Abstract
Clubroot disease and the causal microbe Plasmodiophora brassicae offer abundant challenges to agriculturists and biological scientists. This microbe is well fitted for the environments which it inhabits. Plasmodiophora brassicae exists in soil as microscopic well-protected resting spores and then grows actively and reproduces while shielded inside the roots of host plants. The pathogen is active outside the host for only short periods. Consequently, scientific studies are made challenging by the biological context of the host and pathogen and the technology required to investigate and understand that relationship. Controlling clubroot disease is a challenge for farmers, crop consultants and plant pathology practitioners because of the limited options which are available. Full symptom expression happens solely in members of the Brassicaceae family. Currently, only a few genes expressing strong resistance to P. brassicae are known and readily available. Agrochemical control is similarly limited by difficulties in molecule formulation which combines efficacy with environmental acceptability. Manipulation of husbandry encouraging improvements in soil structure, texture, nutrient composition and moisture content can reduce populations of P. brassicae. Integrating such strategies with rotation and crop management will reduce but not eliminate this disease. There are indications that forms of biological competition may be mobilized as additions to integrated control strategies. This review charts key themes in the development of scientific biological understanding of this host-pathogen relationship by offering signposts to grapple with clubroot disease which devastates crops and their profitability. Particular attention is given to the link between soil and nutrient chemistry.
Résumé
La hernie, ainsi que l’agent causal Plasmodiophora brassicae, pose de nombreux défis aux agriculteurs et aux biologistes. Cet agent est bien adapté aux environnements où il évolue. P. brassicae peuple les sols sous forme de spores de réserve bien protégées puis croît activement et se reproduit tandis qu’il est bien à l’abri dans les racines des plantes hôtes. L’agent pathogène est actif à l’extérieur de l’hôte que pour de courtes périodes. Conséquemment, les études qui s’y rapportent sont complexifiées par le contexte biologique propre à la relation hôte-pathogène et par la technologie requise pour explorer et comprendre cette relation. La lutte contre la hernie pose un défi aux producteurs agricoles, aux consultants et aux phytopathologistes à cause des possibilités limitées dont ils disposent. Tous les symptômes n’apparaissent que chez les membres de la famille des Brassicacées. Seuls quelques gènes qui offrent une vive résistance à P. brassicae sont actuellement connus et utilisables. La lutte intégrée est également restreinte par les difficultés relatives à la formulation de molécules qui combinent efficacité et acceptabilité environnementale. Les pratiques culturales visant à améliorer la structure des sols, leur texture, leur composition en nutriments et leur humidité peuvent contribuer à réduire les populations de P. brassicae. De concert avec la rotation et la gestion des cultures, ces pratiques contribueront à réduire l’incidence de la maladie, mais pas à l’éradiquer. Par ailleurs, on note que certaines formes de compétition biologique pourraient être utilisées pour bonifier les stratégies de lutte intégrée. Le but de cet article est de présenter une synthèse des thèmes clés relatifs à l’évolution de la compréhension, sur le plan biologique, de cette relation hôte-pathogène en offrant des balises qui permettent de lutter contre la hernie qui ravage les cultures et en réduit la profitabilité. Une attention particulière est portée aux liens qui existent entre le sol, la chimie des nutriments et l’activité de P. brassicae.
Introduction
Realistic estimates suggest that food losses in the range of 25–30% of production result from the ravages of pests, pathogens and abiotic stresses between crops growing in the field and the plate. These levels of loss are unacceptable by any yardstick and made less acceptable when measured against estimates that the world population will reach 9 billion at least by 2050. That requires the doubling of the world’s food supply even to stand still. It must be achieved against a background of increasing scarcity of water and productive land, the loss of natural biodiversity and the effects of global climate change causing alterations in temperature and the seasonal distribution of precipitation such that some current forms of crop husbandry are likely to become untenable. Against this background of increasing uncertainty is the frightening prediction that human conflicts will increase (Dixon Citation2012a).
After cereals, the brassicas are the most economically important and nutritionally essential crops worldwide. Members of the family Brassicaceae provide mankind with fresh and in some cases processed food derived from the leaves, flowers, stems and roots. Increasingly, it is recognized that consumption of these foods has health benefits which reduce the risks from cancer, heart diseases and strokes, the ailments of affluence. Vegetable oils derived from brassica seed constitute the world’s major source of unsaturated fats carrying similar benefits for human health and welfare. Brassica oilseeds also provide sources of saturated fats used for oils and industrial lubricants. Other brassicas provide animal fodder and forage, food condiments and flowering amenity ornamentals. These are the obvious agricultural benefits of brassicas (Dixon Citation2007). But their biggest biological service for mankind may yet come from a tiny rockcress ornamental and weed Arabidopsis thaliana. This has become the workhorse of choice for plant molecular biology used in laboratories worldwide as a basic model vehicle for unraveling and understanding genetic control of cell and organism form, structure, inheritance, evolution and ancestry in all biological entities. The importance of A. thaliana is illustrated by it being the first plant where the entire genome was sequenced. That places it on par with the human genome (Anon Citation2000; Adams Citation2000).
A consideration of the interactions between pathogen, host and environment
Challenging this fundamentally essential suite of brassica crop plants are numerous pests, pathogens and abiotic stress disorders. Quite probably the one which offers the greatest challenges to biologists and agriculturists is the microbe Plasmodiophora brassicae, which is the causal agent of clubroot disease. This is a member of the order Plasmodiophorales, all of which are obligate parasites (Tommerup & Ingram Citation1971). The challenge for biologists is its nature as a non-axenic, microscopic, single-celled, soilborne microbe which exists as a dormant, well-protected resting spore which at germination releases a mobile bi-flagellate primary zoospore. Existence in the soil moisture films is very short term and following invasion of brassica root hairs, the microbe enters a primary phase of multiplication leading on to secondary stages which are accompanied by massive host cell hypertrophy, which is induced by disrupted growth regulator production in the cortical cells. On completion of multiplication cycles, resultant resting spores are liberated back into the soil in vast quantities. Biologists are challenged by this secretive lifestyle of P. brassicae, where even the occurrence and position of meiosis is still open to speculation (Dixon Citation1981).
The challenges to agriculturists start with the resting spores. Resting spores of P. brassicae are produced in profusion. Soil samples containing tens of millions of resting spores per gram are not unusual in heavily infested land. These spores are very robust and have evolved an intricate protection mechanism of five spore walls composed of fungal chitin and carbohydrates (Moxham & Buczaki Citation1983). This combination renders the spores resilient to degradation by extra-cellular enzymes produced by predatory soil organisms. For the majority of the life cycle, the component stages – zoospores, sporangia and sporangiospores – develop and grow protected within the host body. Only for a short period is P. brassicae exposed to unfavourable environmental conditions, either biotic or abiotic in nature, when it exists as minute, naked, free-swimming primary zoospores (Dixon Citation2006, Citation2009a). It is known that some of the requirements for favourable edaphic environments include: acidic pH values (<pH 6.8), minimal calcium contents, nitrogen in an ammoniacal (N-NH4) state, poor soil structure and impeded drainage such that the soil becomes waterlogged, and temperatures above 15 °C (Dixon Citation2002). For the majority of its life cycle, P. brassicae exists in a highly protected environment. Here, P. brassicae has immediate access to all the nutrition that is required for growth and reproduction. The presence of P. brassicae leads to the disruption of the host growth regulator metabolism and subsequent formation of distorted structures of host tissue which provide this microbe with an ideal habitat (Dixon Citation2009a). Being protected in this manner is probably one reason why chemical control of this microbe proves so difficult to achieve. Potential agro-chemicals need a systemic mode of action, being translocated through the host roots and being capable of moving into the cortical cells. Alternatively, the molecule must be capable of foliar uptake and then transfer downwards into the root systems. That is a mode of transfer which is unusual. The alternative is developing chemical or biological means of control which disrupt either the resting spore or the primary zoospore. Dormant resting spores have a substantial armoury of protection, making their destruction very difficult (Dixon & Tilston Citation2010).
While resting spore germination appears to be triggered by the presence of host root exudates, evidence with regard to whether brassica root exudates are specifically required is confusing. It is likely that an organism which is as highly evolved as P. brassicae would possess a defence against germination being triggered by non-specific exudates. But this is not proven and it is thought that some plants which are not members of the Brassicaceae, such as poppy (Papaver spp.), strawberry (Fragaria spp.) and rye-grass (Lolium spp.), can provide host environments in which the first phases of the life cycle may take place (Kowalski & Bochow Citation1996; Dixon Citation2009b). That indicates that exudates from these plants may encourage resting spore germination. What is clear is that from the period when germination commences to that where there is entry into a host root hair or epidermal cell is the most vulnerable time for P. brassicae. In this period, the microbe is limited in the distance that it may travel by the energy available in the naked cell, which provides locomotion achieved through the swimming action of the two flagellae which move it towards the root hairs (Kageyama & Asanoo Citation2009). The resting spore must also possess a reserve of energy sufficient for the changes which take place within the primary zoospore when it reaches the surface of the root hair and to provide the structures which enable P. brassicae cytoplasm to be injected forcefully into the root hair or epidermal cell. In all of these processes and activities, the primary zoospore exists solely on the energy stores laid down when the resting spore formed within the clubbed roots of its host. This energy is derived originally via the photosynthesis which took place in the host where the resting spores developed. Plasmodiophora brassicae relies, therefore, on storing and transporting energy produced by one host generation for invasion of the next. That means there are considerable inflexible energy limitations placed on the distance that primary zoospores may move. Considerations of the availability of energy in this host-pathogen system have received little attention (Ludwig-Müller et al. Citation2009; Neuhauser et al. Citation2011).
There are also environmental factors which limit primary zoospore movement. There is a requirement for continuous moisture films existing on and between the soil particles in which the primary zoospore can move by swimming movements produced by the two flagellae. Dry and drying soils reduce the capacity of P. brassicae for movement. Wet and waterlogged soils favour the movement of this microbe. But total water-logging of the soil environment is likely to deter movement since the zoospore must also respire during motion in order to convert the potential energy stored as carbohydrates and lipids into kinetic energy. Respiration will demand a supply of oxygen from the soil atmosphere, unless of course P. brassicae has some as yet undiscovered unusual anaerobic metabolic pathways. Given the nature of this organism, suggesting such a trait would not be entirely fanciful. It is also possible that germinated zoospores are moved physically and involuntarily through the soil profile by water movements and in that way are transferred onto root hairs. This pathway is quite likely to be a route by which P. brassicae is washed downwards in the soil profile, reaching the root hairs of well-established, deeply rooted maturing crops. The soil environment must also be chemically favourable towards P. brassicae. The most obvious component of the chemical environment appears to be an acidic pH (<6.5) for mineral soils. High concentrations of hydrogen ions favour P. brassicae whereas large concentrations of calcium are apparently deleterious. Boron has a similar property in relation to the growth and reproduction of this microbe (Dixon Citation2010) and appears to have even greater impact on the metabolism of P. brassicae. The calcium effect is particularly intriguing since a closely related organism, Spongospora subterranea var. subterranea, the causal organism of powdery scab disease of potato, is encouraged by an environment with large amounts of calcium and is inhibited by high concentrations of hydrogen ions. One of the challenges which P. brassicae poses for agriculture is the correct and sustainable use of forms of calcium as feasible control system. Success or failure of infection by P. brassicae also relies on the presence or absence of other chemical elements (Dixon Citation1996; Dixon & Page Citation1998). Only in the last couple of decades has reliable research-based evidence suggested how these chemical elements affect P. brassicae. Previously, there was a great deal of empirical and at times apocryphal information largely coming from field observations and trials in which different rates, formulations, timing, pathogen concentrations and distributions, times of season and soil type were employed. Frequently, statistical analyses of such trials were either absent or of limited value. The result is that most of these trials could not be compared scientifically with each other or accorded credibility (Dixon & Webster Citation1988).
Heat is the third element of the soil environment which affects the success of primary zoospore movement and infection into root hairs and epidermal cells. Temperatures in excess of 20 °C have been cited as necessary in order to initiate the movement of P. brassicae and subsequent infection processes. In all probability, the field temperature required in practice is considerably less since that figure comes from laboratory experiments. Clubroot symptoms are known to occur at lower temperatures and these must be preceded by movement and infection. Heat is essential for the enzyme reactions which liberate energy within the zoospore cell. Within reason (i.e.,<35°C) enzyme reaction rates increase as temperature rises. Consequently, rising temperatures accelerate zoospore motion and infection. It is considered that temperatures required for symptom development and expression are less than those required for zoospore motion and penetration. There might be scientific justification for that hypothesis since zoospore motion and penetration rely on activities in a vulnerable, single-celled microbe with a very thin wall. Declines or increases in temperature would be rapidly translated into none or enhanced activity by the microbe. Symptom initiation and expression, on the other hand, result from metabolic activity in large, complex, multicellular angiosperm hosts and might be less influenced by changing temperatures. The soil environment in which P. brassicae and its hosts reside is biologically complex. Research indicates considerable interaction between P. brassicae and other microbes (Einhorn et al. Citation1991). Results suggest that predatory microbes are able to disable P. brassicae and that some of their secondary products can inhibit the metabolic activities of the pathogen. The bacterium Bacillus subtilis appears to engage with P. brassicae in a vigorously antagonistic manner (Feng, Guoro et al. Citation2012). There are also suggestions that aromatic plants such as mint (Mentha piperita), basil (Ocimum basilicum), bardana (Arctium minus), chive (Allium fistulosum) and parsley (Petroselinum crispum) may mitigate the severity of clubroot disease (Hasse et al. Citation2007).
Host biology
Relationships between P. brassicae and host brassicas result in either susceptibility or resistant reactions and in some instances the expression of partial resistance. These interactions between the microbe and different host genotypes appear to be complex, or maybe that is simply a function of the small number of scientists who have elected to undertake research in this area (Crute et al. Citation1980; Dixon Citation1980; Gustafsson & Fält Citation1986). Evolution of resistance happens geographically where the pathogen and host come into co-existence. Original ancestral wild brassicas came from north-eastern Africa, possibly not too far from the regions where early humans developed and then spread out across the Middle East, Europe and Asia. Ultimately, some ancestral brassica types which were plants inhabiting arid or possibly semi-desert areas became adapted for life on maritime coasts in the Mediterranean basin. Others moved considerable distances and laid the genetic foundations for Asian brassicas (mainly forms of B. rapa). Still others moved towards the Atlantic seaboard of Western Europe, the Iberian Peninsula and towards what is now Scandinavia. In some of these areas, the natural mutability of the Brassicaceae expressed itself by the development of amphidiploid, fertile new sub-species and species such as B. napus. Additionally in these areas, an enormous range of cultivated forms developed through evolution in domestication. The result is the vast multiplicity of phenotypes found currently within this family. The family Brassicaceae is the most flexible and mutable of all angiosperms by virtue of both natural and artificial hybridization. Consider the huge range of Occidental brassicas within the species Brassica oleracea which contains all of the European brassica vegetables and then compare that with the equally huge range of Oriental brassicas within the species B. rapa (B. campestris) providing the Asian brassica vegetables. Almost every individual crop form found in Oriental types is matched by an Occidental form (Toxopeus et al. Citation1984; Dixon Citation2007).
At some stage, a soil-borne microbe which was an ancestor of P. brassicae came into contact with some of the evolving brassicas. Where and under what circumstances this encounter took place has yet to be uncovered. The encounter resulted in a single-celled vulnerable microbe taking up residence in the lumen of root hairs and exploiting the food sources available within this environment. Ultimately, that microbe was able to complete a life cycle by further multiplication in the cortical cells of the host. Where is it likely that this encounter was most successful – with wild brassicas or with early ancestral cultivated forms? The scant evidence available suggests that P. brassicae is a parasite of cultivation. Infrequently, it is found on native plants although it is fairly commonly present on weeds in cultivated fields. Additionally, surveys of wild brassica species sampled around the Mediterranean Basin failed to detect the presence of P. brassicae (Mats Gustaffson, personal communication). More likely, the pathogen encountered relatively well-fed and irrigated cultivated forms of brassica and exploited their succulence and the readily available energy sources. Whether that encounter took place once in a single location or several times and in different places is a fascinating question associated with all this speculation which ultimately molecular biology may be able to answer.
Studies of clubroot – descriptive records
It is evident that in the Ancient World, there was increasing interest in observing agricultural crop problems and conditions similar to clubroot are recorded. The Romans were aware of root symptoms which they described as spongy fungus-like roots (radices fungosae). Pallatius suggests avoiding stable manure and chaff (Böhner Citation1922) so very early on, a connection had been established between animals eating malformed roots and spreading a disease of crops through their infested faeces. Subsequent early records come from Albert the Great of Cologne (AD 1196/1206 to 1280) who had widely travelled in the Spanish Low Countries (now parts of Belgium and the Netherlands) and Italy. In both locations, brassicas were increasingly important sources of food for man and his animals. Albertus noted spongy, fungus-like roots which have been interpreted as a record of clubroot. Later infections in Spain are recorded on cabbages in the 15th century where cabbages are described as being syphilitic [Chupp (Citation1934) – translation of Woronin (Citation1878) and Lancereaux (Citation1869)]. Prominence is given to the disease in England and Scotland through the 18th and 19th centuries co-incidentally with the agricultural revolution [such as Anderson (Citation1855)] and possibly following the development of swedes and turnips as parts of the Norfolk four-course rotation. Causes ascribed to conditions similar to clubroot include infection by ‘worms’, unsatisfactory soil and poor crop nutrition, particularly the use of unbalanced fertilizer applications as summarized by Karling (Citation1968). He also describes the subsequent spread of records of the incidence of what could be interpreted as ‘clubroot disease’ emerging across northern Europe and into lands which were colonized by European migrants in the 19th and 20th centuries. Karling (Citation1968) also records the multiplicity of vernacular names given to clubroot in European countries. This multiplicity indicates the widespread nature of the disease and its importance for the general population as a cause of devastation to an important set of food crops.
Initial scientific studies
Fortunately, in the mid-19th century, these disease conditions interested the microbiologist Michael Woronin who was working in St. Petersburg, Russia where he lectured in mycology. In northern parts of Europe and western Asia, considerable reliance was placed on the production and storage of cabbage as vital winter supplies of human food and animal fodder. In the 19th century, losses due a disease which turned out to be clubroot were escalating and Woronin took up its study. Previously, he had travelled widely in Western Europe studying botany and mycology under authorities such as Anton de Bary in Freiburg and Holle in Heidelberg. Consequently, he was knowledgeable and had a network of proficient mentors with whom he could correspond and consult. In a 3-year period (1873–1876), Woronin established the identity of the causal agent of clubroot which he classified as Plasmodiophora brassicae, made a description of symptoms, identified key hosts and salient parts of the life cycle. He also established the taxonomic relationships ascribing P. brassicae as belonging to the Protista (as then defined by E. Häckel) (Chupp Citation1934, p. 20). Woronin’s detailed cytology and taxonomic work established the scientific credentials of this organism and its relationship with clubroot symptoms. His advice for its control included sanitation involving removal of infested plants, the elimination of diseased seedlings prior to transplanting, the use of crop rotation and dipping transplant roots in a suspension of soot. This latter probably contained some heavy metals which are now known to be antagonistic to P. brassicae.
Woronin (Citation1878) as translated by Chupp (Citation1934) identified P. brassicae as a protist which resembled the Myxomycetes but differed in the absence of a true sporangial membrane. Plasmodiophora brassicae has subsequently been re-classified several times [for example Cook and Schwartz (Citation1930)]. The movement of P. brassicae through the Proteomyxa of the Protozoa, Mycetozoa, Chytridiales, Archimycetes and many other classifications is catalogued by Karling (Citation1968) and Buczaki (Citation1983). The organism is now placed in the order Plasmodiophorales, family Plasmodiophoraceae (Braselton Citation1995).
Establishing pathogen biology and host responses
Research over the following three-quarters of a century is summarized in the monographs produced by Colhoun (Citation1958) and Karling (Citation1968). Both provide substantial accounts of the life history and reproduction of the pathogen and host reactions. Damage caused by clubroot was substantial in some geographical areas. For instance, three-quarters of the swede crops in the north-east of Scotland, an essential component of the agricultural system which demanded overwintering fodder for sheep, were considered as infected to some degree (Elizabeth Gray, Aberdeen (deceased), personal communication). That resulted in the breeding of swede ‘Bruce’ which exhibited resistance to clubroot in that region. While in Wisconsin, USA substantial disease on cabbage in the 1930s resulted in the selection of the resistant cultivar ‘Badger Shipper’ (Walker & Larson Citation1960). More generally, research focused on establishing the distribution of resistance genes in hosts. It was soon evident that monogenic resistance is concentrated in B. rapa while B. napus and B. oleracea contained numbers of genes of small effect providing forms of field or polygenic resistance. Assessments of resistance in cultivars and strains of host brassicas are listed by both Colhoun (Citation1958) and Karling (Citation1968), but it was not possible at that time to provide logical commentary on reasons for the distribution of genes within host lines. Since then there has been substantial progress in understanding the genetic basis for resistance as reviewed by Diederichsen et al. (Citation2009).
Attempts at defining the life cycle of P. brassicae while following on from Woronin’s classical work were hampered technically by the non-axenic nature of the pathogen and consequently the protracted time required for completion of studies using seedling plants. Ingram and co-workers (Ingram Citation1969; Ingram & Tommerup Citation1972) added substantially to an understanding of the life cycle. They intended using tissue culture and callus culture in order to take this work further. But it was realized that the ploidy of the cultures becomes unstable and hence could influence the resemblance of results to the nature of events in soil. At the same time, Butcher et al. (Citation1974) achieved considerable success in understanding the intimate relationship between host and pathogen by the use of electron microscopy. Studies in the Netherlands (Dekhuizen & Overeem Citation1971) initiated the contention that symptoms reflect disrupted host growth hormone metabolism. Gustaffson and Fält (Citation1986) also observed that as the pathogen passed through the cortical cells, hypertrophy resulted. This is support for the view that P. brassicae is well evolved and once established inside host tissue, is unlikely to re-emerge and be exposed to the rigours of the soil environment as suggested in some putative life cycles.
Collaborative European research evolved the European Clubroot Differential (ECD) series which replaced a multitude of sets of differential phenotypes which had been used worldwide by many different laboratories, making comparability of results difficult to establish, evaluate and compare. Using this set, it was possible to undertake a worldwide study of resistance reactions (Toxopeus et al. Citation1986). That made for opportunities for breeding resistant cultivars, and in the 1980s, interest in the disease was stimulated because of epidemics developing in European spring-sown oilseed rape. The ECD series helped resolve problems relating to the possible existence and importance or otherwise of physiological races of P. brassicae. Jones (Citation1981) and Jones et al. (Citation1982a, Citation1982b) using ECD demonstrated the highly variable nature of the pathogen micro-geographically, while Toxopeus et al. (Citation1986) established that macro-geographically variation is related to cropping sequences. Following that, Voorips and Visser (Citation1993) refined the use of the ECD series and single-spore isolates for plant breeding purposes.
Asian researchers especially in Japan produced a stream of work which identified further details of pathogen reproduction [reviewed by Kageyama and Asanoo (Citation2009)]. This was stimulated by the important uses of Asian brassicas (B. rapa) in the diet and growing concern for the environmental consequences of applying agrochemicals, which contained heavy metals such as mercury and chlorinated hydrocarbon compounds. The evaluation of resistance became part of the regular assessment of cultivar characteristics.
Studies by Williams and co-workers (Williams Citation1966; Williams et al. Citation1971; Aist & Williams Citation1971) in the USA established the cytological and biochemical details of the secondary phase of the life cycle in considerable detail. Host metabolism is badly disrupted and it became evident that galling symptoms resulted from disrupted host growth regulator metabolism. They also defined in considerable detail the sequence of events taking place when the contents of primary zoospores are physically injected into root hairs. Once attached to the root hair, the zoospore flattens and the flagella coil around the zoospore which at this stage is filled with ribosomes, lipid bodies, mitochondria and various vesicles. The flagella are retracted into the zoospore body and then absorbed. The zoospore body ‘rounds up’ and along a tubular vesicle, the ‘rohr’ develops which is bound by a cyst plasma membrane. The open end of the rohr is directed towards the host cell wall. Contained within the rohr is a sharp pointed rod, ‘the statchel’. The vacuole enlarges, filling half of the cyst, and the end of the rohr swells, forming an adhesorium, which enables the cyst to attach onto the root hair. Pressure is created inside the cyst by the enlargement of its vacuole and that forces the statchel through the adhesorium and into the host cell wall. The zoospore protoplasm is injected into the host cell upon evagination of the rohr, forming a primary plasmodium within the host root hair. The infection process is considered to be physical as no enzyme involvement has yet been detected.
Defining the environmental conditions conducive or suppressive to clubroot forms a major part of research interest linked with studies of the effects of soil structure, texture and nutrient composition (Rouxelm Citation1991). This was based on the seminal studies by Garrett in the Cambridge University Botany School in the 1940s (Samuel & Garrett Citation1945; Garrett Citation1956) which tracked root hair infection and outlined the environments conducive to invasion and colonization. Samuel and Garret (Citation1945) found that the level of root hair infection increased with greater resting spore densities and that at high alkalinity (pH 7.2), infection only occurred at the higher resting spore concentrations of 106 and 107. At a pH of 6.2, however, infection occurred at all spore concentrations. This was later supported by the work of Colhoun (Citation1952 Citation1953) who also concluded that the level of clubroot infection increases with increasing resting spore concentration. His studies provided a comprehensive analysis of environmental factors which interact with pathogen and host characteristics and lead to symptom expression.
In support of agricultural and horticultural practice, very considerable efforts were invested in attempts at finding means of chemical control of clubroot. Many of the compounds evaluated failed to enter commercial use because of their potentially damaging effects on the environment. The impetus underpinning the interest of agro-chemical companies resulted from the increasing worldwide importance of clubroot disease. Regrettably, however, the results of such initiatives have largely failed to be taken up in Europe (Dixon & Wilson Citation1994; Dixon et al. Citation1994). Elsewhere, chemicals such as flusulphamide have considerably eased the problems which intensive growers of vegetable brassicas face with this disease, and flusulphamide is now widely used in Japan and New Zealand (Donald & Porter Citation2009).
From the 1960s through to the 1980s, research interest in clubroot waxed and waned with centres of excellence being established briefly, shining brightly for a few years, and then disappearing as key personnel moved elsewhere. Funding for research into clubroot and P. brassicae has been limited. This is at least partially due to the extent of damage and subsequent crop losses being difficult to quantify. This is ascribed to the ease with which clubroot as a cause of crop loss is identified and therefore advisory staff made field-based diagnoses (Joseph Lester, personal communication). Subsequently, they failed to retain adequate records since advice normally consisted of a statement such as ‘don’t grow brassicas there again for at least 5 years’. Additionally, the hosts of economic importance were solely vegetable brassicas. Although these are highly valuable on an individual plant basis, the overall value was regarded as small relative to large areas of broad hectare agricultural crops. Hence clubroot was noted as a disease of ‘minor’ crops for which establishing a cost and benefit formula was at that time difficult. That attitude was made worse by the single failure of one or two high profile European research programmes to produce substantial returns commensurate with the funding invested. Consequently, studies of clubroot were academically unpopular and classed by funding agencies as ‘difficult and unlikely to yield substantial results which are cost-beneficial’.
Epidemiology, host physiology and environmental biology
From the 1980s to the present day, there has been increasing scientific interest in P. brassicae possibly because of the huge effects of infection on host growth regulator metabolism. At the same time came recognition of clubroot disease as a major agricultural problem (Dixon Citation2009c). This possibly reflects recognition of its worldwide impact on a larger range of brassica crops, including some oil seeds and vegetables destined for export trades. Stimulated by disease development in spring-sown oilseed rape in Europe, breeding programmes built around the use of major genes taken from B. rapa developed in Germany and Scandinavia [e.g. those reported by Diederichsen et al. (Citation2006) and Wallenhammar et al. (Citation2000)]. An active programme charting field epidemiology in oil rape crops in Sweden determined the half-life and capacities for disease spread. In these studies, Wallenhammar (Citation1996) established that the half-life of P. brassicae resting spores is 3.6 years. It would therefore take 18 years, in the absence of a suitable host, for a field population of P. brassicae to decrease to 3% of the original spore population. This finding added much needed scientific clarity to estimations of the time needed for crop rotations which have been long advocated as one means of control.
Clubroot has been a scourge of overwintered cauliflower production in France and during this period, studies demonstrated that suppressiveness could be identified in some soils in Brittany, the major area of production. Extensive programmes over several decades studied potential resistance across cruciferous genomes (such as Delorme et al. Citation1998; Jubault et al. Citation2008); Japanese workers had a long-term interest in clubroot stretching back many decades. This interest produced major studies of the life cycle, understandings of interactions with other soilborne organisms, the effects of using crop rotation and non-host crops and detailed aspects of host-pathogen physiology which is now being expressed in considerable interest in biological and integrated control (e.g. Narisawa et al. Citation2005). Breeders produced a stable of new cultivars of Chinese cabbage with varying levels of resistance to clubroot disease (Yoshikawa Citation1993).
There was a long-term interest in the host-pathogen physiology in the Free University in Berlin which produced more details relating to the life cycle and other aspects of P. brassicae. This also led to a major programme concerned with the effects of the pathogen on host physiology led by J. Ludwig-Müller and J. Siemens. Eventually, this programme relocated to Dresden and has continued very productive work (Ludwig-Müller et al. Citation2009, pp. 239–240). These authors discussed and summarized many years of study in the following analysis: ‘The overwhelming of host defence in the first 12 hours after infection results in the down-regulation of reactive oxygen species (ROS) metabolism. It appears that host defenses then recover after 1–2 days and ROS cell death is minimal during the entire progression of gall formation. Cytokinin biosynthesis is up-regulated after 3 days in combination with the parallel down-regulation of adenosine kinase, catalyzing the conversion (inactivation) of active cytokinin ribosides to the corresponding ribotides. Auxin synthesis is gradually increased by inducing myrosinases and nitrilases. Along with the host defense, metabolism (S-adenosylmmethionine (SAM) synthetase and glycolysis) and protein degradation and signaling mechanisms (more specific proteasome proteins and chaperonins) are overwhelmed in the first 12 hours after infection and up-regulated thereafter. Because SAM is also a precursor of ethylene and polyamines it is possible to predict that these plant growth regulators might also have a similar pattern. Cellular defense (via chitinase, lignin biosynthesis, phenol synthesis, tubulins, peroxidases) is down-regulated during the entire primary stage of the infection process. Finally energy production is gradually increased from 4 to 5 days after infection. This latter corresponds with the functioning of the plasmodia as metabolic sinks’. This builds on original studies such as Keen and Williams (1969) and Kavanagh and Williams (Citation1981).
Advances in molecular biology generally produced research technology and tools which enabled previously unattainable results to be achieved in the 2000s. The intriguing relationship between P. brassicae and brassica hosts offers molecular biologists fertile areas for study. Since the model plant for much of molecular biology research, A. thaliana, is a brassica species, this adds impetus to such studies. Much progress has been achieved in identifying clubroot resistance genes (CR). Over 20 quantitative trait loci (QTL) have been mapped in different linkage groups of B. oleracea, along with the dominant genes already recognized in B. napus (Piao et al. Citation2009). The impetus for this work in South Korea has stemmed from the economic and social importance of Chinese cabbage as a vital part of their diet. Similar and comparable work has developed in Europe where the emphasis has focused more predominantly on understanding virulence in the pathogen. Chromosomes of P. brassicae varying from 2.2 Mb to 680 kb have been characterized and the total genome is estimated at 20 Mb (Siemens et al. Citation2009), which has recently been increased to 24 Mb (Schwelm, personal communication). The genomic gene structure and cDNA structure of several genes has been elucidated and the expression of these genes linked with the development of clubroot. The sequence data support the inclusion of P. brassicae in particular and the plasmodiophorids more generally in the Cercozoa (Siemens et al. Citation2009). Combining knowledge of resistance in brassicas with that of virulence in the pathogen provides a robust platform from which the development of resistant cultivars may proceed. These are vital and increasingly important tools needed for the control of the disease, especially where it causes destruction in broad hectare crops, such as oilseed rape, in which the intensive mitigation measures used on vegetable brassicas cannot be deployed for practical reasons.
Combining molecular biology with suppressiveness and gaining control
It is now possible to see research opportunities which offer both avenues for deeper understanding of the relationship of P. brassicae with its environment and increased capacities for controlling the clubroot disease. Even before Woronin, it was realized serendipitously that P. brassicae and clubroot disease are favoured by high concentrations of hydrogen ions in the soil, i.e. those with acidic pH. Soils with high calcium content and pH > 7.0 are antagonistic to the pathogen and the disease. Why should this be? Especially, as other members of the Plamodiophorales react quite differently (e.g. S. subterranea which causes powdery scab of potatoes). Throughout the history of clubroot research, there has been a long trail of field studies attempting to understand the effects of different forms of calcium. Much of this research is of limited value since it had been done with little regard to many uncontrolled biological and agricultural variables. In early studies, the optimum pH for clubroot infection was considered as approximately 6.0 (slightly acidic soils) (Macfarlane 1952; Colhoun Citation1953). Alkaline soils were thought to reduce symptom development, and it was recommended for good control of clubroot that the pH of the soil should be raised to between 7.3 and 7.5 (Anon Citation1984). The debate is as to whether the alkalinity of the soil is responsible for decreased infection or whether this is partly due to the increase in calcium ions (Dixon & Webster Citation1988; Webster & Dixon Citation1991a, Citation1991b). Earlier work by Samuel and Garrett (Citation1945), in which soil alkalinity was altered using nutrient solutions which did not contain calcium, suggested that it was the direct effect of pH which decreased the level of infection. A soil pH greater than 7.2 was found by Myers and Campbell (Citation1985) to decrease primary infections due to abortion of primary thalli before the release of secondary zoospores. At a soil pH greater than 8.0, thalli that did develop zoospores were misshapen and aborted and no clubs developed. This supports the contention that secondary zoospores are necessary for gall development. Infection can occur in alkaline soils if the soil inoculum levels are sufficiently high (Colhoun Citation1953; Karling Citation1968). The importance of soil moisture content has long been hinted at in the literature, but Thuma et al. (Citation1983) established that there is a direct relationship between increased soil moisture content and the level of primary infection, such that as the moisture content of the soil rose, the level of infection increased.
Work by Webster (Citation1986) clearly demonstrated that elevated calcium concentrations in the soil environment retarded the rate of maturation of P. brassicae in root hairs, reducing the speed and quantities of plasmodia which matured into sporangia. Her detailed studies quantified these effects, showing that the quantity of calcium in the rhizosphere could be related to changes in the reproductive rate and maturation of P. brassicae. There had been debate as to whether raising the soil pH is responsible for the decrease in P. brassicae infection or the presence of calcium ions. This was resolved by some elegant experiments involving the use of organic buffers by Webster (Citation1986), who determined that calcium and pH effects were separate but complementary in relation to the promotion and diminution of the growth of the pathogen. She also determined that boron had similar but more intense effects compared with calcium and that the forms of nitrogen present could also regulate the success or failure of the host-pathogen interaction. Boron at elevated concentrations also appeared to be associated with the diminution of the secondary phase of the P. brassicae life cycle and symptom expression was reduced by high concentrations of boron in the root environment. Craig and Dixon (Citation1993) reinforced these findings with studies of root hair infection rates in the presence of elevated boron concentrations. Subsequently, Page (Citation2001) clearly demonstrated that a combination of calcium and nitrate-nitrogen was antagonistic to the growth and reproduction of P. brassicae. By contrast, ammoniacal-nitrogen encouraged the life cycling of P. brassicae. She also demonstrated that repeated field applications of calcium compounds could encourage the development of soil suppressiveness which inhibited the development of disease. In parallel with these studies, Dixon (Citation2012b) has reported that calcium cyanamide fertilizer is considered to stimulate the development of soil microbes which are antagonistic to P. brassicae. It is evident from these studies that calcium, boron and nitrate-nitrogen have suppressive effects which may act synergistically. This evidence provides some scientific basis for the long-term practice of liming soil, changing soil chemistry so that hydrogen ion concentration is reduced, and there are increased quantities of calcium and other elements in the rhizosphere. In all probability, the relative concentrations of these molecules in soil moisture have a retarding effect on the ability of primary zoospores of P. brassicae to reach the root hair, and supports changes to the flora associated with the rhizosphere and root hair surfaces, such that penetration becomes more difficult and, importantly, the internal environment which P. brassicae primary zoospores experience once inside the host becomes less conducive for growth and reproduction. Changing soil chemistry is a well-established farming practice and there are now some good biological reasons underpinning its efficacy. Dixon and co-workers (Dixon Citation2010) spent a couple of decades attempting to inject some scientific understanding into the relationship between the pathogen, the hosts and calcium and other mineral soil constituents. Their results demonstrate that there is a dynamic and integrated relationship. Calcium and boron affect the primary zoospore and the attendant invasion and colonization stages of P. brassicae. Boron is additionally involved in the secondary symptom development and expression stages. Forms of nitrogen may also be involved in some of these events. Pathogenesis results from a sequence of interactive events firstly in the soil and then within the host.
In the last decade, research has re-focused on the opportunities for direct biological control of P. brassicae. Research into epoxydon (5-hydroxy-3-(hydroxymethyl)-7-oxolate bicyclo [4.1.0] hepta-3-en-2-one) may pave the way for a new range of agrochemicals. Epoxydon was obtained from a culture of Phoma glomerata (No. 324 = JCM 9972) from the leaves of Viola spp. Comparisons of the effectiveness of epoxydon with several anti-auxins indicated that the anti-auxin 2, 3, 5 tri-iodo benzoic acid was the active ingredient (Arie et al. Citation1998). More recently, Bacillus subtilis has shown promise in commercial preparations as a bio-control agent (Lahlali et al. Citation2011; Li Xing Yu et al. Citation2012).
Biological control was defined by Garrett (Citation1956) as ‘any conditions under which, or practice whereby, survival or activity of a pathogen is reduced through the agency of any other living organism (except man himself), with the result that there is a reduction in the incidence of the disease caused by the pathogen’. Biological control not only includes individual organisms which reduce disease but also husbandry methods, such as intercropping, flooding, rotations, and the application of manure. These measures which influence the biological components of the habitat are referred to as indirect biological control (Campbell Citation1989).
Some soils which are suppressive to P. brassicae have been identified. In some instances, the soils did not remain suppressive after sterilization, indicating that a biological agent could be responsible but that the physical and chemical properties of the soil were potentially also responsible for the reduction in disease intensity (Hseih & Jaw-Fen Citation1986; Jaw-Fen & Wen-Hsui Citation1986; Tsushima Citation2000; Workhu & Gerhardson Citation1996). It seems likely that suppressiveness could result from a combination of microbial action conditioned by the physical and chemical environment present in the soil. Rouxel (Citation1984; Rouxel et al. Citation1988) studied the receptiveness of soils in Brittany to P. brassicae and concluded that biotic suppressive soils were present and that their performance was influenced by soil pH. This indicated the presence of a specific type of microflora but the bio-control agent was not identified. Factors which influence the suppressiveness of a soil or compost include soil texture, pH, moisture content, organic matter content and mineral or nutrient composition. Suppressiveness may, therefore, be encouraged by altering these factors (Broadbent & Baker Citation1974; Boehm & Hoitink Citation1992). The action of physical or chemical factors which enhance the soil suppressiveness are also likely to increase the capacities of microorganisms for biological control (Campbell Citation1989). Therefore, the addition of organically derived factors, such as chitin and seaweed extracts, to the soil environment can alter its suppressive qualities and help to decrease disease development. These findings suggest that a more holistic approach to clubroot disease control may be the most fruitful.
The work of Dixon and Webster (Citation1988) goes some way towards explaining the occurrence of soils which are suppressive to P. brassicae but not due to biotic factors. Such soils have been identified in Taiwan. These soils had a pH greater than 7.4 and a calcium content of 1210 ppm (Hseith & Wang Citation1986). The latter used suppressive soil samples with a pH of 7.3 and a calcium content of 1460 ppm. When the suppressive soil was diluted with conducive soil, the pH was altered to 6.7 and the calcium content to 1210 ppm. Suppressiveness was lost from soils when they were acidified with sulphuric acid but returned when sodium carbonate was applied. Acidification of the soil increased the level of calcium by the dissolution of calcium compounds. As a result the availability of calcium decreased due to the higher concentrations of hydrogen ions.
The fertilizer calcium cyanamide provides a classic example of the manner by which soil suppressiveness to P. brassicae may be encouraged by the manipulation of soil chemistry, which then encourages the development of microbial flora which are antagonistic to the pathogen. This fertilizer is well established and environmentally benign (Dixon Citation2012b). The activity of calcium cyanamide in relation to P. brassicae infection was recognized as long ago as Citation1928 by Kindshoven. Since that date, there have been numerous studies which related the application of this fertilizer with enhanced microbial activity and the suppression of clubroot disease.
This approach was continued and developed in Australia from the 1990s onward, where clubroot became a major scourge of vegetable brassicas especially cauliflower in Victoria and Western Australia around Perth. Some of these crops are destined for export to neighbouring countries and hence had substantial market value. The programme and its significant results are summarized by Donald (Citation2005) and Donald and Porter (Citation2009). Their detailed work built on and supported that of previous researchers. Their research confirmed that evidence supports the benefits of long rotations, increasing soil pH using liming compounds including calcium cyanamide, applications of calcium and boron and the need for agrochemicals such as flusulphamide and fluazimam. During their work, they developed strategies for the simple and practical hygiene measures which are of especial importance where brassica transplants are being raised in bulk by professional propagators for onward delivery to crop producers. Resistant cultivars of vegetable brassicas and oilseed rape emerged during this period of time (Diederichsen et al. Citation2006; Hirai Citation2006), and these formed important parts of an integrated control programme. Indeed, the use of a range of strategies for control of clubroot disease is essential in order to preserve and protect resistant cultivars from erosion due to the changes in the spectrum of virulence dominating in populations of P. brassicae. This is especially important since Diederichsen et al. (Citation2009) found that isolates of the pathogen display great variation and show tendencies for overcoming resistance sources from either B. rapa and B. oleracea. At present, resistance genes from B. rapa (stubble turnip types) are the most effective and widely used. The biological progress made in mapping resistance and virulence genes should be used in practice by breeding programmes, with the aim of broadening the genetic basis for clubroot resistance by using both natural variation and transgenic strategies (Diederichsen et al. Citation2009).
It is essential that robust diagnostic tools are utilized in order to quantify the inoculum potential (sensu Garrett) and track the efficacy or otherwise of remedial mitigation husbandry. Molecular biology offers methods based on the polymerase chain reaction (PCR) which provides opportunities for assaying soil, tissue and water samples (Faggian & Strelkov Citation2009). They note that, in addition, serological-based tests could, if available, offer a straightforward field testing system.
The Canadian epidemic in canola (oilseed rape, B. napus) crops (Howard et al. Citation2010; Hwang et al. Citation2012) has resulted in the largest and adequately funded programme seen so far. Robust diagnostic tools (Rennie et al. Citation2011) have been developed and put into service to evaluate disease development and spread. These substantial studies of disease epidemiology have shown new and previously under-appreciated means of spread, produced solid basic research results on the disease and pathogen cycles and helped lay the foundations for genetically modified cultivars which are coming on stream from private and public sources (Hasan et al. Citation2012). Systems for the amelioration of soils as part of integrated control have been investigated (Hwang et al. Citation2011). This research programme has investigated the effects of boron on reducing the growth and reproduction of P. brassicae (Deora et al. Citation2011). Alongside all this work runs a strand of more fundamental studies looking at host-pathogen relationships (Deora et al. Citation2012; Feng et al. Citation2012; Gossen et al. Citation2012; Hwang et al. Citation2012). Currently, clubroot is recognized as of major significance in both brassica vegetables and oil seeds in China. The problem there is so huge that special measures are being taken to boost research and attain methods for control.
The approach which preserves and enhances soil integrity linked with capacities for increased agricultural productivity and profitability, offers means by which some of the serious problems which face mankind in increasing food supplies while conserving biodiversity and reducing adverse impacts on the environment may be achieved. This requires a detailed understanding of the complexities of the soil environment in which the hosts of P. brassicae grow and in which clubroot disease develops (). The pathogen P. brassicae and its hosts have been subjected to some detailed scientific studies over the last one and a half centuries. As a result, some of the biological challenges are more clearly understood and some of the agricultural challenges have been reduced. But the combination of P. brassicae and clubroot disease still demands substantial further investigation. This is a biologically intrinsically interesting microbial pathogen with a novel life cycle well adapted to the colonization of hosts which then provide energy supplies for use by its future generations. Understanding the biology of this host-pathogen interaction potentially offers routes for deeper knowledge of cellular metabolism and the manner by which it can be altered in beneficial as well as harmful directions. Some of the interactions between P. brassicae and its hosts in the soil environment have been unraveled over the last two decades. There is now a much enhanced understanding of the effects of soil chemistry on the early vulnerable stage of the life cycle of P. brassicae when naked primary zoospores are released. Additionally, substantial knowledge has been gained of the effects of soil chemistry on the primary invasion and colonization of root hairs. This is summarized in . The practical objective is achieving the suppression of growth by P. brassicae as described in . Agriculturally, there are opportunities for developing far greater understandings of the mechanisms of genetic resistance and how products of plant breeding may be integrated with other controls. Most interestingly in that respect are studies of how rotational cropping alters the microbial flora such that it is antagonistic to P. brassicae. The phenomenon of suppressiveness is now accepted scientifically as a valid area for study and engineering means for developing this are key for sustainable and holistic integrated control strategies. Potentially interweaving crop resistance, rotational cropping and the resultant suppressiveness with biological control and husbandry which tailors soil chemistry and physics towards limiting the disease causing potential of P. brassicae is likely to be the most effective and cost-beneficial agricultural strategy. Now that this disease has penetrated one of the world’s key traded commodity crops produced in Canada and has been admitted to be a scourge in China, which is the world’s most populous country, there are very good reasons for anticipating that this research will proceed.
Fig. 1. (Colour online) Summary of the interactions of physical, chemical and biological factors which form the environment in which the interaction between Plasmodiophora brassicae and brassica hosts takes place.
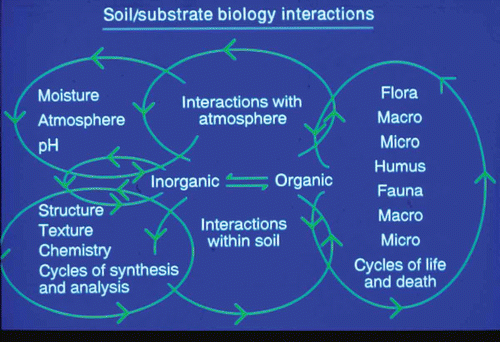
References
- Adams D. 2000. News feature – now the hard ones. Nature. 408:792–793.
- Aist JR, Williams PH. 1971. The cytology and kinetics of of cabbage root hair penetration by Plasmodiophora brassicae. Can J Bot. 49:2023–2034.
- Anderson A. 1855. Report on the disease of finger and toe in turnips. Trans Highland Agric Soc Scotland Ser. 3:118–140.
- Anon. 1984. Agricultural Development and Advisory Service (ADAS) Clubroot Leaflet No 276. London: Her Majesty’s Stationery Office.
- Anon. 2000. (The Arabidopsis Genome Initiative Partnership). Analysis of the genome sequence of the flowering plant Arabidopsis thaliana. Nature. 408:796–815.
- Arie T, Kobayashi Y, Okada G, Kono Y, Yamaguchi I. 1998. Control of soil borne clubroot disease of cruciferous plants by epoxydon from Phoma glomerata. Plant Pathol. 47:743–748.
- Boehm MJ, Hoitink HA. 1992. Sustenance of microbial activity in potting mixes and its impact on the severity of Pythium root rot of Poinsettia. Phytopathology. 82:259–264.
- Braselton JP. 1995. Current status of the Plasmodiophorids. Crit Rev Microbiol. 21:263–275.
- Broadbent D, Baker KF. 1974. Behaviour of Phytophthora cinnamomi in soils suppressive and conducive to root rot. Aust J Agric Res. 25:121–127.
- Buczacki ST. 1983. Plasmodiophora : an inter-relationship between biological and practical problems. In: Buczacki ST, editor. Zoosporic plant pathogens – a modern perspective. London: Academic Press; p. 161–192.
- Butcher DN, El-Tigani S, Ingram DS. 1974. The roles of indole glucosinolates in the clubroot disease of the cruciferae. Physiol Plant Pathol. 4:127–141.
- Böhner K. 1922. Vom Kohlkroof. Nurnberg: Frankischer Kurier; 8. 111.
- Campbell R. 1989. Biological control of microbial plant pathogens. London: Cambridge University Press.
- Chupp C. 1934. A translation of : Plasmodiophora brassicae – the cause of cabbage hernia. In: Woronin MS, editor. 1878. Phytopathological Classics No 4 St. Paul, MN: American Phytopathological Society.
- Colhoun J. 1952. Factors affecting the incidence of club root disease of Brassicae. Nature London. 169:21–22.
- Colhoun J. 1953. A study of the epidemiology of club root disease of Brassicae. Ann Appl Biol. 40:262–283.
- Colhoun J. 1958. Clubroot disease of crucifers caused by Plasmodiophora brassicae a mongraph. A Mongraph. Phytopathological Paper no. 3. Kew, Surrey: The Commonwealth Mycological Institute.
- Cook WRI, Schwartz EJ. 1930. The life-history, cytology and method of infection of Plasmodiophora brassicae Woron., the cause of finger and toe disease in cabbages and other crucifers. Phil Trans R Soc Lond. 218:283–314.
- Craig MA, Dixon GR. 1993. Alterations to the growth of Plasmodiophora brassicae Wor. (clubroot) associated with boron. In Proceedings of the 6th International Congress of Plant Pathology, Montreal, Canada: National Council for Research Canada. Poster 3.4.12 ISBN: 0–660–58038–1.
- Crute IR, Gray AR, Crisp P, Buczacki ST. 1980. Variation in Plasmodiophora brassicae and resistance to clubroot disease in brassicas and allied crops – a critical review. Plant Breed Abst. 50:91–103.
- Dekhuizen HM, Overeem JC. 1971. The role of cytokinins in clubroot formation. Physiol Plant Pathol. 1:151–162.
- Delorme R, Foissart N, Horvais R, Barrett P, Champagne G, Cheung WY, Landry BS, Renard M. 1998. Characterisation of the radish introgression carrying the Rfo restorer gnes for the Ogu-INRA cytoplasmic male sterility in rapseed (Brassica napus L). Theor Appl Gen. 97:129–134.
- Deora A, Gossen BD, McDonald MR. 2012. Infection and development of Plasmodiophora brassicae in resistant and susceptible canola cultivars. Can J Plant Pathol. 34:239–247.
- Deora A, Gossen BD, Walley F, McDonald MR. 2011. Boron reduces development of clubroot in canola. Can J Plant Pathol. 33:475–484.
- Diederichsen E, Beckman J, Jörg Schondelmeier J, Dreyer F. 2006. Genetics of clubroot resistance in Brassica napus ‘Mendel. Acta Hortic. 706:307–311.
- Diederichsen E, Frauen M, Linders EGA, Hatakeyama K, Hirai M. 2009. Status and perspectives of clubroot resistance breeding in cruicifer crops. In: Dixon GR, editor. Plasmodiophora brassicae (clubroot) – a plant pathogen that alters host growth and productivity. Special edition. J Plant Growth Regul, 28, 264–281.
- Dixon GR. 1980. Variation in Plasmodiophora brassicae. Ann Appl Biol. 94:278–280.
- Dixon GR. 1981. Vegetable Crop Diseases. London & Basingstoke: Macmillan Publishers Ltd.
- Dixon GR, Wilson F. 1984. Field evaluation of WL 105 305 (NK 483) for control of clubroot (Plasmodiophora brassicae). Tests of Agrochemicals and Cultivars No 5. Ann Appl Biol. 104(Supplement): 34–35.
- Dixon, GR, Craig, MA, Burgess, PJ, Thomas, J. 1994. MTF 651: a new soil-applied fungicide for the control of plasmodial fungi. In Proc. Brighton Crop Protect. Conf. Pests & Diseases (Session 5A-6), vol 2. Farnham, Surrey: Brit Crop Protect Council; p. 541–548.
- Dixon GR. 1996. Repression of the morphogenesis of Plasmodiophora brassicae Wor. by boron - a review. Acta Hortic. 407:393–402.
- Dixon GR. 2002. Interactions of soil nutrient environment, pathogenesis and host resistance. Plant Prot Sci. 38:87–94.
- Dixon GR. 2006. The biology of Plasmodiphora brassicae Wor., - a review of recent advances. Acta Hortic. 706:271–282.
- Dixon GR. 2007. Vegetable brassicas and related crucifers crop production science in horticulture series No 14 Wallingford, Oxfordshire: Crop Production Science in Horticulture CABI Publishing.
- Dixon GR. 2009a. Plasmodiophora brassicae in its environment. In Dixon GR, editor. Plasmodiophora brassicae (clubroot) – a plant pathogen that alters host growth and productivity. Special edition. J Plant Growth Reg. 28: 212–228.
- Dixon GR. 2009b. Husbandry – the sustainable means of controlling soil borne pathogens:- a synoptic review. In Dixon GR, editor. Proceedings of the Symposium of Horticulture in Europe, Vienna, Austria; 17–21. February 2008. Acta Hort. 817: 233–242.
- Dixon GR. 2009c. The occurrence and impact of Plasmodiophora brassicae and clubroot disease. In Dixon GR, editor. Plasmodiophora brassicae (clubroot) – a plant pathogen that alters host growth and productivity. Special edition. J Plant Growth Regul. 28: 194–202.
- Dixon GR. 2010. Calcium and pH as parts of a coherent control strategy for clubroot disease (Plasmodiophora brassicae). Acta Hortic. 867:151–156.
- Dixon GR. 2010. Calcium and pH as elements in a coherent control system for clubroot. Acta Hortic. 867:151–155.
- Dixon GR. 2012a. Climate change, plant pathogens and food production. Can J Plant Pathol. 34:362–379.
- Dixon GR. 2012b. Calcium cyanamide - a synoptic review of an environmentally benign fertiliser which enhances soil health. Acta Hortic. 938:211–217.
- Dixon GR, Craig MA, Burgess PJ, Thomas J. 1994. A new soil applied fungicide for the control of plasmodial fungi. Proceedings of the Brighton Crop Protection Conference, Pests and Diseases 2: 541–548.
- Dixon GR, Page LV. 1998. Calcium and nitrogen elicting alterations to growth and reproduction of Plasmodiophora brassicae (clubroot). Acta Hortic. 459:343–349.
- Dixon GR, Tilston EL. 2010. Soil-borne pathogens and their interactions with the soil environment. In: Dixon GR, Tilston EL, editors. Soil Microbiology and Sustainable Crop Production (pp. 197–271). Dordrecht: Springer Science Business Media.
- Dixon GR, Webster MA. 1988. Antagonistic effects of boron, calcium and pH on pathogenesis caused by Plasmodiophora brassicae (clubroot) - a review of recent work. Crop Res. (Hortic. Res.). 28:83–95.
- Donald EC. 2005. The Influence of Abiotic Factors and Host Plant Physiology on the Survival and Pathology of Plasmodiophora brassicae of Vegetable Brassicas. [dissertation]. Australia: The University of Melbourne.
- Donald C, Porter I. 2009. Integrated control of clubroot. In Dixon RG, editor. Plasmodiophora brassicae (clubroot) – a plant pathogen that alters host growth and productivity. Special edition. J Plant Growth Reg. 28: 289–303.
- Einhorn G, Bochow H, Huber J, Krebs B. 1991. Methodological studies to detect antagonists of the clubroot pathogen, Plasmodiophora brassicae Wor. Archiv fur Phytopathologie und Pflanzenschutz – Arch Phytopathol Plant Prot. 27:205–208.
- Evans JL, Scholes JD. 1995. How does clubroot alter the regulation of carbon metabolism in its host?. Aspects App Biol. 42:125–132.
- Faggian R, Strelkov SE. 2009. Detection and measurement of Plasmodiophora brassicae. In: Dixon RJ, editor. Plasmodiophora brassicae (clubroot) – a plant pathogen that alters host growth and productivity. Special edition. J Plant Growth Reg. 28: 282–287.
- Feng LQ, Guoru X, Zichao M, Yixin W, Yueqiu H. 2012. Analyses for the colonization ability of Bacillus subtilis XF-1 in the rhizosphere. Acta Phytophyl Sin. 39:425–430.
- Feng J, Xiao Q, Hwang SF, Strelkov SE, Gossen BD. 2012. Infection of canola by secondary zoospores of Plasmodiophora brassicae produced on a nonhost. Eur J Plant Path. 132:309–315.
- Garrett SD. 1956. Biology of Root Invading Fungi. Cambridge (UK): Cambridge University Press.
- Gossen BD, Adhikari KKC, McDonald MR. 2012. Effects of temperature on infection and subsequent development of clubroot under controlled conditions. Plant Pathol. 61:593–599.
- Gustafsson M, Fält A-S. 1986. Genetic studies on resistance to clubroot in Brassica napus. Ann Appl Biol. 108:409–415.
- Hasan MJ, Strelkov SE, Howard RJ, Rahman H. 2012. Screening of Brassica germplasm for resistance to Plasmodiophora brassicae pathotypes prevalent in Canada for broadening diversity in clubroot resistance. Can J Plant Sci. 92:501–515.
- Hasse I, May-De Mio LL, Lima Neto VC. 2007. The effect of pre-plantation with medicinal plants in the Plasmodiophora brassicae control. Summa Phytopathol. 33:74–79.
- Hirai M. 2006. Genetic analysis of clubroot resistance in Brassica rapa. Breed Sci. 56:223–229.
- Howard RJ, Strelkov SE, Harding MW. 2010. Clubroot of cruciferous crops - new perspectives on an old disease. Can J Plant Pathol. 32:43–57.
- Hseih W-H, & Wang J-F. 1986. Investigation on suppressive soils of clubroot crucifers in Taiwan. Plant Prot. Bull. Taiwan Republic China. 28:353–362.
- Hwang SF, Ahmed HU, Zhou Q, Strelkov SE, Gossen BD, Peng G, Turnbull GD. 2012. Assessment of the impact of resistant and susceptible canola on Plasmodiophora brassicae inoculum potential. Plant Pathol. 61:945–952.
- Hwang SF, Strelkov SE, Feng J, Gossen BD, Howard RJ. 2012. Plasmodiophora brassicae: a review of an emerging pathogen of the Canadian canola (Brassica napus) crop. Mol Plant Pathol. 13:105–113.
- Hwang SF, Strelkov SE, Gossen BD, Turnbull GD, Ahmed HU, Manolii VP. 2011. Soil treatments and amendments for amelioration of clubroot of canola. Can J Plant Sci. 91:999–1010.
- Ingram DS. 1969. Growth of Plasmodiophora brassicae in host callus. J Gen Microbiol. 56:55–67.
- Ingram DS, Tommerup IC. 1972. The life history of Plasmodiophora brassicae Woron. Proc. R. Soc. Lond. Ser. B. 180:103–112.
- Jaw-Fen W. 1986. Studies on the suppressive factors and characteristics of suppressive soils of clubroot in crucifers. Plant Prot. Bull. Taiwan Republic China. 28:363–370.
- Jones DR. 1981. Differential Pathogenicity of Plasmodiophora brassicae Wor. [dissertation]. Cambridge (UK): University of Cambridge.
- Jones DR, Ingram DS, Dixon GR. 1982a. Characterisation of isolates derived from single resting spores of Plasmodiophora brassicae and studies of their interactions. Plant Pathol. 31:239–245.
- Jones DR, Ingram DS, Dixon GR. 1982b. Factors affecting tests for differential pathogenicity in populations of Plasmodiophora brassicae. Plant Pathol. 31:229–238.
- Jubault M, Hamon C, Gravot A, Lariagon C, Delorme R, Bouche-Reau A, Manzanzares-Dauleux MJ. 2008. Differential regulation of root arginine catabolism and polyamine metabolism in clubroot-susceptible and partially resistant Arabidopsis genotypes. Plant Physiol. 146:2008–2019.
- Kageyama K, Asanoo T. 2009. Life cycle of Plasmodiophora brassicae. In: Dixon GR, editor. Plasmodiophora brassicae (clubroot) – a plant pathogen that alters host growth and productivity. Special edition. J Plant Growth Reg 28: 203–211.
- Karling JS. 1968. The Plasmodiophorales. New York & London: Hafner Publishing Company.
- Kavanagh JA, Williams PH. 1981. Indole auxins in Plasmodiophora infected cabbage roots and hypocotyls. Mycol Res. 77:125–129.
- Keen NT, Williams PH. 1969. Translocation of sugars into infected cabbage tissues during clubroot development. Plant Physiol. 44:748–754.
- Kindshoven J. 1928. Entseuchhung des Bodens und Bekämpfung der Kohlhernie und Kalkstickstoff. Mitt Deut Landw Gesell. 43:522–523.
- Kowalski K, Bochow H. 1996. Observations on the behaviour of resting spores of Plasmodiophora brassicae in the presence of cruciferous and non-cruciferous plant roots. Acta Hortic. 407:419–422.
- Lahlali R, Peng G, McGregor L, Gossen BD, Hwang SF, McDonald M. 2011. Mechanisms of the biofungicide Serenade (Bacillus subtilis QST713) in suppressing clubroot. Biocontrol Sci Tech. 21:1351–1362.
- Lancereaux E. 1869. A Treatise on Syphilis, Historical and Practical Translation by G Whitley. London: New Sydenham Society; . 2:p. 363.
- Li X, Mao Z, Wang Y, Wu Y, He Y, Long C. 2012. ESI LC-MS and MS/MS characterization of antifungal cyclic lipopeptides produced by Bacillus subtilis XF-1. J Mol Microbiol Biotechnol. 22:83–93.
- Ludwig-Müller J, Prinsen E, Rolfe SA, Scholes JD. 2009. Metabolism and plant hormone action during clubroot disease. In: Dixon GR, editor. Plasmodiophora brassicae (clubroot) – a plant pathogen that alters host growth and productivity. Special edition. J Plant Growth Reg. 28: 229–244.
- McFarlane I. 1952. Factors affecting the survival of Plasmodiophora brassicae Wor. in soil and its assessment by host test. Ann Appl Biol., 39, 239–256.
- McFarlane I. 1955. Variation in Plasmodiophora brassicae Wooron. Ann Appl Biol. 43:297–306.
- Moxham SE, Buczacki ST. 1983. Chemical composition of the resting spore wall of Plasmodiophora brassicae. Mycol Res. 80:17–28.
- Myers DE, Campbell RN. 1985. Lime and the control of clubroot of crucifers: effects of pH, calcium, magnesium and their interactions. Phytopathology. 75:670–673.
- Narisawa K, Shimura M, Usuki F, Fukuhara S, Hashiba T. 2005. Effects of pathogen density, soil moisture and soil pH on biological control of clubroot of Chinese cabbage by Heteroconium chaetospora. Plant Dis. 89:285–290.
- Neuhauser S, Kirchmair M, Gleason FH. 2011. Ecological roles of the parasitic phytomyxids (plasmodiophorids) in marine ecosystems – a review. Marine Freshw Res. 62:365–371.
- Page LV. 2001. Studies of Components for a Potential Integrated Control system for Plasmodiophora brassicae. Dissertation, University of Strathclyde, Glasgow.
- Piao Z, Ramchiary N, Lim YP. 2009. Genetics of clubroot resistance in Brassica species. In: Dixon GR, editor. Plasmodiophora brassicae (clubroot) – a plant pathogen that alters host growth and productivity. Special edition. J Plant Growth Reg. 28: 252–264.
- Rennie DC, Manolii VP, Cao T, Hwang SF, Howard RJ, Strelkov SE. 2011. Direct evidence of surface infestation of seeds and tubers by Plasmodiophora brassicae and quantification of spore loads. Plant Pathol. 60:811–819.
- Rouxel F. 1984. Studies on soil receptivity to clubroot. In Methods for studying the biological control of diseases caused by soil borne pathogens. British Society for Plant Pathology, Anglo-French workshop. 12–13 Sept 1984; London, England: Rothamsted Experiment Station.
- Rouxel F. 1991. Natural suppressiveness of soils to plant diseases. In: Beemster ABR, Bolen GL, Gerlag M, Ruissen MA, Tempel B, Schippers A, editors. Biotic interactions and soil borne diseases Amsterdam: Elsevier.
- Rouxel F, Briard M, Lejeune B. 1988. Studies of soil receptiveness to clubroot caused by Plasmodiophora brassicae: experiments on responses of a series of vegetable soils in Brittany. Progress on Pest Management in Field Vegetables. Proceedings CEC-IOBC Expert Group Meeting, 22–23 Nov, 1985; (pp 145–152). Rennes, France: INRA.
- Samuel G, Garrett SD. 1945. The infected root hair count for estimating the activity of Plasmodiophora brassicae Woron. in the soil Ann Appl Biol. 32:96–101.
- Siemens J, Bulman S, Rehn F, Sundelin T. 2009. Molecular biology of Plasmodiophora brassicae. In G.R. Dixon, editor. Plasmodiophora brassicae (clubroot) – a plant pathogen that alters host growth and productivity. Special edition. J Plant Growth Reg. 28:245–251.
- Thuma BA, Rowe RC, Madden LV. 1983. Relationships of soil temperature and moisture to clubroot (Plasmodiophra brassicae) severity on radish in organic soil. Plant Dis. 67:758–762.
- Tommerup IC, Ingram DS. 1971. The life-cycle of Plasmodiophora brassicae Wor. in Brassica tissue cultures and intact roots. New Phytol. 70:327–332.
- Toxopeus H, Dixon GR, Mattusch P. 1986. Physiological specialisation in Plasmodiophora bassicae – an analysis by international experimentation. Mycol Res. 87:279–287.
- Toxopeus H, Oost EH, Reuling G. 1984. Current aspects of the taxonomy of cultivated brassica species. Cruciferae Newsl. 9:55–58.
- Tsushima S 2000. Perspectives of integrated pest management - a case study: clubroot of crucifers. J Pesticide Sci. 25:296–299.
- Voorips RE, Visser DL. 1993. Examination of resistance to clubroot in accessions of Brassica oleracea using a glasshouse seedling test. Eur J Plant Pathol. 99:269–276.
- Wang J-F, Hseih W-H. 1986. Studies on the suppressive factors and characteristics of suppressive soils of clubroot in crucifers. Plant Prot Bull (Taiwan ROC) 28:363–370.
- Walker JC, Larson RH. 1960. Development of the first clubroot resistant cabbage variety. Wisconsin Agric Exp Stn Bull. 547:12–16.
- Wallenhammar AC. 1996. Prevalence of Plasmodiophora brassicae in a spring oil seed rape growing area in central Sweden and factors influencing soil infestation levels. Plant Pathol. 45:710–719.
- Wallenhammar AC, Johnsson L, Gerhardson B. 2000. Agronomic performance of partly clubroot-resistant spring oilseed turnip rape lines. J Phytopathol. 148:495–499.
- Webster MA. 1986. pH and nutritional effects on infection by Plasmodiophora brassicae [dissertation]. Scotland: University of Aberdeen.
- Webster MA, Dixon GR. 1991a. Boron, pH and inoculum concentration influencing colonisation by Plasmodiophora brassicae. Mycol Res. 95:74–79.
- Webster MA, Dixon GR. 1991b. Calcium, pH and inoculum concentration influencing colonisation by Plasmodiophora brassicae. Mycol Res. 95:64–73.
- Williams PH. 1966. A cytological study of hypertrophy in clubroot of cabbage. Phytopathology. 56:521–524.
- Williams PH, Aist SJ, Aist JR. 1971. Response of cabbage root hairs to infection by Plasmodiophora brassicae. Can J Bot. 49:41–47.
- Workhu Y, Gerhardson B. 1996. Suppressiveness to clubroot, pea root rot and fusarium wilt in Swedish soils. J Phytopathol. 144:143–146.
- Woronin M. 1878. Plasmodiophora brassicae Urheber der Kohlpflanzen-Hernie. Jahrb Wiss Bot. 11:548–574.
- Yoshikawa H. 1993. Studies on breeding of clubroot resistance in cole crops. Bull. Natl. Inst. Veg. Ornam. Plants Tea Jpn. Ser. A. 7:1–165.