Abstract
Fusarium head blight (FHB), caused by Gibberella zeae (anamorph: Fusarium graminearum), is a destructive disease of cereals. Previous studies demonstrated that Clonostachys rosea strain ACM941 is an antagonist of G. zeae that parasitizes the pathogen and reduces FHB severity in wheat. The objective of this research was to evaluate the efficacy of CLO-1, a formulated product of ACM941, for reducing perithecial production on various crop residues in comparison with the registered fungicide Folicur (tebuconazole) under field conditions. When applied on G. zeae inoculated corn, soybean and wheat residues in the spring of 2009 and 2010, CLO-1 significantly inhibited perithecial production on all crop residue types, reducing daily perithecial production (DPP) by 93.1% on corn residue, 94.4% on soybean residue and 84.0% on wheat residue, compared with the untreated control. When applied on naturally infected wheat residues in the autumn of 2009 and 2010, CLO-1 significantly reduced DPP in the following growing season by 72.3% on peduncles, 51.0% on spikelets and 57.2% on stems. These effects were numerically better but not significantly different from those achieved by Folicur fungicide used as a positive control in the same experiments. Results from this study suggest that CLO-1 is a promising biofungicide against G. zeae and may be used as a control measure to reduce the initial inoculum of FHB in an integrated FHB management programme.
Résumé
La brûlure de l’épi causée par le fusarium (BEF), provoquée par Gibberella zeae (forme imparfaite: Fusarium graminearum), est une maladie destructrice des céréales. Des études antérieures ont montré que la souche ACM941 de Clonostachys rosea est un antagoniste de G. zeae: elle parasite l’agent pathogène et atténue la gravité de la BEF chez le blé. Le but de cette recherche était d’évaluer l’efficacité de CLO-1, une préparation à base d’ACM941, quant à la réduction de la production périthéciale sur différents résidus de culture, comparativement au fongicide homologué Folicur (tébuconazole) utilisé en champs. Au printemps de 2009 et de 2010, lorsque appliqué à des résidus de maïs, de soya et de blé inoculés avec G. zeae, CLO-1 a significativement inhibé la production périthéciale sur tous les types de résidus, réduisant la production périthéciale journalière (PPJ) de 93.1 % sur les résidus de maïs, de 94.4 % sur ceux de soya et de 84.0 % sur ceux de blé, comparativement au témoin non traité. Durant l’automne 2009 et celui de 2010, lorsque appliqué à des résidus de blés infectés naturellement, CLO-1 a significativement réduit la PPJ au cours des saisons suivantes de 72.3% sur les pédoncules, de 51.0% sur les épillets et de 57.2% sur les tiges. Ces effets étaient numériquement meilleurs que ceux obtenus avec Folicur utilisé comme solution de contrôle positif au cours des mêmes expériences, mais pas significativement différents. Les résultats de cette étude suggèrent que, comme fongicide biologique, CLO-1 s’avère prometteur contre G. zeae et qu’il peut être utilisé comme mesure visant à réduire la quantité initiale d’inoculum de BEF dans un programme de lutte intégrée contre cette maladie.
Introduction
Fusarium head blight (FHB) is a major disease of cereals worldwide. In Canada, frequent FHB epidemics in the Prairies and in eastern Canada over the past two decades have caused extensive losses due to reduced yield and the discounted price of grains contaminated with Fusarium-damaged kernels (FDK) and their associated mycotoxins, mainly deoxynivalenol (DON) (Gilbert & Tekauz Citation2000; Gilbert & Haber Citation2013). DON poses a health concern in food and feed, by inhibiting protein biosynthesis and affecting the digestive system (Pestka Citation2007; Trail Citation2009; Zain Citation2011) and causing neurotoxic and immunotoxic effects in mammals (D’Mello et al. Citation1999; Desjardins Citation2006). Of the several Fusarium species that are also capable of causing FHB, Gibberella zeae (Schwein.) Petch (anamorph Fusarium graminearum Schwabe) is known to be the major causal agent in North America, although other species including F. acuminatum Ellis and Everhart, F. avenaceum (Corda: Fr.) Sacc., F. equiseti (Corda) Sacc., F. poae (Peck) Wollenw. and F. sporotrichioides Sherb. have been frequently isolated from naturally infected FDK of cereals (Parry et al. Citation1995; Gilbert & Tekauz Citation2000; Xue et al. Citation2004b, Citation2006; McMullen et al. Citation2012; Xue et al. Citation2013). Gibberella zeae produces two types of spores, ascospores (sexual) and conidia (asexual). Although both spore types are equally effective in causing the disease, FHB epidemics are generally considered to originate from ascospores that are formed in perithecia on crop residues (Sutton Citation1982; Parry et al. Citation1995; Fernando et al. Citation2000; Xue et al. Citation2006). Practices for suppressing the production of perithecia on crop residues, such as rotation of cereals with non-host crops and ploughing infested residues, have been reported as effective measures for reducing the initial inoculum and recommended for managing FHB in Canada (Sutton Citation1982; Menzies & Gilbert Citation2003; Gilbert & Haber Citation2013).
Cereal cultivars with high levels of resistance to FHB and DON accumulation in grains are not commercially available. Fungicides have been used to control FHB under high inoculum pressure and favourable environmental conditions, to sustain productivity and grain quality in most wheat production regions (Jones Citation2000; Matthies & Buchenauer Citation2000; Blandino et al. Citation2012; Amarasinghe et al. Citation2013). However, fungicides have not been consistently effective in controlling FHB and reducing DON accumulation (McMullen et al. Citation1997; Jones Citation2000; Matthies & Buchenauer Citation2000; Blandino et al. Citation2006; Wegulo et al. Citation2011). Long-term usage of fungicides has become a public concern because of their perceived impact on the environment (Yuen & Schoneweis Citation2007; Herrero-Hernández et al. Citation2011; Muñoz-Leoz et al. Citation2011, Citation2012; Imfeld & Vuilleumier Citation2012) and their residues may remain in food and feed products, causing a potential risk to human and animal health (Moser et al. Citation2001; Fantke et al. Citation2011).
The application of biological control agents is an alternative to the use of chemical fungicides. Although several microbial agents have been reported as showing promise for biocontrol of FHB, none are currently commercially available in Canada or the USA (Gilbert & Haber Citation2013). Previous studies reported that the bioagent ACM941 (ATCC #74447), a strain of Clonostachys rosea (Link:Fr.) Schroers, Samuels, Seifert and Gams (syn. Gliocladium roseum Bainier), is a mycoparasite of certain fungal pathogens causing root rot of various plant species (Xue Citation2002, Citation2003a, Citation2003b). Recent research also demonstrated that the bioagent ACM941 holds considerable promise as an effective alternative to fungicides for the control of FHB. Foliar application of ACM941 reduced FHB disease severity, FDK and DON in harvested grain, and subsequent crop residue treatment can be used to reduce the initial inoculum of G. zeae (Xue et al. Citation2009a, Citation2009b). The objective of this research was to evaluate the efficacy of CLO-1, a formulated product of ACM941 for reducing perithecial production of G. zeae on different types of crop residues through spring or autumn application under field conditions.
Materials and methods
CLO-1 biofungicide and Gibberella zeae strain
CLO-1 biofungicide is an experimental commercial formulation of the C. rosea strain ACM941 bioagent (ATCC #74447). The product is a wettable powder containing more than 8 × 109 cfu g−1 and was developed using a proprietary technique by Dr. G.E. Harman at Cornell University, NY, USA in 2008. The ACM941 strain used for product development was originally recovered from a field pea plant in 1994 (Xue Citation2002). The fungus was stored by freeze-drying and kept at −20 °C in ampoules. Cultures of the fungus were established by transferring approximately 0.01 g of freeze-dried fungal material to a Petri dish containing potato dextrose agar (PDA) and incubating these dishes at 22–25 °C under mixed long-wave ultraviolet (UV) and fluorescent lighting.
Gibberella zeae isolate DAOM 232369, obtained from the Canadian Collection of Fungal Cultures at the Eastern Cereal and Oilseed Research Centre (ECORC), Ottawa, Canada, was used for this study. This isolate was chosen because it produces an abundance of perithecia in culture and on plant debris as determined by preliminary tests (data not shown). The isolate was cultured on modified PDA (4 g L−1 potato starch, 10 g L−1 dextrose, 15 g L−1 agar, 34 μmol L−1 streptomycin sulfate) and incubated at 22–25 °C under mixed long-wave UV and fluorescent lighting at 12 h light: 12 h dark cycle for 14 days. The modified PDA medium was shown to reduce mycelium growth, possible mutation and poor vigour, and to increase spore production by the pathogen (Xue et al. Citation2004a). To prepare inoculum, 0.5 mL of a concentrated macroconidial suspension (c. 107 spores mL−1) obtained from a culture of the isolate was spread over the surface of modified PDA in 9-cm Petri dishes and incubated as described above for 48 h. Ten millilitres of sterile distilled water containing 0.01% Tween 20 (polyoxyethylene sorbitan monolaurate) was then added to each plate, and the surface was scraped gently with a sterile microscope slide to dislodge spores. The resulting conidial suspension was filtered through two layers of cheesecloth and adjusted to 1 × 105 spores mL−1 using a hemocytometer.
Spring application on corn, soybean and wheat residues
Two identical trials were conducted under field conditions in the spring and early summer each year of 2009 and 2010 at ECORC, Central Experimental Farm, Ottawa, to evaluate the efficacy of spring application of CLO-1 in controlling perithecial production of G. zeae on corn, soybean and wheat residues. Pieces of the respective plant residues (stems and leaves) left on the ground from crops grown in the previous year were collected from the fields in Ottawa in early spring each year. The residues were cut into pieces of 50 × 4 mm for corn, 50 × 5 mm for soybean and 50 × 3 mm for wheat. These residue pieces of each crop type were wrapped in aluminium foil, autoclaved for 30 min, allowed to cool at room temperature, and then divided into two sub-groups. Residues in the first sub-group were treated with the biofungicide or chemical fungicide and inoculated with the pathogen on the same day (0 day). Residues in the second sub-group were treated the same but 3 days ahead of inoculation with the pathogen (+3 days).
For the inoculation, residues were sprayed with a conidial suspension of G. zeae isolate DAOM232369 at a concentration of 1 × 105 spores mL−1. For the treatments, the residues were sprayed with either CLO-1 at 1 × 107 cfu mL−1 or Folicur 432 F (43.2% tebuconazole, Bayer CropScience Inc., Calgary, AB) in a water suspension at 0.292% (vol/vol). The untreated controls were sprayed with sterile distilled water. Both inoculum and fungicide treatments were applied with a 500 mL spray bottle onto the surfaces of residues to incipient run-off and allowed to air dry for 30 min. The inoculated and treated residues were wrapped in aluminium foil and incubated at room temperature for 3 days to allow the mycelium of the pathogen to grow on the surface of the residues. Eighteen pieces of each crop residue were selected at random as the experimental unit and packed in a black nylon mesh bag (10 × 10 cm). The experiment was arranged as a split-split-split plot design with residue types in main plot, timing of treatment application in sub-plot, and treatments in the sub-sub-plot, with four replicates.
The nylon mesh bags containing treated residues were laid out between two rows of wheat plants in a winter wheat field in the third week of May for Trial 1 and in a spring wheat field in the third week of June for Trial 2, when plants were at about 2–3 weeks before anthesis (Zadoks’ scale 15–30), each year in 2009 and 2010. The residues were misted by hand spraying sufficient water on top of the nylon mesh bag once on the day they were placed in the field. Three entire pieces of residues in each bag were sampled 4, 7, 14, 21, 28, 35 and 42 days after being laid out in the field, and the number of perithecia produced on the surface of each residue piece was counted under the dissecting microscope. Each corn, soybean and wheat residue sample was microscopically examined at 15× magnification for the presence or absence of perithecia. The number of perithecia of G. zeae produced over time was summarized as daily perithecial production (DPP).
Autumn application on different types of wheat residues
Two identical field trials to evaluate the effect of the autumn-application of CLO-1 on perithecial production of G. zeae on wheat residues were conducted at ECORC, Ottawa, from 2009 to 2011. Wheat residues used in these trials were peduncles, spikelets and stems with one node in the section. The residues were collected from FHB-infested (natural infection) wheat fields after harvesting in October 2009 for the 2009–2010 field trial and in October 2010 for the 2010–2011 field trial. Each of the three types of wheat residues was cut into 5-cm pieces. Twenty-five pieces of each residue type were subsequently placed into a black nylon mesh bag as described above. Bags were individually sprayed with either CLO-1 at 1 × 107 cfu mL−1, or with Folicur 432F at 0.292% (vol/vol) in a water suspension, or sterile distilled water as the untreated controls. The bags containing the treated residues were allowed to air-dry for 30 min and then laid out in the field 10 cm apart during the third week of October in both 2009 and 2010. The experiments were arranged in a split-split plot design with residue types in the main plot and treatments in the sub-plot with four replicates. Bags were retrieved the following spring at monthly intervals from April to August in 2010 and 2011, respectively. The sample collection and the method of assessing perithecial production were as described above. The number of perithecia produced over time was summarized as DPP.
Statistical analyses
The number of perithecia was subjected to an analysis of variance (ANOVA) without transformation. For all experiments, the residuals for each parameter and trial or year were examined for normality and homogeneity of variances. A combined analysis over experimental repeats or years was conducted using the general linear models procedure in SAS (SAS version 8.1, SAS Institute Inc., Cary, NC). Treatment means were separated using Fisher’s protected Least Significant Difference (LSD) test at a significance level of P ≤ 0.05.
Results
Inhibition of perithecial production on crop residues by spring application
Perithecia were observed on all crop residue types each year and the perithecial production started 7 days after inoculation on the untreated residues, except for wheat residue in 2010 when perithecia were observed after 4 days (). The peak production of perithecia was observed between 21 and 35 days after placement in the fields in both years. On the CLO-1 or Folicur treated residues, perithecial production started 7–10 days later and in a much lower quantity than that of the untreated controls. There was no perithecial production observed on these treated residues 14 days after placement in the field in 2009 and 7 days after the placement in 2010. There was also no clear peak production period observed on residues treated with the CLO-1 biofungicide in both years.
Fig. 1. Effect of spring application of CLO-1 biofungicide on number of perithecia of Gibberella zeae produced on corn, soybean and wheat residues compared with Folicur fungicide and untreated control under field conditions in 2009 and 2010. Data are the mean of two trials and two application timings in each trial. Vertical bars represent standard deviation of the mean.
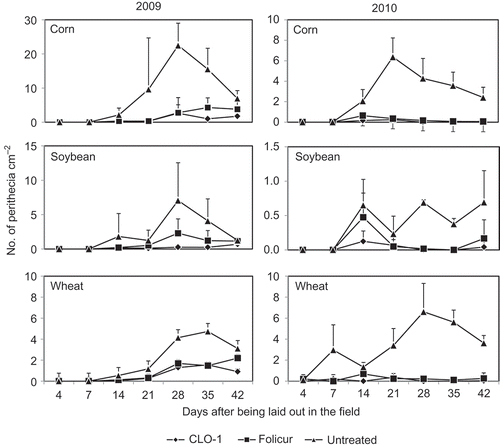
Analysis of variance indicated highly significant differences in DPP among the treatments and among the residue types (P < 0.01) in both 2009 and 2010. The effects of trial and application timing were not significant in either year. The treatment × application timing interaction and the residue type × application timing interaction were significant in 2010 but not in 2009 while the treatment × residue type interaction and treatment × residue type × application timing interaction were not significant in either year. The lack of a significant interaction effect of treatment × residue type indicates that the CLO-1 biofungicide had a similar effect on different crop residues.
CLO-1 inhibited the perithecial production on all crop residue types used (). On average of two trials and two application timings per year, CLO-1 significantly reduced the DPP by 89.6% and 97.4% on the corn residue, 90.3% and 91.2% on soybean residue, and 70.4% and 97.3% on wheat residue, in 2009 and 2010, respectively (). These effects were greater but not significantly different from those by Folicur which reduced DPP by 79.9% and 93.1% on corn residue, by 65.0 and 72.9% on soybean residue, and 57.7 and 92.6% on wheat residue, in 2009 and 2010, respectively.
Fig. 2. Perithecial production of Gibberella zeae on residues of corn (A), soybean (B), and wheat (C) treated with CLO-1 biofungicide (left) and untreated control (right).
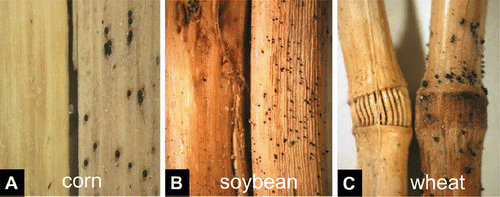
Table 1. Effect of application timing and crop residue type on the efficacy of CLO-1 biofungicide in reducing daily perithecial production (no. perithecia cm−2) compared with Folicur fungicide and untreated control in field trials in 2009 and 2010.
Of the three crop residue types, on a daily basis corn residue produced approximately four-fold more perithecia than soybean residue and two-fold more perithecia than wheat residue averaged over the 2 years (). Overall, CLO-1 reduced DPP by 93.1% on corn residue, 94.4% on soybean residue and 84.0% on wheat residue, in comparison with the untreated controls. These effects were numerically better but not significantly different from those achieved by Folicur fungicide used as positive control in the same experiments.
Fig. 3. Effect of spring application of CLO-1 biofungicide on daily perithecial production of Gibberella zeae on corn, soybean and wheat residues compared with Folicur fungicide and untreated control under field conditions. Data are the mean of 2 years and two trials each year. Means with the same letter for each crop residue type are not significantly different according to Fisher’s Least Significant Difference test at P = 0.05 (LSD).
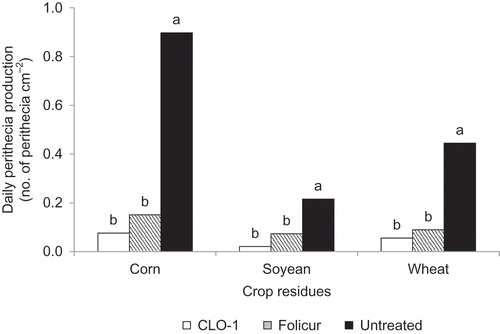
Inhibition of perithecial production on wheat residues by autumn application
Perithecial production was observed on all three types of wheat residues in both 2010 and 2011 (). In 2010, perithecia were observed in a very low amount on 15 April and the peak production period started in June and ended in early August on all three wheat residue types. In 2011, there were no perithecia found on 15 April and very few observed on 11 May on all three types of wheat residue. Perithecial production on the untreated residues showed a slow and continued progression in quantity from May to August, except for spikelet residue where a lower quantity of perithecia was observed in July than in June and August. Overall, there were more perithecia produced on the spikelet residue than on the peduncle and stem residues and in 2010 than in 2011. CLO-1 significantly inhibited perithecial production on all three wheat residue types, reducing DPP by 72.3% on peduncles, 51.0% on spikelets and 57.2% on stems for the average of 2 years (). These effects were better but not significantly different from the Folicur fungicide.
Fig. 4. Effect of autumn application of CLO-1 biofungicide on number of perithecia of Gibberella zeae produced on peduncles, spikelets and stems of wheat residues compared with Folicur fungicide and untreated control under field conditions from April to August of the year following application (2010 and 2011). Vertical bars represent standard deviation of the mean.
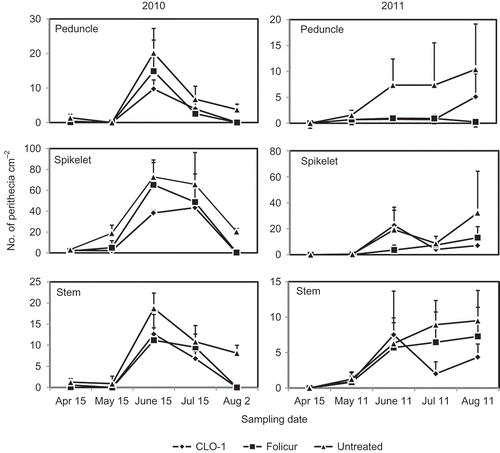
Fig. 5. Effects of autumn application of CLO-1 biofungicide on daily perithecial production of Gibberella zeae on wheat residues compared with Folicur fungicide and untreated control under field conditions in the following spring and summer. Data are mean of 2 years. Means with the same letter for each wheat residue type are not significantly different according to Fisher’s Least Significant Difference test at P = 0.05 (LSD).
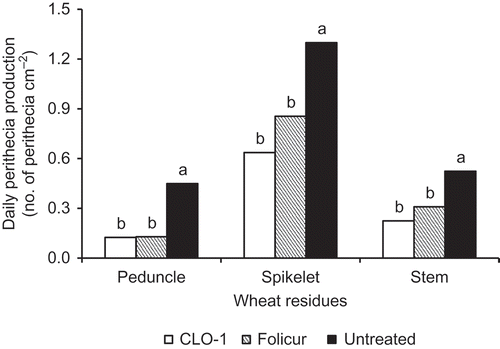
Discussion
There were highly significant differences in the amount of perithecia produced on residues among the test years in both the spring and autumn application trials (, ). In the spring application trials with three crop residue types, the average DPP was 3.2 perithecia cm−2 in 2009, which was about two-fold greater than the DPP of 1.3 perithecia cm−2 in 2010. In the autumn-application trials with three types of wheat residues, the average DPP was 0.7 perithecia cm−2 in 2010, which was about twice that of the DPP of 0.4 perithecia cm−2 in 2011. These results suggest that the number of perithecia produced on a crop residue, either from artificial inoculation or infected naturally, is also affected by environmental conditions. The variation in perithecial production was likely related to the different weather conditions during the different growing seasons. Compared with 2009 and 2010, the summer of 2011 was warmer and dryer, especially in July and early August (). According to records from an Environment Canada weather station located less than 1.0 km from the field sites, the average daily air temperature for 15 May to 4 August, when the spring-application trials were conducted, was 17.3 °C in 2009, which was 2.9 °C lower than in 2010. Likewise, total precipitation for the same period in 2009 was 331.5 mm, which was 102.4 mm higher than in 2010. In the autumn-application trials, the average daily air temperatures for 1 May to 11 August were the same (19.1 °C) for both 2010 and 2011. However, there was more frequent rain in 2010 than in 2011 and the total precipitation for the same period in 2010 was 279.3 mm, which was 89.6 mm higher than in 2011. The link between DPP and weather conditions, mainly precipitation, observed in the present study is in agreement with previous studies (Fernando et al. Citation2000; De Wolf et al. Citation2003; Shaner Citation2003; Dufault et al. Citation2006). These results also support Xue and Chen (Citation2010, Citation2011, Citation2012) who reported FHB epidemics in wheat in 2009 and 2010 but lower disease incidence in 2011 in eastern Ontario where the field trials of the present study were conducted. It is also possible that different G. zeae strains were responsible for the infections observed each year, and that the impact of weather conditions on different strains, as well as differences in fecundity or susceptibility to the fungicides, may have impacted perithecial production.
Fig. 6. Daily air temperatures and precipitation from 1 May to 31 August at the Eastern Cereal and Oilseed Research Centre, Central Experimental Farm, Ottawa, from 2009 through 2011. Data were collected by an Environment Canada weather station located within 1 km from the field sites.
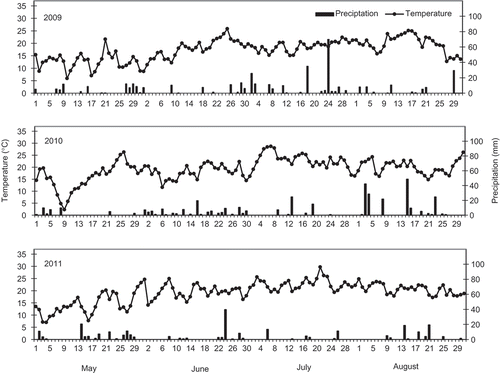
This study demonstrates that the biofungicide CLO-1, a formulated product of C. rosea strain ACM941, is effective in reducing perithecial production of G. zeae on corn, soybean and wheat residues (). The results confirm the previous reports that C. rosea strain ACM941 is an antagonist of G. zeae and inhibits growth and perithecial production of the pathogen (Xue et al. Citation2009a, Citation2009b). Because similar inhibitive effects were observed on corn (93.1%), soybean (94.4%) and wheat (84.0%) residues, as well as the lack of treatment × crop residue type interaction, the results further suggest that crop residue type has little influence on the efficacy of CLO-1 in reducing perithecial production of G. zeae.
The pathogen G. zeae produced more abundant perithecia per surface area on corn residue than on soybean and wheat residues (), suggesting that corn residue is a better substrate for perithecial production. These results were in agreement with Dill-Macky and Jones (Citation2000) and Schaafsma et al. (Citation2005), who demonstrated that the risk of FHB infection would be higher on wheat growing after corn or wheat, and lower on wheat growing after soybean, which was also the least favourable residue for perithecial production by G. zeae in the present study. The significant differences among the crop residues in supporting perithecial production suggest a beneficial effect of crop rotation with soybean and non-host crops of G. zeae in reducing the initial inoculum for FHB.
Timing of application has often been considered as an important factor affecting the efficacy of a biological control event. Bujold et al. (Citation2001), Dawson et al. (Citation2004) and Khan and Doohan (Citation2009) noticed that antagonistic agents were more efficacious when applied ahead of pathogen inoculation with Fusarium spp. causing FHB. In the present research, high levels of efficacy (84.0–94.4%) in the reduction of perithecial production on crop residues were achieved with CLO-1 application either before or after pathogen inoculation based on the average of 2 years’ data and two trials per year. CLO-1 applied 3 days before inoculation with G. zeae showed no significantly greater efficiency than when it was applied the same day on all three crop residue types (). The results suggest that CLO-1 may be applied to residues after harvesting in the autumn or in the following spring when conducive weather conditions are forecast that may lead to a possible FHB epidemic in the summer.
The efficacy of autumn application of CLO-1 in reducing perithecial production on naturally infected wheat residues in the following crop season was examined for 2 consecutive years in the present study. Although the autumn application resulted in relatively low efficiencies (51.0–72.3%) on these naturally infected wheat residues, the effects were still comparable with those achieved by the Folicur fungicide used as positive control in the same experiment (48.3–71.4%) (). The research demonstrated that autumn application of CLO-1 on wheat residues is an effective measure to reduce the initial inoculum of FHB in the following crop season. Results of this study suggest that CLO-1 is a promising biofungicide against G. zeae in either spring or autumn application onto crop residues, as a measure to reduce the initial inoculum in an integrated FHB management programme.
Acknowledgements
This research was co-funded by the Pest Management Centre through the Biopesticide Initiative Program of Agriculture and Agri-Food Canada and by the Ontario Wheat Producers’ Marketing Board. We thank M. Bingley, A. McAlpine, H. Geng, J. Vogel and S. Hargadon for technical assistance.
References
- Amarasinghe CC, Tamburic-Ilincic L, Gilbert J, Brule- Banel AL. 2013. Evaluation of different fungicides for control of fusarium head blight in wheat inoculated with 3ADON and 15ADON chemotypes of Fusarium graminearum in Canada. Can J Plant Pathol. 35:200–208.
- Blandino M, Haidukoski M, Pascale M, Plizzari L, Scudellari D, Reyneri A. 2012. Integrated strategies for the control of Fusarium head blight and deoxynivalenol contamination in winter wheat. Field Crop Res. 133:139–149.
- Blandino M, Minelli L, Reyneri A. 2006. Strategies for the chemical control of Fusarium head blight, effect on yield, alveographic parameters and deoxynivalenol contamination in winter wheat grain. Eur J Agron. 25:193–201.
- Bujold I, Paulitz TC, Carisse O. 2001. Effect of Microsphaeropsis sp. on the production of perithecia and ascospores of Gibberella zeae. Plant Dis. 85:977–984.
- Dawson WAJM, Jestoi M, Rizzo A, Nicholson P, Bateman GL. 2004. Field evaluation of fungal competitors of Fusarium culmorum and F. graminearum, causal agents of ear blight of winter wheat, for the control of mycotoxin production in grain. Biocontrol Sci Technol. 14:783–799.
- De Wolf ED, Madden LV, Lipps PE. 2003. Risk assessment models for wheat Fusarium head blight epidemics based on withinseason weather data. Phytopathology. 93:428–435.
- Desjardins AE. 2006. Fusarium mycotoxins, chemistry, genetics, and biology. St. Paul (MN): APS Press.
- Dill-Macky R, Jones RK. 2000. The effect of previous crop residues and tillage on Fusarium head blight of wheat. Plant Dis. 84:71–76.
- Dufault NS, De Wolf ED, Lipps PE, Madden LV. 2006. Role of temperature and moisture in the production and maturation of Gibberella zeae perithecia. Plant Dis. 90:637–644.
- D’Mello JPF, Placinta CM, MacDonald AMC. 1999. Fusarium mycotoxins, a review of global implications for animal health, welfare and productivity. Anim Feed Sci Technol. 80:183–205.
- Fantke P, Charles R, Alencastro LF, Friedrich R, Jolliet O. 2011. Plant uptake of pesticides and human health, dynamic modeling of residues in wheat and ingestion intake. Chemosphere. 85:1639–1647.
- Fernando WGD, Miller JD, Seaman WL, Seifert K, Paulitz TC. 2000. Daily and seasonal dynamics of airborne spores of Fusarium graminearum and other Fusarium species sampled over wheat plots. Can J Bot. 78:497–505.
- Gilbert J, Haber S. 2013. Overview of some recent research developments in fusarium head blight of wheat. Can J Plant Pathol. 35:149–174.
- Gilbert J, Tekauz A. 2000. Review: recent developments in research on Fusarium head blight of wheat in Canada. Can J Plant Pathol. 22:1–8.
- Herrero-Hernández E, Andrades MS, Marín-Benito JM, Sánchez-Martín MJ, Rodríguez-Cruz MS. 2011. Field-scale dissipation of tebuconazole in a vineyard soil amended with spent mushroom substrate and its potential environmental impact. Ecotoxicol Environ Saf. 74:1480–1488.
- Imfeld G, Vuilleumier S. 2012. Measuring the effects of pes-ticides on bacterial communities in soil, a critical review. Eur J Soil Biol. 49:22–30.
- Jones RK. 2000. Assessment of Fusarium head blight of wheat and barley in response to fungicide treatment. Plant Dis. 84:1021–1030.
- Khan MR, Doohan FM. 2009. Bacterium-mediated control of Fusarium head blight disease of wheat and barley and associated myco- toxin contamination of grain. Biol Control. 48:42–47.
- Matthies A, Buchenauer H. 2000. Effect of tebuconazole (Folicur®) and prochloraz (Sportak®) treatments on Fusarium head scab development, yield and deoxynivalenol (DON) content in grains of wheat following artificial inoculation with Fusarium culmorum. J Plant Dis Prot. 107:33–52.
- McMullen M, Bergstrom G, De Wolf E, Dill-Macky R, Hershman DE, Shaner GE, Van Sanford D. 2012. A unified effort to fight an enemy of wheat and barley: Fusarium head blight. Plant Dis. 96:1712–1728.
- McMullen M, Jones R, Gallenberg D. 1997. Scab of wheat and barley: a re-emerging disease of devastating impact. Plant Dis. 81:1340–1348.
- Menzies J, Gilbert J. 2003. Diseases of wheat. In: Bailey KL, Gossen BD, Gugel RK, Morrall RAA, editors, Diseases of field crops in Canada. 3rd ed. Saskatoon (SK): The Canadian Phytopathological Society, University Extension Press.
- Moser VC, Barone S Jr, Smialowicz RJ, Harris MW, Davis BJ, Overstreet D, Mauney M, Chapin RE. 2001. The effects of perinatal tebuconazole exposure on adult neurological, immunological, and reproductive function in rats. Toxicol Sci. 62:339–352.
- Muñoz-Leoz B, Garbisu C, Antigüedad I, Ruiz-Romera E. 2012. Fertilization can modify the non-target effects of pesticides on soil microbial communities. Soil Biol Biochem. 48:125–134.
- Muñoz-Leoz B, Ruiz-Romera E, Antigüedad I, Garbisu C. 2011. Tebuconazole application decreases soil microbial biomass and activity. Soil Biol Biochem. 43:2176–2183.
- Parry DW, Jenkinson P, McLeod L. 1995. Fusarium ear blight (scab) in small grain cereals – a review. Plant Pathol. 44:207–238.
- Pestka JJ. 2007. Deoxynivalenol: toxicity, mechanisms and animal health risks. Anim Feed Sci Technol. 137:283–298.
- Schaafsma AW, Tamburic-Ilincic L, Hooker DC. 2005. Effect of previous crop, tillage, field size, adjacent crop, and sampling direction on airborne propagules of Gibberella zeae/Fusarium graminearum, Fusarium head blight severity, and deoxynivalenol accumulation in winter wheat. Can J Plant Pathol. 27:217–224.
- Shaner GE. 2003. Epidemiology of Fusarium head blight of small grain cereals in North America. In: Leonard KJ, Bushnell WR, editors. Fusarium head blight of wheat and barley. St. Paul (MN): APS Press; p. 84–119.
- Sutton JC. 1982. Epidemiology of wheat head blight and maize ear rot caused by Fusarium graminearum. Can J Plant Pathol. 4:195–209.
- Trail F. 2009. For blighted waves of grain: Fusarium graminearum in the postgenomics era. Plant Physiol. 149:103–110.
- Wegulo SN, Zwingman MV, Breathnach JA, Baenziger PS. 2011. Economic returns from fungicide application to control foliar fungal diseases in winter wheat. Crop Prot. 30:685–692.
- Xue AG. 2002. Gliocladium roseum strains useful for the control of fungal pathogens in plants. United States patent, US 6,495,133 B1.
- Xue AG. 2003a. Biological control of pathogens causing root rot complex in field pea using Clonostachys rosea strain ACM941. Phytopathology. 93:329–335.
- Xue AG. 2003b. Efficacy of Clonostachys rosea strain ACM941 and fungicide seed treatments for controlling root rot complex of field pea. Can J Plant Sci. 83:519–524.
- Xue AG, Armstrong KC, Voldeng HD, Fedak G, Babcock C. 2004a. Comparative aggressiveness of isolates of Fusarium species causing head blight on wheat in Canada. Can J Plant Pathol. 26:81–88.
- Xue AG, Butler G, Voldeng HD, Fedak G, Savard ME. 2006. Comparison of inoculum sources on development of Fusarium head blight and deoxynivalenol content in wheat in a disease nursery. Can J Plant Pathol. 28:152–159.
- Xue AG, Chen Y. 2010. Diseases of spring wheat in eastern Ontario in 2009. Can Plant Dis Surv. 90:114–115.
- Xue AG, Chen Y. 2011. Diseases of spring wheat in eastern Ontario in 2010. Can Plant Dis Surv. 91:105–106.
- Xue AG, Chen Y. 2012. Diseases of spring wheat in central and eastern Ontario in 2011. Can Plant Dis Surv. 92:109–110.
- Xue AG, Frégeau-Reid J, Rowsell J, Babcock C, Hoekstra GJ, Sparry E. (2004b). Effect of harvesting time on incidence of seed-borne Fusarium spp. in spring wheat in eastern Ontario. Can J Plant Sci. 84, 757–763.
- Xue AG, Ma BL, Vigier B, Clear RM. 2006. Effect of harvesting time on incidence of Fusarium species and accumulation of deoxynivalenol in kernels of silage corn in Ontario. Phytoprotection. 86:189–194.
- Xue AG, Rowsell J, Ho KM, Chen Y, Chi DT, Manceur A, Ren CZ, Zhang SZ. 2013. Effect of harvesting time on grain contamination with Fusarium spp. and deoxynivalenol in barley in northern and eastern Ontario. Phytoprotection. 93:1–7.
- Xue AG, Voldeng HD, Savard ME, Fedak G. 2009a. Biological management of Fusarium head blight and mycotoxin contamination in wheat. World Mycotoxin J. 2:193–201.
- Xue AG, Voldeng HD, Savard ME, Fedak G, Tian X, Hsiang T. 2009b. Biological control of Fusarium head blight of wheat with Clonostachys rosea strain ACM941. Can J Plant Pathol. 31:169–179.
- Yuen GY, Schoneweis SD. 2007. Strategies for managing Fusarium head blight and deoxynivalenol accumulation in wheat. Int J Food Microbiol. 119:126–130.
- Zain ME. 2011. Impact of mycotoxins on humans and animals. J Saudi Chem Soc. 15:129–144.