveness (Vd1396-9, highly aggressive, and Vs06-14, weakly aggressive) were maintained on PDA for 2 weeks. Conidiospores were harvested from the growing cultures in sterilized distilled water and a concentration of 106 conidiospores mL−1 was used for potato inoculation using a root-dipping method (Alkher et al., Citation2009). Briefly, 5 mm of the root tip of each plant was cut to produce a wound to facilitate the infection. Thus, samples of roots, stems and leaves from three replicates of unwounded, non-inoculated plants at time zero, just before inoculation, were harvested and assayed for phenolics to determine the concentrations of these compounds in the plant under normal conditions. Then, data with the wounded non-inoculated control plants were used to subtract the effect of wounding to provide a baseline to compare the effect of inoculation by different isolates of V. dahliae on the accumulation of phenolics. The plants inoculated with either V. dahliae isolate Vd1396-9 or Vs06-14, along with wounded non-inoculated control plants, were transplanted into 16 cm-diameter clay pots filled with an autoclaved mixture of soil, sand, peat and perlite (4:4:4:1, v/v/v/v), received 5 g L−1 NPK (20:20:20) fertilizer and were moved into the greenhouse under the same conditions mentioned earlier. This experiment was repeated at least once. Samples of roots, stems and leaves were collected at 1, 3, 7 and 14 days after inoculation (dai) from three independent replicates for each time point. The root and stem of each plant, approximately 20- and 10-cm in length, respectively, and all the leaves on each plant were cut and put immediately in liquid nitrogen. The same number of samples was collected from the wounded non-inoculated control replicates. The samples were put immediately in liquid nitrogen and kept at −80 °C until used for the extraction of secondary metabolites.
Extraction of phenolics and high performance liquid chromatography (HPLC) analysis
Phenolic extraction and HPLC analysis were carried out as described by Daayf et al. (Citation2000). An amount of 0.5–0.75 g of fresh weight of each sample was ground in liquid nitrogen and used for phenolic extraction. An equal amount of 80% methanol (0.5–0.75 mL) was added to the sample. Then, samples were homogenized using a vortex for 10 s every 15 min for a total of 2 h. Samples were centrifuged at 9600 g for 15 min, and the supernatant was used for injection into an HPLC. The HPLC analysis was performed using a Waters 2695 separation module (Waters Corp.) equipped with a Waters 996 Photodiode Array detector and a 250 × 4 mm RP-18 (LiChrospher 100) column with a particle size of 5 μm. The solvents were acidified water (1 mL phosphoric acid L−1 of ultrapure water) (solvent A) and acetonitrile HPLC grade (solvent B) with an elution flow rate of 1 mL min−1. The gradient used was as follows: time (min)/%A/%B: 0/100/0, 5/95/5, 10/95/5, 14/90/10, 20/80/20, 23/80/20, 30/65/35, 35/65/35, 43/50/50, 48/25/75, 55/0/100 and 60/0/100. The injected volume was 50 μL for each sample.
Three biological replicates for each treatment/time point, for a total of 108 samples, were extracted and injected independently into the HPLC. Empower 2 Chromatography Data Software (Waters Corp.) was used to analyse the chromatograms. Retention time (RT) and absorbance (nm) spectra, and co-elution with commercial standards, were used for identification of the detected phenolics.
Individual phenolic compounds were quantified with standard curves. Standard curves of differentially induced compounds, i.e. chlorogenic acid, caffeic acid, ferulic acid and rutin (Sigma-Aldrich Canada Ltd.) were carried out at a concentration series of 1, 5, 10, 25, 50, 75 and 100 μg mL−1. Three replicates for each concentration were run in the HPLC. The standards were dissolved in 80% methanol and run under the same conditions as the extracted samples. Linear regressions were generated between compound concentration (independent variable) and peak areas (dependent variable). The equations obtained were used to calculate the concentration of each phenolic compound in the analysed samples.
Experimental design and statistical analysis
All of the wounded non-inoculated, Vs06-14 and Vd1396-9 inoculated plants were distributed in a random complete block design in the growth room. Content of the identified phenolic compounds were calculated based on the standard curves. Data were statistically analysed using SAS 9.1 software (SAS Institute Inc.). Differences among the means were compared using the least significant difference (LSD) test at P < 0.05.
Results
Although a large number of peaks representing several phenolics were detected, three main compounds (chlorogenic, caffeic and ferulic acids) showed differential variation in the roots, while two did in the stems (caffeic acid and rutin), and rutin was the only one that expressed variations in the leaves. Concentrations of the four different compounds were further compared in the three potato tissues in response to inoculation with Vd1396-9 (highly aggressive) and Vs06-14 (weakly aggressive) isolates of V. dahliae.
Differential phenolic accumulation in the roots
Chlorogenic acid was detected in potato roots of inoculated and control plants at concentrations ranging from 0.6 to 2.9 μg g−1 root fresh weight (). It increased in response to V. dahliae inoculation at 7 and 14 dai. Caffeic acid content was variable in roots of wounded non-inoculated control potato, and plants inoculated with Vs06-14 or Vd1396-9 (). A significant accumulation of caffeic acid was detected in potato roots inoculated with the highly aggressive isolate Vd1369-9.
Fig. 2. Caffeic acid content (μg g−1 fresh weight) in roots of potato ‘Kennebec’ control plants, and those inoculated with the highly (Vd1396-9) or the weakly (Vs06-14) aggressive V. dahliae isolates over four time points – 1, 3, 7 and 14 days after inoculation. Bars with the same letter(s) are not significantly different according to the least significant difference test at P < 0.05.
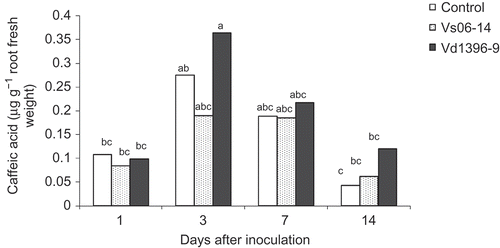
Fig. 1. Chlorogenic acid content (μg g−1 fresh weight) in roots of potato ‘Kennebec’ control plants, and those inoculated with the highly (Vd1396-9) or the weakly (Vs06-14) aggressive V. dahliae isolates over four time points – 1, 3, 7 and 14 days after inoculation. Bars with the same letter(s) are not significantly different according to the least significant difference test at P < 0.05.
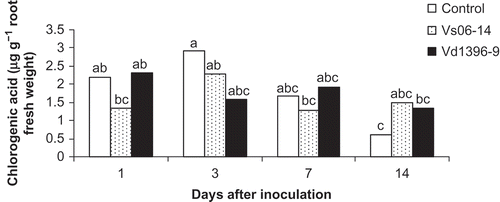
Ferulic acid's concentration gradually and steadily increased in the roots over time after inoculation with either the highly or the weakly aggressive isolate, whereas in the wounded non-inoculated control, such increase at 1 and 3 dai was followed by a significant decline at 14 dai (). At 14 dai, there was 1.78, 1.15 and 0.11 μg g−1 root fresh weight of ferulic acid in Vd1396-9-inoculated, Vs06-14-inoculated and wounded non-inoculated control plants, respectively, indicating significant differences between inoculated and non-inoculated roots.
Fig. 3. Ferulic acid content (μg g−1 fresh weight) in roots of potato ‘Kennebec’ control plants, and those inoculated with the highly (Vd1396-9) or the weakly (Vs06-14) aggressive V. dahliae isolates over four time points – 1, 3, 7 and 14 days after inoculation. Bars with the same letter(s) are not significantly different according to the least significant difference test at P < 0.05.
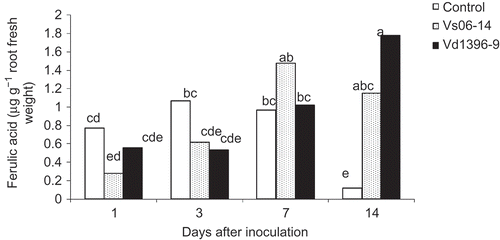
Differential phenolic accumulation in the stems
Caffeic acid and rutin were detected in potato stems after inoculation with either V. dahliae isolate. Caffeic acid was detected in both roots and stems. However, its concentration in stems was two-fold higher than in the roots, especially in plants inoculated with the highly aggressive isolate Vd1396-9 ( and ). The highest level of caffeic acid was found in the stems 14 dai with Vd1396-9 and was higher than all of the other tested treatments. The highest content of caffeic acid in roots (0.36 μg g−1 fresh weight) was detected 3 dai, whereas in stems (0.66 μg g−1 fresh weight), this occurred 14 dai ( and ). The flavonoid, rutin, was found in potato stems in all treatments (). Rutin accumulated in the stems as a response to infection of the plant roots. At 14 dai, its concentration in the stems was significantly higher in plants inoculated with the weakly aggressive isolate Vs06-14 (2.91 μg g−1 FW) as compared with the highly aggressive one Vd1396-9 (0.31 μg g−1 FW).
Fig. 5. Rutin content (μg.g−1 fresh weight) in stems of potato ‘Kennebec’ control plants, and those inoculated with the highly (Vd1396-9) or the weakly (Vs06-14) aggressive V. dahliae isolates over four time points – 1, 3, 7 and 14 days after inoculation. Bars with the same letter(s) are not significantly different according to the least significant difference test at P < 0.05.
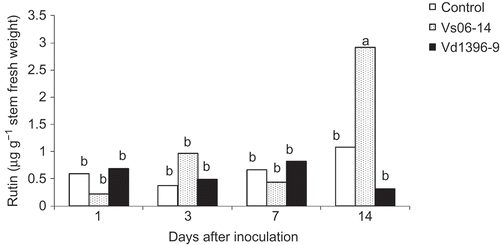
Fig. 4. Caffeic acid content (μg g−1 fresh weight) in stems of potato ‘Kennebec’ control plants, and those inoculated with the highly (Vd1396-9) or the weakly (Vs06-14) aggressive V. dahliae isolates over four time points – 1, 3, 7 and 14 days after inoculation. Bars with the same letter(s) are not significantly different according to the least significant difference test at P < 0.05.
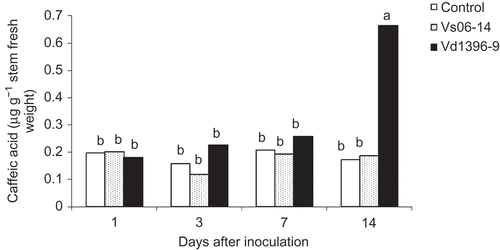
Differential phenolic accumulation in the leaves
Rutin was the only compound that showed differential accumulation in the leaves of potato plants inoculated with either V. dahliae isolate. Its concentration reached 74.7 μg g−1 fresh weight one day after inoculation with the highly aggressive isolate (), whereas its highest concentration in the stems was 2.91 μg g−1 fresh weight in plants inoculated with the weakly aggressive isolate (). Rutin showed differential accumulation in the leaves as a response to all treatments. Starting 1 dai, rutin content gradually decreased over time both in the wounded non-inoculated control and Vd1396-9-inoculated plants. However, there was no similar decrease in rutin content in the leaves of plants inoculated with the weakly aggressive isolate.
Fig. 6. Rutin content (μg g−1 fresh weight) in leaves of potato ‘Kennebec’ control plants, and those inoculated with the highly (Vd1396-9) or the weakly (Vs06-14) aggressive V. dahliae isolates over four time points – 1, 3, 7 and 14 days after inoculation. Bars with the same letter(s) are not significantly different according to the least significant difference test at P < 0.05.
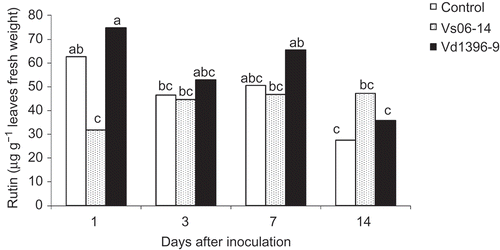
Discussion
This study showed variation in the accumulation of four main phenolic compounds in potato tissues in response to V. dahliae, with specific differences among tissues and inoculated isolates. Other secondary metabolites, such as sesquiterpenes, were not considered in this analysis, since they were extensively covered in previous studies. For this purpose, differential accumulation of phenolics was assessed in the roots, stems and leaves of potato plants inoculated with highly vs weakly aggressive isolates of this pathogen. The variation in accumulation of the four phenolics was observed in roots and stems as compared with only rutin in the leaves. This is not surprising, since V. dahliae is a soilborne pathogen that first infects the roots and colonizes the root cortex before moving and reproducing in the vascular system of the stem, eventually reaching the leaves.
Phenolic compounds are biosynthesized via the phenylpropanoid pathway, which primarily branches from the metabolism of the amino acid phenylalanine, followed by several biochemical reactions to produce cinnamic acid, caffeic acid, flavones and other phenolics (Hahlbrock & Scheel, Citation1989). The role of secondary metabolites in plant–microbe interactions is important in understanding host resistance/susceptibility to pathogens (Hammond-Kosack & Jones, Citation1996; Dixon, Citation2001), and phenolics were previously reported to be produced in plant defence against vascular wilt pathogens, such as Verticillium and Fusarium species in many crops e.g. cotton, olive and date palm (Daayf et al., Citation1997; El Hassni et al., Citation2004; Arfaoui et al., Citation2007; Báidez et al., Citation2007). The compounds that accumulated in different potato tissues in this study are known for their role in plant defences, including in cell wall reinforcement. However, given the ability of V. dahliae to metabolize rutin (El Hadrami et al., Citation2011), hydroxycinnamates are likely more involved in protection against this pathogen in potato, similar to other pathosystems (Daayf et al., 1997; Arfaoui et al., Citation2007; El Hadrami et al., Citation2010). Chlorogenic acid was also previously identified as part of a defence response in potato after infection with Helminthosporium carbonum R.R. Nelson (Kuć et al., Citation1956), Phytophthora infestans (Mont.) de Bary (Friend et al., Citation1973), Streptomyces scabies Lambert and Loria (Johnson & Schaal, Citation1952), and upon tuber physical injury (Johnson & Schaal, Citation1957). In the current study, chlorogenic acid accumulated faster in response to the highly aggressive isolate or wounding, and less with the weakly aggressive isolate, suggesting that it might be more related to tissue damage than response to the low aggressiveness of the pathogen.
Caffeic acid has also been reported to accumulate in potato in response to infection by other fungi (Kuć et al., Citation1956). Roots inoculated with Vd1396-9 had significantly higher levels of caffeic acid 3 dai than 1 dai and more than in wounded control or roots inoculated with the weakly aggressive isolate. This is interesting since caffeic acid is a precursor of chlorogenic acid, and concurs with concentration variations of the latter. Ferulic acid, another close hydroxycinnamic acid that differentially accumulated in this study, was reported as an inhibitor of mycelial growth, conidial germination and hydrolytic enzyme activity of the wilt fungus Fusarium oxysporum f. sp. niveum (E. F. Sm.) Snyd. & Hans (Wu et al., Citation2010), and might play the same role in this pathosystem given the phylogenetic relatedness of the two pathogens. In general, chlorogenic, caffeic and ferulic acids have been suggested to be factors in disease resistance in many plant–microbe interactions, including tobacco–V. dahliae (Sheppard & Peterson, Citation1976), canola–Leptosphaeria spp. (El Hadrami & Daayf, Citation2009), and saskatoon–Entomosporium mespili (DC.) Sacc. (Wolski et al., Citation2010).
The present data indicate that chlorogenic and ferulic acids are more active in potato roots in the 3 days following inoculation to deter the establishment of V. dahliae infection. Therefore, caffeic acid may be produced partly to strengthen the cell wall in response to the structural damage caused by the fungus. However, it may have also engaged in conjugating with quinic acid to form chlorogenic acid for a direct defence function. This would explain the specific variation observed in caffeic and chlorogenic acids in these tissues. The differences between root and stem accumulation of caffeic acid concur with the fact that V. dahliae first colonizes the vascular system of the roots before advancing to the stems. In lettuce, colonization of cortical tissues and entering the vascular system of roots by V. dahliae took 2 weeks after inoculation, while the vascular discoloration of taproot and crown area was observed 8–10 weeks after inoculation (Vallad & Subbarao, Citation2008). In our study, the increase in caffeic acid concentration was observed only in response to the highly aggressive isolate. It is not clear whether this was a response to the higher colonization by this isolate in the tissues, a response that is advantageous to the pathogen, or one indicating the lack of usage of caffeic acid into more effective defence compounds, i.e. chlorogenic acid. For rutin, on the other hand, it is increasingly evident that its generation in the leaves in response to infection is followed by its mobility to the stems where it is used against the weakly aggressive isolate, whereas it is degraded by the highly aggressive one. This is also confirmed by the accumulation profile of rutin in response to the highly aggressive isolate in the leaves 1 dai, with a decrease as the pathogen moves up in the plant, and the lowest accumulation in the stems where the pathogen resides the longest. The resulting differential accumulation of this flavonoid in response to the two isolates is in line with previous studies, where the highly aggressive isolates of V. dahliae were shown to detoxify rutin and overcome potato defence responses (El Hadrami et al., Citation2009a , Citation2009b ), providing them an advantage over the weakly aggressive ones. Similarly, many fungal plant pathogens were reported to detoxify secondary metabolites produced by plants (Bouarab et al., Citation2002; Pedras & Ahiahonu, Citation2005) and overcome their defences.
Phenolic compounds are important components in plant defence mechanisms to a wide range of biotic diseases and abiotic disorders. The present profiling of phenolics during the potato–V. dahliae interaction indicates that hydroxycinnamic acids may contribute to restricting the fungus from spreading in the early stages of root infection, probably in coordination with sesquiterpenes (Daayf et al., 1997). Once the fungus reaches the foliage, caffeic acid and rutin accumulate. The differences in the accumulation pattern of these phenolics in different plant parts in response to the highly vs weakly aggressive V. dahliae isolates indicates that both isolates induce phenolics during the early stage of infection in the host plant. Subsequently, a decrease in the amount of phenolics results in response to the highly aggressive isolate, confirming the ability of the latter to prevent some compounds from accruing to a high level. This may have occurred through one or more of several mechanisms, including pathogenicity factors that suppress plant defence signalling and gene expression, neutralizing the reactive oxygen species and signalling molecules, and subsequently inhibiting the production of phenolic compounds, or ensuring their degradation (El Hadrami et al., Citation2011).
Stress response regulator (SrrA) gene, thioredoxin and NADH-ubiquinone oxidoreductase 29.9 kDa subunit proteins were differentially expressed/produced in the highly aggressive Vd1396-9 isolate (El-Bebany et al., Citation2010, Citation2011). These three genes/proteins play a role in stress signal transduction and oxidative burst tolerance in yeast and several pathogenic fungi (Kim & Kim, Citation2006; Vargas-Perez et al., Citation2007; Fernández-Acero et al., Citation2009). This is in agreement with the ability of isolate Vd1396-9 to suppress phenolic accumulation and overcome plant defence responses, since ROS are important signal molecules in defence including the accumulation of phenolics. Such suppression in signalling plant defences may also help explain the differential down-regulation of SA signalling-related genes (Ha-PAL and Ha-NML1) in V. dahliae–sunflower interactions (Yao et al., Citation2011) where the same two V. dahliae isolates were used as in the present study. In fact, using the same two Vd1396-9 and Vs06-14 V. dahliae isolates was an advantage because we previously tested them using other approaches. However, using only two isolates may represent a limitation, which could be addressed in future research by extending the analysis to include more V. dahliae isolates.
Plants produce hundreds of defence-related secondary metabolites, monomers and polymers. However, in this study, only four phenolic compounds were quantified and used in the comparison. The extraction methods and analytical platforms used here did not detect all compounds, and not all detected compounds were used in the statistical analysis. In the interaction of potato with V. dahliae, the outcome depends on a complex combination of several levels of interaction, including structural and biochemical plant barriers, host and pathogen signalling, and plant response–pathogen adaptation events. Similar defence–counterdefence events involving phenolics and other secondary metabolites were previously reported to occur in a potato interaction with the late blight pathogen P. infestans (Wang et al., Citation2004, Citation2008), and in canola interaction with the blackleg pathogen L. maculans (El Hadrami et al., Citation2010). The results from this study may help to further elucidate a more accurate interpretation of host–pathogen interactions and set the basis for the development of better disease management strategies and sustainable crop protection.
Acknowledgements
We acknowledge funding from a Discovery Grant from the Natural Sciences and Engineering Research Council of Canada (NSERC) and a research grant from Manitoba Agri-Food Research & Development Initiative (ARDI) to Dr F. Daayf. Financial support was provided to A.F. El-Bebany by a PhD scholarship from the Ministry of Higher Education, Egypt.
References
- Alkher , H.A. , El Hadrami , A. , Rashid , K.Y. , Adam , L.R. and Daayf , F. 2009 . Cross-pathogenicity of Verticillium dahliae between potato and sunflower . Eur. J. Plant Pathol. , 124 : 505 – 519 .
- Arfaoui , A. , El Hadrami , A. , Mabrouk , Y. , Sifi , B. , Boudabous , A. , El Hadrami , I. , Daayf , F. and Cherif , M. 2007 . Treatment of chickpea with Rhizobium isolates enhances the expression of phenylpropanoid defense-related genes in response to infection by Fusarium oxysporum f. sp. ciceris . Plant Physiol. Biochem. , 45 : 470 – 479 .
- Báidez , A.G. , Gómez , P. , Del Río , J.A. and Ortuňo , A. 2007 . Dysfunctionality of the xylem in Olea europea L. plants associated with the infection process by Verticillium dahliae Kleb. – role of phenolic compounds in plant defense mechanism . J. Agric. Food Chem. , 55 : 3373 – 3377 .
- Bianchini , G.M. , Stipanovic , R.D. and Bell , A.A. 1999 . Induction of δ-cadinene synthase and sesquiterpenoid phytoalexins in cotton by Verticillium dahliae . J. Agric. Food Chem. , 47 : 4403 – 4406 .
- Bouarab , K. , Peart , J. , Baulcombe , D. and Osbourn , A. 2002 . A saponin-detoxifying enzyme mediates suppression of plant defences . Nature , 418 : 889 – 892 .
- Daayf , F. , Nicole , M. , Boher , B. , Pando , A. and Geiger , J.P. 1997 . Early vascular defense reactions of cotton roots infected with a defoliating mutant strain of Verticillium dahliae . Eur. J. Plant Pathol. , 103 : 125 – 136 .
- Daayf , F. , Ongena , M. , Boulanger , R. , El Hadrami , I. and Bélanger , R.R. 2000 . Induction of phenolic compounds in two cultivars of cucumber by treatment of healthy and powdery mildew-infected plants with extracts of Reynoutria sachalinensis . J. Chem. Ecol. , 26 : 1579 – 1593 .
- Derksen , H. , Badawi , M. , Henriquez , M.A. , Yao , Z. , El-Bebany , A.F. and Daayf , F. 2013 . Differential expression of potato defence genes associated with the salicylic acid defence signalling pathway in response to weakly and highly aggressive isolates of . Verticillium dahliae J. Phytopathol. , 161, 142–153
- Dixon , R.A. 2001 . Natural products and plant disease resistance . Nature , 411 : 843 – 847 .
- El-Bebany , A.F. , Rampitsch , C. and Daayf , F. 2010 . Proteomic analysis of the phytopathogenic soilborne fungus Verticillium dahliae reveals differential protein expression in isolates that differ in aggressiveness . Proteomics , 10 : 289 – 303 .
- El-Bebany , A.F. , Henriquez , M.A. , Badawi , M. , Adam , L.R. , El Hadrami , A. and Daayf , F. 2011 . Induction of pathogenicity-related candidate genes in Verticillium dahliae in response to elicitation with potato root extracts . Environ. Expt. Bot. , 72 : 251 – 257 .
- El Hadrami , A. and Daayf , F. 2009 . Priming canola resistance to blackleg with weakly aggressive isolates leads to an activation of hydroxycinnamates . Can. J. Plant Pathol. , 31 : 393 – 406 .
- El Hadrami , A. , El Hadrami , I. and Daayf , F. 2009a . “ Suppression of induced plant defense responses by fungal pathogens ” . In Molecular–Plant Microbe Interactions , Edited by: Bouarab , K. , Brisson , N. and Daayf , F. 231 – 268 . Wallingford , UK : CAB International .
- El Hadrami , A. , Adam , L.R. and Daayf , F. 2009b . “ Molecular and biochemical analyses of defenses and counter-defenses in the potato × Verticillium dahliae pathosystem ” . In Proceedings of the 10th International Verticillium Symposium , 16 – 20 . Corfu Island : Greece. International Verticillium Steering Committee . Nov
- El Hadrami , A. , Fernando , W.G.D. and Daayf , F. 2010 . Variations in relative humidity modulate Leptosphaeria sp. pathogenicity and interfere with canola mechanisms of defense . Eur. J. Plant Pathol. , 126 : 187 – 202 .
- El Hadrami , A. , Adam , L.R. and Daayf , F. 2011 . Biocontrol treatments confer protection against Verticillium dahliae infection of potato by inducing anti-microbial metabolites . Mol. Plant–Microbe Interact. , 24 : 328 – 335 .
- El Hassni , M. , J'Aiti , F. , Dihazi , A. , Ait Barka , E. , Daayf , F. and El Hadrami , I. 2004 . Enhancement of induced defense responses against Bayoud disease by treatment of date palm seedlings with a hypoaggressive Fusarium oxysporum isolate . J. Phytopathol. , 152 : 182 – 189 .
- El Hassni , M. , El Hadrami , A. , Daayf , F. , Chérif , M. , Ait Barka , E. and El Hadrami , I. 2007 . Biological control of Bayoud disease in date palm: selection of microorganisms inhibiting the causal agent and inducing defense reactions . Environ. Expt. Bot. , 59 : 224 – 234 .
- Fernández-Acero , F.J. , Colby , T. , Harzen , A. , Cantoral , J.M. and Schmidt , J. 2009 . Proteomic analysis of the phytopathogenic fungus Botrytis cinerea during cellulose degradation . Proteomics , 9 : 1 – 11 .
- Friend , J. , Reynolds , S.B. and Aveyard , M.A. 1973 . Phenylalanine ammonia lyase, chlorogenic acid and lignin in potato tuber tissue inoculated with Phytophthora infestans . Physiol. Plant Pathol. , 3 : 495 – 507 .
- Hahlbrock , K. and Scheel , D. 1989 . Physiology and molecular biology of phenylpropanoid metabolism . Annu. Rev. Plant Physiol. Plant Mol. Biol. , 40 : 347 – 369 .
- Hammond-Kosack , K.E. and Jones , J.D.G. 1996 . Resistance gene dependent plant defense responses . Plant Cell , 8 : 1773 – 1791 .
- Hückelhoven , R. 2007 . Cell wall-associated mechanisms of disease resistance and susceptibility . Annu. Rev. Phytopathol. , 45 : 101 – 127 .
- Johnson , D.A. and Dung , J.K.S. 2010 . Verticillium wilt of potato – the pathogen, disease and management . Can. J. Plant Pathol. , 32 : 58 – 67 .
- Johnson , G. and Schaal , L.A. 1952 . Relation of chlorogenic acid to scab resistance in potatoes . Science , 115 : 627 – 629 .
- Johnson , G. and Schaal , L.A. 1957 . Accumulation of phenolic substances and ascorbic acid in potato tuber tissue upon injury and their possible role in disease resistance . Am. Potato J. , 34 : 200 – 209 .
- Kim , J.-H. and Kim , D.-H. 2006 . Cloning and characterization of a thioredoxin gene, CpTrx1, from the chestnut blight fungus Cryphonectria parasitica . J. Microbiol. , 44 : 556 – 561 .
- Kuć , J. , Henze , R.E. , Ullstrup , A.J. and Quackenbush , F.W. 1956 . Chlorogenic and caffeic acids as fungistatic agents produced by potatoes in response to inoculation with Helminthosporium carbonum . J. Am. Chem. Soc. , 78 : 3123 – 3125 .
- Kunkel , B.N. and Brooks , D.M. 2002 . Cross talk between signaling pathways in pathogen defense . Curr. Opin. Plant Biol. , 5 : 325 – 331 .
- Osbourn , A.E. 1996 . Preformed antimicrobial compounds and plant defense against funga1 attack . Plant Cell , 8 : 1821 – 1831 .
- Pantelides , I.S. , Tjamos , S.E. and Paplomatas , E.J. 2010 . Ethylene perception via ETR1 is required in Arabidopsis infection by Verticillium dahliae . Mol. Plant Pathol. , 11 : 191 – 202 .
- Pedras , M.S.C. and Ahiahonu , P.W.K. 2005 . Metabolism and detoxification of phytoalexins and analogs by phytopathogenic fungi . Phytochemistry , 66 : 391 – 411 .
- Pegg , G.F. and Brady , B.L. 2002 . Verticillium Wilts , Wallingford , UK : CAB International .
- Pieterse , C.M.J. , Leon-Reyes , A. , Van der Ent , S. and Van Wees , S.C.M. 2009 . Networking by small-molecule hormones in plant immunity . Nature Chem. Biol. , 5 : 308 – 316 .
- Ratzinger , A. , Riediger , N. , von Tiedemann , A. and Karlovsky , P. 2009 . Salicylic acid and salicylic acid glucoside in xylem sap of Brassica napus infected with Verticillium longisporum . J. Plant Res. , 122 : 571 – 579 .
- Robert-Seilaniantz , A. , Grant , M. and Jones , J.D.G. 2011 . Hormone cross-talk in plant disease and defence – more than just JA/SA antagonism . Annu. Rev. Phytopathol. , 49 : 317 – 343 .
- Rowe , R.C. and Powelson , M.L. 2002 . Potato early dying: management challenges in a changing production environment . Plant Dis. , 86 : 1184 – 1193 .
- Sheppard , J.W. and Peterson , J.F. 1976 . Chlorogenic acid and Verticillium wilt of tobacco . Can. J. Plant Sci. , 56 : 157 – 160 .
- Shi , F.-M. , Yao , L.-L. , Pei , B.-L. , Zhou , Q. , Li , X.-L. , Li , Y. and Li , Y.-Z. 2009 . Cortical microtubule as a sensor and target of nitric oxide signal during the defence responses to Verticillium dahliae toxins in Arabidopsis . Plant Cell Environ. , 32 : 428 – 438 .
- Smit , F. and Dubery , I.A. 1997 . Cell wall reinforcement in cotton hypocotyls in response to a Verticillium dahliae elicitor . Phytochemistry , 44 : 811 – 815 .
- Uppal , A.K. , El Hadrami , A. , Adam , L.R. , Tenuta , M. and Daayf , F. 2007 . Pathogenic variability of Verticillium dahliae isolates from potato fields in Manitoba and screening of bacteria for their biocontrol . Can. J. Plant Pathol. , 29 : 141 – 152 .
- Uppal , A.K. , El Hadrami , A. , Adam , L.R. , Tenuta , M. and Daayf , F. 2008 . Biological control of potato Verticillium wilt under controlled and field conditions using selected bacterial antagonists and plant extracts . Biol. Control , 44 : 90 – 100 .
- Vallad , G.E. and Subbarao , K.V. 2008 . Colonization of resistant and susceptible lettuce cultivars by a green fluorescent protein-tagged isolate of Verticillium dahliae . Phytopathology , 98 : 871 – 885 .
- van Loon , L.C. , Rep , M. and Pieterse , C.M.J. 2006 . Significance of inducible defense-related proteins in infected plants . Annu. Rev. Phytopathol. , 44 : 135 – 162 .
- Vargas-Perez , I. , Sanchez , O. , Kawasaki , L. , Georgellis , D. and Aguirre , J. 2007 . Response regulators SrrA and SskA are central components of a phosphorelay system involved in stress signal transduction and asexual sporulation in Aspergillus nidulans . Eukary. Cell , 6 : 1570 – 1583 .
- Vlot , A.C. , Dempsey , D.A. and Klessig , D.F. 2009 . Salicylic acid, a multifaceted hormone to combat disease . Annu. Rev. Phytopathol. , 47 : 177 – 206 .
- Wang , X. , El Hadrami , A. , Adam , L.R. and Daayf , F. 2004 . US-1 and US-8 genotypes of Phytophthora infestans differentially affect local, proximal and distal gene expression of phenylalanine ammonia-lyase and 3-hydroxy, 3-methylglutaryl CoA reductase in potato leaves . Physiol. Mol. Plant Pathol. , 65 : 157 – 167 .
- Wang , X. , El Hadrami , A. , Adam , L.R. and Daayf , F. 2008 . Differential activation and suppression of potato defense responses by Phytophthora infestans isolates representing US-1 and US-8 genotypes . Plant Pathol. , 57 : 1026 – 1037 .
- Wilhelm , S. 1955 . Longevity of Verticillium wilt fungus in the laboratory and field . Phytopathology , 45 : 180 – 181 .
- Wolski , E.A. , Henriquez , M.A. , Adam , L.R. , Badawi , M. , Andreu , A.B. , El Hadrami , A. and Daayf , F. 2010 . Induction of defense genes and secondary metabolites in saskatoons (Amelanchier alnifolia Nutt.) in response to Entomosporium mespili using jasmonic acid and Canada milkvetch extracts . Environ. Expt. Bot. , 68 : 273 – 282 .
- Wu , H.-S. , Luo , J. , Raza , W. , Liu , Y.-X. , Gu , M. , Chen , G. , Hu , X.-F. , Wang , J.-H. , Mao , Z.-S. and Shen , Q.-R. 2010 . Effect of exogenously added ferulic acid on in vitro Fusarium oxysporum f. sp. niveum . Sci. Hortic. , 124 : 448 – 453 .
- Yao , Z. , Rashid , K.Y. , Adam , L.R. and Daayf , F. 2011 . Verticillium dahliae’s VdNEP acts both as a plant defense elicitor and a pathogenicity factor in the interaction with Helianthus annuus . Can. J. Plant Pathol. , 33 : 375 – 388 .