Abstract
Tan spot is an important foliar disease of wheat caused by Pyrenophora tritici-repentis. Eight races of this fungus have been identified based on their virulence on a wheat differential set. Previous surveys revealed that most isolates collected from the Canadian Prairies belong to races 1 and 2 of P. tritici-repentis and carry the ToxA gene. However, pathogen populations from Alberta, a major wheat-producing province, have not been characterized. In this study, the virulence of 45 isolates of P. tritici-repentis from Alberta was evaluated on six differential wheat genotypes. Race 1 was found to be most common, representing 62% of the isolates tested, while race 2 was the second most common (36% of isolates). A single isolate of race 3 was also found (2% of total), but no other races were identified. Polymerase chain reaction and Southern blot analyses with ToxA and ToxB-specific primers and probes were conducted to confirm the race designations of the tested isolates. The ToxA gene was identified in each isolate classified as race 1 or race 2, but not in the race 3 isolate. Sequencing of ToxA from 16 isolates collected in different parts of the province revealed that the sequences were identical. The ToxB gene was not found in isolates classified as races 1 or 2, but a ToxB homologue was identified in the race 3 isolate. These results confirm that the P. tritici-repentis populations in Alberta follow the toxin or inverse gene-for-gene model, and serve to validate the race-based classification system for this pathogen.
Résumé
La tache helminthosporienne est une grave maladie foliaire du blé causée par Pyrenophora tritici-repentis. Huit races de ce champignon ont été répertoriées en fonction de leur virulence à l’égard d'une série de lignées différentielles de blé. Des études antérieures ont révélé que la plupart des isolats collectés sur les Prairies canadiennes appartiennent aux races 1 et 2 de P. tritici-repentis et portent le gène ToxA. Toutefois, les populations pathogènes de l'Alberta, une des principales provinces productrices de blé, n'ont pas été caractérisées. Dans cette étude, la virulence de 45 isolats de P. tritici-repentis provenant d'Alberta a été évaluée sur 6 génotypes différentiels de blé. La race 1 était la plus courante, représentant 62 % des isolats testés, tandis que la race 2 était la deuxième plus courante (36 % des isolats). De plus, un seul isolat de race 3 a été trouvé (2 % du total), mais aucune autre race n'a été répertoriée. Des analyses par réaction en chaîne de la polymérase et transfert de Southern ont été effectuées avec des amorces et des sondes spécifiques des gènes ToxA et ToxB pour confirmer les races des isolats testés. Le gène ToxA a été identifié dans chaque isolat classifié dans les races 1 ou 2, mais pas dans l'isolat de race 3. Le séquençage du gène ToxA de 16 isolats collectés dans différentes régions de la province a démontré que les séquences étaient identiques. On n'a pas trouvé le gène ToxB dans les isolats classifiés dans les races 1 ou 2, mais on a identifié un gène homologue au gène ToxB dans la race 3. Ces résultats confirment que les populations albertaines de P. tritici-repentis sont conformes au modèle de la toxine, ou à l'image inversée du modèle gène-pour-gène, et servent à valider, pour cet agent pathogène, le système de classification basé sur la race.
Introduction
Tan spot is a destructive foliar disease of wheat caused by the ascomycete fungus Pyrenophora tritici-repentis (Died.) Drechs (anamorph: Drechslera tritici-repentis (Died.) Shoem.). In Canada, the USA and Australia, tan spot is considered a relatively new emerging disease, likely as a consequence of the cultivation of susceptible wheat cultivars and a change in agricultural practices from conventional to no-till, which retains greater levels of inoculum of this stubble-borne pathogen (Hosford, Citation1982; Lamari et al., Citation2005a ; Singh et al., Citation2007). In Canada, more than 90% of the wheat crop is grown on the Prairie provinces (Manitoba, Saskatchewan and Alberta). However, while populations of P. tritici-repentis from Saskatchewan and Manitoba have been fairly well characterized (Lamari et al., Citation1998, Citation2005a ; Singh et al., Citation2007), the population structure of the fungus in Alberta has not been studied. This represents a serious gap in knowledge, particularly since Alberta represents one of the most important wheat-growing regions in Canada.
The pathogenicity of P. tritici-repentis is mainly dependent on the production of its host-selective toxins. Three toxins have been identified so far and are involved in pathogenesis, including the necrosis-inducing toxin Ptr ToxA and the chlorosis-inducing toxins Ptr ToxB and Ptr ToxC (Lamari & Strelkov, Citation2010). Both Ptr ToxA and Ptr ToxB are ribosomally synthesized proteins. Ptr ToxA has a mass of 13.2 kDa and is encoded by the ToxA gene (Ballance et al., Citation1989; Tomás et al., Citation1990; Tuori et al., Citation1995; Ciuffetti et al., Citation1997). In P. tritici-repentis, ToxA is only found as a single copy in isolates that produce Ptr ToxA, and is entirely absent in non-producing isolates (Ballance et al., Citation1996; Ciuffetti et al., Citation1997; Aboukhaddour et al., Citation2009). This gene resides on homologous chromosomes in the different races (Aboukhaddour et al., Citation2009). Ptr ToxA serves as a pathogenicity factor for P. tritici-repentis, and transformation of a non-pathogenic isolate with ToxA is sufficient to render it pathogenic on toxin-sensitive wheat (Ciuffetti et al., Citation1997). Ptr ToxB is a 6.6 kDa protein (Strelkov et al., Citation1999) that causes chlorosis by a mechanism involving chlorophyll photo-oxidation (Strelkov et al., Citation1998; Kim et al., Citation2010). ToxB, the gene coding for Ptr ToxB, is found in multiple copies in races that possess Ptr ToxB activity (Strelkov et al., Citation2002; Martinez et al., Citation2004; Aboukhaddour et al., Citation2009). Homologues of this gene have also been reported in several races that lack Ptr ToxB activity (Strelkov & Lamari, Citation2003; Martinez et al., Citation2004; Strelkov et al., Citation2006) and in other ascomycete fungi that are non-pathogenic on wheat (Andrie et al., Citation2008). Ptr ToxC also causes chlorosis, but on different host genotypes than Ptr ToxB. Ptr ToxC has been described as a non-ionic, polar, low-molecular-mass molecule (Effertz et al., Citation2002), but awaits full biochemical characterization.
Single dominant and independently inherited genes in wheat control sensitivity to the Ptr toxins, with one gene for each toxin (Lamari & Bernier, Citation1989c , Citation1991; Faris et al., Citation1996; Gamba et al., Citation1998). Depending on the toxin(s) produced by specific isolates of P. tritici-repentis and the toxin sensitivity gene(s) present in the infected host, symptoms of tan spot can include tan necrosis and/or extensive chlorosis of the leaf tissue (Lamari & Strelkov, Citation2010). The differential development of symptoms in specific host genotypes allowed for the development of a race-based classification system for fungal isolates (Lamari et al., Citation1995). Eight races of P. tritici-repentis have been identified to date, based on their virulence on a set of differential wheat lines (Lamari et al., Citation2003). Isolates belonging to races 2, 3 and 5 produce a single toxin each (Ptr ToxA, Ptr ToxC or Ptr ToxB, respectively). Isolates belonging to races 1, 6, and 7 produce a combination of two toxins each (Ptr ToxA + Ptr ToxC; Ptr ToxB + Ptr ToxC; Ptr ToxA + Ptr ToxB, respectively). Race 8 isolates produce all three toxins, while race 4 isolates do not produce any known toxins and are non-pathogenic (Lamari & Strelkov, Citation2010).
Several reports on the race structure of P. tritici-repentis in different parts of the world have been published in recent years (Lamari et al., Citation1998, Citation2005b ; Ali & Francl, Citation2003; Friesen et al., Citation2005; Engle et al., Citation2006; Singh et al., Citation2007; Ali et al., Citation2010; Antoni et al., Citation2010; Leisova-Svobodova et al., Citation2010; Lepoint et al., Citation2010; Gamba et al., Citation2012). A common characteristic of P. tritici-repentis populations in Canada, the USA, South America, and Australia has been the predominance of Ptr ToxA-producing isolates from races 1 and 2, and an almost complete absence of Ptr ToxB-producing isolates (Lamari et al., Citation1998, Citation2005b ; Ali & Francl, Citation2003; Friesen et al., Citation2005; Engle et al., Citation2006; Singh et al., Citation2007; Antoni et al., Citation2010; Leisova-Svobodova et al., Citation2010; Gamba et al., Citation2012). However, in a few reports, apparent discrepancies have been noted between the symptoms induced by some isolates and the expected toxin-encoding genes in those same isolates. For example, in a recent analysis of P. tritici-repentis populations from Arkansas, USA (Ali et al., Citation2010), it was reported that 36 of 42 isolates tested lacked the ToxA and ToxB genes, and that 13 of these isolates did not fit the current classification system. In a separate analysis of a collection of P. tritici-repentis isolates with different global origins (Lepoint et al., Citation2010), it was reported that while the results generally supported the current race classification system, a few isolates possessed an ‘uncertain phenotype’, and some had an ‘atypical ToxA profile’ (in which a larger amplicon than expected was amplified with ToxA-specific primers).
In Alberta, an important winter and spring wheat-growing region in Canada, no information regarding the virulence or pathogenicity phenotypes of P. tritici-repentis populations is available. The objectives of this study were, therefore, to determine the race structure of P. tritici-repentis in this province, and to determine whether or not additional virulence phenotypes, not represented by the current race classification system, exist.
Table 1. Name, race classification and geographic origin of Pyrenophora tritici-repentis isolates collected from Alberta, Canada, and analysed in this study
Materials and methods
Fungal isolates
A survey of spring and winter wheat crops for the occurrence of tan spot was conducted in June 2010, in the central and southern regions of Alberta (). Leaves showing symptoms of tan spot were carefully cut and placed in paper envelopes, which were left to air dry at room temperature. The samples were brought back to the laboratory at the University of Alberta, where they were cut into 1–2 cm segments and placed in Petri dishes containing a piece of wet Whatman® No. 1 filter paper (Whatman International Ltd., Maidstone, UK). The dishes were placed under fluorescent light for 24 h at room temperature to induce the formation of conidiophores by P. tritici-repentis. The dishes were then transferred to the dark and incubated for another 24 h at 14 °C to induce the formation of conidia on the tips of the conidiophores (Lamari et al., Citation1995). Single conidia were transferred to individual 9-cm-diameter Petri dishes filled with V8-potato dextrose agar (V8-PDA) (Lamari & Bernier, Citation1989a ) and used to generate inoculum, as described below. A total of 320 single-spore isolates of P. tritici-repentis were obtained, with 267 of the isolates recovered from southern Alberta and 53 isolates from central Alberta. A subset of 45 isolates (33 from southern Alberta and 12 from central Alberta) was selected for further characterization ().
Inoculum preparation and bioassays
Conidia were produced on V8-PDA as previously described (Lamari & Bernier, Citation1989a ). Briefly, the cultured isolates of P. tritici-repentis were grown in darkness at room temperature for 7 days. Mycelial plugs (0.5 cm in diameter) were then excised from the colonies and transferred singly to 9-cm-diameter Petri dishes filled with 25 mL of V8-PDA. The cultures were incubated in darkness at room temperature until the colonies were 4 cm in diameter. The Petri dishes were then flooded with sterile distilled water and the mycelium flattened with the bottom of a sterilized glass tube. The water was decanted and the plates were incubated overnight under light at room temperature, followed by 24 h incubation in darkness at 14 °C to induce sporulation. The sporulating colonies were flooded with sterile distilled water, and the conidia gently dislodged with a sterile wire loop. The spore suspensions were collected and the conidial concentration was adjusted to approximately 3000 conidia mL−1 with sterile distilled water. Spore concentrations were measured with a Fuchs Rosenthal Counting Chamber (Hausser Scientific, Blue Bell, PA).
Wheat differential lines
Four hexaploid (‘Glenlea’, 6B662, 6B365 and ‘Salamouni’) and two tetraploid (‘Coulter’, 4B1149) wheat genotypes were included as a differential set. These six genotypes are effective for the differentiation of the eight currently known races of P. tritici-repentis (Lamari et al., Citation2003). Seeds of each genotype were sown in 10-cm-diameter plastic pots filled with Sunshine potting mix (W.R. Grace and Co., Fogelsville, PA) at a rate of six seeds per pot. One genotype was planted per pot, and all treatments were replicated twice. The seedlings were maintained in a growth cabinet at 20 °C/18 °C (day/night) with a 16 h photoperiod at 180 mmol m−2 s−1, until they were inoculated at the two- to three-leaf stage. Seedlings were rated 6 days after inoculation and were evaluated based on the development of necrosis or chlorosis or no symptoms.
Wheat seedlings were sprayed with the conidial suspension (3000 conidia mL−1 and one drop of Tween 20 [polyoxyethylene sorbitan monolaurate] 100 mL−1) until runoff using a sprayer connected to an air line. Immediately following inoculation, the seedlings were placed in darkness in a misting chamber (relative humidity ≥ 95%) for 24 h, with continuous wetness provided by an ultrasonic humidifier. Seedlings were then transferred to a growth cabinet and kept at 20 °C/18 °C (day/night) with a 16 h photoperiod (180 mmol m−2 s−1) and 60 % relative humidity. The seedlings were monitored daily for symptom development and assessed for tan spot reaction 6 days after inoculation on the five-point rating scale of Lamari & Bernier (Citation1989a ). An isolate classified as race 8 (TS93-71F) with a known infection phenotype (Lamari et al., Citation2003) was included as a control in all of the inoculations.
Genomic DNA extraction
The isolates of P. tritici-repentis were grown for 3 weeks in liquid Fries medium amended with 1.5% yeast extract (Dhingra & Sinclair, Citation1986). The mycelial mats were used for genomic DNA extraction. Briefly, 40 mg of lyophilized mycelium from each isolate was extracted with a Wizard® Genomic DNA Extraction Kit (Promega Corp, Madison, WI) following the manufacturer's protocol for plant material. This was followed by two extractions with phenol chloroform (1 : 1 v/v) followed by one extraction with chloroform. The DNA was quantified with a NanoDrop ND-1000 spectrophotometer (Thermo Scientific, Wilmington, DE).
PCR amplification of the ToxA And ToxB Genes
A ToxA sequence from GenBank (Accession No. AF004369-1) was used to design the ToxA-specific primers used in this study. Two primers, ToxA192 (5′-CGTCCGGCTACCTAGCAATA-3′) and ToxA1155 (5′-TTGTGCTCCTCCTTCTCGAT-3′), were designed to amplify a 964 bp product that included a portion of the 5′ promoter region, the entire open reading frame (ORF), and part of a 3′ transcribed, non-translated region (Ciuffetti et al., Citation1997; ). The ToxB-specific primers ToxB72 (5′-ATCAACGAAGCGGTTATTGC-3′) and ToxB240 (5′-AACAACGTCCTCCACTTTGC-3′) were based on a ToxB sequence from GenBank (Accession No. AY243461; ) and were designed to amplify a 196 bp product within the ORF. All of the primers were designed with Primer 3 software (Rozen & Skaletsky, Citation2000).
Fig. 2. Diagram of the ToxA and ToxB loci in Pyrenophora tritici-repentis. The arrows indicate the primer sites.
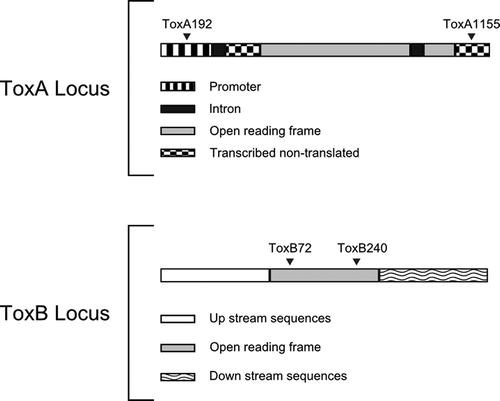
PCR analysis was performed on genomic DNA from the 45 selected P. tritici-repentis isolates to determine the presence or absence of the ToxA and ToxB genes. Reactions were performed with a Gene Amp® PCR System 9700 (Life Technologies Inc, Burlington, ON) in a 25 μL total volume containing 50 ng genomic DNA, 1 × PCR buffer (Invitrogen, Burlington, ON), 25 μm of each of the four dNTPs, 1.5 mm MgCl2, 10 pmol of each primer and 2 U Taq DNA polymerase (Invitrogen). Reaction conditions consisted of an initial denaturation step at 95 °C for 2 min, followed by 30 cycles at 94 °C for 50 s, 55 °C for 50 s and 70 °C for 50 s, and a final extension at 70 °C for 5 min. In order to verify that the correct products had been amplified, a selected number of amplicons were cut from the gel and purified with a Gel Purification Kit (Qiagen, Germany) and submitted for sequence analysis. The selected amplicons were chosen to represent all of the sampled geographical regions in Alberta (). Sequencing was performed by Macrogen USA (Rockville, MD) using Big Dye Terminator cycling conditions on a 3730XL DNA Analyser (Applied Biosystems, Foster City, CA).
Southern hybridization
A Southern blot analysis was performed to confirm the PCR results and to determine ToxA and ToxB copy number. Genomic DNA (10 μg) from five representative race 1 isolates (AB7-2, AB23-5, AB56-2, AB60-4, AB70-1), three representative race 2 isolates (AB28-2, AB34-10, AB41-3) and the single race 3 isolate (AB39-2) identified in the bioassays was digested to completion with the restriction enzymes XbaI and StuI (Invitrogen) for hybridization with the ToxA probe, or NheI and AvrII (Invitrogen) for hybridization with the ToxB probe, according to the manufacturer's instructions. In addition, genomic DNA samples from previously characterized isolates from the collection of Dr L. Lamari (University of Manitoba, Winnipeg, Canada) representing race 1 (isolate 86-124), race 3 (331-2), race 4 (49JA) and race 5 (Alg3-24) of P. tritici-repentis were also included as controls. With the exception of Alg3-24, which was collected from eastern Algeria (Lamari et al., Citation1998), these isolates were collected from different geographical regions in western Canada (Lamari & Bernier, Citation1989b ). The digested DNA was resolved by agarose gel electrophoresis and transferred to Hybond N+ membranes (Amersham Canada, Oakville, ON) with 20 × SSC (1 × SSC is 0.15 m NaCl plus 0.015 m sodium citrate) by capillary transfer overnight. The membranes were hybridized overnight at 62 °C in a solution consisting of 5 × SSC, 5 × Denhardt's reagent, 0.5% (w/v) sodium dodecyl sulfate (SDS), 20 μg of denaturated salmon sperm DNA mL−1 and 50 ng probe mL−1 (Sambrook et al., Citation1989). 32P-radiolabelled probes were generated by random primer labelling (Invitrogen) and consisted of a 743 bp ToxA cDNA fragment (Accession No. AF004369-1) or a 245 bp ToxB cDNA fragment (Accession No. AF483831-1) The membranes were washed at 65 °C for 15 min in 5 × SSC, 0.1% SDS, then twice for 10 min in 2.5 × SSC, 0.1% SDS, and once for 10 min in 1 × SSC, 0.1% SDS. The washed membranes were exposed to X-ray film (Kodak, USA) at −80 °C with an intensifying screen for 1 week.
Results
Race classification
Twenty-eight (62%) of the 45 isolates of P. tritici-repentis tested from Alberta were classified as race 1 in the bioassays (). These isolates caused the development of necrotic symptoms on the host differentials ‘Glenlea’ and ‘Coulter’ and chlorosis on 6B365, but were non-pathogenic/avirulent on 6B662, ‘Salamouni’, and 4B1149 (). Another 16 isolates (36%) were designated as race 2, since they could induce necrosis on ‘Glenlea’ and ‘Coulter’, but were non-pathogenic on 6B365 as well as on 6B662, ‘Salamouni’ and 4B1149 (). A single isolate (2%) was classified as race 3, and was able to cause chlorosis on wheat line 6B365 and necrosis on ‘Coulter’, but was non-pathogenic on ‘Glenlea’, 6B662, ‘Salamouni’ and 4B1149 (). The relative occurrence of races 1 and 2 was very similar in southern and central regions of Alberta, with race 1 representing 60% and 67% of the isolates collected from southern and central Alberta, respectively. Race 2 represented 36% and 33% of the isolates from the southern and central regions of the province, respectively. No other races aside from races 1, 2 and 3 were identified among the Alberta isolates. As expected, the race 8 isolate TS93-71F, which was included as a control in all of the bioassays, caused necrosis on ‘Glenlea’ and ‘Coulter’, chlorosis on ‘6B662’ and ‘6B365’, and was non-pathogenic on ‘Salamouni’ and 4B1149 ().
Fig. 3. Reaction of differential wheat genotypes to inoculation with representative isolates of Pyrenophora tritici-repentis collected from Alberta, Canada, or with a reference race 8 isolate (TS93-71F) included as a control. Race 1 isolates induced necrosis on ‘Glenlea’ and ‘Coulter’, and chlorosis on line 6B365; the other genotypes were resistant to this race. Race 2 isolates induced necrosis only on ‘Glenlea’ and ‘Coulter’. The race 3 isolate AB39-2 induced chlorosis on 6B365 and necrosis on ‘Coulter’. The race 8 isolate induced necrosis on ‘Glenlea’ and ‘Coulter’, and chlorosis on 6B662 and 6B365.
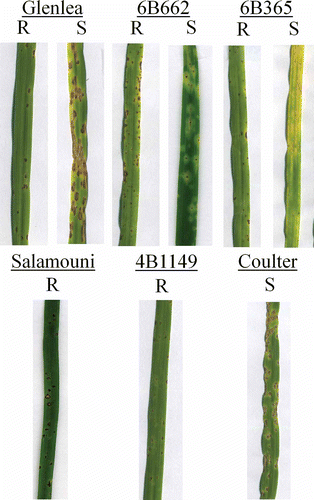
ToxA and ToxB in P. tritici-repentis Populations from Alberta
An amplicon corresponding to ToxA was obtained from genomic DNA of 44 (98%) of the 45 isolates tested (), consistent with the classification of those 44 isolates as race 1 or race 2 in the bioassays. The amplicons from 16 of these isolates were sequenced and found to be identical. No product was obtained when genomic DNA of the isolate AB39-2 was subjected to PCR with the ToxA-specific primers (ToxA192/ToxA1155), a finding consistent with the classification of this isolate as race 3 in the bioassays (). In contrast, while an amplification product could be obtained when genomic DNA of race 3 isolate AB39-2 was subjected to PCR with the ToxB-specific primers (ToxB72/ToxB240), no ToxB amplicon was produced in any of the other 44 isolates (). Sequencing of the product obtained from AB39-2 confirmed its identity as ToxB from P. tritici-repentis. The primers used to amplify ToxB in this study will amplify the forms of the gene found in races 3, 4 and 5; attempts to amplify ToxB with primers specific to another race 3 isolate, D308 (Strelkov et al., Citation2006), did not produce a detectable product.
Fig. 4. PCR amplification assays with primer sets specific for the ToxA or ToxB genes. The reactions from a subset of isolates of Pyrenophora tritici-repentis collected in Alberta, Canada, are shown. Genomic DNA was subjected to PCR analysis with ToxA-specific primers (ToxA192/ToxA1155) or ToxB-specific primers (ToxB72/ToxB240), and the reaction products were resolved in 1% agarose gels stained with Sybr Safe (Invitrogen). A single band of the expected 964 bp size was amplified with the ToxA-specific primers from isolates classified as races 1 or 2 (top panel), but no product was obtained from these isolates with the ToxB-specific primers (bottom panel). In contrast, a band of the expected 196 bp size was amplified with the ToxB-specific primers from the race 3 isolate AB39-2, but not with the ToxA-specific primers.
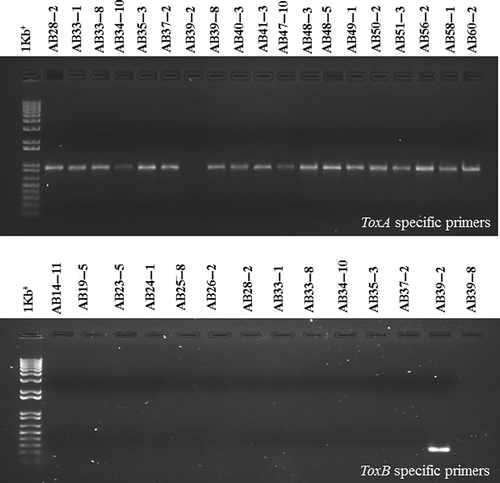
Southern blotting analysis with a ToxA cDNA clone as a probe revealed the presence of a single hybridizing fragment in genomic DNA from the races 1 and 2 isolates that had tested as ToxA+ in the PCR assay (). In contrast, no ToxA hybridization band was detected in genomic DNA digests from the race 3 isolate AB39-2, which was found to be ToxA− in the PCR assay. When using a ToxB cDNA clone as a probe, two hybridizing bands (one strong, one weak) were detected in genomic DNA from AB39-2 (), which was also the only isolate found to be ToxB+ in the PCR analysis. Similarly, the ToxB probe revealed two hybridizing bands (one strong and one weak) from genomic DNA digests of one of the reference isolates, 49JA (race 4), and multiple bands from another reference isolate, Alg3-24 (race 5) (). A single band hybridized with the ToxB probe in digests of the reference race 3 isolate, 331-2 (). No band hybridizing with the ToxB probe was detected in any of the isolates of races 1 and 2 (data not shown).
Fig. 5. Southern blot analysis with probes specific for the ToxA and ToxB genes. A subset of isolates of Pyrenophora tritici-repentis collected in Alberta, Canada, are shown, and represent race 1 (AB7-2, AB23-5, AB56-2, AB60-4, AB70-1), race 2 (AB28-2, AB34-10, AB41-3), and race 3 (AB39-2) of the fungus. Reference isolates 86-124 (race 2), 331-2 (race 3), 49JA (race 4) and Alg3-24 (race 5) are also included for comparison. Total genomic DNA was digested with XbaI and StuI or NheI and AvrII and hybridized, respectively, with a P32-labelled ToxA probe (top panel) or ToxB probe (bottom panel). A single fragment hybridized with the ToxA probe in DNA digests of isolates classified as races 1 or 2 (top), but not in digests of isolates classified as races 3, 4 or 5 (not shown). In contrast, single or multiple fragments hybridized with the ToxB probe in DNA digests of isolates classified as races 3, 4 or 5 (bottom), but not in digests of isolates classified as races 1 or 2 (not shown).
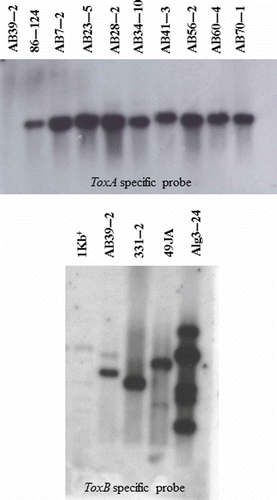
Discussion
Populations of P. tritici-repentis collected from central and southern Alberta were characterized for their virulence patterns on six differential wheat genotypes. The isolates recovered were predominantly classified as race 1 (62%) or race 2 (36%), with a single race 3 (2%) isolate found in southern Alberta. These results are consistent with previous studies, in which races 1 and 2 have been found to be predominant on wheat in Canada (Lamari et al., Citation2005a , Citation2005b ), the USA (Friesen et al., Citation2005; Engle et al., Citation2006), Australia (Antoni et al., Citation2010) and the southern cone region of South America (Gamba et al. Citation2012). In contrast, in limited surveys of North Africa, the Caucasus and the Fertile Crescent region, additional races have been more commonly identified (Lamari et al., Citation1995, Citation2005b ; Strelkov et al., Citation2002).
In the current study, the race classifications based on virulence phenotypes in the bioassays were consistent with the results of the PCR and Southern blot assays. All isolates that induced necrosis on ‘Glenlea’ were shown to possess the ToxA gene. The only isolate (AB39-2) that contained ToxB was identified as race 3 based on its virulence phenotype and lacked any homologue to ToxA. The PCR primers used for amplification of ToxB could not distinguish between the wild-type and other forms of the gene, but based on the absence of chlorosis on wheat line 6B662, it does not appear that isolate AB39-2 produced any active Ptr ToxB. The gene(s) responsible for production of Ptr ToxC are unknown, and therefore their presence could not be evaluated by PCR or Southern blot analysis. Nevertheless, the race 3 isolate identified was able to specifically cause chlorosis on the Ptr ToxC sensitive-wheat line 6B365, in a manner consistent with the expected symptoms ().
One of the most significant achievements in tan spot research was the development of an accurate and reliable system to identify ‘pathotypes’ of P. tritici-repentis, based on a qualitative assessment of symptoms (necrosis and chlorosis) induced on susceptible wheat genotypes (Lamari & Bernier, Citation1989c , Citation1991). This was followed by the development of a race classification system, in which isolates of the fungus are classified into races based on their pathogenicity on a host differential set (Lamari et al., Citation1995). The ability to recognize the distinct symptoms (necrosis/chlorosis) produced by isolates of P. tritici-repentis on wheat had significant implications for genetic and wheat breeding programmes, since they helped to explain the basis for susceptibility to the pathogen. These symptoms result from highly specific interactions between the toxins produced by P. tritici-repentis and putative receptors or targets in the host (Lamari & Strelkov, Citation2010).
While most studies have identified races 1 and 2 of P. tritici-repentis as predominant in the Americas and Australia, there have been a few reports suggesting the existence of novel races or atypical host reactions (Andrie et al., Citation2007; Ali et al., Citation2010; Lepoint et al., Citation2010). In an analysis of P. tritici-repentis from Arkansas (Ali et al., Citation2010), 63 of 93 isolates (67%) tested were classified as race 1, and 30 isolates (32%) were classified as race 3 based on their virulence phenotypes on the tan spot differential set. However, when a subset of 42 isolates was assessed for the presence of the ToxA and ToxB genes, 13 of 19 isolates that produced typical race 1 necrosis were found to lack the ToxA gene. Moreover, of six isolates found to possess both ToxA and ToxB, none could induce chlorosis on the Ptr ToxB-sensitive wheat line 6B662. These unusual findings seemed to indicate a very unique race composition in Arkansas, and led Ali et al. (Citation2010) to suggest that these isolates produced novel toxin(s) on wheat.
Discrepancies in the assessment of tan spot symptoms can occur; for example, in earlier research, cultivars considered to be resistant in certain studies (da Luz & Hosford, Citation1980; Hosford, Citation1982; Lee & Gough, Citation1984; Loughman & Deverall, Citation1986) were found to be susceptible in others (Gilchrist et al., Citation1984; Lamari & Bernier, Citation1989c ). Such discrepancies between individual reports may be explained by differences in the rating approach of researchers, and/or by differences in the fungal isolates used in particular studies. Nevertheless, the identification of such a large number of isolates exhibiting atypical reactions on the wheat differential set from a restricted geographical area such as Arkansas (Ali et al., Citation2010) is unusual. Wheat cultivation in Arkansas started relatively recently, in the early 1960s (Bacon et al., Citation2001), and the first report of tan spot in Arkansas is also recent (Hirrell et al., Citation1990). Other surveys in an area encompassing Arkansas and from the Great Plains (Ali & Francl, Citation2003; Friesen et al., Citation2005) to Ohio (Engle et al., Citation2006) revealed a clear dominance of races 1 and 2, with ‘atypical’ isolates being quite rare. Since the same winter wheat varieties are grown in Arkansas and Ohio, it seems unlikely that selection pressure imposed by the cropping of different host genotypes could explain the results. Additional studies, in which isolates from Arkansas are characterized further, may be necessary to resolve this apparent discrepancy. In contrast, the results of the current study are consistent with more than two decades of previous surveys in Canada, and with most surveys of other regions of the Americas and Australia, in that most isolates clearly fit the current race classification system (Lamari et al., Citation2003).
Of course, the ability to identify the full complement of races of a pathogen is limited by the effectiveness of the differential set, and it is likely that uncharacterized races (and additional host-specific toxins) of P. tritici-repentis exist (Lamari et al., Citation1995; Strelkov & Lamari., Citation2003). A combination of phenotypic and genotypic characterization has been recommended to strengthen race classification (Andrie et al., Citation2007), and indeed this approach was used to confirm the identification of races 7 and 8 of P. tritici-repentis (Lamari et al., Citation2003). However, to clarify the ambiguity of atypical race phenotypes or the unexpected presence or absence of toxin-encoding genes (Andrie et al., Citation2007; Ali et al., Citation2010; Lepoint et al., Citation2010), a combination of toxin infiltration and genetic studies can help to elucidate the relationship between a possible fungal toxin and its corresponding sensitivity gene in the host.
In the current study, the sequence of the ToxA gene from 16 different isolates was identical. Such uniformity is evidence for structural and functional conservation of ToxA within this population of P. tritici-repentis. Highly conserved sequences are thought to have functional value. Yet, ToxA, like ToxB, does not appear to control vital biological functions in the pathogen. The conidia of avirulent isolates are able to germinate, form appressoria and penetrate the host (Larez et al., Citation1986). The identical sequence of ToxA among the different isolates in this and previous studies (Friesen et al., Citation2006; Stukenbrock and McDonald, Citation2007; Miyamoto, Citation2008) is consistent with the theory of a single introduction of this gene into the genome of P. tritici-repentis (Friesen et al., Citation2006).
Isolate AB39-2, identified in this study, was classified as race 3 and confirmed to carry a ToxB sequence. Similarly, D308, another race 3 isolate described earlier, lacked Ptr ToxB activity, but also carried a homologue of the ToxB gene (Strelkov et al., Citation2006). A ToxB homologue was also reported in avirulent race 4 isolates of the fungus and in other ascomycetes that are not pathogenic on wheat (Strelkov et al., Citation2006; Andrie et al., Citation2008). The form of Ptr ToxB encoded by the race 4 isolates of P. tritici-repentis possesses little or no chlorosis-inducing activity (Kim & Strelkov, Citation2007; Figueroa Betts et al., Citation2011). Interestingly, while isolates D308 (race 3) and 90-2 (race 4) were previously shown to possess a single copy of the ToxB/ToxB-like gene (Strelkov et al., Citation2006), in this study isolates AB39-2 (race 3) and 49JA (race 4) contained at least two copies of the gene (). Further research is needed to clone and sequence ToxB from these isolates. Nevertheless, ToxB and its homologues appear to be very rare in Canadian populations of P. tritici-repentis, although the reason for this is not clear, since wheat cultivated in Canada is sensitive to Ptr ToxB (Lamari et al., Citation2005a ). The rarity or absence of Ptr ToxB-producing isolates has also been noted in P. tritici-repentis isolate collections from South America, the USA and Australia (Friesen et al., Citation2005; Lamari et al., Citation2005b ; Engle et al., Citation2006; Antoni et al., Citation2010; Gamba et al., Citation2012). In fact, most Ptr ToxB producing isolates have been collected from durum (tetraploid) wheat (Lamari et al., Citation2005b ). In North America, most cultivated wheat is hexaploid. The host-specificity imposed by the different toxins may have led to differences in the evolution of virulence in P. tritici-repentis (Aboukhaddour et al., Citation2011). The role of the ToxB gene in P. tritici-repentis isolates lacking Ptr ToxB activity is not yet fully understood, but it has been suggested to play additional roles in the basic pathogenic ability of the fungus, aside from its role in inducing chlorosis on toxin-sensitive wheat lines (Strelkov et al., Citation2006; Amaike et al., Citation2008; Aboukhaddour et al., Citation2012).
To our knowledge, this study represents the first detailed analysis of the population structure of P. tritici-repentis in Alberta. However, this pathogen is likely not new to the province. It was identified on wheat seeds from Alberta in samples collected from 1995 to 1997 (Turkington et al., Citation2002), and there is an early report of an uncharacterized leaf spot disease consisting of ‘small brown spots with light borders’ that affected wheat plants in southern Alberta in 1928 (McCurry, Citation1929). In Canada, the unintentional introduction of tan spot susceptible lines has been traced back to the year 1880 (Lamari et al., Citation2005a ). The sensitivity to Ptr ToxA and Ptr ToxB in Canadian wheat was introduced and is still maintained through breeding programmes that rely on limited genetic backgrounds (Lamari et al., Citation2005a ). This limited diversity in host germplasm, coupled with the current findings that most isolates of P. tritici-repentis from Alberta carry the ToxA gene, suggest that the fungus represents a threat to wheat in this province.
Acknowledgements
The authors would like to thank Ms Anh Van Ti Tran (University of Alberta) for help with some of the inoculations, Dr Denis Gaudet and Mr Byron Puchalski (Agriculture and Agri-Food Canada) for assistance with the collection of isolates, and Mr Derek Rennie for help in preparation of the figures. Financial support provided by the Natural Sciences and Engineering Research Council of Canada and the A.W. Henry Endowment Fund (University of Alberta) is gratefully acknowledged.
References
- Aboukhaddour , R. , Cloutier , S. , Ballance , G.M. and Lamari , L. 2009 . Genome characterization of Pyrenophora tritici-repentis isolates reveals high plasticity and independent chromosomal location of ToxA and ToxB . Mol. Plant Pathol. , 10 : 201 – 212 .
- Aboukhaddour , R. , Cloutier , S. and Strelkov , S.E. 2011 . Simple sequence repeats and diversity of globally distributed populations of Pyrenophora tritici-repentis . Can. J. Plant Pathol. , 33 : 339 – 399 .
- Aboukhaddour , R. , Kim , Y.M. and Strelkov , S.E. 2012 . RNA-mediated gene silencing of ToxB . Pyrenophora tritici-repentis. Mol. Plant Pathol. , 13 : 318 – 326 . in
- Ali , S. and Francl , L.J. 2003 . Population race structure of Pyrenophora tritici-repentis prevalent on wheat and noncereal grasses in the Great Plains . Plant Dis. , 87 : 418 – 422 .
- Ali , S. , Gurung , S. and Adhikari , T.B. 2010 . Identification and characterization of novel isolates of Pyrenophora tritici-repentis from Arkansas . Plant Dis , 94 : 229 – 235 .
- Amaike , S. , Ozga , J.A. , Basu , U. and Strelkov , S.E. 2008 . Quantification of ToxB gene expression and formation of appressoria by isolates of Pyrenophora tritici-repentis differing in pathogenicity . Plant Pathol. , 57 : 623 – 633 .
- Andrie , R.M. , Pandelova , I. and Ciuffetti , L.M. 2007 . A combination of phenotypic and genotypic characterization strengthen Pyrenophora tritici-repentis race identification . Phytopathology , 97 : 694 – 701 .
- Andrie , R.M. , Schoch , C.L. , Hedges , R. , Spatafora , J.W. and Ciuffetti , L.M. 2008 . Homologs of ToxB, a host-selective toxin gene from Pyrenophora tritici-repentis, are present in the genome of sister-species Pyrenophora bromi and other members of the Ascomycota . Fungal Gen. Biol , 45 : 363 – 377 .
- Antoni , E.A. , Rybak , K. , Tucker , M.P. , Hana , J.K. , Solomon , P.S. , Drenth , A. , Shankar , M. and Oliver , R.P. 2010 . Ubiqity of ToxA and absence of ToxB in Australian populations of Pyrenophora tritici-repentis . Austr. Plant Pathol , 39 : 63 – 68 .
- Bacon , R.K. , Kolb , F.L. and Murphy , J.P. 2001 . “ The U.S. soft red wheat pool ” . In The World Wheat Book , Edited by: Bonjean , A. and Angus , W. 469 – 478 . Cachan , France : Editions Lavoisier . InEds.pp.
- Ballance , G.M. , Lamari , L. and Bernier , C.C. 1989 . Purification and characterization of a host-selective necrosis toxin from Pyrenophora tritici-repentis . Physiol. Mol. Plant Pathol , 35 : 203 – 213 .
- Ballance , G.M. , Lamari , L. , Kowatsch , R. and Bernier , C.C. 1996 . Cloning expression and occurrence of the gene encoding the Ptr necrosis toxin from Pyrenophora tritici-repentis. Mol. Plant Pathol. On-line Publication/1996/1209/ballance
- Ciuffetti , L.M. , Tuori , R.P. and Gaventa , J.M. 1997 . A single gene encodes a selective toxin causal to the development of tan spot of wheat . Plant Cell , 9 : 135 – 144 .
- Da Luz , , W.C. and Hosford , R.M. Jr. 1980 . Twelve Pyrenophora trichostoma races for virulence to wheat in the Central Plains of North America . Phytopathology , 70 : 1193 – 1196 .
- Dhingra , O.D. and Sinclair , J.B. 1986 . Basic Plant Pathology Methods , Boca Raton , FL : CRC Press .
- Effertz , R.J. , Meinhardt , S.W. , Anderson , J.A. , Jordahl , J.G. and Francl , L.J. 2002 . Identification of a chlorosis-inducing toxin from Pyrenophora tritici-repentis and the chromosomal location of an insensitivity locus in wheat . Phytopathology , 92 : 527 – 533 .
- Engle , J.S. , Madden , L.V. and Lipps , P.E. 2006 . Distribution and pathogenic characterization of Pyrenophora tritici-repentis and Stagonospora nodorum in Ohio . Phytopathology , 96 : 1355 – 1362 .
- Faris , J.D. , Anderson , J.A. , Francl , L.J. and Jordahl , J.G. 1996 . Chromosomal location of a gene conditioning insensitivity in wheat to a necrosis-inducing culture filtrate of Pyrenophora tritici-repentis . Phytopathology , 86 : 459 – 463 .
- Figueroa Betts , M. , Manning , V.A. , Cardwell , K.B. , Pandelova , I. and Ciuffetti , L.M. 2011 . The importance of the N-terminus for activity of Ptr ToxB, a chlorosis-inducing host-selective toxin produced by Pyrenophora tritici-repentis . Physiol. Mol. Plant Pathol , 75 : 138 – 145 .
- Friesen , T.L. , Ali , S. , Klein , K.K. and Rasmussen , J.B. 2005 . Population genetic analysis of a global collection of Pyrenophora tritici-repentis, causal agent of tan spot of wheat . Phytopathology , 95 : 1144 – 1150 .
- Friesen , T.L. , Stukenbrock , E.H. , Liu , Z. , Meinhardt , S. , Ling , H. , Faris , J.D. , Rasmussen , J.B. , Solomon , P.S. , Mcdonald , B.A. and Oliver , R.P. 2006 . Emergence of a new disease as a result of interspecific virulence gene transfer . Nat. Genet. , 38 : 953 – 956 .
- Gamba , F.M. , Lamari , L. and Brûlé-Babel , A.L. 1998 . Inheritance of race specific necrotic and chlorotic reactions induced by Pyrenophora tritici-repentis in hexaploid wheats . Can. J. Plant Pathol. , 20 : 401 – 407 .
- Gamba , F.M. , Strelkov , S.E. and Lamari , L. 2012 . Virulence of Pyrenophora tritici-repentis in the Southern Cone Region of South America . Can. J. Plant Pathol. , 34 : 545 – 550 .
- Gilchrist , S.L. , Fuentes , F. and Islade Bauer , M.L. 1984 . Determinación de fuentes de resistencia contra Helminthosporium tritici-repentis bajo condiciones de campo e invernadero . Agrociencia , 56 : 95 – 105 .
- Hirrell , M.C. , Spradley , J.P. , Mitchell , J.K. and Wilson , E.W. 1990 . First report of tan spot caused by Drechslera tritici-repentis on winter wheat in Arkansas . Plant Dis , 74 : 252
- Hosford , R.M. Jr. 1982 . “ Tan spot developing knowledge 1902–1981, virulent races and wheat differentials, methodology, rating systems, other leaf diseases, literature ” . In Tan Spot of Wheat and Related Diseases Workshop , Edited by: Hosford , R.M. Jr. 1 – 24 . Fargo , ND : North Dakota Agricultural Experiment Station . InEd.pp.
- Kim , Y.M. and Strelkov , S.E. 2007 . Heterologous expression and activity of Ptr ToxB from virulent and avirulent isolates of Pyrenophora tritici-repentis . Can. J. Plant Pathol. , 29 : 232 – 242 .
- Kim , Y.M. , Bouras , N. , Kav , N.N.V. and Strelkov , S.E. 2010 . Inhibition of photosynthesis and modification of the wheat leaf proteome by Ptr ToxB: a host-specific toxin from the fungal pathogen Pyrenophora tritici-repentis . Proteomics , 10 : 2911 – 2926 .
- Lamari , L. and Bernier , C.C. 1989a . Evaluation of wheat lines and cultivars for reaction to tan spot (Pyrenophora tritici-repentis) based on lesion types . Can. J. Plant Pathol. , 11 : 49 – 56 .
- Lamari , L. and Bernier , C.C. 1989b . Virulence of isolates of Pyrenophora tritici-repentis on 11 wheat cultivars and cytology of the different host reactions . Can. J. Plant Pathol. , 11 : 284 – 290 .
- Lamari , L. and Bernier , C.C. 1989c . Toxin of Pyrenophora tritici-repentis: host-specificity, significance in disease, and inheritance of host-reaction . Phytopathology , 79 : 740 – 744 .
- Lamari , L. and Bernier , C.C. 1991 . Genetics of tan necrosis and extensive chlorosis in tan spot of wheat caused by Pyrenophora tritici-repentis . Phytopathology , 81 : 1092 – 1095 .
- Lamari , L. and Strelkov , S.E. 2010 . The wheat/Pyrenophora tritici-repentis interaction: progress towards an understanding of tan spot disease . Can. J. Plant Pathol. , 32 : 4 – 10 .
- Lamari , L. , Sayoud , R. , Boulif , M. and Bernier , C.C. 1995 . Identification of a new race in Pyrenophora tritici-repentis: implications for the current pathotype classification system . Can. J. Plant Pathol. , 17 : 312 – 318 .
- Lamari , L. , Gilbert , J. and Tekauz , A. 1998 . Race differentiation in Pyrenophora tritici-repentis and survey of physiologic variation in western Canada . Can. J. Plant Pathol. , 20 : 396 – 400 .
- Lamari , L. , Strelkov , S.E. , Yahyaoui , A. , Orabi , J. and Smith , R.B. 2003 . The identification of two new races of Pyrenophora tritici-repentis from the host centre of diversity confirms a one-to-one relationship in tan spot of wheat . Phytopathology , 93 : 391 – 396 .
- Lamari , L. , Mccallum , B.D. and De Pauw , R.M. 2005a . The forensic pathology of Canadian hard red spring wheats: the case of tan spot . Phytopathology , 95 : 144 – 152 .
- Lamari , L. , Strelkov , S.E. , Yahyaoui , A. , Amedov , M. , Saidov , M. , Djunusova , M. and Koichibayev , M. 2005b . Virulence of Pyrenophora tritici-repentis in the countries of the Silk Road . Can. J. Plant Pathol. , 27 : 383 – 388 .
- Larez , C.R. , Hosford , R.M. Jr. and Freeman , T.P. 1986 . Infection of wheat and oats by Pyrenophora tritici-repentis and initial characterization of resistance . Phytopathology , 76 : 931 – 938 .
- Lee , T.S. and Gough , F.J. 1984 . Inheritance of Septoria leaf blotch (S. tritici) and Pyrenophora tan spot (P. tritici-repentis) resistance in Triticum aestivum cv. Carifen 12 . Plant Dis , 68 : 848 – 851 .
- Leisova-Svobodova , L. , Hanzalova , A. and Kucera , L. 2010 . Expansion and variability of the Ptr ToxA gene in populations of Pyrenophora tritici-repentis and Pyrenophora teres . J Plant Pathol. , 92 : 729 – 735 .
- Lepoint , P. , Renard , M.E. , Legreve , A. , Duveiller , E. and Maraite , H. 2010 . Genetic diversity of the mating type and toxin production genes in Pyrenophora tritici-repentis . Phytopathology , 100 : 474 – 483 .
- Loughman , R. and Deverall , B.J. 1986 . Infection of resistant and susceptible cultivars of wheat by Pyrenophora tritici-repentis . Plant Pathol. , 35 : 443 – 450 .
- Martinez , J.P. , Oesch , N.W. and Ciuffetti , L.M. 2004 . Characterization of the multiple copy host selective toxin gene, ToxB, in pathogenic and nonpathogenic isolates of Pyrenophora tritici-repentis Mol . Plant-Microbe Inter , 17 : 467 – 474 .
- Miyamoto , M. 2008 . Comparison of ToxA and ToxB Genes of Pyrenophora tritici-repentis Isolates from Different Geographical Regions of the World. M.Sc. Thesis , Winnipeg , Canada. : University of Manitoba .
- Mccurry , J.B. 1929 . Report on the Prevalence of Plant Diseases in the Dominion of Canada for the years 1927 and 1928 , 12 Canada Department of Agriculture, Division of Botany, Dominion Experimental Farms . p.
- Rozen , S. and Skaletsky , H. 2000 . Primer3 on the WWW for general users and for biologist programmers . Meth. Mol. Biol. , 132 : 365 – 386 .
- Sambrook , J. , Fritsch , E.F. and Maniatis , T. 1989 . Molecular Cloning: A Laboratory Manual , 2nd , Cold Spring Harbor , NY : Cold Spring Harbor Laboratory .
- Singh , P.K. , Mergoum , M. and Hughes , G.R. 2007 . Variation in virulence to wheat in Pyrenophora tritici-repentis population from Saskatchewan, Canada, from 2000 to 2002 . Can. J. Plant Pathol. , 29 : 166 – 171 .
- Strelkov , S.E. and Lamari , L. 2003 . Host–parasite interactions in tan spot [Pyrenophora tritici-repentis] of wheat . Can. J. Plant Pathol. , 25 : 339 – 349 .
- Strelkov , S.E. , Lamari , L. and Ballance , G.M. 1998 . Induced chlorophyll degradation by a chlorosis toxin from Pyrenophora tritici-repentis . Can. J. Plant Pathol. , 20 : 428 – 435 .
- Strelkov , S.E. , Lamari , L. and Ballance , G.M. 1999 . Characterization of a host-specific protein toxin (Ptr ToxB) from Pyrenophora tritici-repentis . Mol. Plant-Microbe Inter. , 12 : 728 – 732 .
- Strelkov , S.E. , Lamari , L. , Sayoud , R. and Smith , R.B. 2002 . Comparative virulence of chlorosis-inducing races of Pyrenophora tritici-repentis . Can. J. Plant Pathol. , 24 : 29 – 35 .
- Strelkov , S.E. , Kowatsch , R. , Ballance , G.M. and Lamari , L. 2006 . Characterization of the ToxB gene from North African and Canadian isolates of Pyrenophora tritici-repentis . Physiol. Mol. Plant Pathol. , 67 : 164 – 170 .
- Stukenbrock , E.H. and Mcdonald , B.A. 2007 . Geographical variation and positive diversifying selection in the host-specific toxin SnToxA . Mol. Plant Pathol. , 8 : 321 – 332 .
- Tomás , A. , Feng , G.H. , Reeck , G.R. , Bockus , W.W. and Leach , J.E. 1990 . Purification of a cultivar-specific toxin from Pyrenophora tritici-repentis, causal agent of tan spot of wheat . Mol. Plant-Microbe Inter. , 3 : 221 – 224 .
- Tuori , R.P. , Wolpert , T.J. and Ciuffetti , L.M. 1995 . Purification and immunological characterization of toxic components from cultures of Pyrenophora tritici-repentis . Mol. Plant-Microbe Inter , 8 : 41 – 48 .
- Turkington , T.K. , Clear , R.M. , Burnett , P.A. , Patrick , S.K. , Orr , D.D. and Xi , K. 2002 . Fungal plant pathogens infecting barley and wheat seed from Alberta, 1995–1997 . Can. J. Plant Pathol. , 24 : 302 – 308 .