Abstract
Plasmodiophora brassicae, the obligate biotrophic plant pathogen causing clubroot of Brassica crops, has been present in Australia since the 1890s. The disease spread rapidly in vegetable Brassica crops, causing widespread losses during the 1980s and 1990s due to the increasing intensity of Brassica production and the extensive use of transplants. In response to the escalating problems caused by this disease in vegetable Brassica crops, a survey and pilot studies were initiated in 1993. This grew to become a coordinated national programme delivering clubroot research, development and extension to the Brassica industry until 2010. The key outcome of the programme was reduced crop losses through more effective and sustainable management of clubroot. This was achieved by the development and implementation of integrated, disease management strategies involving the use of good farm and nursery hygiene, early disease detection, application of lime (as calcium oxide) to maintain soil alkalinity, use of calcium and boron fertilizers, and strategic application of fungicides. Successful use of these integrated strategies reduced industry reliance on soil fumigants for disease control. This review details the history and impact of clubroot in Australia and research efforts directed towards its control.
Résumé
Plasmodiophora brassicae, l’agent pathogène biotrophique obligatoire des plantes qui cause la hernie dans les cultures du genre Brassica, est connu en Australie depuis les années 1980. La maladie s’est répandue rapidement chez les légumes de la famille des crucifères à cause de l’intensification de la production des plantes appartenant à ce genre et de l’utilisation à grande échelle de plants repiqués, ce qui a engendré des pertes considérables dans les années 1980 et 1990. En 1993, à la suite des problèmes sans cesse croissants causés par la hernie chez ces légumes, une étude et des projets pilotes ont été lancés. Cette initiative s’est transformée en programme national coordonné qui a permis de transmettre aux différents acteurs de l’industrie les résultats vulgarisés des recherches appliquées menées sur la hernie, et ce, jusqu’en 2010. Une des principales conséquences du programme a été la réduction des pertes par une gestion plus efficace et durable de la maladie. Cela a été accompli en développant et en appliquant des stratégies de lutte intégrée ayant recours à de bonnes pratiques d’hygiène tant à la ferme qu’à la pépinière, à la détection hâtive de la maladie, au chaulage (avec de l’oxyde de calcium) pour maintenir l’alcalinité des sols, à l’utilisation de fertilisants à base de calcium et de bore ainsi qu’à des applications stratégiques de fongicides. Les succès obtenus grâce à ces stratégies ont poussé les producteurs à délaisser les fumigants de sol. Cet article raconte en détail l’histoire et les répercussions de la hernie en Australie ainsi que les efforts consentis pour l’éradiquer.
Introduction
The Australian horticultural Brassica industry produces in excess of AU $207 million worth of produce annually (gross value for 2008/2009; Ausveg Citation2012). This figure consists of production data for Brassica oleracea L. vars. italica (broccoli 48.9%), botrytis (cauliflower 24.0%), capitata (cabbage 21.3%) and gemmifera (Brussels sprouts 5.7%), but does not include the many Asian vegetable Brassica crops for which accurate statistical data are difficult to obtain. In Australia, these vegetables tend to be grouped with many other minor crops as ‘Asian vegetables’ with a farm gate value of production in 2008/2009 of AU $69 million. More than one-third, 77 808 t or 37.5%, of Australia’s Brassica vegetables are produced in Victoria, 23.9% in Queensland and 15.9% in New South Wales (ABS Citation2007).
There are four main areas of production in Victoria; Werribee South, a traditional market gardening region on the western outskirts of metropolitan Melbourne, South Eastern Melbourne between Dandenong and Rosebud, East Gippsland around the floodplains of the Lindenow Valley and, more recently, Sunraysia, Northern Victoria. The regions which border metropolitan Melbourne (Werribee South and South Eastern Melbourne) are amongst the most intensive and productive regions for brassicas in Australia. Land holdings are small and Brassica vegetables are grown on raised beds under fixed irrigation. Generally, lettuce (Lactuca sativa L.), onions (Allium cepa L.) and fennel (Foeniculum vulgare Mill.) (Werribee South) or lettuce, celery (Apium graveolens (Mill.) Pers.), leeks (Allium ampeloprasum L.), carrots (Daucus carota subsp. sativus (Hoffm.) Schübl. & G. Martens), parsnips (Pastinaca sativa L.), potatoes (Solanum tuberosum L.) and other minor crops (South Eastern Melbourne) are grown in rotation with the Brassica vegetables. Often three different rotation crops will be grown in the same soil in a year; however, brassica crops are generally only sown on the same land once in any particular year. Increasingly, these areas, like those around the metropolitan outskirts of most capital cities, are coming under pressure as Australia’s cities expand and the suburbs move further out into traditional farming land.
Clubroot was first reported in Australia in the early 1890s (McAlpine Citation1891). It is most likely to have been brought into the country with the early settlers as diseased planting material (Watson & Baker Citation1969), although fodder or grazing animals represent an alternative source of contamination. In Victoria, clubroot is now endemic in all the major production regions, particularly in clay and sandy soils with neutral to acidic pH. It is predominantly a disease which affects summer-grown crops, especially those under regular irrigation, when soil temperatures are above 15 ºC. The clubroot pathogen has spread through movement of infested soil on machinery, contract labour and equipment and via furrow irrigation, in contaminated irrigation water and during floods. In 1998, the authors received a single report of clubroot from a new northern production region in Victoria, where soils traditionally have a higher pH of about 8.7. This was the first, and to date the only, formal report of the disease in the district. Further reports of new sites of infection from properties on heavier soils in more temperate regions have been confirmed by the authors at Trentham (100 km northwest of Melbourne) and Cora Lynn (60 km east of Melbourne) in February 1999.
Nationally, clubroot is considered endemic around the market gardens of the Sydney basin and is also a significant problem in Bathurst and Cowra (New South Wales), Virginia and the Adelaide hills (South Australia) and northern Tasmania. Clubroot outbreaks have occurred in Stanthorpe (Queensland Citation1997) and Manjimup (Western Australia 1993) and the disease has spread within these regions (Donald Citation2005). Clubroot is now a significant problem in every state of Australia. The authors confirmed a single report of the disease on a farm in Gatton, Queensland, in 2001. This was the last of Australia’s major production regions to be affected by clubroot. Growers in this region apply a voluntary summer break from brassicas. During this time, no brassicas are planted in the district. Together with high natural soil pH (approximately 8.0), this is likely to have delayed the onset of disease in this region.
Several practices have contributed to the spread of the disease locally. Firstly, the Australian vegetable brassica industry is almost completely dependent upon cell grown (modular) transplants as a source of planting material. Most of these transplants are produced in a relatively small number of large commercial nurseries which generally follow very strict hygiene programmes and are mostly outside brassica production regions. However, many growers continue to produce their own seedlings in small on-farm nurseries and these have been shown to be a source of spread. Secondly, several brassica production regions in Australia have been, or remain, dependent upon shared (contract) machinery and labour. Finally, movement of contaminated soil and water, in irrigation channels and on machinery and equipment, between properties is often unavoidable as many market gardens are small and may be divided between two or more properties, separated by several kilometres of shared road. Farm machinery and contract labour moves between these properties daily.
In the last 30 years, canola (Brassica napus L.) production has increased to become Australia’s third most valuable broadacre crop, with a gross value of AU $1.7 billion after wheat and cotton valued at AU $7.5 and $2.3 billion, respectively (ABS Citation2012). Thirty years ago, only 2.5% of canola crops from 18 countries were reported to be affected by clubroot (Crete Citation1981; cited in Rod & Havel Citation1992). Since then, production of canola has expanded significantly worldwide. Consequently, clubroot is now a serious disease of canola in many countries, including France (Rouxel & Regnault Citation1985), Canada (Howard et al. Citation2010), Czechoslovakia (Rod & Havel Citation1992), Sweden (Wallenhammar Citation1996), Britain (Davies Citation1986) and Germany (Cristen et al. Citation1999). In 2003, a paper presented to the Australian research assembly on brassicas identified clubroot as an imminent threat to the Australian canola industry (Donald & Porter Citation2003). Two key issues of concern, production on land that has produced fodder brassicas and contact with horticultural brassicas (including the use of irrigation water from sources which have previously received run-off from infested soils) were cited. Clubroot has since been reported from canola fields in the northern agricultural region of Western Australia (Khangura & Wright Citation2012) but is not yet widespread in Australian canola fields. All of the research conducted to date in Australia has been directed towards understanding the disease and minimizing crop losses in vegetable Brassica crops. This review is a summary of the Australian research effort.
An overview of Australian clubroot research projects
In 1974, the first study of Plasmodiophora brassicae epidemiology in Australia reported the reactions of 42 Brassicaceae cultivars and hybrids grown in soil from five clubroot-affected areas of Victoria. Further, this study utilized the Williams and European Clubroot Differentials (ECD) series to classify populations from three sources (Chambers Citation1977). Subsequent Australian research was directed towards control in commercial farms, including an evaluation of the use of solarization, alone (Porter & Merriman Citation1985) or in combination with low rates of fumigants (Porter et al. Citation1991). Control of clubroot using pentachloronitrobenzene (PCNB)-based fungicides was common during the 1970s and 1980s. Control with the fungicide fluazinam was first reported in Australia by Ransom et al. (Citation1991).
The prevalence of clubroot and associated crop losses increased substantially in Australia in the 1980s and 1990s. This occurred because of industry expansion, increased use of transplants, shorter rotations, more intensive cropping on the same soil and suspected increased movement of the pathogen on trucks, bulk bins and other farm equipment.
In response to the escalating clubroot problem, the Horticultural Research and Development Corporation (HRDC), now known as Horticulture Australia Limited (HAL), funded a survey and some pilot studies in 1993 to examine the effectiveness of existing control measures and the need for more extensive research on disease management. This survey showed that more than 70% of Victorian properties that were growing brassicas were affected by clubroot (Porter et al. Citation1994). The average farm level crop loss from clubroot in that year was 6.4% across the properties where clubroot infection had occurred. For those properties with a history of clubroot, including those that did not experience an outbreak in 1993 but had a history of infection, the average annual crop loss was 5.3%. Because of the unreported sub-lethal losses from clubroot, it was assumed that the average crop loss was 8.5%. Crop losses of up to 25 ha per property were reported and only 26% of growers indicated that they believed they had an effective method of clubroot control. Most of these growers cited the use of new land as their preferred method of ‘control’. The survey showed that there was no effective method to control clubroot in Australia, and only partial control was achieved by soil fumigation with metham sodium. It also demonstrated that the traditional cultural control practices, such as addition of lime (calcium carbonate) to raise soil pH, were gradually becoming less effective and the benefit of using new forms of lime such as calcium oxide was mostly unknown. Up until 1990, PCNB was widely used for clubroot control; however, it only provided substantial control at high application rates (18 kg ha−1) under conditions of low disease pressure (Colhoun Citation1958) and its use was largely replaced by routine use of the soil fumigant metham sodium in many of the major production regions. In research trials, solarization combined with low dose rates of dazomet was shown to be very effective at controlling clubroot, but commercial use of this technique was restricted because it was too costly, impractical to use under plastic film and could only be used during summer when many commercial crops were most productive (Porter et al. Citation1991).
In response to the survey and pilot studies, and the 1993 outbreak of clubroot in Manjimup, Western Australia, which threatened the region’s cauliflower exporting industry, a multifaceted study was commenced in 1996 to limit the spread of clubroot to unaffected land and minimize its impact where the disease was already endemic. Led by Drs. Ian Porter and Caroline Donald (Department of Primary Industries, Victoria), the project expanded to become a national programme of research, development and extension which was active until 2010. The programme involved 13 research, development and communication projects, over 40 field trials to improve control across Australia at large commercial farms infested with clubroot, and numerous controlled laboratory and microscopic studies. It linked researchers from government departments in five Australian states and included five PhD projects. Funding for research conducted under the national programme was primarily through Horticulture Australia Limited and the Victorian Department of Primary Industries with co-contributions from other state government departments, universities and industry organizations, such as fertilizer, seed and chemical companies. With the exception of unpublished private research conducted by seed and/or chemical companies and a single industry-funded PhD project (Kong Kaw Wa Citation2009) all clubroot research conducted after 1993 was conducted under this national clubroot programme.
In 2007, a national vegetable industry IPM pathology gap analysis reported that clubroot was no longer considered a priority pathogen (pathogen of concern) for the vegetable industry (Porter et al. Citation2007). Consequently, funding for further research through Horticulture Australia Limited ceased in Citation2010.
The Australian pathogen population
Variation in the Australian pathogen population has been studied using the Williams (Williams Citation1966; Chambers Citation1977) and European Clubroot Differential (ECD) series (Donald Citation2005; Donald et al. Citation2006a; Kong Kaw Wa Citation2009) and using molecular methods (Badi et al. Citation2010). All of these studies indicate that physiological variants of P. brassicae exist in Australia. Bioassay work using differential host plants indicates that the Australian populations appear more similar to populations reported from the USA than those from Europe. These studies report almost no variation in the reaction of the Australian pathogen collections to the Brassica rapa differential hosts (01–05) with only one causing a susceptible host reaction in a B. rapa host other than host 05 (‘Granaat’), assumed to be universally susceptible (Donald et al. Citation2006a). This is unsurprising since stubble turnips (B. rapa) are not frequently grown in mainland Australia, hence strong virulence towards this group of hosts is not likely to have segregated in mainland pathogen collections.
A wide range of virulence toward the B. napus differential hosts (06–10) exists worldwide (Toxopeus et al. Citation1986). By contrast, Donald et al. (Citation2006a) reported that 80.5% of the 41 Australian collections screened failed to produce a susceptible reaction on any of the B. napus hosts or only caused a susceptible reaction on B. napus hosts 06 and/or 07. Screening conducted by Kong Kaw Wa (Citation2009) produced a similar result for the B. napus differential hosts. Chambers (Citation1977) reported the presence of races 6 and 7 (Williams’ differentials) corresponding to ECD race numbers 16/2/30, 16/0/30 and 16/2/31, 16/0/31, respectively. That indicates the presence of high levels of virulence to B. oleracea differentials. Australian collections sent to the USA from New South Wales were identified as races 3, 6 and 7. A collection from Victoria was identified as race 6 (Williams Citation1996). Taken together, these studies conducted using differential hosts indicate a lack of virulence towards B. napus hosts 08, 09 and, to a lesser extent, 10 in Australian pathogen populations.
Differential variation in host reaction was greatest for the B. oleracea group of hosts; however, the poor differentiating ability of hosts 13 and 14 reported elsewhere (Toxopeus et al. Citation1986) was confirmed with susceptible reactions being recorded from these hosts for 93 or 86% of the collections screened by Donald et al. (Citation2006a) and Kong Kaw Wa (Citation2009), respectively.
There have been several Australian reports of different ECD codes being assigned to the same pathogen collection when that collection has been used in multiple screening bioassays (Donald et al. Citation2006a; Agarwal et al. Citation2009). This lack of reproducibility in the ECD analysis may result from the large proportion of indeterminate host reactions, the presence of mixed populations of resting spores present in the galls used for inoculum preparation (Crute et al. Citation1980; Jones et al. Citation1982; Scholze et al. Citation2002) or may be due to variable environmental conditions during the bioassay.
A microsatellite-based molecular technique was proposed by Faggian et al. (Citation1999b) as a first step towards fast, accurate and reliable identification of P. brassicae. This type of approach is appealing as it avoids the need for a bioassay based-system and the associated problems of indeterminate host reactions and environmental influences on the host reaction. This work is the subject of a current PhD project which is using RAPD and microsatellite primers to investigate polymorphism in Australian collections of P. brassicae. RAPD primers OPA 1, 8, 11; OPB 3, 7, 20; OPM 2, 13, 16; and microsatellite primers (GACA)4, HKB 17/9, HKB 23/52 were shown to reveal key differences between the collections of P. brassicae investigated in the project (Badi et al. Citation2010).
Farm and nursery hygiene
Anecdotally the incidence and severity of clubroot disease became a major economic problem to the brassica vegetable industry during the late 1980s and early 1990s. In part this was due simply to increasing intensity of production and poor crop rotation practices. However, reports of outbreaks of clubroot from previously unaffected properties, often in areas geographically isolated from known sites of infection, concerned brassica growers and made farm and nursery hygiene a high priority for research.
Molecular detection and quantification
As an obligate biotroph, detection of P. brassicae is challenging as the pathogen cannot be cultured. A range of detection methods including plant bioassays, fluorescence microscopy, serology and DNA-based methods have been used to detect P. brassicae in soil, water and plant samples. These methods have been reviewed elsewhere by Faggian and Strelkov (Citation2009). In Australia, visual or microscopic examination of bait plants grown in a sample of soil under controlled conditions has been used historically to estimate soil inoculum load. Visual assessment of gall development is time-consuming and requires considerable amounts of glasshouse space. Microscopic observation of root hair infection is faster but several workers have reported that no linear relationship exists between root hair infection and subsequent gall development (MacFarlane Citation1952; Naiki et al. Citation1978). A fluorescent staining technique utilizing two fluorochromes, calcofluor white M2R and ethidium bromide, to differentiate between viable and non-viable resting spores was developed by Takahashi and Yamaguchi (Citation1988), but the presence of fluorescing soil particles and other artifacts made it difficult to identify and enumerate P. brassicae resting spores in soil samples. Even when soil was excluded from the test system, the fluorescent stain did not correlate well with disease severity measured using bait plants in two out of three experiments conducted by Donald et al. (Citation2002).
Faggian et al. (Citation1999a) developed a nested PCR detection protocol directed at the ribosomal genes (rDNA) and internal transcribed spacer (ITS) regions of P. brassicae. The assay enabled detection down to 10 spores per gram and was developed as a commercial test in 2001 by the Victorian Department of Primary Industries. Its use was predominantly to resolve disputes between growers, determining sources of spread, and within research programmes to predict soil inoculum loads. Routine use of the assay by industry was limited by the high cost of DNA-based methods, initially AU $110 per sample during 2001 but increasing to as much as AU $450 under a full cost recovery model in later years (Faggian & Strelkov Citation2009).
The method used to extract DNA from soil was further refined by Faggian and Parsons (Citation2002) utilizing a bead-beating mill and polyvinylpolypyrrolidone (PVPP) spin columns to remove unknown inhibitory compounds which had previously resulted in poor amplification efficiency from some soil types. These authors also developed a quantitative real-time PCR assay and used it to predict disease severity in field plots.
Large-scale field evaluation of the quantitative assay was conducted by Donald et al. (Citation2004a) who reported good correlation between actual and predicted disease severity at 32 of the 55 sites sampled. However, DNA of P. brassicae was not detected in 23 samples from a range of soil types in which disease developed. The cause of these observed false negative results was the subject of further investigation (Donald et al. Citation2004a; Faggian et al. Citation2007). Several of the ‘problem’ soils were Ferrosols (Krasnozems) with relatively high free iron (>5% Fe) contents. A third soil was a grey loamy sand with a low organic matter content (1.4%). These soils contain mainly kaolinite clay particles, together with iron and aluminium sequioxides, which can develop a net positive charge at low pH. From these results together with the work of Shinoda et al. (Citation2003), it was hypothesized that the cause of the false negative test results was the electrical adsorption of negatively charged P. brassicae resting spores onto the surface of soils with a net positive charge. Binding of alcian blue, a dye with four positively charged sites in the molecule, to resting spores of P. brassicae confirmed their negative surface charge. Five methods were then used to pre-treat soil before extraction to overcome any binding of the spores to positively charged sites in the soil. The fastest and simplest of these methods, the use of Calgon™ (100% sodium hexametaphosphate), together with pH adjustment, was recommended as a pre-extraction treatment for all soil samples before testing for clubroot.
Subsequently, Faggian et al. (Citation2007) used a PCR reaction additive (T4 Gene 32 Protein) to reduce the inhibitory effects of humic/fulvic acids and reported 10-fold greater sensitivity using this method compared with Calgon™ treatment. Treatment with T4 Gene 32 Protein is a post-extraction procedure that involves the addition of protein to the PCR reaction mix. It is much quicker and simpler than Calgon™ treatment, and more effective. It also has a completely different mode of action, since it acts directly on the DNA polymerase and enables the enzyme to function in the presence of inhibitors. Faggian et al. (Citation2007) concluded that their results using the T4 Gene 32 Protein and the finding that updated soil extraction kits were able to extract DNA from soil more reliably than previous iterations, suggested that the mechanism of action of Calgon™ may not involve the soil-spore interaction at all. Instead, the more likely scenario is that the pH adjustments inherent in Calgon™ treatment somehow reduce the co-purification of humic/fulvic acids during downstream DNA extraction procedures. Faggian et al. (Citation2007) were also able to demonstrate improved yields of DNA extracted from soil using FastDNA Spin Kits with the addition of skim milk powder as an adsorption competitor to reduce the number of binding sites on soil.
In spite of the substantial amount of work undertaken to develop and optimize PCR-based assays in Australia and to overcome the issues of poor yield of extracted DNA and inhibition of the PCR reaction in some soil types, commercial use of the final diagnostic assay in Australia was limited due largely to its high cost (up to AU $450 per sample). A low-cost alternative utilizing monoclonal antibody technology on a lateral flow test device was proposed by Roy Kennedy and Alison Wakeham (Warwick HRI, UK) (pers. comm). This technique utilizes monoclonal antibody technology on a lateral flow test device. This technique has been used with success to detect and quantify fungal plant pathogens (Thornton et al. Citation2004). Unlike polyclonal antisera, monoclonal antisera can be produced indefinitely. These tests are also very inexpensive, can be conducted on site by the farmer and, potentially, a single lateral flow device can be used to detect or quantify multiple diseases. Under a collaborative research project, a prototype kit was successfully field-tested in Australia (Faggian et al. Citation2007; Wakeham et al. Citation2008), but the work has since not been supported by the Australian industry.
Clubroot hygiene strategy
The PCR-based molecular detection test (Faggian et al. Citation1999a) was used to detect P. brassicae in dams, boreholes and nurseries in a brassica production region in Victoria, Australia (Faggian et al. Citation1999c). Of particular concern in this study was the identification of contamination by P. brassicae on plastic seedling trays being returned to the nursery for reuse and in dust. Frequently, these trays were dipped in a disinfectant bath before reuse, however, laboratory screening indicated that many of the commercial disinfectant compounds in use were ineffective at either reducing or eradicating P. brassicae spore viability (Donald et al. Citation2002).
The potential for irrigation water contaminated with P. brassicae to cause clubroot in seedlings or crops was investigated in a series of experiments (Donald Citation2005). Spore suspensions containing 108 spores mL−1 were stored at 2°C for periods of 1 to 3.5 years. No relationship was found between the ability of the stored suspensions to cause root galling in bait plants and the duration of storage. Some suspensions that were approximately 1 year old failed to cause root galling in bait plants whilst others that were almost 3 years old remained infective. Subsequent daily irrigation of bait plants with water containing 0, 101, 102, 103, 104 or 106 spores mL−1 indicated that even at the lowest concentration of inoculum, half of the bait plants became infected. In spite of this, irrigation with water collected from contaminated farm dams in Victoria has failed to induce symptoms of clubroot in pot trials. In farm dams, resting spores might be expected to settle to the bottom of the dam over time. This rate of settling of P. brassicae resting spores in an undisturbed body of water was estimated at 25 cm day−1 using settling columns (Donald Citation2005).
A range of chemical amendments were screened for their ability to prevent clubroot in cell-grown brassica transplants (Donald Citation2005; ). Incorporation of fluazinam or lime (CaO) into inoculated potting mixture before sowing completely eliminated visual symptoms of root galling (a); however, seedling emergence was reduced by approximately 8% by the fluazinam treatment (b). Seedlings grown in fluazinam-treated potting mixture were also significantly smaller and leaf yellowing was evident in the lower leaves (c). Symptoms of phytotoxicity were not observed for the other fungicide treatments (flusulfamide and PCNB) but a small number of plants with root galls were evident after their use (a). Since lime was as effective as fluazinam, but did not cause any reduction in seedling emergence, loss of seedling vigour or symptoms of phytotoxicity, and was much cheaper than the chemical alternatives, it was the obvious choice to use in nurseries.
Table 1. Chemical amendments screened for their ability to prevent clubroot in nursery-grown transplants (rates expressed as rate of product, not active ingredient).
Fig. 1. (a) Clubroot severity rating (visual scale 1–9 where 1 = no root galling & 9 = no healthy roots, 4 weeks after sowing, LSD (P = 0.05), broc = 0.4, ccabb = 0.5); (b) seedling emergence (%, assessed 2 weeks after sowing, LSD (P = 0.05) broc = 6.9, ccabb = 6.8); (c) dry weight (g, 4 weeks after sowing, LSD (P = 0.05) broc = 0.1, ccabb = 0.1) of Chinese cabbage (open bar) and broccoli (black bar) seedlings. Lime, ground burnt agricultural (GBA) lime (76% CaO, 12% MgO); PCNB, pentachloronitrobenzene.
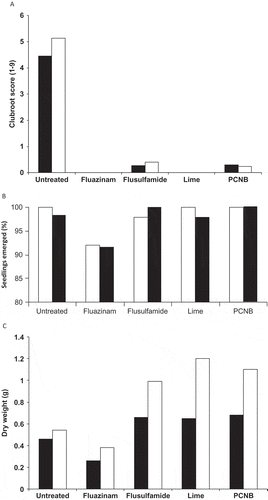
The results of this research were used as part of a ‘clubroot hygiene strategy’ distributed to vegetable brassica growers nationally. The recommendations included: (1) high-pressure washing of reusable plastic seedling trays before steam-sterilization or disinfection to reduce inoculum load and avoid the build-up of organic matter in disinfectant solutions; (2) the use of sodium hypochlorite (commercial bleach) as a cheap and effective alternative to commercial disinfectants; (3) avoiding use of water from dams for irrigations of nursery stock, since resting spores can survive for long periods in water and can, at least theoretically, cause infection at concentrations as low as 10 spores mL−1; (4) placing the irrigation intake pipe into the stillest part of the dam, mounted on a float to ensure that water is collected near the surface of the dam where the concentration of resting spores is likely to be lowest.
Relatively simple recommendations such as these together with inexpensive changes to farm and nursery design (including restricted access and installation of vehicle wash-down facilities), have virtually eliminated commercial nurseries as a source of contaminated planting material in Australia and, at least anecdotally, reduced its spread in recently affected areas.
Host-pathogen interactions
In the 135 years since the original reference linking P. brassicae to clubroot disease of crucifers (Woronin Citation1878), considerable research effort has been directed towards understanding the taxonomy, life history and pathology of this pathogen. Recent taxonomic thinking has seen the group included in the Order ‘Plasmodiophorida’, Class ‘Phytomyxea’, within the infraKingdom or supergroup ‘Rhizaria’ (Cavalier-Smith Citation2002, Citation1993; Goeche et al. Citation2012). Previously, the group has been included in the Kingdoms Protoctista (Margulis et al. Citation1989) and Protozoa (Barr Citation1992) as well as being described as primitive fungi by early workers (Karling Citation1968). Debate over the taxonomic status of the Plasmodiophorids remains current, arising largely from an incomplete knowledge of the lifecycles of many of the Plasmodiophorids. Braselton (Citation1995) comments that the most obvious gap in our understanding of this group is the identification of the point at which karyogamy occurs in the life cycle, or, if it does not occur, how nuclei become capable of meiosis. Zoospore fusion (Köle & Gielink Citation1961; Tommerup & Ingram Citation1971) or fusion of motile myxamobae (Mithen & Magrath Citation1992) have been suggested as a means of genetic recombination in Plasmodiophora; however, neither of these processes have been extensively studied in Brassica spp. and the evidence is currently far from conclusive. The current status of the life history of P. brassicae has been reviewed by Kageyama and Asano (Citation2009).
Once penetration of host tissue has occurred, P. brassicae induces in its host a suite of changes in primary and secondary metabolism which eventually cause gross physical distortion of the infected root. Relatively recent advances in technologies available to study changes in gene expression and protein composition have contributed significantly to our understanding of the pathology of P. brassicae, including changes in host nutrition, signalling and the role of key plant hormones in defence responses. The past 25 years has seen rapid growth in the number of papers published relevant to clubroot in this field of research. These have been reviewed by Ludwig-Müller et al. Citation(2009).
In Australia, the long-term objective of host-pathogen studies has been the manipulation of this interaction as a means of reducing the effects of disease. To this end, Australian researchers have been involved in studying the physiological response of resistant and susceptible hosts to infection and growth of P. brassicae (Donald et al. Citation2008), investigating gene expression in the susceptible reaction (Agarwal Citation2009; Kong Kaw Wa Citation2009; Agarwal et al. Citation2011) and manipulation of the host-pathogen interaction to induce a systemic acquired resistance response and reduce the impact of disease in an otherwise susceptible host (Lovelock Citation2013; Lovelock et al. Citation2013).
Anatomy of the resistant reaction
Following an extensive breeding programme detailed in Diederichsen et al. (Citation2009), the first clubroot-resistant cultivars of B. oleracea were released by Syngenta Seeds BV into the UK and European markets in 2005 (Diederichsen et al. Citation2009) and subsequently the Australian market during 2007 (Donald & Porter Citation2009). Australian researchers involved with the screening of these resistant breeding lines against Australian pathogen collections (Donald et al. Citation2006) studied differences between the resistant and susceptible host reactions using light and electron microscopy (Donald et al. Citation2008). The experiments were conducted in sand solution culture (Donald & Porter Citation2004) for ease of observation of root hair stages and a comparison of the infection in a clubroot-resistant line of B. oleracea var. botrytis (cauliflower) with the susceptible cultivar ‘White Rock’. Using this method, primary (root hair) and secondary (cortical) stages of P. brassicae were observed in both resistant and susceptible hosts. Cell wall breaks, the presence of vesicles or inclusion bodies within cell walls, cell wall thickening in association with plasmodesmata and enlarged and/or disorganized host nuclei were observed in the cortex of inoculated resistant and susceptible hosts. The key difference observed between infected resistant and susceptible hosts was the absence of degradation of the secondary thickening and cell walls of the xylem in the resistant host (Donald et al. Citation2008). Agarwal et al. (Citation2009) subsequently attempted to replicate this work using a known clubroot-susceptible Arabidopsis thaliana ecotype Col-0 which facilitated the selection of time points for a microarray experiment.
Differences between the resistant and susceptible host responses in cauliflower were further studied using light microscopy to observe lignification after staining with 1% (w/v) phloroglucinol in 70% ethanol for 12 h. Additionally fluorescence microscopy was used to observe callose deposition after staining with 0.5% (w/v) aniline blue in 1.15 M dipotassium hydrogen orthophosphate for 12 h (Donald Citation2005). No evidence of lignification in response to inoculation in either the resistant or susceptible roots was observed. The resistant host reaction was, however, associated with production of α 1–3 glucan (callose) and two types of reactions were observed. The first type was a ‘local’ reaction in which small deposits were usually, but not exclusively, observed at the base of infected root hairs (a). This reaction occurred in 14% of resistant and only 1% of inoculated susceptible root hairs. The second type was a ‘general’ reaction in which the whole root hair and/or basal epidermal cell would fluoresce (b). This reaction occurred in 17% of the root hairs from inoculated resistant plants and only 3% from the inoculated susceptible plants. For both of these reaction types, the difference between the number of resistant and susceptible root hairs exhibiting ‘localized’ or ‘generalized’ fluorescence following inoculation was significant (P = 0.05) (Donald Citation2005). Callose is a glucan that can be rapidly deposited in stressed, wounded or pathogen-infected cells because of the presence of a pre-existing synthase enzyme present in the plasma membrane (Lamb et al. Citation1989). Deposition of callose is one of few host responses to pathogen invasion that does not require novel gene expression (Kobayashi et al. Citation1995). For this reason, it can be the ‘first line’ of defence against pathogen invasion.
Gene expression
Siemens et al. (Citation2006) reported the use of microarray analysis to examine gene expression at 10 and 23 days after inoculation (DAI) in Arabidopsis thaliana ecotype Col-0 following inoculation with a single-spore isolate of P. brassicae. Two subsequent Australian studies sought to extend this analysis to the primary stages of the host-pathogen interaction by studying changes in host gene expression at earlier time points in B. rapa (Kong Kaw Wa Citation2009) and A. thaliana (Agarwal Citation2009; Agarwal et al. Citation2011).
Two methods were used to study gene expression in partially resistant and susceptible B. rapa. The first used a low-cost ‘boutique’ Brassica oligonucleotide array of 150 Arabidopsis–Brassica derived features constructed using nucleotide sequences, of mostly defence associated and regulatory genes, from GenBank. This indicated a high number of constitutively and differentially expressed genes at 48 h after inoculation (HAI) as opposed to 24 or 72 HAI. The author proposed that this 48 h time point correlates with timing of penetration by primary zoospores. Key observations included the constitutive over-expression of a pathogenesis-related protein, chitinase, as well as the upregulation of a lignin biosynthesis enzyme, caffeoyl-CoA 3-O-methyltransferase, in the partially resistant cultivars. A more sophisticated microarray platform, the Affymetrix Arabidopsis ATH1 GeneChip, was used for whole-genome transcriptional profiling of B. rapa cultivars at 48 HAI. This analysis showed three major salicylic acid (SA) dependent defence responses that were consistent with elevated levels of SA in partially resistant and resistant cultivars.
Agarwal et al. (Citation2011) used the Affymetrix Arabidopsis ATH1 GeneChip to study host gene expression in A. thaliana (susceptible) ecotype Col-0 during the primary stages of infection (4, 7 and 10 DAI) by P. brassicae. The study revealed significant induction or suppression of a relatively low number of genes in a range of functional categories. Differential expression was greatest 4 DAI with 147 genes differentially expressed compared with 27 genes and 37 genes at 7 and 10 DAI, respectively. This confirmed results obtained using a qPCR assay to detect and monitor the progression of P. brassicae infection in A. thaliana roots up to 23 DAI (Agarwal Citation2009). In this work, P. brassicae could not be detected in inoculated roots at 1, 2 or 3 days after inoculation. Pathogen DNA was first detected in roots at 4 DAI confirming pathogen penetration.
Differentially expressed genes were classified into functional categories and related to the life-cycle stage of the pathogen in A. thaliana for the time point of interest (; Agarwal et al. Citation2011). In the susceptible reaction, salicylic acid and ethylene biosynthesis were downregulated 4 DAI but jasmonic acid biosynthesis was induced (Agarwal et al. Citation2011). The elevated levels of SA in resistant and partially resistant B. rapa/P. brassicae interactions (Kong Kaw Wa Citation2009) together with the downregulation of SA biosynthesis in the compatible Arabidopsis Col-0/P. brassicae interaction (Agarwal et al. Citation2011) provide clear evidence for the role of SA in host defence responses to P. brassicae.
Fig. 3. Left panel: the life-cycle stages of P. brassicae (primary root hair phase) at 4, 7 and 10 days after inoculation (DAI). Right panel: summary table of up-and downregulated genes and functional groups at 4, 7 and 10 DAI (reproduced from Agarwal et al. Citation2011).
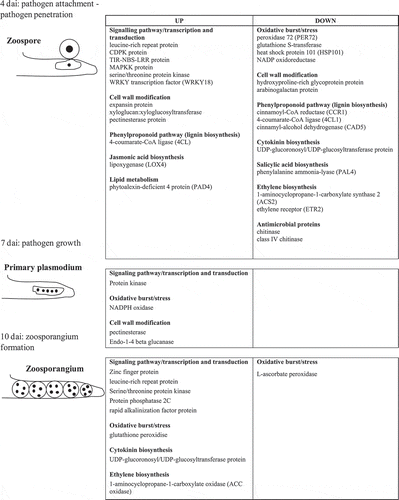
Systemic acquired resistance
Agarwal et al. (Citation2011) reasoned that since SA biosynthesis was downregulated in the compatible (susceptible) interaction, exogenous application of SA should reduce the susceptibility of A. thaliana Col-0 to P. brassicae. These authors went on to demonstrate that dipping 14-day-old A. thaliana seedlings in 500 µM unbuffered SA for 60 s before planting and inoculation with P. brassicae reduced the disease index from 81.5 (inoculated/untreated) to 20 (inoculated/SA treated) out of a possible 100. Lovelock et al. (Citation2013) continued this work reporting systemic transportation of exogenously applied SA from roots to leaves in B. oleracea. This was associated with upregulation of pathogenesis-related genes PR-1 and PR-2 in a ‘systemic acquired resistance like’ response as early as 24 h after treatment. Application of concentrations greater than 5 mM significantly reduced root galling by between 25 and 65% measured 6 weeks after inoculation, but concentrations in excess of 20 mM were phytotoxic, significantly reducing root and shoot weights.
The use of Arabidopsis mutants further confirmed the role of SA in the host defence against P. brassicae (Lovelock Citation2013). The mutants cpr1 and dnd1 over-express SA. Both showed reduced root galling after inoculation with P. brassicae compared with the SA deficient NahG mutant and the wild type Col-0. Elevated levels of PR-1 were measured in the over-expressor mutants and in the wild-type treated with 0.5 or 1 mM SA.
Clubroot control
Survey work conducted during 1993 indicated that traditional cultural control practices including rotation of susceptible crops with non-brassica crops and addition of lime (calcium carbonate) to raise soil pH were gradually becoming less effective and there was an urgent need to identify cost-effective controls for clubroot in Australia (Porter et al. Citation1994). A large-scale programme of field trials was therefore commenced in Australia in 1994. This work was initially directed towards identifying new control treatments and comparing their efficacy with the most commonly used and effective existing grower practice of fumigation with metham sodium. Optimum rates and methods of application were subsequently developed for a range of alternative effective treatments and their use as part of an effective integrated control strategy was investigated in relation to pathogen epidemiology. Summaries of the integration of classical and molecular approaches to control the disease have previously been presented (Porter et al. Citation2004). The range of control methods available to manage clubroot worldwide has also been reviewed previously (Donald & Porter Citation2009). A summary of the most effective treatments from the Australian experience, based predominantly on extensive field trials from 1994 to 2003, is summarized below. identifies the major changes observed in the shift of alternatives over the last 50 years. It also shows that the key alternatives used to control clubroot today are the use of calcium oxide-based lime to raise pH and application of calcium nitrate with boron (Nitrabor™) during the first 3 weeks of transplanting crops. These treatments, together with greater knowledge of the pathogen epidemiology, have enabled growers to reduce reliance on soil fumigation with metham sodium and to avoid the need for chemical treatments, such as fluazinam, which are only considered necessary when high disease levels are anticipated. To be effective, this fungicide needed to be applied correctly as a banded spray incorporated around the transplant root zone (Donald et al. Citation2001).
Table 2. Summary of key control measures used to control clubroot commercially in Australia.
A summary of the major trial results is presented below. In total, over 40 field trials were conducted and although the effect of some treatments varied from site to site, the key trends are discussed in the following sections.
Lime and nutrients products
Managing clubroot by raising soil pH is one of the oldest and most widely practiced methods of control (Karling Citation1942). Since 1994, Australian trials have predominantly utilized ground burnt agricultural (GBA) lime (76% calcium oxide, 12% magnesium oxide, David Mitchell Ltd, Lilydale) because it is very reactive with water and can effectively raise soil pH in a short period of time (generally at least 1.0 pH unit). It also can be applied at half the rate of calcium carbonate as it has a higher neutralizing value and pH (NV = 160%, pH 12–12.5), and finer particle size than calcium carbonate-based limes (NV = 80–100%, pH 7.5). GBA lime and similar products such as Quicklime™ (82% CaO, 4.4% MgO) must be applied a week before transplanting to avoid phytotoxicity (as observed with Quicklime™ in trials in 1996) ().
Table 3. The effect of lime and nutrient treatments on soil pH, clubroot severity and marketable yield of broccoli in field trials conducted at Werribee, Victoria.
Calcium and boron both have widely accepted roles in the inhibition of clubroot disease (Webster & Dixon Citation1991a, Citation1991b; Donald & Porter Citation2009) and the interaction of pH with calcium is well known (Myers & Campbell Citation1985; Donald & Porter Citation2004). Products such as gypsum (calcium sulphate) and calcium nitrate that contain calcium but do not cause a significant increase in soil pH failed to significantly (P = 0.05) reduce symptoms of disease when applied alone or cause a consistent, significant increase in marketable yield. By contrast, when calcium nitrate was applied together with lime, the resulting reduction in root galling and increase in marketable yield was greater than the effect of either product used alone (). This supports laboratory results (Donald & Porter Citation2004) which suggest that pH is the dominant factor influencing the disease outcome. At low pH values, disease will be severe regardless of calcium application and at high pH, disease severity will be reduced irrespective of the amount of calcium applied. At intermediate pH values, there is a role for calcium in the control of clubroot. In the laboratory, there was a significant effect of added calcium at soil pH values between 6.5 and 7.5. At pH 8, there was no effect of added calcium (Donald & Porter Citation2004). In the complex soil environment, the relative effects of calcium and lime will depend upon a number of factors, including the buffering capacity of the soil, the soil organic matter content and the calcium: magnesium ratio. In general terms, however, these trials demonstrate the potential benefits of the use of integrated strategies for disease control that combine several different effects such as pH, boron and calcium. In the trials reported here (), three split applications of calcium nitrate formulations amended with boron in the first 3 weeks after transplanting were the most effective method of application of this treatment. Whilst there may be scope to reduce the frequency of application, these trials suggest that early frequent applications of calcium are required to protect the young transplants from infection.
Calcium cyanamide
Calcium cyanamide can provide excellent control of clubroot and at present it is still used commercially on some farms in Australia as it also provides economic benefits as a fertilizer and has activity for weed control. The ability of this product to control clubroot had been known internationally for many years (Walker Citation1935; Karling Citation1968) and its efficacy in Australia previously demonstrated (Porter et al. Citation1997; Donald et al. Citation2004b). However, when used at an application rate of 1 t ha−1, coupled with shipping costs to Australia that drive the cost of the product up to AU $1400 t−1, this treatment has been considered too expensive by many vegetable growers. Studies have also demonstrated that changes in its formulation to remove the fine particles made the product less effective (Donald et al. Citation2004b). Despite this, however, it is used by some growers as a mild soil disinfestant and the potential still exists to improve its cost effectiveness by strategic field application with banding machines as discussed below.
Table 4. The effect of organic amendments on clubroot severity of broccoli in field trials conducted over 3 years on the same site at Boneo, Victoria, compared with fumigation using metham sodium and the standard fertilizer practice.
Table 5. Treatments and application details for chemicals used in clubroot control trials conducted during 1994–1996.
Organic amendments
Organic products, although often increasing yields due to their fertilizer value and benefits to soil health, have not been shown to be effective for control of clubroot. This is because in most cases they acidify soils and increase the cation exchange capacity (CEC) in soils. The resultant increase in CEC and moisture levels also appears to contribute to a more conducive environment for disease. Early studies with Dynamic Lifter™ (pelletized chicken manure, ) and more recent studies evaluating lignite, raw chicken manure, composted green waste and silage showed that none could reduce disease, and lignite in particular promoted disease (Porter et al. Citation2010, ).
Fungicides, fumigants and surfactants
The fungicides fluazinam and flusulfamide, and the surfactant alkyl phenyl ethylene oxide (Agral™), were selected for evaluation in broad-scale studies in Victoria based on initial reports from Ransom et al. (Citation1991), Dixon et al. (Citation1994) and Humpherson-Jones (Citation1993), respectively. These were compared with ground burnt agricultural lime (76% CaO, 12% MgO), an existing fungicide treatment of PCNB and two existing fumigant treatments, metham sodium and dazomet (). Dip or in-furrow soil drench applications of the fungicides PCNB and fluazinam suppressed clubroot as effectively as high rate application (423 L a.i. ha−1) of the soil fumigant metham sodium. These methods of fungicide application, however, were associated with phytotoxicity that resulted in lower than anticipated yields (). Spot drench application (100 mL plant−1) of either fungicide, whilst not as effective at suppressing symptoms of clubroot as the dip or in-furrow drenches, consistently increased the marketable yield of broccoli ().
Table 6. The effect of chemical treatments on clubroot severity and marketable yield of broccoli in field trials conducted at Werribee, Victoria.
In 1996, fluazinam, formulated as 50% suspension concentrate (SC), was registered in Australia for use as a high-volume drench to control clubroot disease of brassica crops: cabbage, broccoli, Brussels sprouts, cauliflower and kohlrabi. This method of application requires that each plant is drenched with a high volume of fungicide solution at transplanting. Commercially, this method of application, whilst very effective, was problematic as very few commercial transplant machines were designed to deliver liquid treatments in this way. In addition, due to the large volumes of water required, frequent breaks to refill the fungicide tank greatly increased the time taken for the transplanting operation. Also, when added to soil, fluazinam becomes strongly associated with organic matter and can be relatively immobile in the soil environment and be phytotoxic (Peter Taylor, Crop Care Australasia, pers. comm.). This limited the effectiveness of the label recommended drench applications, particularly in heavy soils where infiltration can be poor (Porter et al. Citation1998). Registration of flusulfamide in Australia was not pursued by the chemical supplier; therefore, the fungicide is not available commercially.
The methyl isothiocyanate (MITC) generator fumigants, metham sodium and dazomet, have been evaluated extensively and shown to be very effective for clubroot control. Since the late 1990s, however, growers have tended to move away from fumigation owing to user safety concerns, low cost-effectiveness and lack of product effectiveness at the low rates being commercially used throughout the industry at the time. During our studies, however, the efficacy of the fumigant treatments against clubroot was shown to be influenced by soil type. Very low rates of the fumigant dazomet were shown to be very effective treatments in the sandy soils of Victoria (Boneo site, ), but ineffective in heavy clay loams (Werribee site, ). In contrast, metham sodium was more effective in the heavy clay soils of Werribee, although the lowest rate used (212 L a.i. ha−1) was probably marginal for this soil type and produced some inconsistent results ( and ).
Table 7. The effect of treatments on clubroot rating and marketable yield of broccoli at Boneo, Victoria in Citation1996.
Strategic application
Commercially suitable methods for application of fluazinam were developed in subsequent field trials conducted in Victoria and Western Australia. Preliminary trials ( and ) indicated that poor product infiltration using the spot drench or continuous spray, and inadequate dispersal around the transplant root zone using the dip and in-furrow drench applications, had resulted in problems of inadequate control and phytotoxicity, respectively. A prototype machine consisting of a small tool bar to which two spray jets and two small rotary hoes were fitted was developed to distribute the fungicide evenly within the plant root zone along the planting rows. Each rotary hoe was mounted in a metal box 23 cm wide to retain treated soil in the planting row following tillage. Incorporation of fluazinam (as Shirlan; 50% a.i. SC; Crop Care Australasia, at a rate of 1.5 L a.i. in 2500 L ha−1 water) using this purpose-built machine was consistently more effective than either a spot drench (1.5 L a.i. ha−1 in 5000 L ha−1 water applied at 100 mL plant−1) or the ‘grower preferred’ continuous spray (1.5 L a.i. ha−1 in 2500 L ha−1 water) over the plants immediately after transplanting (Donald et al. Citation2001). This method of application consistently increased the marketable yield of broccoli and cauliflower. In one instance, yields of both crops were increased by at least 80% compared with other commercial methods of application in use (Donald et al. Citation2001). The method was effective in a range of soil types and remained effective when the volume of water used was reduced to 500 L ha−1.
The above method of application was subsequently adapted to suit application of granular products, in particular calcium cyanamide. Banded incorporation of calcium cyanamide into the transplant rows reduced the amount of product applied by approximately two-thirds with no significant reduction in the level of disease control associated with this fertilizer. When applied at the equivalent rate of 1 t ha−1, this method of application increased the profitability of calcium cyanamide by between AU $822 and AU $1482 compared with broadcast application at the same rate (Donald et al. Citation2004b). Greater potential for the use of calcium cyanamide in Australia appears possible by the development of more sophisticated machinery for its application.
Integrated control strategies
Having evaluated non-chemical and chemical treatments for the control of clubroot and recognizing that many of these treatments may have differing sites of action in P. brassicae and its hosts, field trials were devised to investigate the potential for using these treatments together in an integrated approach to control this pathogen. A summary of the steps taken to develop this approach have been presented previously and rely on knowledge of the pathogen life cycle, inoculum densities, the mode of action and site of infection and strategic application of effective methods of control (Porter et al. Citation2005, Donald et al. Citation2006b, ).
On low pH sites (trials conducted during 1997 with soil pH (water) of between 6.4 and 6.9), the most effective control strategies were treatments that consisted of combinations of two or three treatments including lime ( and ; ). As previously mentioned, the preferred treatments adopted were based on fertilizer treatments using calcium oxide (CaO) based lime, calcium nitrate and boron and if necessary, fluazinam as shown in . With the exception of the fluazinam/lime combination used on broccoli at Werribee where the rate of fluazinam was marginal (0.75 kg a.i. ha−1), the two-way treatment combinations (either boronated calcium nitrate (CaNO3/B) blend, fluazinam, or fumigants) that included lime were consistently more effective at reducing root galling due to clubroot and increasing marketable yield than the lime-alone treatment. Whilst the root galling observed as a result of the combination treatments was generally not significantly less than could be achieved by application of GBA lime alone, this translated to between 0.6 and 2.5 t ha−1 increased yield of broccoli or between 4 and 7.1 t ha−1 more cauliflower than could be achieved by application of lime alone (). This produced an increase in crop profitability of between AU $203 and AU $6579 than could be obtained using lime alone ().
Table 8. Soil pH, disease severity (visually assessed 1–9 scale 6 weeks after transplanting) and marketable yield following application of various treatments. Trials were conducted during 1997 at Werribee and Lindenow (L), Victoria using two host species (source: Donald et al. Citation2006).
Table 9. Additional profit (AU $ ha−1 above the untreated control) resulting from treatment application for clubroot.
Fig. 4. (Colour online) Effect of three nutrient and chemical treatments either alone or integrated together on the marketable yield (t ha−1) of cauliflower affected by clubroot at Werribee, Victoria in Citation1997. LSD (P = 0.05) 6.8.
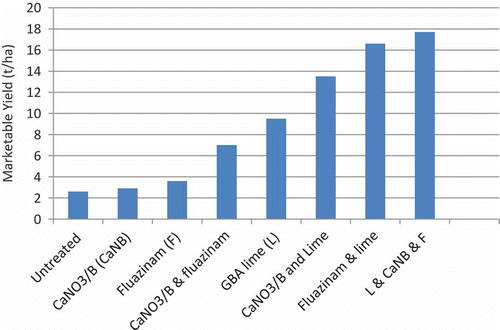
Fig. 5. (Colour online) (Left panel) Potential site of action of chemical and nutrient treatments on the P. brassicae life cycle and (right panel) a schematic relationship of the impact of three integrated treatments (GBA lime (76% Ca0, 12% MgO), calcium nitrate with boron (Nitrabor™) and fluazinam) on inoculum (log10 spores/g soil) and clubroot expression.
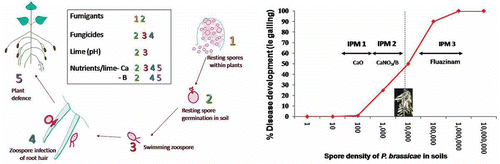
The three-way treatment combinations were consistently amongst the most effective treatments used and most profitable ( and , ). At some sites in Victoria (e.g. the Lindenow site), which have high disease pressure, the only treatment to significantly reduce root galling due to clubroot was the three-way treatment combination of fluazinam, CaNO3/B blend (Nitrabor™) and lime (). At other sites, however, the additional benefit in terms of increased yield from the addition of the fungicide treatment did not always translate directly to increased profitability, due to the additional treatment costs and growth reduction occurring with some methods of application of fluazinam, or low disease pressure. On some sites where disease pressure was extremely high, either fumigation or fluazinam applied using the optimum method incorporated around the root zone were the only treatments which gave added economic benefits as the nutrient treatments did not reduce the disease effectively alone. Owing to difficulties in the application of fluazinam, growers preferentially manage clubroot using calcium and boron fertilizers, application of calcium oxide-based lime to increase soil pH, rotation with non-brassica crops and sowing of badly infested fields in the cooler autumn and winter months when soil temperatures are less conducive to development of disease.
In the future, growers will need to be vigilant to ensure they adhere to the use of integrated programmes where they continue to be reliant on clean transplants, either prediction of disease levels using cheap diagnostic tests or good records of paddock (field) histories, and strategic use of precautionary nutrient treatments as discussed above. The use of resistant cultivars will also become a major part of any future integrated programmes in Australia, providing agronomic traits can be found suitable for domestic markets.
Economic evaluation of the Australian clubroot research programme
During 2011, the Department of Primary Industries commissioned independent consultants BDA Group to conduct an economic evaluation of investment into clubroot research and development (R & D) between 1993 and 2010. Losses from clubroot prior to the R&D were estimated using results of an industry survey in 1993 (Porter et al. Citation1994). As no published data were available to quantify any reduction in crop losses resulting from the research, the consultants conducted follow-up phone and email discussions with industry representatives and assessed the percentage reductions in clubroot losses as reported from the various commercial trials carried out under the clubroot R&D programme.
Feedback from industry confirmed that, since 1993, clubroot losses had fallen between 30% and 60%, with reductions expressed in terms of average crop losses each year. It was also noted that there can be significant seasonal variability in the incidence of the disease, depending on climatic conditions and the effectiveness of control undertaken (BDA Group 2011, cited in Emmett et al. Citation2012). Threshold analysis was used to determine the break-even reduction in clubroot losses and then to assess the likelihood that a greater level of reduction had occurred. Investment in the clubroot R&D programme delivered significant benefits to Victoria and Australia. After the R&D programme commenced in 1993, average crop losses caused by the disease declined by 30–60%, depending on farm disease incidence, seasonal climatic conditions and the disease control measures undertaken. On this basis, the estimated return to Victoria from the R&D investment was between AU $4.4 and $10.9 million (net present value). This represents a return of between AU $3.1 and $6.1 for every dollar invested, respectively. The estimated returns to Australia from the R&D investment was between AU $15.1 and $36.0 million (net present value). This represents a return of between AU $3.6 and $7.2 for every dollar invested (Emmett et al. Citation2012).
Ian J. Porter
Department of Environment and Primary Industries, La Trobe University, 5 Ring Road, Bundoora, Victoria 3083, Australia
Correspondence to: E. C. Donald. E-mail: [email protected]
E. Caroline Donald
Department of Environment and Primary Industries, La Trobe University, 5 Ring Road, Bundoora, Victoria 3083, Australia
Acknowledgements
We gratefully acknowledge the efforts of all who were involved with the Australian clubroot research programme over many years. The programme was funded by vegetable growers through the research and development levy, Horticulture Australia Ltd. and the Victorian Department of Primary Industries with co-contributions from other state government departments, universities and industry organizations such as fertilizer, seed and chemical companies.
References
- ABS. 2007. Ausstats, 7112.0 Selected agricultural commodities, Australia, Preliminary, 2005–2006. Table 3, Vegetables, Production – Year ended 30 June 2006, Australian Bureau of Statistics, Canberra, Australia; [revised 2007 Jul 26; cited 2013 Apr 17]. Available from http://www.abs.gov.au/AUSSTATS/[email protected]/DetailsPage/7112.02005-06
- ABS. 2012. Ausstats, 7501.0 Value of Principal Agricultural Commodities Produced, Australia, Preliminary, 2011–2012 Year ended 30 June 2012, Australian Bureau of Statistics, Canberra, Australia; [revised 2012 Nov 15; cited 2013 Apr 17]. Available from http://www.abs.gov.au/AUSSTATS/[email protected]/Lookup/7501.0main ± features32011-12
- Agarwal A. 2009. Interactions of Plasmodiophora Brassicae with Arabidopsis Thaliana [dissertation] Geelong (Australia): Deakin University.
- Agarwal A, Kaul V, Faggian R, Cahill DM. 2009. Development and use of a model system to monitor clubroot disease progression with an Australian field population of Plasmodiophora brassicae. Aust Plant Path. 38:120–127.
- Agarwal A, Kaul V, Faggian R, Rookes JE, Ludwig-Müller J, Cahill DM. 2011. Analysis of global host gene expression during the primary phase of the Arabidopsis thaliana-Plasmodiophora brassicae interaction. Funct Plant Biol. 38:462–478.
- Ausveg. 2012. Industry statistics, the domestic industry, detailed data by vegetable. Ausveg, National peak industry body for vegetable and potato growers, Australia; [revised 2012 Aug 24; cited 2013 Apr 17 ]. Available from http://www.ausveg.businesscatalyst.com/resources/statistics/domestic-industry/detailed-data.html
- Badi A, Lawrie AC, Donald EC. 2010. Genetic diversity of Plasmodiophora brassicae in Australia. In: Stirling GR, editor. Proceedings of the 6th Australasian Soilborne Diseases Symposium; 2010 Aug 9–11. Twin Waters, Queensland, Australia; p. 32.
- Barr DJS. 1992. Evolution of kingdoms of organisms from the perspective of a mycologist. Mycologia. 84:1.
- Braselton JP. 1995. Current status of the Plasmodiophorids. Crit Rev Microbiol. 21:263–275.
- Cavalier-Smith T. 1993. Kingdom Protozoa and its 18 phyla. Microbiol Rev. 57:953–994.
- Cavalier-Smith T. 2002. The phagotrophic origin of eukaryotes and phylogenetic classification of Protozoa. Int J Syst Evol Micr. 52:297–354.
- Chambers SC. 1977. Studies on some populations of Plasmodiophora brassicae (Woron) in Victoria. Research project series No. 20 (Agdex 637). Victoria (Australia): Department of Agriculture.
- Colhoun J. 1958. Club root disease of brassicas caused by Plasmodiophora brassicae Woron. Phytopathological Paper No. 3. Kew: Commonwealth Mycol Instit.
- Cristen O, Evans E, Nielsson C, Haldrup C. 1999. Oilseed rape cropping systems in NW Europe. In Proceedings of the 10th International Rapeseed Congress; 1999 Sept 26–29; Canberra, Australia.
- Crute IR, Gray AR, Crisp P, Buczacki ST. 1980. Variation in Plasmodiophora brassicae and resistance to clubroot disease in brassicas and allied crops – a critical review. Plant Breed Abst. 50:91–104.
- Davies JML. 1986. Diseases of Oilseed rape. In: Scarisbrick DH, Daniels RW, editors. Oilseed Rape London: Collins; p. 195–236.
- Diederichsen E, Frauen M, Linders EGA, Hatakeyama K, Hirai M. 2009. Status and perspectives of clubroot resistance breeding in crucifer crops. J Plant Growth Regul. 28:265–281.
- Dixon GR, Craig MA, Burgess PJ, Thomas J. 1994. MTF 651: a new soil-applied fungicide for the control of plasmodial fungi. Proc Brighton Conf. 1994:541–548.
- Donald EC. 2005. The influence of abiotic factors and host plant physiology on the survival and pathology of Plasmodiophora Brassicae of Vegetable Brassicas [dissertation]. Melbourne (Australia): Melbourne University.
- Donald E, Porter I. 2003. Clubroot (Plasmodiophora brassicae) an imminent threat to the Australian canola industry. Proceedings of the 13th biennial Australian Research Assembly on Brassicas; 2003 Sept 8–12;Tamworth (Australia): State of New South Wales, NSW Agriculture.
- Donald EC, Porter IJ. 2004. A sand solution culture technique used to observe the effect of calcium and pH on root hair and cortical stages of Plasmodiophora brassicae. Aust Plant Pathol. 33:585–589.
- Donald EC, Porter IJ. 2009. Integrated control of clubroot. J Plant Growth Regul. 28:289–303.
- Donald EC, Porter IJ, Lancaster RA. 2001. Band incorporation of fluazinam (Shirlan) into soil to control clubroot of vegetable brassica crops. Aust J Exp Agr. 41:1223–1226.
- Donald EC, Lawrence JM, Porter IJ. 2002. Evaluation of a fluorescent staining technique as an indicator of pathogenicity of resting spores of Plasmodiophora brassicae. Aust Plant Pathol. 31:373–379.
- Donald EC, Czerniakowski B, Porter IJ. 2004a. Optimising a diagnostic test for clubroot. Final report for project VG03022. Sydney (Australia): Horticulture Australia Limited.
- Donald EC, Lawrence JM, Porter IJ. 2004b. Influence of particle size and application method on the efficacy of calcium cyanamide for control of clubroot of vegetable brassicas. Crop Prot. 23:297–303.
- Donald EC, Cross SJ, Lawrence JM, Porter IJ. 2006a. Pathotypes of Plasmodiophora brassicae, the cause of clubroot, in Australia. Ann Appl Biol. 148:239–244.
- Donald EC, Porter IJ, Faggian R, Lancaster RA. 2006b. An integrated approach to the control of clubroot in vegetable brassica crops. Acta Hortic. 706:283–300.
- Donald EC, Jaudzems G, Porter IJ. 2008. Pathology of cortical invasion by Plasmodiophora brassicae in clubroot resistant and susceptible Brassica oleracea hosts. Plant Pathol. 57:201–209.
- Emmett RW, Edwards J, Porter IJ, Donald C. 2012. Economic Evaluation of Clubroot R & D, an Evaluation of Integrated Pest and Disease Management (IPDM) Case Study. Victoria (Australia): Department of Primary Industries.
- Faggian R, Parsons S. 2002. A Rapid Diagnostic Test for Clubroot Final Report for Project VG99008. Sydney (Australia): Horticultural Research and Development Corporation.
- Faggian R, Strelkov SE. 2009. Detection and measurement of Plasmodiophora brassicae. J Plant Growth Regul. 28:282–288.
- Faggian R, Bulman SR, Lawrie AC, Porter IJ. 1999a. Specific PCR primers for the detection of Plasmodiophora brassicae in soil and water. Phytopathology. 89:392–397.
- Faggian R, Donald C, Porter IJ, Lawrie AC. 1999b. The use of molecular techniques to investigate the genetic variation of Australian clubroot isolates. Proceedings of the 12th Australasian Plant Pathology Society Conference; 1999 Sept 27–30; Canberra (Australia).
- Faggian R, Donald C, Porter IJ, Lawrie AC. 1999c. Epidemiology of recent clubroot outbreaks using PCR to trace sources of inoculums. In: Proceedings of the 12th Australasian Plant Pathology Society Conference; 1999 Sept 27–30; Canberra (Australia).
- Faggian R, Kaul V, Donald C, Porter IJ, Kennedy R, Wakeham A. 2007. On Farm Diagnostic Kits for Brassica Diseases Final Report for Project VG04059. Sydney (Australia): Horticulture Australia Limited.
- Goeche F, Wiese J, Núnez A, Labes A, Imhoff JF, Neuhauser S. 2012. A novel phytomyxean parasite associated with galls on the bull-kelp Durvillaea antartica (Chamisso) Hariot. PLoS ONE. 7:e45358. doi:10.1371/Journal.pone0045358
- Howard RJ, Strelkov SE, Harding MW. 2010. Clubroot of cruciferous crops – new perspectives on an old disease. Can J Plant Pathol. 32:43–57.
- Humpherson-Jones FM. 1993. Effect of surfactants and fungicides on clubroot (Plasmodiophora brassicae) of brassicas. Ann Appl Biol. 122:457–465.
- Jones DR, Ingram DS, Dixon GR. 1982. Factors affecting tests for differential pathogenicity in populations of Plasmodiophora brassicae. Plant Pathol. 31:229–238.
- Kageyama K, Asano T. 2009. Life cycle of Plasmodiophora brassicae. J Plant Growth Regul. 28:203–211.
- Karling JS. 1942. The Plasmodiophorales. 1st ed. New York: J. S. Karling.
- Karling JS. 1968. The Plasmodiophorales. 2nd ed. New York: J. S. Hafner Publishing Company.
- Khangura RK, Wright DG. 2012. First report of clubroot caused by Plasmodiophora brassicae on canola in Australia. Plant Dis. 96:1075.
- Kobayashi I, Murdoch LJ, Kunoh H, Hardham AR. 1995. Cell biology of early events in the plant resistance response to infection by pathogenic fungi. Can J Bot. 73:S418–S425.
- Köle AP, Gielink AJ. 1961. Electron microscope observations on the flagella of the zoosporangial zoospores of Plasmodiophora brassicae and Spongospora subterranea. Proceedings Koninklijke Nederlandse Akademie van Wetenschappen. Series C. Biol. Medical Sci.. 64:157–161.
- Kong Kaw Wa SL. 2009. Discovering clubroot resistance genes in Brassica vegetable crops [dissertation]. Melbourne (Australia): RMIT University.
- Lamb CJ, Lawton MA, Dron M, Dixon RA. 1989. Signals and transduction mechanisms for activation of plant defenses against microbial attack. Cell. 56:215–224.
- Lovelock DA. 2013. Salicylic acid and its role in defence against Plasmodiophora brassicae [dissertation]. Geelong (Australia): Deakin University.
- Lovelock DA, Donald EC, Conlan XA, Cahill DA. 2013. Salicylic acid suppression of clubroot in broccoli (Brassica oleracea var. italic) caused by the obligate biotroph Plasmodiophora brassicae. Aust Plant Pathol. 42:141–153.
- Ludwig-Müller J, Prinsen E, Rolfe SA, Scholes JD. 2009. Metabolism and plant hormone action during clubroot disease. J Plant Growth Regul. 28:229–244.
- MacFarlane I. 1952. Factors affecting the survival of Plasmodiophora brassicae Wor. in the soil and its assessment by a host test. Ann Appl Biol. 39:239–256.
- Margulis L, Corliss JO, Melkonian M, Chapman DJ. 1989. Handbook of Protoctista. Boston: Jones and Bartlett Publishers.
- McAlpine D. 1891. Report on clubroot of cauliflowers, cabbages, turnips and other Brassica plants. Department of Agriculture Victoria, Bulletin 14:36–44.
- Mithen R, Magrath R. 1992. A contribution to the life history of Plasmodiophora brassicae: secondary plasmodia development in root galls of Arabidopsis thaliana. Mycol Res. 96:877–885.
- Myers DF, Campbell RN. 1985. Lime and the control of clubroot of crucifers: effects of pH, calcium, magnesium, and their interactions. Phytopathology. 75:670–673.
- Naiki T, Kageyama K, Ikegami H. 1978. The relation of spore density of Plasmodiophora brassicae Wor. to the root hair infection and club formation in Chinese cabbage (studies on the clubroot of cruciferous plant II). Ann Phytopathol Soc Jpn. 44:432–439.
- Porter IJ, Merriman PR. 1985. Evaluation of soil solarization for control of root diseases of row crops in Victoria. Plant Pathol. 34:108–118.
- Porter IJ, Merriman PR, Keane PJ. 1991. Soil solarization combined with low rates of soil fumigants controls clubroot of cauliflowers, caused by Plasmodiophora brassicae Woron. Aust J Exp Agric. 31:843–851.
- Porter IJ, Cross SJ, Asirifi N, Morgan W. 1994. Integrated Management of Clubroot of Crucifers: survey and Pilot Studies. Final report for project VG306. Sydney (Australia): Horticultural Research and Development Corporation.
- Porter IJ, Donald EC, Lancaster R. 1997. Integrated strategies to control and prevent the spread of clubroot. Final report for project VG431. Sydney, (Australia): Horticultural Research and Development Corporation.
- Porter IJ, Donald EC, Cross SJ. 1998. Field evaluation of fluazinam against clubroot (Plasmodiophora brassicae) of cruciferous vegetable crops. Tests of Agrochemicals and Cultivars No. 19. Ann Appl Biol. 132(Supplement):12–13.
- Porter IJ, Donald EC, Lancaster R, Faggian R. 2004. Integration of classical and molecular approaches to successfully control clubroot of crucifers. In: Proceedings of the 7th Conference of the European Foundation for Plant Pathology & British Society for Plant Pathology Presidential Meeting; 2004 Sept 5–10; Aberdeen (UK).
- Porter IJ, Cross SJ, Donald EC. 2005. Integrated control of clubroot, Plasmodiophora brassicae, of crucifers – A model system for soilborne pathogens. 15th Australasian Plant Pathology Society Conference Handbook; 2005 Sept 26–29; Geelong (Australia).
- Porter IJ, Donald EC, Minchinton E. 2007. IPM gap analysis for vegetable pathology. Final report for project VG06092. Sydney (Australia): Horticulture Australia Limited.
- Porter I, Mattner S, Edwards J, Brett R, Riches D, Hall C, Guijarro B, Fridman M, Fisher P, O’Halloran N, Engleitner S, Partington D. 2010. Benchmarking soil health for improved crop health, quality and yields in the temperate Australian vegetable industries. Final report for project VG7008. Sydney (Australia): Horticulture Australia Limited.
- Ransom LM, Collins D, Taylor PA, Baker A. 1991. A new weapon to beat clubroot. 8th Australasian Plant Pathology Conference; Sydney (Australia). [Abstr.].
- Rod J, Havel J. 1992. Assessment of oilseed rape resistance to clubroot (Plasmodiophora brassicae). Tests of Agrochemicals and Cultivars No. 13. Ann Appl Biol. 132(Supplement):106–107.
- Rouxel F, Regnault Y. 1985. Comparaison de la réceptivité des sols à la hernie des crucifères: application à l’évaluation des risques sur quelques sols à culture de colza oléagineuz. Premières Journées d’Études sur les maladies des plantes. Versailles (France): ANPP.
- Scholze P, Lalorny M, Drippe R. 2002. A comparative study of reaction to clubroot (Plasmodiophora brassicae Wor.) by inoculating plants with single races or a race mixture. J Plant Dis Prot. 109:217–226.
- Shinoda H, Murakami K, Goto I. 2003. An improved method for measurement of the resting spore density of Plasmodiophora brassicae from infested soils. Jpn J Soil Sci Plant Nutr. 74:287–297.
- Siemens J, Keller I, Sarx J, Kunz S, Schuller A, Nagel W, Schmulling T, Parniske M, Ludwig-Muller J. 2006. Transcriptome analysis of Arabidopsis clubroots indicate a key role for cytokinins in disease development. Mol Plant-Microbe Inter. 19:480–494.
- Takahashi K, Yamaguchi T. 1988. A method for assessing the pathogenic activity of resting spores of Plasmodiophora brassicae by fluorescence microscopy. Ann. Phytopathol. Soc. Jpn. 54:466–475.
- Thornton CR, Groenhof AC, Forrest R, Larnotte R. 2004. A one-step, immunochromatographic lateral flow device specific to Rhizoctonia solani and certain related species, and its use to detect and quantify R. solani in soil. Phytopathology. 94:280–288.
- Tommerup IC, Ingram DS. 1971. The life-cycle of Plasmodiophora brassicae Woron. in Brassica tissue cultures and intact roots. New Phytol. 70:327–332.
- Toxopeus H, Dixon GR, Mattusch P. 1986. Physiological specialization in Plasmodiophora brassicae: an analysis by international experimentation. Trans Brit Mycol Soc. 87:279–287.
- Wakeham A, Faggian R, Kennedy R. 2008. Development and validation of ‘in field’ detection kits for the clubroot pathogen Plasmodiophora brassicae. J Plant Pathol. 90:425.
- Walker JC. 1935. Calcium cyanamide in relation to control of clubroot in cabbage. J Agric Res. 51:183–189.
- Wallenhammar AC. 1996. Prevalence of Plasmodiophora brassicae in a spring oilseed rape growing area in central Sweden and factors influencing soil infestation levels. Plant Pathol. 45:710–719.
- Watson AG, Baker KF. 1969. Possible gene centres for resistance in Brassica to Plasmodiophora brassicae. Econ Bot. 23:245–252.
- Webster MA, Dixon GR. 1991a. Calcium, pH and inoculum concentration influencing colonization by Plasmodiophora brassicae. Mycol Res. 95:65–73.
- Webster MA, Dixon GR. 1991b. Boron, pH and inoculum concentration influencing colonization by Plasmodiophora brassicae. Mycol Res. 95:74–79.
- Williams PH. 1966. A system for the determination of races of Plasmodiophora brassicae that infect cabbage and rutabaga. Phytopathology, 56:624–626.
- Woronin M. 1878. Plasmodiophora brassicae, Urheber de Kohlpflanzen-Hernie. Jb Wiss Bot. 11:548–574.