Abstract
Loose smut [Ustilago tritici (Pers) Rostr.] of wheat (Triticum aestivum L) causes losses in grain yield roughly proportional to the incidence of the disease. Efforts to control this disease utilizing genetic resistance are being made in breeding programmes around the world. DNA markers for genetic resistance would greatly enhance breeding efforts by reducing the substantial time and labour resources necessary to screen for resistant lines using traditional inoculation methods. The objective of this study was to identify DNA markers associated with resistance. Doubled haploid progeny from the four crosses ‘Glenlea’/‘AC Taber’, ‘SC8021V2’/‘AC Karma’, ‘Diamant’/TD12A, and 9340-CP*/‘AC Vista’ were evaluated with individual races of U. tritici and simple sequence repeat markers. Simple interval mapping was performed using MQTL software and single point analysis. ‘Glenlea’, which has complete and broad resistance to all known races of U. tritici in Canada, contributed a QTL for resistance to races T2, T10, T15, T19 and T39 at Xwmc160 on chromosome 5B. ‘Glenlea’ also contributed QTL for resistance to races T2, T9 and T15 at Xgwm302 on chromosome 7B and to races T9, T19 and T39 on chromosome 3A at marker Xwmc559. The chromosome 5B QTL from ‘Glenlea’ coincided with a race T39 resistance locus from ‘AC Karma’. The 7B QTL from ‘Glenlea’ coincided with resistance to race T9 from 9340-CP* and T2, T9 and T27 resistance from TD12A. The QTL on 3A from ‘Glenlea’ was coincidental with T9 resistance from 9340-CP*. When evaluated with race T10, a QTL was identified near Xbarc183 on chromosome 6D from ‘SC8021V2’. A QTL to race T9 from ‘SC8021V2’ within the interval Xcfa2019 to Xgwm344a was identified on 7A. A third QTL from ‘SC8021V2’ was located on 6B at Xbarc223 and Xbarc332b. The chromosome 5B locus together with either the 7A or 7B genetic loci would provide resistance to predominant races of loose smut found on the Canadian prairies.
Résumé
Le charbon nu (Ustilago tritici [Pers.] Rostr.) du blé (Triticum aestivum L.) engendre des pertes de rendement relativement proportionnelles à l’incidence de la maladie. Des efforts pour maîtriser cette dernière par résistance génétique sont déployés dans le cadre des programmes de sélection partout dans le monde. Des marqueurs d’ADN propres à la résistance génétique amélioreraient grandement les efforts consentis à la sélection en réduisant substantiellement le temps et les ressources affectées actuellement au criblage de lignées résistantes en utilisant les méthodes traditionnelles d’inoculation. Le but de cette étude était d’identifier les marqueurs d’ADN associés à la résistance. La progéniture dihaploïde issue de quatre croisements (‘Glenlea’/‘AC Taber’, ‘SC8021V2’/‘AC Karma’, ‘Diamant’/‘TD12A’ et ‘9340-CP*’/‘AC Vista’) a été évaluée avec des races individuelles d’U. tritici et des marqueurs des répétitions de séquences simples. La cartographie par intervalle simple a été dressée à l’aide du logiciel MQTL et par analyse ponctuelle. Le cultivar ‘Glenlea’, qui possède une résistance étendue à toutes les races d’U. tritici au Canada, a fourni un locus à caractère quantitatif (QTL) pour la résistance aux races T2, T10, T15, T19 et T39, au marqueur Xwmc160 sur le chromosome 5B. ‘Glenlea’ a également fourni un QTL pour la résistance aux races T2, T9 et T15, au marqueur Xgwm302 sur le chromosome 7B, ainsi qu’aux races T9, T19 et T39 sur le chromosome 3A, au marqueur Xwmc559. Le QTL du chromosome 5B de ‘Glenlea’ a coïncidé avec un locus de résistance à la race T39 du cultivar ‘AC Karma’. Le QTL du chromosome 7B de ‘Glenlea’ a coïncidé avec la résistance à la race T9 de ‘9340-CP*’ et aux races T2, T9 et T27 de ‘TD12A’. Le QTL du chromosome 3A de ‘Glenlea’ a coïncidé avec la résistance à la race T9 de ‘9340-CP*’. Lorsqu’il a été évalué en fonction de la race T10, un QTL a été identifié près du marqueur Xbarc183 sur le chromosome 6D de ‘SC8021V2’. Un QTL de la race T9 de ‘SC8021V2’, dans l’intervalle Xcfa2019 à Xgwm344a, a été identifié sur le chromosome 7A. Un troisième QTL de ‘SC8021V2’ a été localisé sur le chromosome 6B, aux marqueurs Xbarc223 et Xbarc332b. Le locus du chromosome 5B, de concert avec soit les locus génétiques du chromosome 7A ou du chromosome 7B, fournirait la résistance au charbon nu chez les races qui prédominent sur les Prairies canadiennes.
Introduction
Loose smut [Ustilago tritici (Pers.) Rostr.] is a disease of wheat (Triticum aestivum L.) that is typically controlled by genetic resistance or seed treatment with a systemic fungicide. The cost of chemical control is roughly equal to 1% of the crop value in western Canada; however, losses to this disease can be substantially greater. In the absence of control measures, loose smut causes losses in grain yield roughly proportional to the incidence of the disease (Knox & Menzies Citation2012). Therefore, the deployment of genetic resistance to loose smut through breeding of commercial cultivars is desirable.
The loose smut pathogen infects the developing caryopsis of the host after entry of a spore into the floret around the time of anthesis (Nielsen & Thomas Citation1996). The fungus continues to develop when the infected seed is planted and is sustained in the growing point of susceptible cultivars. As the spike develops, the fungus develops spores that replace most of the spike tissue. To breed for resistance to loose smut, individual plants must be inoculated and a second generation grown out to assess the genotype response. This effort consumes considerable resources.
Races of the pathogen exist; therefore, surveillance of the constantly changing pathogen population is necessary, involving evaluation and classification of races and assessment of their virulence on deployed sources of resistance. For example, in 1992–1993, race T9 of U. tritici was identified as new to Canada (Menzies et al. Citation2003). Canada Western Extra Strong wheat cultivars and many Canada Western Red Spring wheat cultivars were resistant to race T9, but Canada Prairie Spring cultivars considered to be resistant to loose smut prior to the presence of T9, were susceptible to T9. In assessing effective resistance, it is critical to understand the race structure of the pathogen for the region of deployment of resistant host lines so that breeding can stay a step ahead of the pathogen.
Many sources of resistance to U. tritici have been identified (Nielsen Citation1983; Knox & Menzies Citation2012). Understanding the genetics of resistance in the context of pathogen virulence allows effective resistance gene combinations to be deployed. Furthermore, geneticists can focus on developing DNA markers to genes that will be effective in resisting the disease.
The Canada Western Extra Strong cultivar ‘Glenlea’ is completely resistant (immune) to all races of U. tritici collected from Canada and around the world (Nielsen Citation1983), including race T9. ‘Glenlea’, a descendent of ‘Thatcher’, is an ancestor of cultivars in a number of Canadian wheat classes. Knox et al. (Citation2008a) studied the genetics of resistance in ‘Glenlea’, and found ‘Glenlea’ carried up to five genes for resistance. They used multiple races in the genetic analysis and noted segregation of a resistance differential between progeny lines and races. They concluded that either of two two-gene combinations provided by ‘Glenlea’ were required to provide broad resistance to virulence found in loose smut races of the Canadian prairies. One combination in ‘Glenlea’ was two additive partial resistance genes, while the other combination was two major genes with narrow but complementary resistance to Canadian races of U. tritici.
Markers are developed for resistance to many different types of diseases for purposes such as cataloguing resistance genes, for marker-assisted selection, and as first steps in sequencing a gene. Marker-assisted selection for loose smut resistance would be more efficient than the traditional time-consuming and often highly variable disease inoculation and evaluation methods. By using multiple individual races in the study of inheritance, researchers can identify which genes are effective to which races and therefore which genes should be the focus of marker development for gene deployment (Knox et al. Citation2008a).
There are many reports of genetic analysis of resistance to U. tritici in wheat (Knox & Menzies Citation2012). Resistance to disease may be inherited as a qualitative trait or as a quantitative trait, and both types of inheritance have been reported for loose smut. Major genes for loose smut resistance were assigned by various researchers to chromosomes 2B, 3D, 4D, 5B, 6A, 7A and 7B, and other chromosomes with partial resistance effects were also implicated (Knox & Menzies Citation2012). Major genes Ut1to 4 for resistance to U. tritici have been inferred (McIntosh et al. Citation2013) based on the gene-for-gene relationship and inheritance of virulence studies in the pathogen (Nielsen Citation1977, Citation1982). Markers have been developed for resistance to loose smut. In T. aestivum, Procunier et al. (Citation1997) reported random amplified polymorphic DNA and restriction fragment length polymorphism markers to a gene in the line ‘Biggar BSR’ for resistance to race T10. The gene originally named Ut-x will be known as Ut5 here on. In T. turgidum, Knox et al. (Citation2002) reported a marker for resistance from the cultivar ‘DT676’ (Knox et al. Citation2008b) to race T33. Randhawa et al. (Citation2009) identified both amplified fragment length polymorphism markers and simple sequence repeat markers to loose smut resistance in T. turgidum.
Qualitative genetic analysis is usually applied when resistance is controlled by one or more major genes with a high level of penetrance and expressivity, or by one or two minor genes with moderate penetrance. When multiple major genes with the same disease phenotype, for example a complete resistance phenotype, are present in a single genetic background, it is not possible to classify progeny segregating for such resistance into classes possessing the different genes that is necessary for qualitative assessment of markers. However, a quantitative approach to mapping is possible. No reports of quantitative trait locus (QTL) analysis could be found for resistance to U. tritici, but QTL analysis has been commonly performed in wheat for resistance to various other diseases, with common bunt (Knox et al. Citation2013), rust (Singh et al. Citation2013) and fusarium head blight (Xue et al. Citation2011) as examples. To map resistance from the cultivar ‘Glenlea’, which possesses multiple major genes for resistance to U. tritici and possibly minor genes with cumulative effects (Knox et al. Citation2008a), a quantitative genetic analysis is appropriate. The objective of this study was to identify, map and validate DNA markers associated with resistance to U. tritici primarily from the wheat cultivar ‘Glenlea’.
Materials and methods
Genetic populations
The four crosses ‘Glenlea’ by ‘AC Taber’ (abbreviated Glenlea/Taber), ‘SC8021V2’ by ‘AC Karma’ (abbreviated 8021/Karma), ‘Diamant’ by TD12A (abbreviated Diamant/TD12A), and 9340-CP* by ‘AC Vista’ (abbreviated 9340/Vista) were utilized to investigate the genetics of resistance to U. tritici. All populations were doubled haploid, developed using the maize pollen and dicamba protocol described by Knox et al. (Citation2000). The relationships of the parents of the crosses are shown in ‘Glenlea’ was first registered in Canada as a utility wheat (Evans et al. Citation1972) and later utilized in the Canada Western Extra Strong market class (Hussain & Lukow Citation1997). ‘Glenlea’ is completely resistant (immune) to a broad spectrum of races collected from Canada and around the world (Nielsen Citation1983). ‘AC Taber’ Canada Prairie Spring wheat is broadly and highly susceptible to loose smut races (Knox et al. Citation1992; DePauw et al. Citation1994). ‘SC8021V2’ is a germplasm line with a white seed coat (Kenya 321.BT.I.B.1/‘Peck’) developed for improved preharvest sprouting resistance but is also resistant to loose smut (DePauw et al. Citation1992). ‘AC Karma’ Canada Prairie Spring wheat has ‘Glenlea’ in its pedigree as a source of loose smut resistance to races T2, T10 and T39 (Knox et al. Citation1995), but it is susceptible to race T9. ‘Diamant’ is a loose smut differential line (D-6) from the former Soviet Union and susceptible to most races of loose smut except one race from eastern Siberia (Nielsen & Tikhomirov Citation1993). The line TD12A (‘Thatcher’/‘Regent’//‘Reward’) is a component of the Canadian loose smut differential set (Nielsen Citation1987) and is resistant to prairie races T2 and T9 but susceptible to T10 and T39 (Menzies et al. Citation2003). ‘Thatcher’ is an ancestor of ‘Glenlea’ with the coefficient of parentage between ‘Thatcher’ and ‘Glenlea’ of 0.31 () using the International Crop Information System [IRRI-CIMMYT Crop Research Informatics Laboratory (CRIL) Citation2014] which uses the Sneller (Citation1994) algorithm. The line 9340-CP* is derived from the cross ‘Glenlea’*2/ND674. ‘AC Vista’, like ‘AC Karma’, has ‘Glenlea’ in its pedigree as a source of loose smut resistance to races T2, T10 and T39, but is susceptible to race T9 (DePauw et al. Citation1998).
Fig. 1 Pedigree of parental lines used in crosses from which wheat populations were developed and tested for segregation of factors for resistance to Ustilago tritici. Parental lines are identified by the eight-point star symbol.
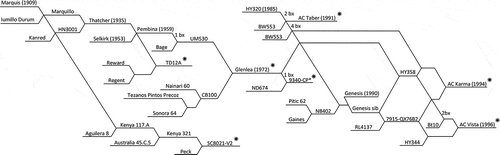
Table 1. The coefficient of parentage between pairs of wheat cultivars using the Sneller algorithm and generated by the International Crop Information System.
The Glenlea/Taber population was previously developed and used for classical genetic analysis of loose smut resistance (Knox et al. Citation2008a) and the 8021/Karma population was developed and previously used to study preharvest sprouting resistance (Clarke et al. Citation2005). The 9340/Vista population was developed from the F1 seed of four lines of 9340-CP (02, 03, 04 and 09) pollinated with ‘AC Vista’. The Diamant/TD12A population was developed from the F1 seed of reciprocal crosses ‘Diamant’ by TD12A and TD12A by ‘Diamant’. Crossing involved planting male and female parents in separate labelled containers within a growth chamber. Female plants were emasculated by clipping back florets to facilitate removal of green anthers and subsequent pollination. The emasculated spikes were covered with a glassine bag (Wells & Caffey Citation1956; Wells Citation1962). Pollinations were made within 4 days of emasculation by clipping the top off the glassine bag, inserting the spike of the male parent and spinning pollen from the spike possessing mature anthers into the bag surrounding the female spike. The bag was folded closed after pollination. Female spikes were labelled with a tag indicating details of the cross, and grown to maturity. Spikes of crossed seed were harvested at maturity into labelled envelopes with one spike per envelope and hand threshed.
Phenotypic evaluation
One or more spikes per line were syringe-inoculated with teliospores of individual U. tritici races in a controlled environment as described by Knox et al. (Citation1999). The 62 lines of the Glenlea/Taber population along with the parents ‘Glenlea’ and ‘AC Taber’, and susceptible control ‘Biggar’, were inoculated with races T2, T9, T10, T15, T19 and T39 at Swift Current. A set of 103 lines of the 8021/Karma population were syringe-inoculated with teliospores of race T9, 93 lines were inoculated with T39, and 45 lines with T10 at Swift Current. During inoculations, spikes were limited in number by the degree of tillering; therefore the highest priority was inoculation with T9 and T39 to produce an adequate number of seed, while T10 was given a lower priority on the 8021/Karma population. Parents ‘SC8021V2’ and ‘AC Karma’ of the 8021/Karma population, and susceptible control ‘Biggar’, were also inoculated with each of the races used on the population. A set of 199 lines from the 9340/Vista population was inoculated with race T9 at Swift Current. Initially 100 random lines were planted for inoculation. The plants were inoculated twice, but in both instances one line did not produce heads, giving 99 inoculated lines. To improve precision, a second set of 100 random lines was subsequently inoculated twice. In addition to the parents, 9340-CP and ‘AC Vista’, in the first set of 99, and HY446 and ‘Biggar’ were controls; in the second set of 100 lines, ‘Biggar’, HY446, ‘Glenlea’, and ND674 were inoculated controls. A total of 150 lines (75 from each cross direction) of the Diamant/TD12A population were inoculated at Winnipeg and Swift Current to races T2, T9 and T27, but one line inoculated with race T2 produced too few scorable plants to be included in the study. The parents ‘Diamant’ and TD12A were inoculated in Winnipeg, while the parents and ‘Biggar’ were inoculated in Swift Current. The results are presented for all races inoculated on all populations where a minimum number of inoculated seed were obtained. In rare cases, there are missing data for a particular race-line combination when plant(s) did not grow, or there was insufficient inoculated seed to include the line. This appears as different population sizes among races for a given genetic population.
Inoculated spikes were labelled with coloured tags, with a particular colour assigned to a particular race. Multiple rounds of inoculations were performed as described by Knox et al. (Citation2008a) to attain a minimum sample size of 15 plants per line to rate, but typically many more than 15 plants per line were rated. At seed maturity, inoculated spikes were harvested into labelled envelopes and subsequently threshed with a mechanical thresher as individual spikes.
Inoculated seeds were planted with one spike per row in greenhouse beds. Each row was labelled to track line identity and the race with which the seed was inoculated. Disease incidence was assessed by counting the number of plants and rating each for the presence of loose smut sori such that any plant with any level of loose smut was considered susceptible. Plants were rated within each race-wheat line combination. The per cent incidence of disease in each line for each race was calculated as a ratio of the total plants smutted over the total plants rated multiplied by 100.
Conditions for plant growth
Conditions for growth of plants used for crossing and inoculation were similar to those described by Knox et al. (Citation1999). The plants were grown in a growth chamber with a 16 h light and 8 h dark lighting regime initially at a constant 15 °C and near anthesis at 18 °C during the light period and 15 °C during the dark. Lighting was a combination of fluorescent and incandescent lamps at approximately 250 µmol m−2 s−1 light intensity. Air was constantly circulated within the chamber to improve the uniformity of temperature. The seeds were planted about 1 to 1.5 cm deep in 1 L wax-cardboard containers (dimensions 10 × 10 × 25 cm) with open tops and two 1 cm holes punched in the bottom. Peat moss was placed in the bottom of the containers to cover the holes. The containers were filled with pasteurized field soil (Swinton loam) which was tamped, and the space created by tamping was filled again, but with a 2:1 mix of soil and potting mix (Sungro Sunshine LB2 mix Basic). The potting mix was then pressed down to leave approximately a 1.5 cm space at the top of the container for watering.
Conditions for growth of plants used for evaluation of loose smut symptoms were similar to those described by Knox et al. (Citation1999) with a 16 h light, 8 h dark photoperiod and temperatures ranging from 15 to 25 °C. Daylight was supplemented with sodium vapour lamps. Light canopies were maintained at least 30 cm from the top of the plants. Inoculated seeds were planted in soil beds containing pasteurized Swinton loam soil with a 2.5 cm topping mixture of 2 parts soil to 1 part potting mix. Plants were provided with 200 mg L−1 of 20–20–20 (N–P–K) at the time of watering.
Genetic evaluation
Genomic DNA was isolated from plants at the one to two leaf stage, with a 10 cm leaf segment harvested from the primary leaf of each plant. Isolation of DNA for PCR was conducted using a modified cetyl-trimethylammonium bromide (CTAB) method (Doyle & Doyle Citation1987). The concentration of DNA was quantified spectrophotometrically using a Gene Quant instrument to allow equalization of concentrations for PCR.
A total of 738 microsatellite primer pairs (gwm, wmc, barc, cfa, cfd, gdm) as referenced by Knox et al. (Citation2012) were tested for polymorphism between ‘Glenlea’ and ‘AC Taber’ with 191 being polymorphic. After testing the 191 markers on a subset of the population, all 62 lines of the Glenlea/Taber population were tested with 128 microsatellite primers found to be polymorphic on the subset. The primer sets were also tested on the four 9340-CP lines and ‘AC Vista’. From 142 markers polymorphic between 9340-CP and ‘AC Vista’, 72 were tested on lines of the population. The lines tested were bulks with the 10 most resistant and 10 most susceptible incidence scores. Putatively associated markers were tested on nine resistant and 21 susceptible lines from the 9340/Vista population to determine which should be tested on the whole population. Ultimately 198 lines of the 9340/Vista population were genotyped with 14 of the microsatellite primers. The 8021/Karma population generated 621 polymorphic loci on 92 lines. Forty-four microsatellite primers were used to evaluate the ‘Diamant’ and TD12A with 30 polymorphic results. Fifty-seven lines chosen for their phenotype were evaluated with 17 microsatellite primers and eight primers were tested on 149 lines of the Diamant/TD12A population. The primers tested represented the whole genome based on the SSR map developed by Somers et al. (Citation2004). Primer sequence, annealing temperature, and chromosome locations were those reported by Röder et al. (1998a, 1998b), Somers et al. (Citation2004) and the GrainGenes database (http://wheat.pw.usda.gov). The PCR reactions were performed as described by Knox et al. (Citation2013). In some instances, despite best attempts, marker data were missing for a particular line which appears as different population sizes across races for a given genetic population.
Statistical and genetic analysis
The two inoculation series for each experimental set of lines performed at different times were treated as randomized blocks during the assessment phase. Means of replicates over time were calculated for the 9340/Vista and Diamant/TD12A populations using the LSMeans option in SAS for a randomized complete block design (SAS Citation1999). Frequency distributions of loose smut incidence for each race in each of the doubled haploid populations were plotted. The LS means of the two sets of lines of the 9340/Vista population were combined for QTL analysis after plotting the data and determining the distributions were consistent. The greater number of degrees of freedom from combining data from the two sets was considered to improve precision in the QTL analysis more than any loss in precision from using results from two experiments. Initially single point quantitative analysis for association between markers and loose smut resistance was performed using SAS Student’s t-test to compare the mean loose smut incidence of lines with the molecular variant of the resistant parent with the mean of the lines with the molecular variant of the susceptible parent for each loose smut race. The mean and standard error of each mean was generated by SAS. Markers were mapped using JoinMap® (Van Ooijen Citation2006). Simple interval mapping was subsequently performed using MQTL software (Tinker & Mather Citation1995). One thousand permutations of the data were performed within MQTL to determine what is referred to as the Test Statistic values at the critical level. The Test Statistic relates to LOD but is not an LOD per se. Molecular markers highly significantly associated with a resistance reaction at P < 0.001 were considered major QTL (Lander & Kruglyak Citation1995).
Results
Knox et al. (Citation2008a) described the loose smut incidence results for the Glenlea/Taber population. Per cent loose smut incidence levels in the susceptible check cultivars were generally very high, with the majority of check values across races averaging greater than 80% incidence for ‘Biggar’ and ‘AC Taber’. ‘AC Vista’ and ‘AC Karma’ also averaged greater than 80% incidence of loose smut when inoculated with race T9. ‘Diamant’ averaged greater than 60% incidence of loose smut to race T2 and greater than 80% for races T9 and T27. The broad ranges in incidence values within the populations are presented in also shows the class interval in which the parents fell. ‘Glenlea’ showed complete resistance to loose smut races T2, T9, T10, T15, T19 and T39 (Knox et al. Citation2008a) as did 9340-CP* to race T9. TD12A expressed nearly complete resistance with only a trace of loose smut which when rounded off fell within the 0% incidence class for T2 and T9, and resistance was complete to T27. ‘SC8021V2’ was also resistant but with a lower penetrance than ‘Glenlea’, 9340-CP* and TD12A. ‘AC Karma’ was susceptible to race T9, and expressed complete resistance to races T39 and T10 (no rateable plants) with the majority of the T39 inoculated plants and all of the T10 inoculated plants expressing the hypersensitive reaction.
Table 2. Frequency of wheat lines within class intervals of per cent loose smut incidence for populations 9340/Vista, Diamant/TD12A, Glenlea/Taber, and 8021/Karma and appropriate races. Intervals are represented by the midpoint of the class.
The number of observations on which incidence values were based for each line in the genetic populations varied because of differences in size of inoculated spikes, the number of spikes inoculated, seed set, and seed and plant survival. The mean number of plants scored per line for each population was well above the target of 15 – for the 8021/Karma population it was 42 (range 15 to 97), for the 9340/Vista it was 88 (range 8 to 234, only one line with fewer than 20 plants scored), and for the Diamant/TD12A it was 102 (range 10 to 261, each of three lines with less than 15 rateable plants had over 90 seeds sown).
The distributions of loose smut incidence of the 13 race-population combinations are shown in The 9340/Vista segregated for incidence to race T9 with nearly three times as many resistant as susceptible lines using the traditional 10% division point between resistant and susceptible (Knox et al. Citation2008a). The segregation fitted a 3:1 ratio (χ2 = 1.610, P = 0.20), indicating the presence of two major genes for resistance. Similarly, the Diamant/TD12A to T27 indicated two major genes (χ2 = 0.142, P = 0.71), as did 8021/Karma to T10 (χ2 = 2.674, P = 0.10). The Diamant/TD12A showed a similar segregation pattern to race T9 () and to T2; however, the bimodal distribution ratios did not fit 1:1 resistant to susceptible (T9 χ2 = 4.167, P = 0.04), indicating some degree of genetic complexity. The 8021/Karma to race T39 continuously skewed distribution () also indicated complex segregation. The 8021/Karma to race T9 () showed the simplest segregation, indicating a single major gene (χ2 = 0.350, P = 0.55).
The 8021/Karma displayed a differential response to the three loose smut races as was observed with the Glenlea/Taber population (Knox et al. Citation2008a). No lines were susceptible to T10 alone, yet there were lines susceptible to the three races T9, T10 and T39. A group of lines were susceptible to only T9 and a different group of lines were susceptible to only T39. Another group of lines were susceptible to both T9 and T39.
summarizes the population, chromosome location and races for which QTL for resistance to U. tritici were identified. There are too many combinations of race by population by QTL to go into great detail on each. We will therefore focus in detail on the races to which the greatest QTL effect was observed. The results from ‘Glenlea’ are presented first followed by validations with other populations, and finally results of additional QTL from non-‘Glenlea’ populations will be considered. Figs 2–7 show plots of the MQTL Test Statistic across markers with the threshold shown in each chart as a straight dotted line for the P = 0.05 level of significance. Where multiple races are reported in one chart the most conservative threshold is indicated. We report within the following text the MQTL Test Statistics (TS) and associated probability (P) of individual marker-race-population combinations.
Table 3. Chromosome location and parental source of QTL for resistance to races of Ustilago tritici in four wheat biparental mating populations.
A QTL, QUt.spa-5B, was identified in the interval Xwmc734 to Xbarc59 (25.6 cM) on chromosome 5B for resistance to races T2, T10, T15, T19 and T39 in the Glenlea/Taber population from ‘Glenlea’ (). The QTL peak was at Xwmc734 for races T10 (TS = 22.0, P < 0.0001) and T15 (TS = 16.2, P < 0.01), and Xwmc160 for races T2 (TS = 13.4, P < 0.0001), T19 (TS = 64.9, P < 0.0001) and T39 (TS = 66.4, P < 0.0001) (). The effect of resistance of the QTL was greatest and similar for races T19 and T39. The maximum difference in loose smut incidence between the molecular variant associated with resistance derived from ‘Glenlea’ and the molecular variant associated with susceptibility for marker Xwmc160 was 57% (±5.4) for race T19. No significant effect was observed for the 5B locus against race T9. Another QTL derived from ‘Glenlea’ () identified in the Glenlea/Taber population, QUt.spa-7B, had the greatest impact on resistance to race T9 (TS = 33.3, P < 0.0001) and was located in the interval Xgwm302 to Xwmc723 (5.4 cM) on chromosome 7B, with the QTL peak at Xgwm302 (). The locus also appeared as a QTL for resistance to races T2 (TS = 9.6, P < 0.05) and T15 (TS = 15.4, P < 0.01) at Xgwm302 using MQTL analysis and no significant effect against races T10, T19 and T39 was detected. The maximum difference in loose smut incidence for race T9 between the ‘Glenlea’ derived molecular variant and the molecular variant associated with susceptibility for marker Xgwm302 was 42.5% (±6.5).
Fig. 2 The LOD for QTL for resistance to Ustilago tritici identified for Glenlea/Taber chromosome 5B with races T2 (■, solid line), T10 (●, dashed line), T15 (×, solid line), T19 (▲, dotted line) and T39 (Δ, dashed line). The MQTL Test Statistic threshold is shown as a straight dotted line for the P = 0.05 level of significance for the most conservative threshold.
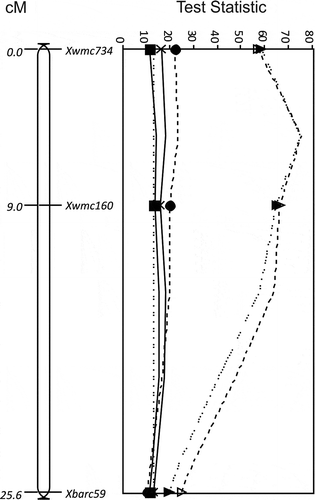
Fig. 3 The LOD for QTL for resistance to Ustilago tritici identified for Glenlea/Taber chromosome 7B with races T2 (■, solid line), T9 (○, solid line) and T15 (×, solid line). The MQTL Test Statistic threshold is shown as a straight dotted line for the P = 0.05 level of significance for the most conservative threshold.
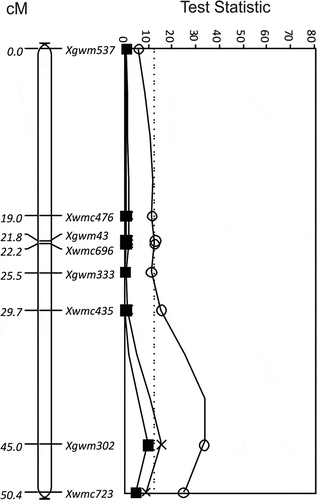
Table 4. Summary of chromosome, line, gene, races to which genes were found to be effective and ineffective, peak QTL, QTL bracketing markers and other significant markers within the QTL interval.
A QTL was identified with MQTL deriving from ‘Glenlea’ on chromosome 3A (QUt.spa-3A) with resistance to races T9 (TS = 11.5, P = 0.08), T19 (TS = 13.1, P = 0.05) and T39 (TS = 14.4, P = 0.02) at marker Xwmc559 (). The QTL was highly significant (e.g. t = 3.97, P < 0.001 for race T39) in single point analysis not only at Xwmc559, but also at markers Xwmc96 and Xgwm497. The ‘Glenlea’ molecular variant was responsible for a 30.3% (±8.1) reduction in T19 loose smut incidence.
As with ‘Glenlea’, the interval Xgwm604 to Xbarc59 on chromosome 5B (34.2 cM) expressed the QTL QUt.spa-5B for resistance to race T39 in the 8021/Karma population using MQTL analysis (). The resistance derived from ‘AC Karma’ () with the peak at marker Xgdm133a (TS = 54.8, P < 0.0001). The parents ‘SC8021V2’ and ‘AC Karma’ were very polymorphic which is reflected in the high density of markers on the map of the 8021/Karma population and as a result 13 markers were significantly associated with resistance (). The Xwmc160 and Xbarc59 markers identified in the Glenlea/Taber population were among the markers within the QTL interval of the 8021/Karma population; however, the Xwmc734 marker appeared only in the Glenlea/Taber population. The greatest reduction in incidence with race T39 of 37.5% (±4.8) was observed at marker Xgdm133a.
Fig. 4 The LOD for QTL for resistance to Ustilago tritici identified for 8021V2/Karma chromosome 5B with race T39 (Δ, dashed line). The MQTL Test Statistic threshold is shown as a straight dotted line for the P = 0.05 level of significance for the most conservative threshold.
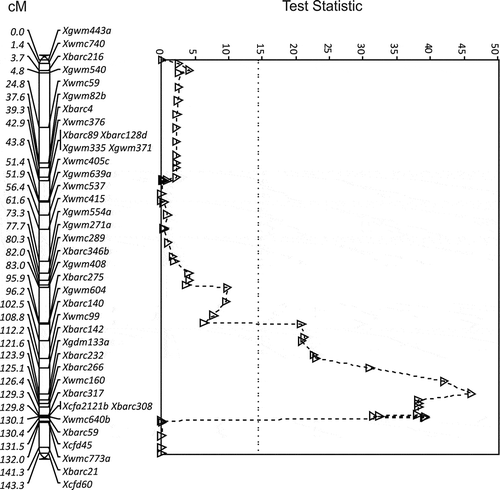
The same region as the 7B QTL in ‘Glenlea’ also produced the QUt.spa-7B QTL for resistance to race T9 from 9340-CP* () and T2, T9 and T27 from TD12A (). QUt.spa-7B was strongest at Xwmc723 in both the Diamant/TD12A (TS = 69.8, P < 0.0001) and 9340/Vista populations (TS = 307.4, P < 0.0001) (). QUt.spa-7B in the 9340/Vista population was associated with seven markers on chromosome 7B that spanned 87.6 cM (). The QUt.spa-7B on 7B in the Diamant/TD12A population spanned 39.6 cM and included four markers that were in a slightly different order to those in the same map region of 9340/Vista. The Xwmc723 marker was associated with loose smut resistance in all three populations, Glenlea/Taber, 9340/Vista and Diamant/TD12A. Xwmc723 was associated with a maximum reduction of 68.3% (±2.2) loose smut incidence from race T9 in the Diamant/TD12A population and 37.6% (±4.2) in the 9340/Vista population.
Fig. 5 The LOD for QTL for resistance to Ustilago tritici identified for 9340/Vista chromosome 7B with race T9 (○, solid line). The MQTL Test Statistic threshold is shown as a straight dotted line for the P = 0.05 level of significance for the most conservative threshold.
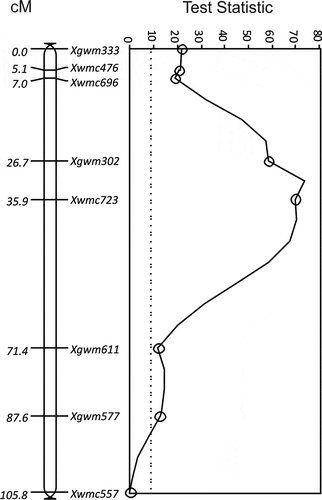
Fig. 6 The LOD for QTL for resistance to Ustilago tritici identified for Diamant/TD12A chromosome 7B with races T2 (■, solid line), T9 (○, solid line) and T27 (◊, dotted line). The MQTL Test Statistic threshold is shown in each chart as a straight dotted line for the P = 0.05 level of significance for the most conservative threshold.
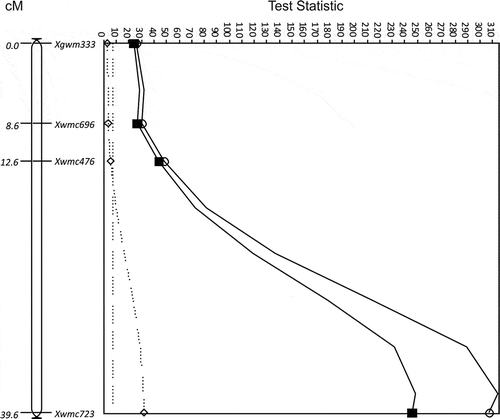
QUt.spa-3A also was identified as a QTL for resistance to race T9 in the 9340/Vista population at the only two markers mapped in the region, Xwmc559 and Xbarc69 (TS = 52.5, P < 0.0001), on chromosome 3A from 9340-CP* (). The markers, Xbarc69 and Xwmc559, mapped 2 cM apart. The difference in incidence between lines pooled by molecular variant was 33.3% (±4.3) for both markers. The Xwmc559 was associated with resistance to race T39 in the 9340/Vista and Glenlea/Taber populations.
The QTL, QUt.spa-7A, for resistance to race T9 was uniquely identified in the 8021/Karma population from ‘SC8021V2’ () within the interval Xcfa2019 to Xgwm344a (26.3 cM) on chromosome 7A (). The greatest reduction in incidence from race T9 was 70.4% (±3.3) observed at marker Xcfa2240 (TS = 167.3, P < 0.0001). Another unique QTL, QUt.spa-6D, for resistance to race T10 was also identified from ‘SC8021V2’ on chromosome 6D near Xbarc183 (=11.0, P < 0.05) (). The locus was associated with a 27.3% (±7.5) reduction in loose smut incidence. A third unique QTL for resistance was to race T39 on chromosome 6B in the 8021/Karma population identified as highly significant (P < 0.001) using single point analysis () for two markers and P < 0.01 for 12 markers that mapped contiguously. This QTL for resistance derived from ‘SC8021V2’, but was just below the significance threshold using MQTL. The maximum difference in loose smut incidence associated with marker Xbarc223 was 19.6% (±5.3).
Fig. 7 The LOD for QTL for resistance to Ustilago tritici identified for 8021V2/Karma chromosome 7A with race T9 (○, solid line). The MQTL Test Statistic threshold is shown as a straight dotted line for the P = 0.05 level of significance for the most conservative threshold.
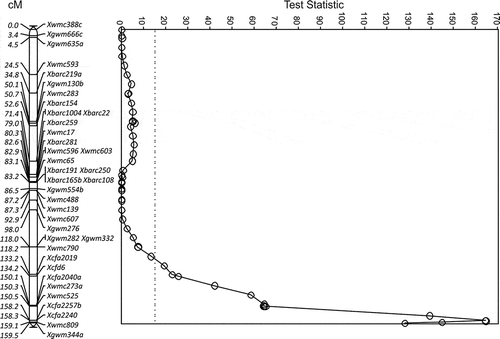
The distribution of resistance to race T10 in the Glenlea/Taber population is noteworthy. Only one QTL was detected for resistance to race T10 yet the lines segregated 48 resistant (less than 4% incidence) to 10 susceptible (greater than 60% incidence) and four intermediate (12.5%, 22.5%, 47.5% and 50.0% incidence) indicating more than one resistance gene segregating.
Discussion
The high incidence level of loose smut, generally around 90%, in the check cultivars and the segregation of susceptible lines within the populations indicated that disease pressure was high, with few escapes. The high infection level meant the expression of resistance phenotypes was optimized so that the differential among susceptible and resistance phenotypes of varying penetrance was maximized. For example, the incomplete penetrance for resistance to T9 is obvious in the 8021/Karma and Diamant/TD12A populations, with only a few of the resistant lines showing a small proportion of plants with loose smut symptoms. Less than complete resistance expression is likely in the other race-population combinations as well, but merging with reactions of intermediate to moderately susceptible lines.
The differential pattern of segregation to the three races applied to the 8021/Karma population indicated that multiple genes were present in the population and supported the finding of multiple QTL. Knox et al. (Citation2008a) also concluded segregation from ‘Glenlea’ to be complex because of the differential reaction to different races by the progeny. We further demonstrated complexity of segregation, with the distribution of loose smut incidence in the progeny of the resistant class being skewed, indicating the need for modifier genes for complete expression of major genes, as was the case with 9340/Vista to race T9. Alternatively, a complex segregation ratio that demonstrated complementary gene action combined with a major gene was seen with Diamant/TD12A to race T9. In the 8021/Karma population to race T39, segregation in lines in the half of the population not associated with a major gene was continuous and quantitative, further supporting yet another form of complexity. The quantitative genetic analysis was justified based on the complex patterns of segregation in these race-population combinations. But not all segregation was complex because resistance to race T9 in the 8021/Karma population, for example, was apparently under simple genetic control. The simple pattern of segregation of resistance derived from ‘SC8021V2’ to race T9 that indicated a single major gene was supported by the QTL analysis in which a single major QTL, QUt.spa-7A, was observed within the interval Xcfa2019 to Xgwm344a on chromosome 7A. The 8021/Karma was a validation population for resistance derived from ‘Glenlea’ within ‘AC Karma’ primarily to race T39, but also to T10, but this population also provided the opportunity to study the loose smut resistance within ‘SC8021V2’. ‘SC8021V2’ contributed QUt.spa-7A and QUt.spa-6D QTL which did not appear in the Glenlea/Taber population. Even though these QTL were not determined to derive from ‘Glenlea’ in this study, the presence of QUt.spa-7A or QUt.spa-6D within ‘Glenlea’ cannot be ruled out. It may be that the genetic map was not sufficiently dense to identify the QTL in the Glenlea/Taber population. Dhitaphichit et al. (Citation1989) reported the presence of a gene with complete resistance to race T6 on chromosome 7A from ‘Hope’, which supports our finding of the QUt.spa-7A QTL on 7A. Given that the QTL did not appear with inoculations of the 8021/Karma population to races T39 and T10, the gene does not provide resistance to races T39 and T10. The gene controlling loose smut resistance within the QUt.spa-7A QTL was designated Ut7.
Despite the complex nature of segregation of some race-population combinations, the pattern of segregation supported the outcome of QTL analysis and was suggestive of the number of genes involved and of the nature of the gene interactions. The indication of two major genes for resistance from the segregation pattern of 9340/Vista reinforced the identification of two major QTL, QUt.spa-7B and QUt.spa-3A, for resistance to race T9 in the population. Given that both QTL were derived from the 9340-CP* parent in the 9340/Vista population, and the high coefficient of parentage with ‘Glenlea’, 9340-CP* likely inherited the resistance from ‘Glenlea’. The resistance in 9340-CP* and ‘Glenlea’ to race T9 residing in the same regions on chromosomes 3A and 7B solidifies the relationship between the two parents for QUt.spa-3A and QUt.spa-7B. Although ‘AC Vista’ inherited loose smut resistance from ‘Glenlea’ for races T2, T10 and T39, the results show that it received neither the 3A nor the 7B locus for resistance to T9 from ‘Glenlea’. No segregation was expected in the 9340/Vista population to races T2, T10 and T39, therefore the additional races were not applied, but the Glenlea/Taber population revealed more about the breadth of resistance of QUt.spa-7B and QUt.spa-3A. From assessment of the Glenlea/Taber population, not only does QUt.spa-3A control resistance to race T9, but also to T19 and T39 (). Similarly, QUt.spa-7B controls resistance to T9 as well as to T15 and T2 (). The occurrence of QUt.spa-7B and QUt.spa-3A in the 9340/Vista population validates our discovery of their presence in ‘Glenlea’.
The QUt.spa-7B QTL was also identified in TD12A, which is the replacement of the TD12, Thatcher-based loose smut differential with simplified resistance (Nielsen & Dyck Citation1988). The commonality of this QTL between ‘Thatcher’, ‘Glenlea’ and 9340-CP* is not solely based on the similar location and common phenotype of resistance to race T9, but on ‘Thatcher’ and ‘Glenlea’ having a substantial coefficient of parentage (). Not only was QUt.spa-7B within TD12A associated with resistance to race T9, it was also linked to resistance to races T27 and T2 (). McIntosh et al. (Citation2013) postulated, based on the segregation of virulence in the pathogen studied by Nielsen (Citation1982), a gene for resistance in TD12 to race T2, and proposed the name Ut4 for the gene. We confirmed the presence of a gene in TD12A, which segregated as a major gene with resistance to race T2, to be in the QTL QUt.spa-7B and consider it to be Ut4. Although the progeny of the Diamant/TD12A cross generated a bimodal distribution, the preponderance of susceptible lines that was observed () indicated genetic control beyond a single major gene. Work by Dhitaphachit et al. (1989) and confirmed by Knox (Citation1994), using ‘Chinese Spring’ (‘Thatcher’) disomic chromosome substitution lines, demonstrated that ‘Thatcher’ possesses a major gene conferring complete resistance to race T6 on chromosome 7B. Both studies also found that other chromosomes carry factors that affected resistance. Although the deviation from a 1:1 segregation in the present work could be by chance, it is unlikely given the size of the population. Instead, the segregation pattern could reflect the presence of a susceptibility or suppressor factor. Such a disease resistance suppressor has been reported in ‘Thatcher’ for rust resistance (Kerber & Green Citation1980). To summarize, based on information from all populations, the QUt.spa-7B gene, Ut4, is resistant to races T2, T9, T15 and T27, but is susceptible to races T10, T19 and T39, which is the same pattern reported by Menzies et al. (Citation2003) for TD12A. We conclude from the chromosomal location and host-race interaction that ‘Glenlea’ and 9340-CP* also possess Ut4.
The QTL, QUt.spa-3A, on chromosome 3A from ‘Glenlea’, is the same as that derived from 9340-CP* based on the chromosomal location at Xwmc559 and the common T9 resistance phenotype. As with QUt.spa-7B QTL, the high coefficient of parentage between ‘Glenlea’ and 9340-CP* () further supports the notion that these two lines could share a common resistance factor within QUt.spa-3A. The distribution of progeny from 9340-CP* suggests there are two major genes for resistance to race T9 and is consistent with stacked genes. The results from the 9340/Vista population validate the results from the Glenlea/Taber population. The gene within QUt.spa-3A was designated Ut8. Based on the testing of multiple races on the Glenlea/Taber population, the Ut8 gene, like Ut4, provided resistance to race T9, and also resistance to races T19 and T39 but not to races T15, T10 and T2 (). Although ‘AC Vista’ and ‘AC Karma’ were selections from crosses involving ‘Glenlea’ as a source of loose smut resistance, their susceptibility to race T9 indicated that not only did they not acquire Ut4, they also did not inherit Ut8. The loss of resistance in ‘AC Karma’ compared with ‘Glenlea’ is not unexpected given that in the development of ‘AC Karma’, five backcrosses were made to susceptible ‘HY320’ after an initial cross with ‘Glenlea’, and race T9 was not used during the selection of progeny.
The third QTL, QUt.spa-5B, identified from ‘Glenlea’ in this study, was found on chromosome 5B in the interval Xwmc734 to Xbarc59 with the QTL peak near Xwmc160. The QTL was substantiated by also being identified in the 8021/Karma population, and derived from ‘AC Karma’ in the same chromosomal region as the QTL identified from ‘Glenlea’. From ‘Glenlea’, although ineffective against race T9, QUt.spa-5B provided resistance to races T19, T39, T15, T2 and T10 in the Glenlea/Taber population (). QUt.spa-5B was identified in the 8021/Karma population through resistance to race T39 (T19, T15 and T2 were not used in this population), but unlike the Glenlea/Taber population, the QTL was not significant for resistance to race T10 even though an effect was observed with several markers at the locus using single point analysis. The distribution of the progeny from the 8021/Karma population suggested more than just one major gene was segregating for resistance to T39. The very high proportion of resistant progeny (48 resistant out of 62 plants) indicated that more than one gene for resistance to race T10 segregated in Glenlea/Taber. The complexity of interactions among genes may be the reason that race T10 resistance was not convincingly identified for QUt.spa-5B in the 8021/Karma population. Knox et al. (Citation2008a) suggested that the Glenlea/Taber segregation best fit a model of one major gene and multiple additive genes for resistance to race T10. Nonetheless, the gene in the QUt.spa-5B from ‘Glenlea’ was validated in the 8021/Karma population and was designated Ut6. Given that ‘AC Karma’ has ‘Glenlea’ in its pedigree and a coefficient of parentage of 0.2 (), it is likely that ‘AC Karma’, which is resistant to races T2, T10 and T39, but not T9, acquired Ut6 from ‘Glenlea’. Given ‘AC Vista’ has the same response to races T2, T10, T39 and T9 as ‘AC Karma’, indications are that ‘AC Vista’ also possesses Ut6, and it is very likely that 9340-CP* with resistance to races T2, T10 and T39 possesses Ut6. With Ut6 in both parents, no segregation was expected in 9340/Vista for resistance to races T2, T10 and T39.
In the course of validating QTL identified from ‘Glenlea’ in the Glenlea/Taber population, other QTL were identified in the 8021/Karma population. The major QTL, QUt.spa-7A, was discussed previously, but a minor QTL was located on chromosome 6D with SIM and a highly significant QTL, QUt.spa-6B, was identified on chromosome 6B at Xbarc223 and Xbarc332b with single point QTL analysis (). Both QTL were derived from ‘SC8021V2’. Dhitaphichit et al. (Citation1989) and Knox (Citation1994) noted a substantial reduction in loose smut when the ‘Thatcher’ 6D chromosome was substituted into ‘Chinese Spring’. Based on the unique chromosome location, we designate the gene for resistance to U. tritici on chromosome 6D as Ut10.
Experiment-wise error leading to the false declaration of significant markers is considered to be a risk associated with single point analysis. The QUt.spa-6B QTL produced a real resistance effect, that is to say that it was not a spurious result because of experiment-wise error, because multiple contiguous markers demonstrated the same or similar phenotypic effects. The likelihood of experiment-wise error generating multiple spurious results in at least 12 adjacent markers is extremely low. Based on the unique chromosome location of QUt.spa-6B, we designate the gene on chromosome 6B from ‘SC8021V2’ as Ut9.
Knox et al. (Citation1999) determined the presence of two genes segregating to race T19 in a cross involving the two resistant parents HY377 and ‘SC8021V2’. HY377 is a backcross line of ‘HY320’ (DePauw et al. 1987) that used ‘Glenlea’ as a source of loose smut resistance. The gene in HY377 provided resistance to races T2, T10, T19 and T39, which matches the pattern for Ut6. Therefore, it is likely that HY377 acquired Ut6 from ‘Glenlea’. Knox et al. (Citation1999) used race T19 in their study involving ‘SC8021V2’, and T19 has similar virulence as that of race T39 (Nielsen Citation1987) that was used in the present study. The second gene in the cross of HY377 by ‘SC8021V2’ is possibly the 6B gene, Ut9, identified from ‘SC8021V2’ in the present study.
Even though numerous QTL were found in this study, there were limitations. Density and coverage of markers on the maps affects discovery of resistance genes. Both low density maps and sporadic coverage affect whether or not a locus is identified. In some of the crosses, both parents had resistance to the same races. The parents may then have genes that do not segregate and therefore would not be observed in this study. Further work is needed to understand the breadth of resistance of the genes in single gene backgrounds. Future work should involve increasing the map density to improve marker coverage to fill in gaps. Fine mapping is needed to identify markers closer to the genes, and more data are needed to confirm the QTL identified on chromosome 6D. With greater map density and coverage it may be possible to identify additional resistance loci. Epistasis analysis would be useful to clarify the nature of gene interactions.
The QTL markers, specific genes encompassed, associated plant lines, breadth of resistance to races tested, and associated markers are summarized in . The population Glenlea/Taber was evaluated for complete and broad resistance from ‘Glenlea’ to races that covered the virulence in the predominant races of loose smut found on the Canadian prairies. The ‘Glenlea’ QTL, QUt.spa-7B, occurred at the same locus in three crosses, while QUt.spa-3A and QUt.spa-5B each appeared in two crosses. In total, six QTL were identified that have potential for use in marker-assisted selection in breeding programmes. Given the present information, three of the genes identified can be classified as major genes because of their substantial effect on reducing disease incidence. Ut4 and Ut7 each reduced the level of loose smut incidence by nearly 70%, and Ut6 by nearly 60% in cases of maximum expression of resistance. The Ut6 gene in combination with one of Ut4, Ut7 or Ut8 would provide resistance to the prevalent races of Ustilago tritici found on the Canadian prairies. Given ‘Glenlea’ is derived from ‘Thatcher’, as are many other registered spring wheat cultivars in Canada, the markers identified here should be useful in Canadian breeding programmes for resistance to U. tritici.
Acknowledgements
This study was financially supported by the Matching Investment Initiative of Agriculture and Agri-Food Canada and the Western Grains Research Foundation industry partnering funds. We thank Brad Meyer, Theresa Colenutt, Alana Balliett, Stephen Walter, Kristen Stengler, Malina Poppy and Yanfen Zheng for their technical support.
References
- Clarke FR, Knox RE, DePauw RM. 2005. Expression of dormancy in a spring wheat cross grown in field and controlled environment conditions. Euphytica. 143:297–300. doi:10.1007/s10681-005-7886-9
- DePauw RM, Knox RE, Morrison RJ, McCaig TN, Clarke JM, McLeod JG. 1994. Registration of ‘AC Taber’ hard red spring wheat. Crop Sci. 34:305. doi:10.2135/cropsci1994.0011183X003400010066x
- DePauw RM, McCaig TN, Clarke JM, McLeod JG, Knox RE, Fernandez MR. 1992. Registration of sprouting-tolerant white-kerneled wheat germplasms SC8019R1 and SC8021V2. Crop Sci. 32:838. doi:10.2135/cropsci1992.0011183X003200030069x
- DePauw RM, McCaig TN, Knox RE, Clarke JM, Fernandez MR, McLeod JG. 1998. AC Vista hard white spring wheat. Can J Plant Sci. 78:617–620. doi:10.4141/P97-150
- Dhitaphichit P, Jones P, Keane EM. 1989. Nuclear and cytoplasmic gene control of resistance to loose smut (Ustilago tritici (Pers.) Rostr.) in wheat (Triticum aestivum L.). Theor Appl Genet. 78:897–903. doi:10.1007/BF00266678
- Doyle JJ, Doyle JL. 1987. A rapid DNA isolation procedure for small quantities of fresh leaf tissue. Phytochem Bull. 9:11–15.
- Evans LE, Shebeski LH, McGinnis RC, Briggs KG, Zuzens D. 1972. Glenlea red spring wheat. Can J Plant Sci. 52:1081–1082. doi:10.4141/cjps72-184
- Hussain A, Lukow OM. 1997. Influence of Gliadin-Rich Subfractions of Glenlea Wheat on the Mixing Characteristics of Wheat Flour1,2. Cereal Chem. 74:791–799. doi:10.1094/CCHEM.1997.74.6.791
- IRRI-CIMMYT Crop Research Informatics Laboratory (CRIL). 2014. International Crop Information System, Philippines and Mexico [Internet]. Available from: http://cropwiki.irri.org/icisweb/
- Kerber ER, Green GJ. 1980. Suppression of stem rust resistance in the hexaploid wheat cv. Canthatch by chromosome 7DL. Can J Bot. 58:1347–1350. doi:10.1139/b80-166
- Knox R, Menzies J. 2012. Resistance in wheat to loose smut. In: Sharma I. editor. Disease resistance in wheat. Oxfordshire, UK: CAB International.
- Knox RE. 1994. Chromosomal location and linkage analysis of loose smut resistance in wheat. [dissertation]. Winnipeg (MB): University of Manitoba. Available from: http://hdl.handle.net/1993/18126.
- Knox RE, Campbell HL, Clarke JM, DePauw RM, Procunier JD, Howes NK. 2008a. Genetics of resistance to Ustilago triticiin ‘Glenlea’ wheat (Triticum aestivum). Can J Plant Pathol. 30:267–276. doi:10.1080/07060661.2008.10540542
- Knox RE, Campbell HL, Clarke JM, Fernandez MR, McLeod JG, DePauw RM. 2008b. Registration of DT676 loose smut resistant durum wheat germplasm. J Plant Registrations. 2:156–161. doi:10.3198/jpr2007.06.0342crg
- Knox RE, Campbell HL, DePauw RM, Gaudet D, Puchalski B, Clarke FR. 2013. DNA markers for resistance to common bunt in ‘McKenzie’ wheat. Can J Plant Pathol. 35:328–337. doi:10.1080/07060661.2013.763292
- Knox RE, Clarke FR, Clarke JM, Fox SL, DePauw RM, Singh AK. 2012. Enhancing the identification of genetic loci and transgressive segregants for preharvest sprouting resistance in a durum wheat population. Euphytica. 186:193–206. doi:10.1007/s10681-011-0557-0
- Knox RE, Clarke JG, Menzies J.G, Aung T, Howes NK, Penner GA. 2002. Genetic analysis of resistance to loose smut and an associated DNA marker in durum wheat doubled haploids. Can J Plant Pathol. 24:316–322. doi:10.1080/07060660209507015
- Knox RE, Clarke JM, DePauw RM. 2000. Dicamba and growth condition effects on doubled haploid production in durum wheat crossed with maize. Plant Breed. 119:289–298. doi:10.1046/j.1439-0523.2000.00498.x
- Knox RE, DePauw RM, McCaig TN, Clarke JM, McLeod JG, Fernandez MR. 1995. AC Karma white spring wheat. Can J Plant Sci. 75:899–901. doi:10.4141/cjps95-150
- Knox RE, DePauw RM, McCaig TN, Clarke JM, McLeod JM, Morrison RJ. 1992. AC Taber red spring wheat. Can J Plant Sci. 72:1241–1245. doi:10.4141/cjps92-154
- Knox RE, Fernandez MR, Brûleacute;-Babel AL, DePauw RM. 1999. Inheritance of loose smut (Ustilago tritici) resistance in two hexaploid wheat (Triticum aestivum) lines. Can J Plant Pathol. 21:174–180. doi:10.1080/07060669909501209
- Lander E, Kruglyak L. 1995. Genetic dissection of complex traits: Guidelines for interpreting and reporting linkage results. Nat Genet. 11:241–247. doi:10.1038/ng1195-241
- McIntosh R, Yamazaki Y, Dubcovsky J, Rogers J, Morris C, Appels R, Xia X. 2013. Catalogue of gene symbols for wheat [Internet]. Japan: Yokohama, Available from: http://www.shigen.nig.ac.jp/wheat/komugi/genes/download.jsp;jsessionid=690DE9AC6838788C9FD4C22D6DE1E 6C7.lb1. [accessed 4 April 2014].
- Menzies JG, Nielsen J, Thomas PL, Knox RE. 2003. Virulence of Canadian isolates of Ustilago tritici: 1964–1998, and the use of the geometric rule in understanding host differential complexity. Can J Plant Pathol. 25:62–72. doi:10.1080/07060660309507050
- Nielsen J. 1977. Inheritance of virulence of loose smut of wheat, Ustilago tritici, on the differential cultivars Renfrew, Florence x Aurore, Kota and Little Club. Can J Bot. 55:260–263. doi:10.1139/b77-036
- Nielsen J. 1982. Inheritance of virulence of Ustilago triticion the differential cultivars Carma, Red Bobs, and a derivative of the cross Thatcher × Regent. Can J Bot. 60:1191–1193. doi:10.1139/b82-148
- Nielsen J. 1983. Spring wheats immune or highly resistant to Ustilago tritici. Plant Dis. 67:860–863. doi:10.1094/PD-67-860
- Nielsen J. 1987. Races of Ustilago tritici and techniques for their study. Can J Plant Pathol. 9:91–105. doi:10.1080/07060668709501888
- Nielsen J, Dyck PL. 1988. Three improved differential hosts to identify races of Ustilago tritici. Can J Plant Pathol. 10:327–331. doi:10.1080/07060668809501707
- Nielsen J, Thomas P. 1996. Loose smut. In: Wilcoxson RD, Saari EE, editors. Bunt and smut diseases of wheat: Concepts and methods of disease management. Mexico (DF): CIMMYT; pp. 33–47.
- Nielsen J, Tikhomirov V. 1993. Races of Ustilago tritici identified in field collections from eastern Siberia using Canadian and Soviet differentials. Can J Plant Pathol. 15:193–200. doi:10.1080/07060669309500822
- Pauw RM, Hurd EA, Towni.Ky-Smith TF, McCrystal GE, Lendrum CWB. 1987. HY320 red spring wheat. Can J Plant Sci. 67:807–811. doi:10.4141/cjps87-110
- Procunier JD, Knox RE, Bernier AM, Gray MA, Howes NK. 1997. DNA markers linked to a T10 loose smut resistance gene in wheat (Triticum aestivum L.). Genome. 40:176–179. doi:10.1139/g97-025
- Randhawa HS, Popovic Z, Menzies J, Knox R, Fox S. 2009. Genetics and identification of molecular markers linked to resistance to loose smut (Ustilago tritici) race T33 in durum wheat. Euphytica. 169:151–157. doi:10.1007/s10681-009-9903-x
- Röder MS, Korzun V, Gill BS, Ganal MW. 1998. The physical mapping of microsatellite markers in wheat. Genome. 41:278–283. doi:10.1139/g98-009
- Röder MS, Korzun V, Wendehake K, Plaschke J, Tixier MH, Leroy P, Ganal MW. 1998. A microsatellite map of wheat. Genetics. 149:2007–2023.
- SAS. 1999. SAS/Stat software: Changes and enhancements through release 8. Cary (NC): SAS Institute Inc.
- Singh A, Pandey MP, Singh AK, Knox RE, Ammar K, Clarke JM, Clarke FR, Singh RP, Pozniak CJ, DePauw RM, et al. 2013. Identification and mapping of leaf, stem and stripe rust resistance quantitative trait loci and their interactions in durum wheat. Mol Breed. 31:405–418. doi:10.1007/s11032-012-9798-4
- Sneller CH. 1994. SAS programs for calculating coefficient of parentage. Crop Sci. 34:1679–1680. doi:10.2135/cropsci1994.0011183X003400060047x
- Somers DJ, Isaac P, Edwards K. 2004. A high-density microsatellite consensus map for bread wheat (Triticum aestivum L.). Theor Appl Genet. 109:1105–1114. doi:10.1007/s00122-004-1740-7
- Tinker NA, Mather DE. 1995. MQTL: Software for simplified composite interval mapping of QTL in multiple environments. J Agric Genomics (formerly J Quant Trait Loci). 1/2:2.
- Van Ooijen JW. 2006. JoinMap® 4.0, software for the calculation of genetic linkage maps in experimental populations. Wageningen: Plant Research International.
- Wells DG. 1962. Notes on the hybridization of wheat and barley. Crop Sci. 2:177–178. doi:10.2135/cropsci1962.0011183X000200020034x
- Wells DG, Caffey HR. 1956. Scissor emasculation of wheat and barley. Agron J. 48:496–499. doi:10.2134/agronj1956.00021962004800110005x
- Xue S, Xu F, Tang M, Zhou Y, Li G, An X, Lin F, Xu H, Jia H, Zhang L, et al. 2011. Precise mapping Fhb5, a major QTL conditioning resistance to Fusarium infection in bread wheat (Triticum aestivum L.). Theor Appl Genet. 123:1055–1063. doi:10.1007/s00122-011-1647-z