Abstract
Emerging models indicate that nitrogen availability is an important environmental cue for the induction of virulence in pathogenic fungi. This study explored gene expression patterns of the nitrogen-responsive gene FGSG_03881 and its role in virulence and toxin production in Fusarium graminearum. Using transcriptional fusion pFGSG_03881::GFP, we showed that FGSG_03881 exhibits differential expression profiles in 22 different nitrogen compounds. Since the growth patterns of the fungus also change under these conditions, a mathematical equation was derived to normalize the growth with the expression of FGSG_03881. We determined that expression of FGSG_03881 increased in the non-preferred sources of nitrogen, and decreased in the preferred nitrogen sources like glutamine. Additionally, we determined that this gene is neither linked to the biosynthesis of the mycotoxin DON nor regulated by the global regulator Tri6. Disruption of FGSG_03881 also resulted in increased infection and disease symptoms on wheat. Cumulatively, we suggest that FGSG_03881 is responsive to nitrogen availability and affects virulence in F. graminearum.
Résumé
Les nouveaux modèles indiquent que la disponibilité de l’azote est un important déclencheur environnemental quant à l’induction de la virulence chez les champignons pathogènes. Cette étude a exploré les patrons de l’expression génique du gène FGSG_03881 réceptif à l’azote et son rôle quant à la virulence et à la production de toxine chez Fusarium graminearum. À l’aide de la fusion transcriptionnelle pFGSG_03881::GFP, nous avons montré que le gène FGSG_03881 affiche des profils d’expression différentielle dans 22 composés azotés différents. Étant donné que, dans ces conditions, les modes de croissance du champignon changent également, une équation mathématique a été dérivée afin de normaliser la croissance du champignon et l’expression du gène FGSG_03881. Nous avons établi que l’expression génique de FGSG_03881 s’accroissait dans les sources non préférentielles d’azote et décroissait dans les sources préférentielles comme la glutamine. De plus, nous avons déterminé que ce gène n’est ni lié à la biosynthèse de la mycotoxine DON ni régulé par le régulateur global Tri6. La disruption génique de FGSG_03881 a également provoqué un accroissement de l’infection et des symptômes de la maladie chez le blé. Cumulativement, nous suggérons que le gène FGSG_03881 est réceptif à la disponibilité de l’azote et qu’il influence la virulence chez F. graminearum.
Introduction
Fusarium graminearum (Schwabe) is a devastating pathogen of cereal crops and is a major causal agent of Fusarium head blight (FHB) in wheat (Goswami & Kistler, Citation2004). The fungus produces the trichothecene mycotoxin deoxynivalenol (DON), which accumulates in infected tissues. The toxicity of DON to both humans and livestock results in severe losses to the agricultural sector (McMullen et al., Citation1997; Windels, Citation2000). In addition to its toxicity to animals, DON is also involved in the spread of the pathogen in planta. During a compatible interaction, studies have shown that DON is required to bypass host barriers, specifically at the nodes to the rachis (Jansen et al., Citation2005). Understanding factors that contribute to pathogenicity and the production of DON in the fungus is important from both health and economic perspectives.
Studies indicate that pathogenicity and toxin production by fungi may be closely associated with nutrition and nutrient sensing (Snoeijers et al., Citation2000; Divon & Fluhr, Citation2007). In tomato plants infected with Fusarium oxysporum, genes involved in metabolism and transport of nitrogenous compounds have been reported, indicating a requirement for the pathogen to modulate genes involved in nitrogen uptake during the infection process (Divon et al., Citation2005). Similarly, in wheat and barley plants infected with F. graminearum, microarray analyses revealed that the fungus expresses genes involved in the metabolism and transport of nitrogen-containing compounds during infection (Güldener et al., Citation2006; Lysøe et al., Citation2011). In fact, 86% of the F. graminearum probe sets identified during infection of wheat overlap with probe sets identified from nitrogen stress conditions (Lysøe et al., Citation2011). A similar overlap between the expression of genes involved in metabolism and virulence has been observed in other plant pathogenic fungi and bacteria; this has led to a model where nitrogen-limitation acts as a cue for the activation of secondary metabolism and disease development (Snoeijers et al., Citation2000; Divon & Fluhr, Citation2007).
In F. fujikuroi, the secondary metabolites gibberellin, bikaverin and carotenoids are all produced under nitrogen stress, but are blocked by an abundance of ammonium nitrate, a preferred source of nitrogen (Rodriguez-Ortiz et al., Citation2009). Similarly, in F. graminearum, agmatine and putrescine, which are non-preferred nitrogen sources, induce genes associated with DON production, while preferred nitrogen sources, such as glutamine, asparagine and ammonia, represses these genes (Gardiner et al. Citation2009a). Ammonia also blocks the penetration of cellophane by F. graminearum and F. oxysporum, an in vitro method used to demonstrate host penetration (López-Berges et al. Citation2010). The presence of ammonia also reduced both hyphal fusion and root adhesion in F. oxysporum (López-Berges et al. Citation2010). These studies are adding to our knowledge of the role of nitrogen availability in toxin production and virulence.
In F. graminearum, most of the genes responsible for biosynthesis, transport and regulation for DON production are located in a gene cluster composed of 12 genes; the regulators being Tri6 and Tri10 (Kimura et al. Citation2007). Disruption of either Tri6 or Tri10 results in reduced expression of many genes involved in trichothecene biosynthesis, inhibition of DON production, and renders the strain non-virulent, suggesting that both are positive regulators (Proctor et al. Citation1995; Tag et al. Citation2001; Nasmith et al. Citation2011). One study in F. sporotrichiodes implicated Tri15 as a potential negative regulator of T2 toxin biosynthesis (Alexander et al. Citation2004). This was based on the observation that in culture, exogenous addition of T-2 toxin led to down-regulation of this gene (Alexander et al. Citation2004). In a study referred by Kimura et al. (Citation2007) it was indicated that FGSG_11025, a homologue of Tri15 in F. graminearum, does not have a role in DON biosynthesis, and that FGSG_11025 expression is not regulated by Tri6. This agrees with our unpublished results that showed that deletion of FGSG_11025 did not have any effect on DON production or virulence.
The Munich Information Center for Protein Sequences database also annotated another gene FGSG_03881 in F. graminearum as Tri15 (Wong et al., Citation2010). Microarray analyses show that FGSG_03881 is expressed in both barley and wheat plants infected with F. graminearum (Lysøe et al., Citation2011). Analyses also showed expression was observed in cultures deprived of a nitrogen source, compared with either in a complete or in carbon-deprived media (Lysøe et al., Citation2011). In another study, addition of trichodiene, a precursor substrate for DON biosynthesis, to cultures did not affect the expression of FGSG_03881 (Seong et al., Citation2009). These observations suggested that FGSG_03881 might have properties different from its Tri15 homologue. Therefore, we hypothesized that FGSG_03881 may have a role in toxin production and virulence in F. graminearum.
This study provides evidence to show that expression of FGSG_03881 is linked to type of nitrogen source. The presence of a non-preferred nitrogen source increased FGSG_03881 expression; preferred nitrogen sources decreased its expression. The expression pattern paralleled the expression of Tri6 and Tri10, the positive regulators of virulence and DON production. However, unlike Tri6 and Tri10, deletion of FGSG_03881 increased virulence on wheat heads that was independent of DON production.
Materials and methods
Fungal strains and inoculum preparation
Fusarium graminearum GZ3639 was used as the wildtype strain and all transgenic strains were derived from this parental strain. Conidia were produced in carboxymethylcellulose media and were used as the inoculum in all experiments (Schreiber et al., Citation2011). The mutant strain Δfgsg_03881 was derived from the transformation of protoplasts with the selection marker hygromycin flanked by 1 kb of the 5′ and 3′ ends of the gene using P1–P6 primers, using methods described previously for the construction of Δtri6 (Schreiber et al., Citation2011). The complementation strain (Δfgsg_03881/FGSG_03881) was constructed by amplifying FGSG_03881 and 1 kb of its promoter region by the complementation F/R primers and insertion into the pRF-HU vector, which was modified to contain a geneticin resistance marker in place of hygromycin resistance (Frandsen et al., Citation2008). The resultant construct was transformed into the mutant Δfgsg_03881 using Agrobacterium-mediated transformation (Frandsen et al., Citation2008). The wildtype strain was transformed with the separate vector containing green fluorescent protein under the control of a 1 kb promoter region for FGSG_03881, which was generated using complementation F/R and GFP 1–4 primers. This generated a transcriptional fusion construct pFGSG_03881::GFP, which allowed us to infer the expression of FGSG_03881 under various conditions. All primers used in the construction of the strains are listed in .
Table 1. Primers used in the construction and manipulation of F. graminearum transgenic strains and qRT-PCR reactions
Quantitative real-time PCR analyses
A 4 mL glucose yeast extract peptone (GYEP) (1.5 g NH4Cl, 1 g MgSO4•7H2O, 0.1 g FeSO4•7H2O, 1 g KH2PO4, 1 g peptone, 1 g yeast extract, 1 g malt extract and 10 g glucose in 500 mL H2O) culture was inoculated with either the wildtype, Δfgsg_03881, Δtri6, or pFGSG_03881::GFP strain at a concentration of 5000 spores mL−1 and incubated at 28 °C and 170 rpm for 24 h. After 24 h of growth in the GYEP media, the cultures were transferred to fresh GYEP medium, minimal medium (MM; Leslie & Summerell, Citation2006), MM with 2 g L−1 NH4Cl as a sole nitrogen source, MM lacking nitrogen, or a nitrogen-limiting 15-ADON inducing medium (1 g (NH4)2HPO4, 3 g KH2PO4, 0.2 g MgSO4•7H2O, 5 g NaCl, 40 g sucrose and 10 g glycerol in 1 L H2O). The cultures were grown for an additional 4 h and mycelia were flash frozen in liquid nitrogen prior to RNA isolation. RNA was isolated by Trizol (Life Technologies, Carlsbad, CA) and purified by InviTrap Spin Cell RNA Mini Kit (Invitek, Berlin, Germany). RNA was converted into cDNA by the Applied Biosystems cDNA synthesis kit (Life Technologies). All of the qRT-PCR reactions were performed in triplicate by Applied Biosystems Power SYBR Green kit (Life Technologies) and the Applied Biosystems StepOne Plus Real-Time PCR System according to manufacturer’s instructions (Life Technologies). For relative quantification, a standard curve for each primer set was created. β-tubulin (FGSG_09530) was used as the internal standard between samples. The data were imported and analysed in StepOne 2.1 software. A qRT-PCR analysis was also used to assess copy number of the pFGSG_03881::GFP insert in the pFGSG_03881::GFP transgenic strain. Primer pair qRT-PCR FGSG_03881 promoter F/R was used with genomic DNA isolated from the pFGSG_03881::GFP transgenic strain and compared with the wildtype strain. The primer pair qRT-PCR FGSG_03881 F/R was used to assess the copy number of the native FGSG_03881 and served as a control for the qRT-PCR analysis. Standard curves were constructed using a 10-fold dilution series of genomic DNA ranging from 25 ηg to 0.025 ηg. For relative quantification, one ηg of genomic DNA from each strain was used and Tri6, with a copy number of one, was used as an internal control (Mason et al., Citation2002; Shou et al., Citation2004). All samples were assessed in triplicate using the Applied Biosystems platform mentioned previously. The primers for qRT-PCR are listed in .
High-throughput growth and gene expression analyses of the pFGSG_03881::GFP strain under different nitrogen conditions
Eight hundred spores from the pFGSG_03881::GFP strain were inoculated into wells of a 96-well plate containing 200 μL of MM with different nitrogen sources at 2 g L−1. All of the cultures were grown in triplicate. The plate was incubated at 28 °C to measure both GFP fluorescence (520 nm) and fungal growth (620 nm) in a POLARstar OPTIMA plate reader (BMG LabTech, Cary, NC, USA). A total of 181 data points were collected over a 5-day period and the data were analysed by the FLUOstar OPTIMA 1.32 software (BMG LabTech).
Normalization of GFP fluorescence with growth in various nitrogen conditions
GFP fluorescence was expressed as a function of growth and their relationship was modelled using several different mathematical relationships (i.e. exponential, logarithmic, polynomial); the model with the greatest fit and most consistency across samples was a fourth-order polynomial equation: FGFP (predicted) = α growth4 + β growth3– γ growth2 + δ growth – ε, where α, β, γ and ε are constants. This polynomial equation was used to derive separate formulae for three different nutrient conditions (glutamine, sodium nitrate and ammonium phosphate dibasic), which were used as a standard to predict GFP values given an amount of growth in each of the 22 different nitrogen-containing media. The relative quantification (RQ) of the promoter activity was then calculated as a ratio of the measured GFP fluorescence to the predicted GFP fluorescence (i.e. RQ = FGFP (measured) FGFP (predicted)−1).
Pathology and mycotoxin analyses
The susceptible wheat cultivar ‘Roblin’ was grown in a growth chamber until mid-anthesis as described previously (Schreiber et al., Citation2011). The plants were inoculated between the palea and lemma with 1000 conidia of the wildtype, Δfgsg_03881, or Δfgsg_03381/FGSG_03881 strain. The plants were then transferred to a greenhouse with regular misting for 2 days. Wheat heads with infection beyond the inoculation site were recorded and infection was scored by counting the number of infected spikelets at 7, 10, 14 and 21 days post-inoculation (dpi). Mycotoxin production by the wildtype and Δfgsg_03881 strains in culture were assessed by the two-stage media method and 15-ADON was quantified by HPLC as described previously (Nasmith et al., Citation2011).
Results
FGSG_03881 is related to Tri15
The MIPS (Munich Information Center for Protein Sequences) F. graminearum database describes FGSG_03881 as a gene ‘related to TRI15 – a putative transcription factor’. Tri15 was originally described in a related species, F. sporotrichioides (FS), which produces T2 toxin (similar to DON) (Alexander et al., Citation2004). A pairwise alignment by ClustalW2 indicated that FS_Tri15 shares 83% identity to FGSG_11025, the proposed orthologue of Tri15 and 25% protein identity to FGSG_03881 (Alexander et al. Citation2004). The alignment also revealed that all three proteins share two similar C2H2 zinc finger domains (). These zinc fingers were identified in FGSG_03881 by Pfam (E-value 4.1e-22).
Fig. 1 Alignment of FGSG_03881, FGSG_11025, and FS_Tri15 proteins. Amino acid sequences were aligned by ClustalW2. Identical amino acids between all three proteins are highlighted in black, identities between FGSG_03881 and FS_Tri15 are highlighted in light grey, identities between FGSG_11025 and FS_Tri15 highlighted in dark grey, and the zinc fingers are labelled Zn1 and Zn2, respectively.
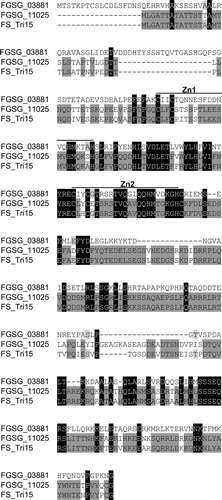
Targeted deletion of FGSG_03881 increases virulence on wheat
To investigate the role of FGSG_03881, we constructed a gene deletion strain and assessed it for disease phenotypes. a and b document the construction and verification of FGSG_03881 deletion (Δfgsg_03881) and complemented (Δfgsg_03381/FGSG_03881) strains. The pathology tests were performed on ‘Roblin’, a susceptible cultivar of wheat, and the disease progression was monitored at 7, 10 and 21 dpi (c). As the results indicate, at 7 dpi, 43% of wheat heads inoculated with Δfgsg_03881 had disease symptoms beyond the inoculation site compared with only 24% with the wildtype strain (P = 0.04). Even at 10 dpi, the disease progression was more prevalent with the Δfgsg_03881 strain (71%) than with the wildtype strain (56%, P = 0.07) (Fig. S2). After 10 dpi, the difference between Δfgsg_03881 and wildtype strains was less pronounced. At 21 dpi, there was no observable difference in infection between the two strains. The FGSG_03881 complemented strain (Δfgsg_03381/FGSG_03881) (b) displayed an infection pattern similar to the wildtype strain at 7, 10, 14 and 21 dpi (c), confirming the virulence phenotype of the mutant. Since the deletion of FGSG_03881 resulted in increased disease symptoms at days 7 and 10, we suggest that FGSG_03881 is involved in the early stages of infection.
Fig. 2 The Δfgsg_03881 strain is more virulent at early stages of infection than the wildtype F. graminearum strain. (a) PCR confirmation of the disruption of FGSG_03881. Genomic DNA was extracted from the Δfgsg_03881 (1) and the wildtype (2) strains and amplified with primers specific to hygromycin marker gene (Hyg) and primers specific to FGSG_03881. (b) PCR confirmation of the complement Δfgsg_03381/FGSG_03881 strain. Genomic DNA was extracted from the wildtype (1) and Δfgsg_03381/FGSG_03881 (2) strains and amplified with primers specific to the Geneticin marker gene (Geneticin) and FGSG_03881. The primers used are listed in . (c) A susceptible cultivar of wheat ‘Roblin’ was point inoculated with the wildtype, Δfgsg_03881, and the complement Δfgsg_03381/FGSG_03881 strains and scored for disease symptoms after 7, 10, 14 and 21 dpi. The number of wheat heads inoculated with each strain is indicated by ‘n’. The number of wheat heads with symptoms beyond the inoculation site is represented as percentage infection (%infection). Error bars reflect the standard error and the ‘P values’ were calculated by one-tailed unpaired t-tests comparing the wildtype and test strains. Significant ‘P values’ are bolded to highlight observed infection differences.
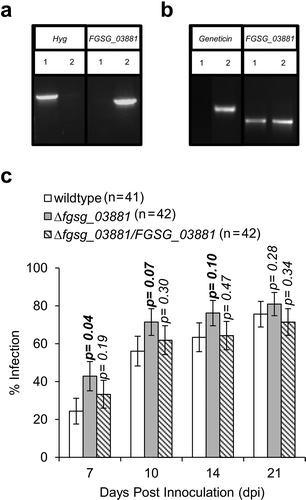
FGSG_03881 is co-expressed with trichothecene genes during nitrogen stress
In F. graminearum, genes associated with virulence, such as Tri6 are regulated by nitrogen availability (Gardiner et al., Citation2009b; Nasmith et al., Citation2011). To determine if FGSG_03881 is similarly regulated by nutrient limiting conditions, a quantitative real-time PCR analysis (qRT-PCR) was used to monitor the expression of FGSG_03881, Tri6 and Tri10 in media with different nitrogen compounds (a). The expression of FGSG_03881 was approximately 20 times greater in the 15-ADON inducing medium that contains ammonium phosphate dibasic as a sole nitrogen source (N-limiting, a) compared with the nutrient-rich GYEP medium (GYEP, a). Under this condition, both Tri6 and Tri10 was expressed 10–15-fold more than FGSG_03881 (N-limiting, a). A similar gene expression pattern was observed in minimal media containing other single nitrogen sources, such as ammonium chloride (MM NH4Cl, a) and sodium nitrate (MM NaNO3, a). The expression of all three genes was greatest in the medium with no added nitrogen (MM -N, a).
Fig. 3 FGSG_03881 expression is dependent on nitrogen limitation and is independent of Tri6. (a) The expression of FGSG_03881, Tri6, and Tri10 is dependent on nitrogen. The wildtype strain was grown in nutrient rich medium (GYEP) for 24 h and transferred to a fresh GYEP medium, a 15-ADON inducing medium (N-limiting), minimal medium (MM) containing ammonium chloride (MM NH4Cl), MM containing sodium nitrate (NaNO3), and MM containing no nitrogen (MM -N) and incubated for an additional 4 h. Relative expression (RQ) of FGSG_03881 (white), Tri10 (grey) and Tri6 (black) was quantified with β-tubulin as standard and normalized to the GYEP conditions. (b) The expression of FGSG_03881 is independent of Tri6. Expression levels of FGSG_03881 (RQ) in the 15-ADON inducing medium (N-limiting) for the wildtype (white), Δtri6 (grey) and Δfgsg_03881 (black) strains are shown. The expression was normalized to the GYEP conditions with β-tubulin as standard. The primers used in the qRT-PCR experiments are listed in . (c) HPLC analyses of the Δfgsg_03881 and wildtype strains in 15-ADON inducing medium compared with a 15-ADON toxin standard; quantification using peak integration and a standard curve determined that the cultures had 120 ppm and 166 ppm of 15-ADON, respectively. Absorbance values were offset to reduce overlap between samples. The result is representative of three independent biological experiments.
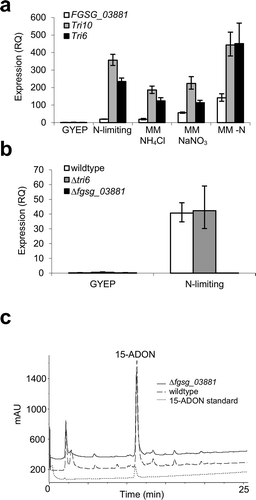
Since FGSG_03881 was co-expressed with other Tri genes, we determined if FGSG_03881 also regulated the production of 15-ADON. The nitrogen-limiting condition used in the qRT-PCR experiments (N-limiting, a) is also suited to produce 15-ADON. As indicated in the HPLC profile, the accumulation of 15-ADON is not significantly affected in the Δfgsg_03881 strain compared with the wildtype strain under these conditions (c). This suggested that FGSG_03881 is not involved in the biosynthetic pathway leading to DON production. Additionally, qRT-PCR analysis indicated that the expression of FGSG_03881 is also not affected by the absence of Tri6 (b), suggesting that unlike FS_Tri15, the expression of FGSG_03881 is not influenced by the presence or absence of 15-ADON (Alexander et al., Citation2004). As expected, we did not observe any expression of FGSG_03881 in the Δfgsg_03881 strain (b). Altogether, these results confirmed that FGSG_03881 is regulated by nitrogen limitation; however, biosynthesis of 15-ADON is not governed by FGSG_03881 ().
Nitrogen availability influences F. graminearum growth
In order to get more insight into the regulation of FGSG_03881 by nitrogen, a GFP transcriptional fusion vector (pFGSG_03881::GFP) was constructed and transformed into wildtype F. graminearum (a). This transgenic strain allowed us to monitor the expression of FGSG_03381 by GFP fluorescence during growth in media containing 22 different nitrogen compounds. Prior to monitoring the growth and expression, the transgenic strain was assessed for the integrity of the native FGSG_03881. As shown, the integrity of the native FGSG_03881 gene was maintained (Fig. S1a). A qRT-PCR analysis of genomic DNA revealed two inserts of the pFGSG_03881::GFP construct in the transgenic strain (Fig. S1b). However, RT-PCR analysis demonstrated that both GFP and FGSG_03881 were co-expressed in the 15-ADON inducing media (Fig. S1c). These results confirmed that neither the integrity of the native FGSG_03881 nor its expression pattern is compromised in the pFGSG_03881::GFP transgenic strain.
Fig. 4 Growth rate of F. graminearum is dependent on nitrogen availability. (a) PCR confirmation of pFGSG_03881::GFP transcriptional fusion strain. Genomic DNA was extracted from the wildtype (1) and pFGSG_03881::GFP (2) strains and amplified with primers specific to the Geneticin marker gene (Geneticin) and green fluorescent protein (GFP). The primers used are listed in . (b) The growth of the pFGSG_03881::GFP transcriptional fusion strain was measured in minimal media appended with different nitrogen sources at 2 g L−1. Growth was measured over a 5-day period at 620 nm in a 96-well plate in a POLARstar Optima plate reader. All data points are the average of three biological replicates and are presented as a percentage of the maximum observed value.
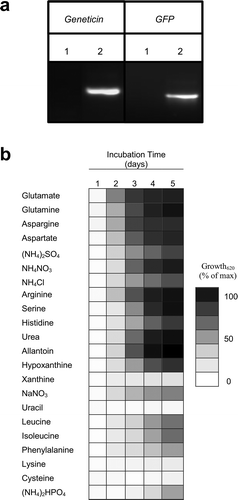
Before monitoring the expression of FGSG_03881, we were interested to know if the pFGSG_03881::GFP strain exhibited differential growth properties in the media containing these nitrogen compounds. Optical measurements at 620 nm revealed that fungal growth was dependent on the nitrogen source (b). As indicated in the growth scale, there was more growth in media supplemented with preferred sources of nitrogen, such as glutamine and most ammonium containing compounds, than in the non-preferred nitrogen sources, such as sodium nitrate (NaNO3) (b). It is important to note that the cultures that contained ammonium phosphate dibasic ((NH4)2 HPO4), the nitrogen source used in the 15-ADON inducing medium, had reduced growth relative to other ammonium-containing media. Reduced growth was also observed in media that contained xanthine, uracil, lysine dichloride and cysteine. Interestingly, the culture that contained allantoin, another non-preferred nitrogen source, resulted in the greatest amount of growth. The media containing arginine and serine also supported significant growth.
Normalization of FGSG_03881 expression with fungal growth in different nitrogen sources
Since we observed growth differences in various nitrogen sources (b), the expression of FGSG_03881 had to be normalized to fungal growth for a given nitrogen source. Here we describe the steps undertaken to normalize FGSG_03881 expression in three culture conditions that displayed differential growth rates: the culture medium with glutamine supported a fast growth rate, while the medium with sodium nitrate and ammonium phosphate dibasic exhibited intermediate and slow growth, respectively. After normalization of GFP to fungal growth, these three culture conditions were used as reference points to infer FGSG_03881 expression in media appended with various nitrogen compounds.
We first measured both GFP fluorescence and growth simultaneously in all three culture conditions (). We observed robust growth in glutamine, a preferred nitrogen source compared with non-preferred nitrogen sources, such as NaNO3 and (NH4)2 HPO4 (before normalization, ). We also observed a concomitant increase in GFP fluorescence during this time. However, the increase in GFP fluorescence was not proportional to the amount of growth across all culture conditions (before normalization, ). Therefore, growth was normalized with respect to GFP fluorescence in all three conditions (after normalization, ), and each curve was represented by a fourth-order polynomial equation (R2 > 0.9) equation. These equations were used as reference points to infer the relative expression of FGSG_03881 given the amount of growth in other nitrogen-containing media.
Fig. 5 Normalization of FGSG_03881 expression. The growth media containing glutamine, sodium nitrate and ammonium phosphate dibasic were selected as reference conditions for relative quantification. Before normalization: Fungal growth (620 nm) and GFP fluorescence (520 nm) were measured simultaneously. Both measurements are represented as transmittance units (×103) in the y-axis. After normalization: GFP fluorescence expressed as a function of growth for all three media conditions. A fourth order polynomial equation was derived from each of the normalized growth curves with coefficient of determination R2 glutamine = 0.99, = 0.99,
= 0.93.
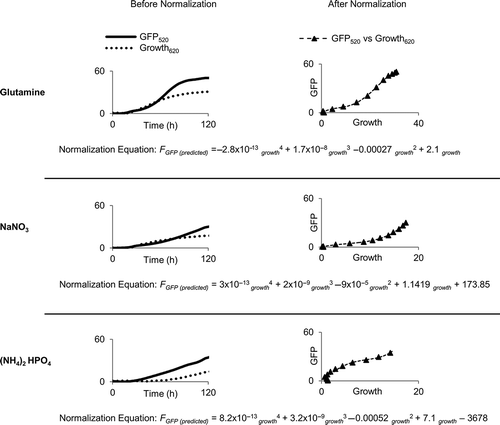
Nitrogen sources affect the expression of FGSG_03881
Each mathematical formula was used independently to predict GFP values for a given growth value for each of the 22 nitrogen sources. A ratio of the predicted and measured GFP values (RQ) was used as an indicator of expression of FGSG_03881 for a given nitrogen source (). As indicated in the heat map, when glutamine was used as a reference point, the expression of FGSG_03881 was greatest in media containing non-preferred sources of nitrogen such as cysteine and (NH4)2HPO4 and intermediate expression was observed in a subset of non-preferred nitrogen sources, including xanthine, uracil, leucine, isoleucine, phenylalanine and lysine (glutamine, ). When we used sodium nitrate as a reference point, we were able to show that media appended with (NH4)2 HPO4 and cysteine demonstrated increased FGSG_03881 expression, while media containing preferred nitrogen sources such as glutamine, glutamate, aspargine, aspartate and most ammonium-containing compounds displayed reduced expression (). Finally, when we used (NH4)2 HPO4 as a reference point, we were able to show that nearly all other nitrogen sources resulted in reduced FGSG_03881 expression ((NH4)2 HPO4, ). Thus, by the use of three reference media, we were able to ascertain with confidence that FGSG_03881 expression is responsive to specific nitrogen sources.
Fig. 6 (Colour online) Expression of FGSG_03881 in 22 different nitrogen conditions. The heat map shows relative expression (RQ) of FGSG_03881 determined using a ratio of the predicted GFP and measured GFP fluorescence values; predicted values were obtained using the formula derived from three different reference conditions (sodium nitrate, glutamine and ammonium phosphate dibasic) that are found in . The RQ is shown by the gradation scale with high expression represented by red and low expression represented by green. This result is a representative of three independent biological replicates.
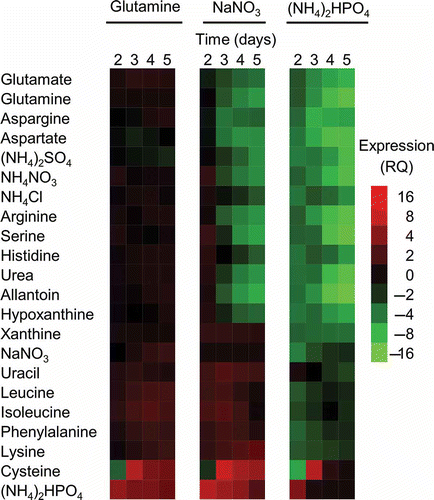
Discussion
In accordance with many studies in fungi, the growth rate of F. graminearum varies depending on the availability and type of nitrogen sources (Gardiner et al., Citation2009a). Our data demonstrated that glutamine and aspargine, preferred sources of nitrogen, supported significant growth compared with non-preferred nitrogen sources, such as sodium nitrate. The data also revealed striking differences in growth patterns within a specific nitrogen category. For example, for the ammonia-based compounds, ammonium phosphate dibasic supported very little growth, while ammonium sulphate or ammonium nitrate supported growth equal to the preferred source, glutamine. Interestingly, allantoin, which is a non-preferred nitrogen source, supported substantial levels of growth. Recently, allantoin was suggested as a potential source of nitrogen used by F. graminearum during the infection process in wheat (Lysøe et al., Citation2011). These results may suggest that distinct adaptation strategies have evolved in F. graminearum isolates to metabolize various nitrogen sources.
Since growth is dependent on nutrient availability, we derived a mathematical formula to normalize the expression of GFP fluorescence and growth of the fungus to allow for relative comparisons between media that contain different nutrient sources. The fourth order polynomial equation derived from three different reference points allowed us to compare gene expression with confidence in both fast- and slow-growing cultures. Overall, the results from 22 different nitrogen compounds indicated that expression of FGSG_03881 was lower in media appended with preferred nitrogen sources, such as glutamine, and greater in non-preferred nitrogen sources, such as cysteine and ammonium phosphate dibasic (NH4)2HPO4 (). There was a strong concordance between the expression of FGSG_03881 (a) and the GFP fluorescence data (), thus alleviating concerns regarding the presence of extra copies of pFGSG_03881::GFP. These observations also complement other studies that demonstrated that the expression of most Tri genes increases in the presence of non-preferred nitrogen sources, such as agmatine, compared with preferred nitrogen sources, such as glutamine (Gardiner et al., Citation2009a, Citation2009b). In addition to non-preferred sources influencing gene expression, we also documented that the expression of Tri6, Tri10 and FGSG_03881 are greatest when nitrogen is not present in the medium; this suggested that these genes respond to general nitrogen stress signals as well as the presence of distinct non-preferred nitrogenous compounds. Although co-expression of Tri6 and Tri10 suggests a common regulatory function shared between FGSG_03881 and the regulatory genes of the trichothecene pathway, there is no apparent role for FGSG_03881 in 15-ADON biosynthesis.
A successful infection requires the pathogen to bypass the host’s defences and gain access to essential nutrients that would allow the fungus to complete its life cycle (Brown et al., Citation2010). An analysis of gene expression patterns in F. graminearum during the infection process in wheat and barley indicated that expression patterns are dynamic (Lysøe et al., Citation2011). The study indicated that genes involved in nutrient acquisition and secondary metabolism are expressed at the early stages of infection (Lysøe et al., Citation2011). Expression of these genes likely involves a complex network of regulatory mechanisms, including perception and coordination of environmental signals by transcription factors (Schönig et al., Citation2008; Gardiner et al., Citation2009a, Citation2009b; López-Berges et al., Citation2010; Lysøe et al., Citation2011; Nasmith et al., Citation2011; Min et al., Citation2012). The present study shows that in F. graminearum, we have identified another factor, namely FGSG_03881, that responds to environmental signals such as nitrogen and influences pathogenicity. Since we did not observe any differences in the production of 15-ADON in culture conditions between the ∆fgsg_03881 and wildtype strains, we suggest that the difference in virulence observed in the mutant is independent of DON biosynthesis (c). Moreover, the fact that the expression of FGSG_03881 is not affected in the Tri6 mutant strain (b) suggests that increased virulence observed with the ∆fgsg_03881 strain is independent of Tri6 function. A chromatin immunoprecipitation followed by next generation sequencing with FGSG_03881 could reveal potential targets and a comparison with Tri6 targets (Nasmith et al., Citation2011) could reveal common genes associated with nitrogen responses and virulence.
In a recent large-scale mutational analysis of transcription factors in F. graminearum, which included FGSG_03881, 17 traits were examined (Son et al., Citation2011). Interestingly, FGSG_03881 was not linked to any of the 17 traits, including virulence. However, the same study also showed no link between Tri6 and virulence. It is conceivable that differences in the wheat cultivar used by Son et al. (Citation2011) could explain the discrepancies. Nevertheless, the study concluded that transcription factors that control the normal growth development of the fungus may also be linked to factors governing virulence, which includes toxin production (Son et al., Citation2011).
In conclusion, identification and characterization of genes responsive to nitrogen signals that both positively and negatively affect virulence emphasizes the complex relationship between environmental signals and pathogenesis. As datasets related to mutagenesis, gene expression and protein interaction accumulate, advanced networking tools will enable us to disentangle the mechanisms by which various environmental signals, such as nitrogen availability, are integrated into pathways involved in virulence in F. graminearum through genes such as Tri6 and FGSG_03881.
Supplemental Figure 1
Download TIFF Image (458.5 KB)Supplemental Figure 2
Download TIFF Image (2.2 MB)Acknowledgements
We would like to thank Winnie Leung and Christopher Mogg for technical assistance.
References
- Alexander NJ, McCormick SP, Larson TM, Jurgenson JE. 2004. Expression of Tri15 in Fusarium sporotrichioides. Curr Genet. 45: 157–162. doi:10.1007/s00294-003-0467-3
- Brown NA, Urban M, Van de Meene AML, Hammond-Kosack KE. 2010. The infection biology of Fusarium graminearum: defining the pathways of spikelet to spikelet colonisation in wheat ears. Fungal Biol. 114: 555–571. doi:10.1016/j.funbio.2010.04.006
- Divon HH, Fluhr R. 2007. Nutrition acquisition strategies during fungal infection of plants. FEMS Microbiol Lett. 266: 65–74. doi:10.1111/j.1574-6968.2006.00504.x
- Divon HH, Rothan-Denoyes B, Davydov O, Di Pietro A, Fluhr R. 2005. Nitrogen-responsive genes are differentially regulated in planta during Fusarium oxyspsorum f. sp. lycopersici infection. Mol Plant Pathol. 6: 459–470. doi:10.1111/j.1364-3703.2005.00297.x
- Frandsen RJ, Andersson JA, Kristensen MB, Giese H. 2008. Efficient four fragment cloning for the construction of vectors for targeted gene replacement in filamentous fungi. BMC Mol Biol. 9: 70. doi:10.1186/1471-2199-9-70
- Gardiner DM, Kazan K, Manners JM. 2009a. Nutrient profiling reveals potent inducers of trichothecene biosynthesis in Fusarium graminearum. Fungal Genet Biol. 46: 604–613. doi:10.1016/j.fgb.2009.04.004
- Gardiner DM, Kazan K, Manners JM. 2009b. Novel genes of Fusarium graminearum that negatively regulate deoxynivalenol production and virulence. Mol Plant Microbe Interact. 22: 1588–1600. doi:10.1094/MPMI-22-12-1588
- Goswami RS, Kistler HC. 2004. Heading for disaster: Fusarium graminearum on cereal crops. Mol Plant Pathol. 5: 515–525. doi:10.1111/j.1364-3703.2004.00252.x
- Güldener U, Seong K-Y, Boddu J, Cho S, Trail F, Xu J-R, Adam G, Mewes H-W, Muehlbauer GJ, Kistler HC. 2006. Development of a Fusarium graminearum Affymetrix GeneChip for profiling fungal gene expression in vitro and in planta. Fungal Genet Biol. 43: 316–325. doi:10.1016/j.fgb.2006.01.005
- Jansen C, Von Wettstein D, Schafer W, Kogel K-H, Felk A, Maier FJ. 2005. Infection patterns in barley and wheat spikes inoculated with wild-type and trichodiene synthase gene disrupted Fusarium graminearum. Proc Natl Acad Sci USA. 102: 16892–16897. doi:10.1073/pnas.0508467102
- Kimura M, Tokai T, Takahashi-Ando N, Ohsato S, Fujimura M. 2007. Molecular and genetic studies of Fusarium trichothecene biosynthesis: pathways, genes, and evolution. Biosci Biotechnol Biochem. 71: 2105–2123. doi:10.1271/bbb.70183
- Leslie JF, Summerell BA. 2006. The Fusarium Laboratory Manual. Iowa: Blackwell Publishers; 11 pp.
- López-Berges MS, Rispail N, Prados-Rosales RC, Di Pietro A. 2010. A nitrogen response pathway regulates virulence functions in Fusarium oxysporum via the protein kinase TOR and the bZIP protein MeaB. Plant Cell. 22: 2459–2475. doi:10.1105/tpc.110.075937
- Lysøe E, Seong K-Y, Kistler HC. 2011. The transcriptome of Fusarium graminearum during the infection of wheat. Mol Plant Microbe Interact. 24: 995–1000. doi:10.1094/MPMI-02-11-0038
- Mason G, Provero P, Vaira AM, Accotto GP. 2002. Estimating the number of integrations in transformed plants by quantitative real-time PCR. BMC Biotechnol. 2: 20. doi:10.1186/1472-6750-2-20
- McMullen M, Jones R, Gallenberg D. 1997. Scab of wheat and barley: a re-emerging disease of devastating impact. Plant Dis. 81: 1340–1348. doi:10.1094/PDIS.1997.81.12.1340
- Min K, Shin Y, Son H, Lee J, Kim J-C, Choi GJ, Lee Y-W. 2012. Functional analyses of the nitrogen regulatory gene areA in Gibberella zeae. FEMS Microbiol Lett. 334: 66–73. doi:10.1111/j.1574-6968.2012.02620.x
- Nasmith CG, Walkowiak S, Wang L, Leung WWY, Gong Y, Johnston A, Harris LJ, Guttman DS, Subramaniam R. 2011. Tri6 is a global transcription regulator in the phytopathogen Fusarium graminearum. PLoS Pathog. 7: e1002266. doi:10.1371/journal.ppat.1002266
- Proctor RH, Hohn TM, McCormick SP. 1995. Reduced virulence of Gibberella zeae caused by disruption of a trichothecene toxin biosynthetic gene. Mol Plant Microbe Interact. 8: 593–601. doi:10.1094/MPMI-8-0593
- Rodriguez-Ortiz R, Limon MC, Avalos J. 2009. Regulation of carotenogenesis and secondary metabolism by nitrogen in wild-type Fusarium fujikuroi and carotenoid-overproducing mutants. Appl Environ Microbiol. 75: 405–413. doi:10.1128/AEM.01089-08
- Schönig B, Brown DW, Oeser B, Tudzynski B. 2008. Cross-species hybridization with Fusarium verticillioides microarrays reveals new insights into Fusarium fujikuroi nitrogen regulation and the role of AreA and NMR. Eukaryot Cell. 7: 1831–1846. doi:10.1128/EC.00130-08
- Schreiber KJ, Nasmith CG, Allard G, Singh J, Subramaniam R, Desveaux D. 2011. Found in translation: high-throughput chemical screening in Arabidopsis thaliana identifies small molecules that reduce Fusarium head blight disease in wheat. Mol Plant-Microbe Interact. 24: 640–648. doi:10.1094/MPMI-09-10-0210
- Seong K-Y, Pasquali M, Zhou X, Song J, Hilburn K, McCormick S, Dong Y, Xu J-R, Kistler HC. 2009. Global gene regulation by Fusarium transcription factors Tri6 and Tri10 reveals adaptations for toxin biosynthesis. Mol Microbiol. 72: 354–367. doi:10.1111/j.1365-2958.2009.06649.x
- Shou H, Frame BR, Whitham SA, Wang K. 2004. Assessment of transgenic maize events produced by particle bombardment or Agrobacterium-mediated transformation. Mol Breed. 13: 201–208. doi:10.1023/B:MOLB.0000018767.64586.53
- Snoeijers SS, Pérez-García A, Joosten MHA, De Wit PJG. 2000. The effect of nitrogen on disease development and gene expression in bacterial and fungal plant pathogens. Eur J Plant Pathol. 106: 493–506. doi:10.1023/A:1008720704105
- Son H, Seo Y-S, Min K, Park AR, Lee J, Jin J-M, Lin Y, Cao P, Hong S-Y, Kim E-K, et al. 2011. A phenome-based functional analysis of transcription factors in the cereal head blight fungus, Fusarium graminearum. PLoS Pathog. 7: e1002310.
- Tag AG, Garifullina GF, Peplow AW, Ake C, Phillips TD, Hohn TM, Beremand MN. 2001. A novel regulatory gene, Tri10, controls trichothecene toxin production and gene expression. Appl Environ Microbiol. 67: 5294–5302. doi:10.1128/AEM.67.11.5294-5302.2001
- Windels CE. 2000. Economic and social impacts of Fusarium head blight: changing farms and rural communities in the Northern Great Plains. Phytopathology. 90: 17–21. doi:10.1094/PHYTO.2000.90.1.17
- Wong P, Walter M, Lee W, Mannhaupt G, Munsterkotter M, Mewes H-W, Adam G, Guldener U. 2010. FGDB: revisiting the genome annotation of the plant pathogen Fusarium graminearum. Nucleic Acids Res. 39: D637–D639. doi:10.1093/nar/gkq1016