Abstract
Fungicides are the last line of defence in the armoury of an integrated disease management (IDM) approach. They do not create yield, but protect an inherent yield potential that the grower may realize in the absence of disease. In the field, securing effective disease control from fungicide applications is dependent upon the disease pressure and the effectiveness of the fungicide to control that disease. Globally, the same fungicide active ingredients are used against a similar range of fungal pathogens. However, in the presence of the pathogen, the level of economic response to fungicide applications is primarily driven by the prevailing environmental conditions and their interactions with crop development and the pathogen. Fungicides are canopy management tools that influence the size and duration of the green leaf area (GLA) of the crop. The total number of fungicide applications links to the length of the growing season and the disease risk in that period. For example, the top three leaves of a wheat crop canopy might warrant protection for approximately 120 days in an irrigated wheat crop on the Canterbury Plains of New Zealand, but only 60 days in the dry land wheat crops of the Victorian Mallee in Australia. Combining our knowledge of fungicide effect on the crop canopy with soil water and nutrient availability enables better matching of fungicide product, dose and timing to a specific disease risk. It also enables better use of crop physiology models, such as APSIM (Agricultural Production Systems Simulator), to assist with in-crop fungicide decisions. This paper reviews the role of fungicides, principally the triazoles (FRAC Group 3), strobilurins (Group 11) and SDHI’s succinate dehydrogenase inhibitors (Group 7), in cereal disease management. It explains (i) why applying foliar fungicide by plant development stage (as well as disease threshold) confers advantages when fungicide mode of action and on-farm logistics are taken into consideration; (ii) gives examples of how fungicide management strategies are adjusted in Australia and New Zealand to take account of environmental conditions; and (iii) explains the importance of green leaf retention (GLR) in the realization of an economic response from fungicides.
Résumé
Les fongicides constituent l’ultime ligne de défense dans l’arsenal d’une approche de gestion des maladies par lutte intégrée. Ils n’accroissent pas le rendement, mais protègent le rendement possible qu’un producteur pourrait obtenir en l’absence de maladies. Au champ, la lutte efficace contre les maladies découlant de l’application de fongicides dépend de la pression exercée par la maladie et de l’efficacité du fongicide à lutter contre cette maladie. Globalement, les mêmes ingrédients actifs d’un fongicide sont utilisés contre une gamme d’agents pathogènes fongiques similaires. Toutefois, en présence d’un agent pathogène, le niveau de réponse économique aux applications de fongicide est avant tout conditionné par les conditions courantes du milieu et leurs interactions avec le développement végétatif et l’agent pathogène. Les fongicides sont des outils de gestion du couvert végétal qui influencent la taille et la durée de la surface foliaire verte de la culture. Le nombre total d’applications de fongicide est lié à la longueur de la saison de croissance et au risque d’incidence de la maladie durant cette période. Par exemple, les trois feuilles supérieures du blé, en tant que couvert végétal, peuvent offrir une protection pendant environ 120 jours dans un champ irrigué dans les plaines de Canterbury en Nouvelle-Zélande, mais seulement 60 sur les terres arides du Mallee australien de la région de Victoria. La combinaison de nos connaissances sur les effets des fongicides sur le couvert végétal de la culture à l’eau du sol et à la disponibilité des nutriments permet de mieux cibler le produit ainsi que la quantité à utiliser et de synchroniser son application en fonction du risque possible d’une maladie particulière. Cela permet également une meilleure utilisation des modèles physiologiques des cultures comme APSIM (Agricultural Production System Simulator) afin d’aider dans le choix du fongicide pour la première application de postlevée. Cet article passe en revue le rôle des fongicides, principalement des triazoles (FRAC Groupe 3), des strobilurines (Groupe 11) et des inhibiteurs de la succinate déshydrogénase (Groupe 7) dans la gestion des maladies des céréales. Il explique pourquoi l’application d’un fongicide foliaire en fonction des stades de développement de la plante (et du seuil de la maladie) offre des avantages quand le mode d’action du fongicide et la logistique associée à la ferme sont pris en considération; il donne des exemples de l’adaptation des stratégies de gestion des fongicides utilisées en Australie et en Nouvelle-Zélande visant à prendre en compte les conditions de milieu; et il explique l’importance de la rétention de la surface foliaire verte afin d’obtenir une réponse économique à l’utilisation des fongicides.
Introduction
Modern systemic fungicides typified by the triazoles (FRAC Group 3) are a relatively recent introduction to cereal crop management, having been commercially introduced in the 1970s (Morton & Staub Citation2008). This group of fungicides is still the basis of cereal disease management strategies worldwide, particularly in Europe, North America, Australia and New Zealand. In cereal disease management programmes, they are primarily mixed with strobilurins (Group 11) which were introduced in 1992 (Francl Citation2001) and with the new generation pyrazole carboxamide (e.g. isopyrazam, bixafen) SDHI’s succinate dehydrogenase inhibitors (Group 7), introduced in 2010. Growers use these products as part of an integrated disease management (IDM) approach, whereby fungicides are used in combination with cultural control and genetic resistance in the host in order to secure economic cereal crop disease control (Wallwork Citation2000). Fungicides are primarily employed as seed treatments, in-furrow granular applications or foliar sprays onto the growing crop. This paper addresses the role of foliar fungicides in disease control in cereal crops and examines four key considerations in order to secure effective disease control in broad acre cereal crop husbandry: (i) understanding fungicide mode of action and the movement of fungicides in the plant; (ii) applying foliar fungicides by growth stage versus applying by disease threshold; (iii) developing strategies based on the importance of the plant structures being protected; (iv) the influence of environmental conditions in securing increased green leaf retention and an economic response from fungicide application. The review will focus primarily on disease control in wheat and barley crops and is based on research conducted in Australia, Europe and New Zealand.
Integrated disease management approach
Foliar fungicides are the last line of defence in an integrated disease management (IDM) approach for cereal disease control. These chemicals are used to cover weaknesses in the cultivar’s genetic resistance and cultural control methods that have already been employed (Brent & Hollomon Citation2007). The combination of plant host resistance, environment and pathogen can be represented in the concept of the disease triangle (), where disease pressure is governed by how these three factors interact (Stevens Citation1960). Rather than visualizing a static equilateral triangle, it is more realistic to picture a dynamic triangle that can change shape and size depending on the contribution of each individual factor, thus representing the range of disease susceptibility (Francl Citation2001). For example, the disease pressure represented by a triangle with a dry environment, a moderately resistant host, and strong pathogen virulence will look different than a triangle with a humid environment, a susceptible host and strong pathogen virulence.
Fig. 1. (Colour online) The disease triangle with vertices representing the contributing factors to plant disease (Francl Citation2001).
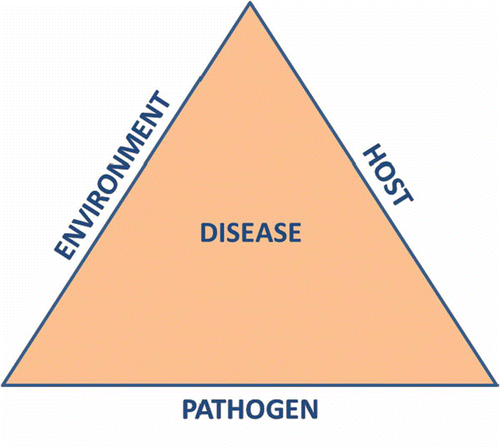
Host plant resistance
Developing a fungicide strategy for any field crop first depends on recognizing the strengths and weaknesses of the cultivar’s genetic resistance package. Assuming a cultivar has been selected with the optimum characteristics for yield and quality, cultivars vary enormously in their genetic predisposition to different diseases (Mundt et al. Citation2002). Selection of germplasm with greater resistance means not only that the grower is less dependent on fungicides (Viljanen-Rollinson et al. Citation2010) but also lessens the likelihood of disease spread occurring between cropping season for those diseases more dependent on a ‘green bridge’ (living host between cropping seasons). For example, yellow rust (Puccinia striiformis) in Australia relies on cereal volunteers to form part of the green bridge between cropping seasons (Wallwork Citation2000), but volunteers of a resistant cultivar will be less likely to host the pathogen, which can reduce spread between cropping seasons. Where genetic resistance is incomplete, fungicides can be used to cover weaknesses in the host plant resistance. Growers may, on occasion, adopt a policy whereby they opt to grow a cultivar that is susceptible to a disease that can be controlled with fungicides, but which has excellent resistance to a disease for which there are few agrichemical control options available. This was recently the case with northern grain growers in Australia opting for crown rot (Fusarium pseudograminearum) resistance at the expense of being more predisposed to yellow rust susceptibility in their cultivar choice because yellow rust could be effectively managed with fungicides (Daniel Citation2007). The relationship between the host crop plant and the pathogen is not necessarily stable since pathogens, through mutation or genetic recombination, can acquire new virulence on previously resistant cultivars (Mundt et al. Citation2002).
Pathogen
Pathogens vary in their epidemiology and thus the part of the plant that they damage. They also vary in their aggressiveness and their mode of dispersal (Wallwork Citation2000). All of these characteristics should be considered when choosing a disease management option. Pathogen populations have the ability to change and adapt genetically over time as their hosts and the environment change. Changes in the virulence of the pathogen or the incursion of an exotic pathotype can have large effects on the development of a disease epidemic. An example of an exotic incursion and pathotypes acquiring new virulence has been seen in yellow rust populations in Europe, Australia and New Zealand over the last decade (Wellings Citation2007, Citation2010).
Environment
Fungicide application, plant nutrition and cultural practices influence the environment for the pathogen and form part of the management interventions that come under the environment when considering the disease triangle. However, the non-management components of the ‘environment’ side of the disease triangle, such as temperature, moisture and humidity, tend to be more important drivers for disease development (Calhoun Citation1964). Given similar inoculum in the environment and the same genetic predisposition it is the environmental conditions, particularly those coinciding with stem elongation and grain fill, that most often dictate the economic response to fungicides (Wegulo et al. Citation2012).
Fungicide mode of action and movement in the plant
Controlling the fungus
In devising a fungicide strategy, it is important to recognize that the principal foliar fungicides all have different modes of action, which influence the performance of these fungicides in the field. The triazoles, which belong to Group 3 family of fungicides, are ergosterol biosynthesis inhibitors (EBI) that block the production of a key fungal cell membrane component (FRAC Citation2013). However, since the initial stages of spore germination are sustained by reserves of ergosterol in the fungal spore, triazole fungicides are not effective anti-sporulants compared with Group 11 strobilurin and Group 7 SDHI fungicide groups (Hanssler & Kuck Citation1987). In contrast, strobilurin and SDHI fungicide groups, which are the other principal fungicides employed for cereal disease control, are mitochondrial inhibitors that block respiration in the fungal cell and inhibit the ability of the spore to germinate (FRAC Citation2013). This mode of action makes the strobilurins and SDHI very effective protectant fungicides with variable curative activity depending on active ingredient (Bartlett et al. Citation2001; McKay et al. Citation2011). Strobilurins and SDHIs, whilst both being mitochondria inhibitors, are not cross-resistant, i.e. resistance to one family does not infer resistance to the other (FRAC Citation2013).
Fungicide movement on and in the plant
There are important differences in movement in and on the plant that influence the level of disease control provided by both the triazole and strobilurin fungicide groups (Bartlett et al. Citation2001, Citation2002). Triazole fungicides diffuse into the leaf surface and are transported in the xylem vessels. This movement of fungicide ensures the products have a degree of systemic activity within the plant that can be used to control fungal infection in the latent phase of development. Movement in the xylem vessels means that foliar fungicides only move in an upwards direction to the leaf tip. This limits the systemic activity of these fungicides to protection of only tissue that has emerged at the time of application. The triazole fungicides are therefore unable to move back down the leaf in order to provide protection of leaf tissue that had not emerged at the time of application (Bartlett et al. Citation2002). The uptake and movement of triazole fungicides into the cereal leaf varies amongst active ingredients found in the Group 3 family of fungicides (Kendall et al. Citation1994). These differences were demonstrated further by Bartlett () when the movement of three Group 3 fungicides (epoxiconazole, tebuconazole and flutriafol) were compared with a Group 11 fungicide, azoxystrobin. In the field, Group 3 fungicides with rapid uptake and movement can cause scorch symptoms if applied during warm conditions, whilst slower-translocating active ingredients tend to be more persistent but can be subject to poorer coverage depending on their water solubility and other physiochemical characteristics (Dahmen & Staub Citation1992).
Fig. 2. (Colour online) Study of foliar fungicide movement in the cereal leaf three days after application to the base of the cereal leaf (Poole Citation2009 – FAR).
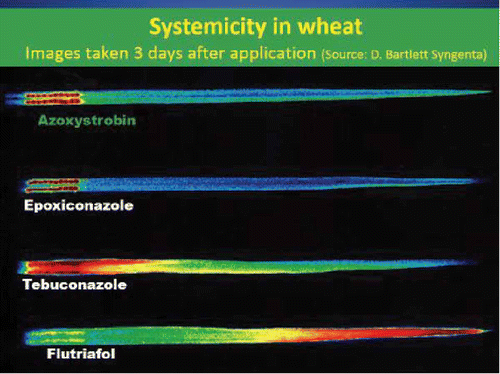
There is considerable variation in the movement of the strobilurin fungicides, with active ingredients varying in two key attributes – vapour activity on the surface of the leaf and systemic movement in the xylem (Bartlett et al. Citation2002) (). For example, picoxystrobin has both vapour activity and is xylem translocated while azoystrobin is xylem translocated but has no vapour activity on the surface. These different movement characteristics combined with differences in biological efficacy result in a group of fungicides that are very effective protectants, with the activity on the leaf surface being a key factor in their efficacy (Bartlett et al. Citation2001).
Fig. 3. (Colour online) Graphic illustrating the differences in movement of strobilurin fungicide in the cereal leaf (Bartlett et al. Citation2002).
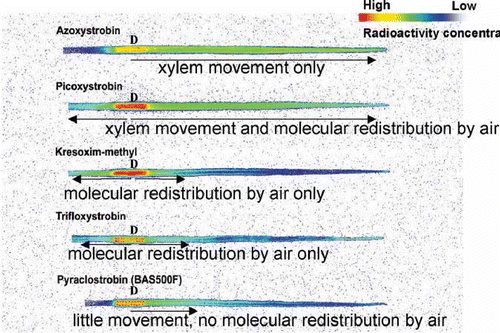
Less is known about the movement characteristics of the SDHIs; however, initial data released by agrichemical manufacturers would suggest that their primary activity is at the surface of the leaf, similar to the strobilurins, and that they have variable systemic characteristics, depending on the host and pathogen (McKay et al. Citation2011).
The consequence of these different modes of action and movement characteristics make fungicide mixtures of Group 3 with Group 7 and/or 11 fungicides not only very effective disease control options but ensure a stronger anti-resistance strategy, since strobilurins and SDHIs are at higher risk for pathogen resistance developing than are triazole fungicides (FRAC Citation2013). Fungicide resistance strategies in New Zealand require SDHIs to be mixed with a fungicide from a different group to reduce the risk for cereal crop application (McKay et al. Citation2011), and the same approach is suggested for the strobilurins (Beresford Citation2005).
Applying fungicide by plant growth stage versus disease threshold
Although triazole fungicides have a degree of systemic activity, this is limited by direction of movement. In addition, although these fungicides provide partial control of diseases in the latent phase, they are unable to return diseased plant tissue to its original state if infection is already visible. This was illustrated in England and Wales in the late 1980s, where the Group 3 fungicides provided preventative control of yellow rust and Septoria leaf spot (Septoria tritici), but were unable to eradicate the disease after expression (Cook et al. Citation1999). These limitations mean that whether these fungicides are used alone or in combination with strobilurins or SDHIs, applications to cereal crops work better as protectants rather than in a curative or eradicant mode. This raises the question as to whether the use of fungicides in cereal crops should be based on a disease threshold (where disease reaches a specified level before action is taken) or by application at a specific development stage of the crop. This concept was explored in some detail in an experiment conducted in New Zealand (Viljanen-Rollinson et al. Citation2010) using winter and spring wheat cultivars of differing disease resistance. Following artificial inoculation, fungicide programmes were either employed prophylactically at specific growth stages or on the basis of when disease was first observed. Work on autumn-sown wheat and yellow rust illustrated that where the cultivar was susceptible to disease, foliar fungicides were more effective when applied at specific growth stages during stem elongation (GS31 and 37) (Zadoks et al. Citation1974) than when disease was first noted (Viljanen-Rollinson et al. Citation2010). With a moderately resistant cultivar, where the adult plant resistance naturally slowed down disease development, results indicated less need to be prophylactic with fungicides use (Viljanen-Rollinson et al. Citation2010). This indicates that both approaches (prophylactic versus threshold) have a role in the use of foliar fungicides but that the application of threshold is likely to be more successful where cultivars already have a minimum level of genetic resistance (in this example, a moderately resistant rating) in order to slow down disease development. Where cultivars are moderately susceptible or susceptible to the disease, applications based on development stages of the crop are likely to work more effectively. This inevitably means that foliar fungicides could be applied prophylactically with no evidence of the disease at application time, while in other cases it would mean fungicide is applied to the key leaves at stem elongation with reference to the presence of disease on the leaves and those lower in the crop canopy. In a practical situation where growers apply the majority of fungicides by a specific development stage, fungicide product and rate would be dictated by genetic resistance of the cultivar, presence or absence of the disease on the lower leaves and knowledge of the ensuing weather conditions that may or may not be prevalent for disease development. In reality, when examining cereal crop production zones in Europe and New Zealand, growers tend to apply fungicides by crop development stage, whilst in zones where yields are lower and fungicide responses more variable, e.g. Australia, fungicides are employed more on a threshold basis, sometimes purely based on the presence of the disease in the crop.
Developing fungicide strategies based on the importance of the plant structure being protected
The limited nature of fungicide activity and movement in the plant means that foliar fungicides are better applied to plant tissue before it is heavily infected. For cereal crops, this requires fungicides to be applied to the plant structure (leaves, stems and heads) intended to be protected shortly after emergence and before appreciable infection destroys green leaf tissue. In many cereal crops, it is possible to detect foliar disease very early in the crop development stage (pre tillering in some cases), particularly if the disease is seed-borne, such as net form of net blotch (Pyrenophora teres f. teres) (Wallwork Citation2000). When foliar disease establishes in a crop at such an early stage, it is questionable whether foliar fungicides should be applied as multiple sprays aiming to protect new growth every 14–21 days. Whilst such an approach may give optimal disease control, it does not consider the economics or the pathogen resistance risks associated with multiple applications. As a consequence, fungicide strategies have been developed on the basis of applying foliar fungicide to the important parts of the crop canopy, recognizing that the cost effectiveness of fungicides in the field is strongly correlated to the protection of the uppermost leaves in the crop canopy (Hansen et al. Citation1994; Poole Citation2009b). For example, Hardwick et al. (Citation2001) found that the majority of farmers in Wales and England were applying fungicides at full ear emergence (GS59) prior to 1994, but have been timing their applications to flag leaf emergence (GS39) since 1994, realizing the importance of protecting the flag leaf from disease. Fungicide strategies have been developed in Europe, Australia and New Zealand that aim to protect the leaves that most contribute to the final grain yield.
Value of different crop canopy leaf layers
It has been calculated that the contribution of the top three leaves of an autumn-sown crop generate nearly 70% of the grain yield through their contribution of photosynthetic sugars (HGCA Citation2011). In winter wheat, the majority of the contribution of the top three leaves is due to the activity of the flag leaf (43%) with lower contributions from flag-1 (23%), flag-2 (7%) and flag-3 (3%). The activity of the green areas of the head contributes a further 22% to the final grain yield. The contribution of leaves gives a hierarchy of importance in terms of disease protection, which is why many fungicide strategies for wheat specify targeting the flag leaf. However, the likelihood of a yield response to fungicide application at this timing is still dependent on the presence of disease or subsequent infection resulting in disease. It is acknowledged that the hierarchy of leaf layer yield contributions is likely to vary with environmental conditions during grain fill, since in drier environments, the leaf sheaths and stem are proportionately more important (Thomas et al. Citation1989; Borwing & Fettell Citation2011).
In barley, the contribution of the different leaf layers is structured differently to wheat, mainly due to the small size of the flag leaf in barley (HGCA Citation2006). Therefore, in barley, the flag leaf sheath rather than the leaf laminae is an important contributor to yield and flag-1 is more important than the flag leaf, the reverse of the situation in wheat. The actual percentage of contribution of each leaf is more disputed than in wheat, but it is thought to be 5–12% flag leaf laminae, 25–35% flag leaf sheath, 20–40% flag-1, 10–15% flag-2, 5–10% flag-3, and 13–18% head (Biscoe et al. Citation1975; Poole Citation2009b). However, it is still the top four leaves of the crop canopy along with the flag leaf sheath and head that are responsible for the photosynthetic capacity that fills the grain and correlates to grain yield.
Emergence of key leaves in the crop canopy
If the aim is to protect the top three to four leaves of the crop canopy, and effective protection requires fungicide application before leaves become appreciably infected, it is obvious that one would be interested in knowing exactly when these top leaves emerge. It is easier to establish which is the flag leaf, since there are no more leaves to emerge; however, earlier in stem elongation, it becomes difficult to establish which is an emerging flag-1 versus emerging flag-2. The only certain way of ascertaining which leaf is emerging in the crop is to dissect the growing stem, and counting the number of leaves yet to emerge. However, there is an approximate relationship between nodal growth stage and leaf emergence (HGCA Citation2000). This work showed that flag-1 has emerged on the main stem when the third node (GS33) is fully defined on the main stem using the modifications carried out to Zadoks scale by Tottman (Citation1987). This study also stated that for the second and subsequent nodes to be defined as nodes, they must have a clear internode space of least 2 cm underneath the node. The leaf that emerges on the main stem at second node is flag-2 (Tottman Citation1987). This is an approximate relationship and is less well synchronized with very early and very late sowings. By knowing at which stem elongation growth stages the most important leaves in the crop canopy emerge, this can lead to the construction of a prophylactic fungicide programme based on target development stages which coincide with the emergence of the most important leaves. For example, in Europe, fungicides are centred on specific development stages – Timing 1 (T1) is applied at first to second node (GS31-32), Timing 2 (T2) is applied at the flag leaf emergence stage (GS39) and Timing 3 (T3) is applied at head emergence (GS59). In barley, the shorter growing season leads to commonly applied two spray programmes, with T1 at GS30-31 (slightly earlier than wheat) due to the greater importance of the lower leaves (e.g. flag-3 in barley). Timing 2 is applied from flag leaf emergence through to the end of booting (GS39-49).
These development stages are ideal application timings to protect the most important structures (leaves, stems and heads) in the upper crop canopy of wheat and barley; however, the actual level of yield response is still geared to the onset of disease and the conditions for disease and plant development.
Relationship between development stage and disease onset
Having established a clear hierarchy of importance for plant structures in the upper crop canopy and the need to apply fungicides at specific development stages in order to protect them, the overall level of yield response is still dependent on the time of disease onset. In NSW Australia, a program called ‘RustMan’ was released in the 1990s (Murray & Ellison Citation1988) which calculated the impact of yellow rust in wheat crops as well as the benefit of spraying based on the growth stage of the wheat at the time of disease onset. From a number of trials, the ‘RustMan’ program modelled the yield loss in cultivars of differing resistance ratings to the impact of disease at different development stages of onset. An example derived from the ‘RustMan’ model is given in .
Table 1. Influence of stripe rust onset in wheat and its influence on yield of cultivar with different levels of genetic resistance, derived from the ‘RustMan’ model.
Securing an economic response from fungicide application
This review has so far concentrated on how fungicides work and how timing the products at particular growth stages can be an effective way to employ these agrichemicals to secure an economic response. However, since fungicides need to be applied to the important leaf layers before appreciable disease is evident, fungicide strategies are only to be considered as insurance policies, the economic response of which is influenced by a number of factors. These include: (i) the influence of product and rate of application; (ii) the importance of green leaf retention in securing an economic response; and (iii) the influence of soil water availability and heat during grain fill. Most growers apply fungicides before they know whether the disease epidemic will develop and cause yield loss. That stated, fungicide strategies can be constructed with different ‘insurance premiums’ to match different levels of perceived risk. For example, a susceptible cultivar showing the target disease on the lowest leaves, under weather conditions conducive for that disease, demands greater input than a moderately resistant cultivar grown under conditions which are not conducive for the target disease. Adjusting the level of fungicide cover to combat a potential disease risk may involve a number of different adjustments to a planned fungicide strategy. Threshold versus growth stage based fungicide applications has already been addressed, but on many occasions the level of fungicide protection can be adjusted on the basis of the biological efficacy of the product and the rate of agrichemical employed.
Influence of product and rate
Different fungicide products have different levels of biological efficacy against different foliar diseases. In some cases, a number of products can be approved for control of a specific disease, but products differ in their effectiveness. Having an independent reference to the strengths and weaknesses of different active ingredients is a key piece of information for growers and advisers to have access to. Lower perceived disease risk could result in growers adopting either lower rates or cheaper products with less biological efficacy in order to reduce the level of expenditure to better match the likelihood of a yield response in the crop being treated. Recent work in Australia () testing biological efficacy of commercial fungicides against stem rust (Puccinia graminis var. tritici) highlighted the clear differences in the control of this disease amongst the different active ingredients (Poole & Wylie Citation2011). Testing the different levels of biological activity in fungicide active ingredients is a key function of a number of grower-directed levy organizations worldwide (e.g. Grains Research Development Corporation (GRDC), Foundation for Arable Research (FAR) in New Zealand and Home Grown Cereals Authority (HGCA) in the UK).
Fig. 4. (Colour online) Influence of fungicide active ingredient and rate on the incidence of stem rust in wheat – (mean of 4 Australian trials conducted in 2010) (Poole & Wylie Citation2011).
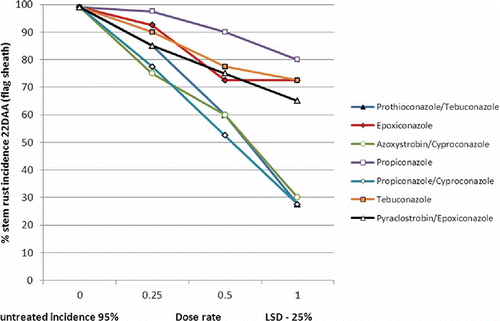
Importance of green leaf retention
Achieving disease control from fungicide application depends on the fungicide’s efficacy to control the disease that would have developed in the most important leaf layers (head or stem) of the crop canopy had the fungicide not been applied. However, securing an economic response from that application is dependent on the disease difference being translated into a green leaf difference during grain fill. The larger the differences in green leaf retention, the greater the differences in final grain yield. An illustration of differences in green leaf retention during grain fill is illustrated with reference to a barley trial conducted in South Australia in 2007 (). The correlation between green leaf retention and yield has been observed in a number of trials (Reynolds et al. Citation2009; Ali et al. Citation2010; Hunt & Poole Citation2010) and can be seen with reference to trial work in barley conducted by the author in 2009 () where every 1% reduction in green leaf area on flag-1 at GS80 correlated to a 20 kg/ha loss in yield. Therefore, while the basic advantage of fungicides is control of the disease-causing organisms that utilize carbohydrates in the plant that could otherwise be accumulated in the grains, the subsequent benefits are delayed senescence and longer green leaf area duration (Lorenz & Cothren Citation1989; Wu & von Tiedemann Citation2001; Cromey et al. Citation2004). Yield enhancements as a result of delayed senescence and a longer grain fill period were reported by Spiertz (Citation1977) who saw a growth rate of 204 to 230 kg/ha per day from milk-ripe to dough-ripe stage.
Fig. 5. (Colour online) Influence of no treatment (a), triadimenol seed treatment (b), two triazole fungicide sprays (c) and two triazole/strobilurin mixtures (d) on green leaf retention in barley during grain fill following leaf rust infection – cv ‘Gairdner Plus’ barley, South Australia 2007 (Poole Citation2009 – GRDC).
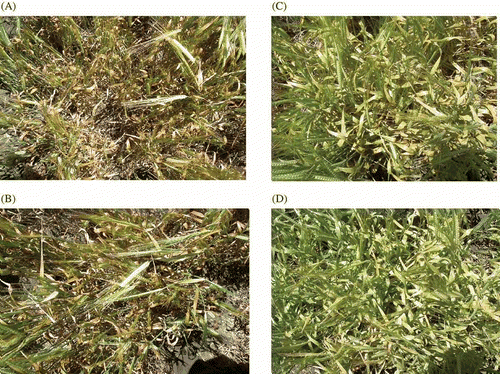
Fig. 6. (Colour online) Correlation between green leaf retention of flag-1 at GS80 and yield in trial work on barley (red data point – In furrow fungicide followed by 1 foliar spray, orange data point – best 2 spray foliar treatment) (Poole Citation2009 – GRDC).
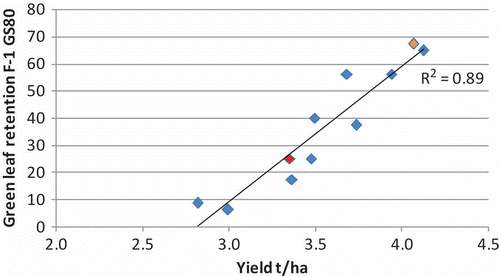
Influence of soil water availability and heat during grain fill
In many drier cereal production areas, it is water supply in the post-anthesis period that is the ultimate driver of green leaf retention or, more correctly, leaf area duration (LAD) (Hunt & Poole Citation2010). LAD is calculated by taking the sum of daily values of leaf area index from mid-flowering until maturity. Therefore, LAD is influenced by the disease control achieved with the fungicide programme, but it is the soil water availability during grain fill that is a stronger determinant of overall yield response and an economic response from the fungicide. As a result of low soil water availability post-anthesis, a grower in the Mallee region of Australia frequently grows crops with grain fill periods limited by drought and curtailed LAD. In such situations, not only is lower humidity restricting disease development but soil water availability is restricting the grain fill period and the need for fungicide protection. In many cases, the period of grain fill for wheat is considerably less than 30 days. In contrast, growers with irrigation in cooler regions of New Zealand have to protect a grain fill period that can cover between 45 to 60 days (assuming grain fill is typically 550 day degrees). Work by FAR conducted in spring sown barley in the 2012 season () illustrated that running the identical trial in a dryland and irrigated scenario influences the overall yield, the response to fungicide and the importance of different timings within the fungicide strategy (unpublished observations). Under dryland conditions, all four fungicide treatments gave a significant yield response but there was no significant advantage to two fungicide applications over one, and no advantage to a coded material (FAR 11/02) based on both triazole and SDHI chemistry combined. Where the equivalent trial was irrigated only 6 metres away with all inputs applied on the same dates, there was a significant advantage to two sprays, and when using two sprays, an advantage to FAR 11/02 over the triazole treatment alone (based on prothioconazole).
Green leaf retention calculator
As soil water is the overriding driver for green leaf retention rather than fungicide, the extent to which a fungicide can deliver a benefit is going to be determined by a combination of soil water availability post-anthesis and the extent to which the fungicide is able to preserve green leaf (largely dependent on the extent to which disease has been controlled). The Agricultural Production Systems Simulator (APSIM) model used extensively in Australia and in other parts of the world has been shown to adequately simulate LAD and corresponding yield under the influence of varying water supply (Hunt & Poole Citation2010). From this work a look-up table was derived which estimates likely yield loss based on expected yield in the absence of disease and expected disease effects on LAD (). Further development and validation of the green leaf retention calculator is required before it can be routinely used to better inform fungicide decisions; however, where the yield response associated with disease control is constrained by drier conditions during grain fill, e.g. dryland cropping in regions such as Australia, the concept of the green leaf retention calculator is an excellent starting point for matching fungicide expenditure to likelihood of return.
Table 2. Look-up table derived from APSIM estimating yield loss due reductions in LAD relative to an expected yield of a healthy crop.
Concluding remarks
By combining knowledge of how fungicides work and their movement in the plant with what structures within the plant are important to protect from disease, it is possible to construct a basic framework of development stage timings that could be used to best protect the crop. In wheat crops, there are typically three key fungicide timings for fungicide intervention: start of stem elongation (GS31-32), flag leaf emergence (GS39) and head emergence (GS59). In barley, fungicide strategies have been developed around two primary timings – start of stem elongation (GS30-31) and flag leaf to booting (GS39-49). Putting in place fungicide protection at these timings provides protection of the key leaf layers and head structure that are responsible for driving the assimilate for grain fill. However, under disease pressure, this timing information has to be integrated with knowledge of the ‘disease triangle’, whereby the genetic resistance of the host crop, influence of cultural control and environmental effects on plant growth and disease can be taken into account.
Acknowledgements
We would like to thank the Canadian Phytopathological Society (CPS) for the opportunity to speak at the 2013 conference.
References
- Ali MA, Hussain M, Khan MI, Ali Z, Zulkiffal M, Anwar J, Sabir W, Zeeshan M. 2010. Source-sink relationship between photosynthetic organs and grain yield attributes during grain filling stage in spring wheat (Triticum aestivum). Int J Agric Biol. 12:509–515.
- Bartlett DW, Clough JM, Godfrey CRA, Godwin JR, Hall AA, Heaney SP, Maund SJ. 2001. Understanding the strobilurin fungicides. Pestic Outlook. 12:143–148.
- Bartlett DW, Clough JM, Godwin JR, Hall AA, Hamer M, Parr-Dobrzanski B. 2002. The strobilurin fungicides. Pest Manag Sci.. 58:649–662.
- Beresford RM. 2005. Qo Inhibitor (QoI) management strategy. In: Martin NA, Beresford RM, Harrington, KC, editors. Pesticide resistance: prevention and management strategies. Hastings (New Zealand): Plant Protection Society.
- Biscoe PV, Gallagher JN, Littleton EJ, Monteith JL, Scott RK. 1975. Barley and its environment. IV. Sources of assimilate for the grain. J Appl Ecol. 12:295–318.
- Borwing A, Fettell N. 2011. The contribution of wheat plant components to yield and implications for management. In: Farm Business Update Proceedings Booklet; 2013. Barton (ACT): Grains Research and Development Corporation.
- Brent KJ, Hollomon DW. 2007. Fungicide resistance: the assessment of risk. FRAC Monographs No. 2 Rev. edit. Brussels (Belgium): Global Crop Protection Federation.
- Calhoun J. 1964. Presidential address: environment and plant disease. Trans Br Mycol Soc. 47:1–13.
- Cook RJ, Hims MJ, Vaughan TB. 1999. Effects of fungicide spray timing on winter wheat disease control. Plant Pathol. 48:33–50.
- Cromey MG, Butler RC, Mace MA, Cole ALJ. 2004. Effects of fungicides azoxystrobin and tebuconazole on Didymella exitialis, leaf senescence and grain yield in wheat. Crop Prot. 23:1019–1030.
- Dahmen H, Staub T. 1992. Biological characterization of uptake, translocation, and dissipation of difenoconazole (CGA 169374) in wheat, peanut, and tomato plants. Plant Dis. 76:523–526.
- Daniel R. 2007. Northern stripe rust management: an evaluation of timing and efficacy of foliar fungicides. GRDC Adviser Update Paper. Towoomba (QLD): Northern Grower Alliance.
- FRAC. 2013. Fungicide Resistance Action Committee (FRAC) Code list 2013: fungicides sorted by mode of action (including FRAC code numbering); [cited 2013 Aug 27]. Available from http://www.frac.info/publication/anhang/FRAC%20Code%20List%202013-final.pdf
- Francl LJ. 2001. The disease triangle: a plant pathological paradigm revisited. Plant Health Instruct. doi:10.1094/PHI-T-2001-0517–01.
- Hansen JG, Secher BJM, Jørgensen LN, Welling B. 1994. Thresholds for control of Septoria spp. in winter wheat based on precipitation and growth stage. Plant Pathol. 43:183–189.
- Hanssler G, Kuck KH. 1987. Microscopic studies on the effect of folicur on pathogenesis of brown rust of wheat (Puccinia recondite f. sp. tritici). Pflanzenschutz-Nachr Bayer. 40:153–180.
- Hardwick NV, Jones DR, Slough JE. 2001. Factors affecting diseases of winter wheat in England and Wales, 1989–98. Plant Pathol. 50:453–462.
- HGCA. 2000. The wheat disease management guide. Warwickshire: Home Grown Cereals Authority (HGCA Publication).
- HGCA. 2006. The barley growth guide. Warwickshire: Home Grown Cereals Authority (HGCA Publication).
- HGCA. 2011. The HGCA wheat disease management guide 2011. Warwickshire: Home Grown Cereals Authority (HGCA Publication).
- Hunt J, Poole N. 2010. Simulating leaf area duration to predict yield response to foliar fungicide in wheat and barley. In: Dove H, Culvenor RA, editors. Food security from sustainable agriculture. Proceedings of 15th Agronomy Conference; 2010 Nov; Lincoln, New Zealand.
- Kendall SJ, Hollomon DW, Stormonth DA. 1994. Towards the rational use of triazole mixtures for cereal disease control. In: Brighton Plant Protection Conference, Pests and Diseases; 1994 Nov 21–24; Brighton, UK, 2; p. 549–556.
- Lorenz EJ, Cothren JT. 1989. Photosynthesis and yield of wheat (Triticum aestivum) treated with fungicides in a disease-free environment. Plant Dis. 73:25–27.
- McKay AH, Hagerty GC, Follas GB, Moore MS, Christie MS, Beresford RM. 2011. Succinate dehydrogenase inhibitor (SDHI) fungicide resistance prevention strategy. N Z Plant Protec. 64:119–124.
- Morton V, Staub T. 2008. A short history of fungicides. APSnet Features. St. Paul (MN): American Phytopathological Society (US). doi:10.1094/APSnetFeature–2008–0308.
- Mundt CC, Cowger C, Garrett KA. 2002. Relevance of integrated disease management to resistance durability. Euphytica. 124:245–252.
- Murray GM, Ellison P. 1988. Use of Rustman, a decision support system for spraying wheat for control of stripe rust. In: The Impact of Computer-based Information Systems on Pasture and Crop Productivity, Standing Committee on Agriculture Workshop; 1988 Aug 15–17; Melbourne, VIC, Australia.
- Poole N, Wylie T. 2011. Stem rust control in wheat- 2010 trials review. GRDC Adviser Update Paper. Towoomba (QLD): Northern Grower Alliance.
- Poole NF. 2009a. Disease management & canopy canopies: what are the interactions?. Barton (ACT): Grains Research Development Corporation. (GRDC Publication).
- Poole NF. 2009b. Cereal growth stages: the link to disease management. Templeton (New Zealand): Foundation for Arable Research. FAR Focus Issue no. 2
- Reynolds M, Foules MJ, Slafer GA, Berry P, Parry MA, Snape JW, Angus WJ. 2009. Raising wheat potential. J Exp Bot. 60:1899–1918.
- Spiertz JHJ. 1977. The influence of temperature and light intensity on grain growth in relation to the carbohydrate and nitrogen economy of the wheat plant. Eur J Agron. 25:182–197.
- Stevens RB. 1960. Cultural practices in disease control. In: Horsfall JG, Dimond AE, editors. Plant pathology, an advanced treatise, Vol. 3. New York: Academic Press; p. 357–429.
- Thomas MR, Cook RJ, King JE. 1989. Factors affecting development of Septoria tritici in winter wheat and its effect on yield. Plant Pathol. 38:246–257.
- Tottman DR. 1987. Growth stage identification key, cereals. Bracknell: British Crop Protection Council.
- Viljanen-Rollinson SLH, Marroni MV, Butler RC. 2010. Benefits from plant resistance in reducing reliance on fungicides in cereal disease management. N Z Plant Prot. 63:145–150.
- Wallwork H. 2000. Cereal leaf and stem diseases. Kingston (ACT): Grains Research and Development Corporation.
- Wegulo S, Stevens J, Zwingman M, Baenziger PS. 2012. Yield response to foliar fungicide application in winter wheat. In: Dhanasekaran D, editor. Plant and animal diseases. Rijeka (Croatia): InTech; p. 227–244.
- Wellings C. 2007. Puccinia striiformis in Australia: a review of the incursion, evolution, and adaptation of stripe rust in the period 1979-2006. Aust J Agric Res. 58:567–575.
- Wellings C. 2010. Global status of stripe rust. In Proceedings of BGRI2010 Technical Workshop; 2010 May 30–31; St. Petersburg (Russia).
- Wu YX, von Tiedemann A. 2001. Physiological effects of azoxystrobin and epoxiconazole on senescence and the oxidative status of wheat. Pestic Biochem Phys. 71:1–10.
- Zadoks JC, Chang TT, Konzak CF. 1974. A decimal code for the growth stages of cereals. Weed Res. 14:415–421.