Abstract
The genetic diversity and population structure among 72 rice-infecting isolates of Rhizoctonia solani AG-1 IA, collected from 12 counties (subpopulations) of Guangdong, Guangxi and Hainan provinces (populations) in south China, were investigated using nine inter-simple sequence repeat (ISSR) markers. A total of 116 bands were amplified, with a majority of amplified fragments ranging from 500 bp to 2500 bp in size, of which 110 (94.8%) were polymorphic. Seventy-two isolates were grouped into six major clusters at 73% genetic similarity coefficient by the unweighted pair group method with arithmetic mean (UPGMA) with Dice’s distance matrices. The genetic diversity was high [percentage of polymorphic bands (P %) = 94.83%; Shannon’s diversity index (I) = 0.3175; Nei’s diversity (h) = 0.2034] at the population level, but low within populations [P % = 53.38%; Shannon’s diversity index (I) = 0.2734; Nei’s diversity (h) = 0.1811]. The mean coefficient of gene differentiation (Gst) was 0.165, indicating that 83.5% of the genetic diversity resided within the population. The genetic similarity values among 12 subpopulations ranged from 0.9672 to a minimum of 0.8641, and genetic distance values ranged from 0.1461 to 0.0333. Total gene flow (Nm) of 5.5810 indicated that there was significant gene flow among the sampled populations. Analysis of molecular variance (AMOVA) demonstrated that there was a relatively high level (81.93%) of genetic variation within subpopulations, with the gene differentiation coefficient (ΦST) being 0.181. A Mantel test suggested that there was no significant correlation between genetic differentiation and geographical distance.
Résumé
La diversité et la structure génétiques des populations de 72 isolats de Rhizoctonia solani (AG-1 IA) infectant le riz, collectés dans 12 comtés (sous-populations) des provinces du Guandong, du Guangxi et d’Hainan dans le sud de la Chine (populations), ont été étudiées à l’aide de 9 marqueurs ISSR (amplification intermicrosatellite). En tout, 116 bandes ont été amplifiées, dont la majorité des fragments mesurait de 500 bp à 2 500 bp, desquelles 110 (94.8 %) étaient polymorphes. En outre, 72 isolats ont été groupés en 6 principaux clusters dont le coefficient de similarité était de 73 % selon la méthode des moyennes par paire non pondérée, en concordance avec les matrices de distance de Dice. Le taux de diversité génétique était élevé à l’échelle de la population (pourcentage de bandes polymorphes [P %] = 94.83 %; indice de diversité de Shannon [I] = 0.3175; indice de diversité de Nei [h] = 0.2034), mais faible au sein des populations (P % = 53.38 %; indice de diversité de Shannon [I] = 0.2734; indice de diversité de Nei [h] = 0.1811). Le coefficient moyen de différenciation génétique (Gst) était de 0.165, indiquant que 83.5 % de la diversité génétique se trouvait au sein de la population. Les valeurs de similarité génétique chez 12 sous-populations variaient de 0.9672 à 0.8641, et celles de distance génétique, de 0.1461 à 0.0333. Le flux génique total (Nm) de 5.5810 indiquait que le flux génique parmi les populations échantillonnées était significatif. L’analyse de variance moléculaire (AMOVA) a démontré qu’il y avait un taux relativement élevé (81.93 %) de variation génétique au sein des sous-populations, avec un coefficient de différenciation génétique (ΦST) de 0.181. Un test de Mantel a suggéré qu’il n’y avait pas de corrélation significative entre la différenciation génétique et la distance géographique.
Introduction
Rice sheath blight (RSB), caused by the soilborne basidiomycetous fungus Rhizoctonia solani Kühn [teleomorph Thanatephorus cucumeris (A. B. Frank) Donk], is one of the most devastating fungal diseases of rice worldwide (Stodart et al. Citation2007; Taheri et al. Citation2007; Bernardes-de-Assis et al. Citation2009). In China, RSB affects c. 15–20 million ha of paddy-irrigated rice and causes a yield loss of about 6 million tons of rice grain per year. Furthermore, yield losses per growing season can reach up to 50% (Bernardes-de-Assis et al. Citation2009; Zheng et al. Citation2013).
RSB is difficult to control due to the low level of inherent resistance of rice against this disease (Anderson et al. Citation1972; Khodayari et al. Citation2009). Although partial genetic resistance to RSB has been reported and major genes responsible for resistance have been found (Pan et al. Citation1999), RSB is still not efficiently controlled by resistance breeding (Cubeta & Vilgalys Citation1997; Wu et al. Citation2003). The major management strategies include agricultural preventive treatments and the use of the antifungal antibiotics jinggangmycin or validamycin A, neither of which is highly effective (Jiang et al. Citation2012). Moreover, the application of fungicides can result in environmental concerns (Willocquet et al. Citation2000; Ciampi et al. Citation2008; Taheri & Tarighi Citation2011; González et al. Citation2012). Hence, development of resistant varieties as a means to control RSB is desirable, but greatly dependent on an understanding of the genetic diversity and population structure of the pathogen.
A method based on classification of isolates into anastomosis groups (AG) has been used for the identification and classification of isolates of R. solani (Liu & Sinclair Citation1992; Cubeta & Vilgalys Citation1997). Thus far, 14 AGs have been identified within the R. solani complex. Isolates of AG-1 have been further divided into three subgroups, i.e. IA, IB and IC (Ogoshi Citation1987). Among them, isolates of R. solani of AG-1 IA have been associated with sheath blight in rice (Wang et al. Citation2013). However, anastomosis groupings do not reflect genetic variation or taxonomic relationships within and between AGs. Sometimes, isolates within an AG are more similar than between different AGs, and genetically distinct subgroups exist within AGs (Wang et al. Citation2013). Therefore, problems associated with studying genetic diversity in R. solani can be best addressed by the use of DNA-based molecular techniques (Cubeta & Vilgalys Citation1997; Toda et al. Citation1999; Sharma et al. Citation2005).
Numerous genetic markers were developed to evaluate genetic variability and characterization of R. solani. These genetic markers included isoenzymes (Liu & Nickrent Citation1990; Neeraja et al. Citation2003), DNA base sequence complementary analysis (Cubeta & Vilgalys Citation1997; Ceresini et al. Citation2002), restriction fragment length polymorphisms (RFLP) (O’Brien Citation1994; Rosewich et al. Citation1999; Ceresini et al. Citation2002), random amplified polymorphic DNA (RAPD) (Duncan et al. Citation1993; Zhou et al. Citation2002; Dubey et al. Citation2012; Mirmajlessi et al. Citation2012; Wang et al. Citation2013), amplified fragment length polymorphism (AFLP) (Taheri et al. Citation2007), simple sequence repeats (SSR) (Bernardes-de-Assis et al. Citation2009) and inter-simple sequence repeats (ISSR) (Sharma et al. Citation2005; Guleria et al. Citation2007; Stodart et al. Citation2007; Khodayari et al. Citation2009; Dubey et al. Citation2012; Mirmajlessi et al. Citation2012).
ISSR markers were first developed in 1994, and can be used to rapidly differentiate closely related individuals. ISSR analysis employs a dominant inheritance marker and can generate a great number of highly informative and reproducible alleles. This technique involves amplification of genomic DNA segments between two identical SSR regions with a single primer 16–18 bp long composed of a repeated sequence anchored at the 3′ or 5′ end by 2–4 arbitrary nucleotides (Zietkiewicz et al. Citation1994; Wang et al. Citation2005). ISSR analysis could be highly variable within a species and has higher repeatability and stability than RAPD analysis in utilizing longer primers that allow more stringent annealing temperatures (Nan et al. Citation2003; Stodart et al. Citation2007). Moreover, ISSR is less expensive to conduct than AFLP analysis, and this technique has been widely applied to studies of genetic diversity, phylogeny and in plant breeding (Pradeep et al. Citation2002; Stodart et al. Citation2007; Dubey et al. Citation2012). Li et al. (Citation2010) used ISSR analysis to evaluate 100 isolates of R. solani from 10 counties in Fujian Province, China. The tested isolates could be classified into five groups and high genetic diversity and significant genetic variance were found among populations. Hu et al. (Citation2010) isolated 186 Rhizoctonia-like fungal strains in 28 counties of Guangxi Province and found that R. solani was the most virulent and common species. Recently, Wang et al. (Citation2013) reported considerable genetic variation among 180 isolates of R. solani AG-1 IA from six provinces in southern China, and that most genetic diversity was distributed within populations. The reasons for this distribution might include a high gene flow and low genetic differentiation among populations. Three virulence groups were identified, with moderately virulent isolates predominant in the R. solani population. Virulence has been a very useful feature for the characterization of diversity among strains of R. solani AG-1 IA. At least four different methods have been developed to evaluate the virulence of R. solani isolates on rice under glasshouse conditions (Wang et al. Citation2013).
In the present study, the ISSR technique was used to investigate genetic variation of R. solani AG-1 IA, the causal agent of rice sheath blight, collected from three provinces (populations) in south China. The objective of this study was to assess the population genetic structure and genetic differentiation level of R. solani AG-1 IA, and understand the genetic relationship and genetic variation patterns in different regions.
Materials and methods
Field sampling, fungal isolates and DNA extraction from R. solani AG-1 IA
A total of 72 isolates of R. solani AG-1 IA were collected from 12 counties (subpopulations) in three provinces (populations) [Guangdong (GD), Guangxi (GX) and Hainan (HN)]. These counties and provinces represent the important rice producing areas in south China and the details of the 72 isolates are given in and . One isolate of Colletotrichum gloeosporioides was used as an outgroup control.
Fig. 1 The distribution of the 72 isolates of Rhizoctonia solani AG-1 IA collected from Guangdong, Guangxi and Hainan provinces.
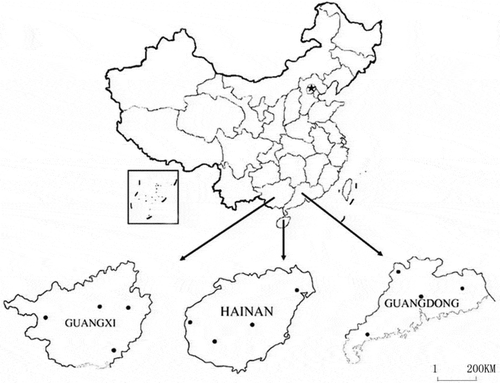
Table 1. Details of the 72 isolates of Rhizoctonia solani AG-1 IA from Guangdong (GD), Guangxi (GX) and Hainan (HN).
ISSR analysis
Nine ISSR markers were identified with 69 primers, synthesized by Beijing AuGCT DNA-SYN Biotechnology Co., Ltd, and used for PCR amplification (). Amplification reactions were performed in a 25 μL volume containing 2 μL of 10 × PCR buffer, 2 μL of 2.5 mm dNTP, 1 μL 0.5 μm of each primer, 0.2 μL Taq DNA polymerase (5 U μL−1) (TAKARA, Kyoto, Japan), 1 μL (8 ng) template DNA and 17.8 μL ddH2O.
Table 2. Sequences of arbitrary primers, annealing temperature, numbers of amplified and polymorphic bands resulting from ISSR analysis.
Table 3. Genotype diversity of Rhizoctonia solani AG-1 IA from the 12 subpopulations.
The optimized PCR analysis was performed using a VeritiTM 96 gradient thermal cycler (Applied Biosystems, CA, USA) with the following amplification conditions: initial denaturation at 94 °C for 4 min, followed by 38 cycles of denaturation at 94 °C for 60 s, appropriate annealing temperature for each primer () for 1 min and extension at 72 °C for 1 min. A final extension step at 72 °C for 4 min was included as a final step. PCR products were resolved with 1% (w/v) agarose gel electrophoresis at 120 V for 30 min in 1 × TAE (Tris-acetate-EDTA) buffer. The gels were stained with GoldviewTM (Dingguo, Shanghai, China) (10 mg mL−1), and photographed under UV light with Alpha Imager HP system (ProteinSimple, CA, USA). A DL 5000 bp DNA ladder was used as a molecular weight marker to determine ISSR profiles.
Data analysis
Amplified products, which were reproducible and consistent in performance, were scored for band presence (1) or absence (0) and a binary qualitative data matrix was constructed with UVP Lab Works. A cluster analysis of all isolates was carried out using the unweighted pair group method with arithmetic mean (UPGMA) and a dendrogram was generated by using Ntsys-PC 1.8 (Rohlf Citation1993). The percentage of polymorphic loci (P), Shannon’s diversity index (I) and Nei’s gene diversity (h) were used to estimate genetic variation using POPGENE ver. 1.32 (Yeh et al. Citation1997). Average genetic diversity within population (HS) and total genetic diversity among populations (HT) were also calculated. Population differentiation was analysed for polymorphism among populations by GST. Gene flow (Nm) was estimated from Nm = 0.5 (1–GST)/GST (Nei Citation1978).
POPGENE ver. 1.32 software (Yeh et al. Citation1997) was used to investigate the distribution of genetic differentiation in R. solani AG-1 IA. Genetic differentiation among populations was estimated by pairwise values of фST. Analysis of molecular variance was used to compare the partition of the genetic variation differing in geographical origin. AMOVA calculations were performed in WINAMOVA ver. 1.55. Mantel’s test was used for assessing the relationships between pairwise values of фST and the natural geographical distances among 12 subpopulations. The significance of the relationship was assessed with 9999 permutations.
Results
Genetic diversity
Sixty-nine primers were tested for their ability to produce polymorphic bands ranging from 500 bp to 2500 bp with a subset of the R. solani AG-1 IA isolates (). Nine of the primers were determined to produce bright, polymorphic and reproducible amplicons. For the 72 isolates, 116 scored bands were produced with an average of 12.22 markers per primer, of which 110 bands (94.83%) were polymorphic ().
Fig. 2 An example of the ISSR polymorphism of Rhizoctonia solani AG-1 IA isolates revealed by using ISSR primer EZ27. (Left to right: lane M, molecular weight marker DL5000 DNA ladder; lanes 1–16 represent the isolates).
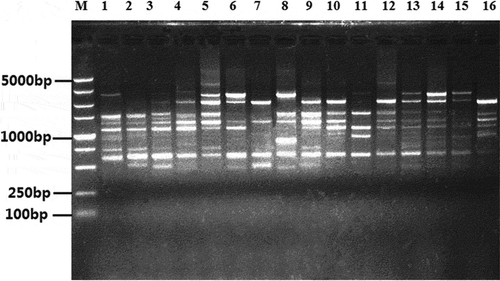
At the subpopulation level, Nei’s diversity (h) ranged from 0.1291 to 0.2248 with an average of 0.1811. Shannon’s diversity index (I) varied from 0.1985 to 0.3405 with an average of 0.2734. The percentage of polymorphic loci (P %) ranged from 41.38% (LZGD) to 66.38% (LZGX) with an average of 53.38%. At the population level, Nei’s diversity (h), Shannon’s diversity index (I) and percentage of polymorphic loci (P %) were 0.2034%, 0.3175% and 94.83%, respectively (). These findings demonstrated that genetic diversity among populations was higher than within populations.
For the 72 R. solani AG-1 IA isolates, Nei and Li’s similarity coefficient ranged from a high of 0.97 to a minimum of 0.72. Cluster analysis indicated that there was a genetically distinct variation among the 72 isolates. On the dendrogram, six main clusters (groups) were produced at 0.73 similarity coefficient (). These results indicated that high genetic variance occurred among the 72 isolates, and that isolates with different geographical origins assembled to different clusters with diverse similarity coefficients. The isolates from the Guangdong (GD) and Hainan (HN) populations mainly formed a distinct cluster. However, those from Guangxi (GX) population were grouped into five clusters, indicating that the GX population possesses the highest genetic differentiation. The genetic relationship between the GX and GD populations was relatively close, which may have resulted from gene exchange.
Genetic differentiation and gene flow
The total genetic diversity (Ht) was calculated to be 0.2372. Within population genetic diversity (Hs) ranged from 0.1665 (HN) to 0.2053 (GX) with an average of 0.1811 (). The mean of gene differentiation (Gst = 0.165) indicated that most of the genetic differentiation (83.5%) of R. solani AG-1 IA was found within populations. The total gene flow number (Nm) was 5.581, indicating that higher gene exchange has taken place between populations, and that genetic differentiation was mainly maintained within populations.
Table 4. Comparisons of genetic diversity and gene differentiation in three populations of Rhizoctonia solani AG-1 IA from south China.
Genetic structure of R. solani AG-1 IA populations
For the purpose of detecting the relationship among populations, among subpopulations and within subpopulations, molecular variance was examined using AMOVA (). The percentage of variance component found among populations was 9.14% (фST = 0.091, P < 0.002), while it was 8.92% (фST = 0.098, P < 0.002) among subpopulations and 81.93% (фST = 0.181, P < 0.002) within subpopulations. All P values were statistically significant, indicating that the geographical region was a significant factor affecting the genetic structure of these populations.
Table 5. Analysis of molecular variance (AMOVA).
To further investigate the genetic structure of R. solani AG-1 IA, the genetic similarity values and genetic distance of R. solani AG-1 IA isolates from 12 subpopulations were compared. The results showed that the genetic similarity coefficient ranged from 0.9672 (between subpopulations of LZGX and BSGX) to 0.8641 (between subpopulations of LZGD and LZGX), and the counterparts of genetic distance ranged from 0.1461 to 0.0333 (S1). These results demonstrated that there was a high degree of similarity among subpopulations. A UPGMA dendrogram showed a major division between the Guangdong population and all others () based on Nei’s genetic distance. Within the second group, subpopulations from Hainan and Guangxi provinces clustered together. The Guangxi population showed a closer genetic relationship to the Hainan population than to the Guangdong population. The LZGX and BSGX subpopulations clustered into one sub-group and appeared to be closer to each other.
Fig. 4 UPGMA dendrogram of the genetic relationships among 12 subpopulations of Rhizoctonia solani AG-1 IA inferred from a matrix of Nei’s genetic distances. The scale bar at the bottom represents the genetic distance.
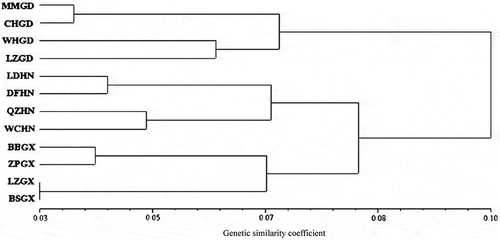
To test the relationships among subpopulations and distance, a matrix was constructed based on the pairwise ΦST distance among subpopulations (S2). The genetic differentiation coefficient (ΦST) ranged from −0.0070 to 0.3089, and the highest pairwise ΦST was between the LZGD and ZPGX subpopulations, indicating that these possessed the greatest genetic differentiation. Mantel tests were used to calculate the relationship between the matrices of geographical distance and pairwise ΦST coefficients with the MXCOMP program (). The results showed that the data points were distributed without distinct regularity, suggesting that there was no significant correlation between genetic differentiation and geographical distance in three populations of R. solani AG-1 IA from south China. The phylogenetic relationships of the 12 subpopulations in this study did not meet the model of isolation by distance.
Discussion
The results from this study clearly demonstrated that high genetic diversity exists among populations of R. solani AG-1 IA, a finding that is consistent with the results of previous reports using the ISSR technique (Guleria et al. Citation2007; Khodayari et al. Citation2009; Dubey et al. Citation2012; González et al. Citation2012). Cluster analysis showed that most isolates from the Guangdong and Hainan populations belonged to the same group, whereas the isolates from the Guangxi population were distributed into four groups. These findings indicated that clusters were significantly correlated with the geographical origin of the isolates. Some isolates from BSGX and LZGX were clustered in three different clades, and the percentage of polymorphic loci of these subpopulations was very high (66.38 and 63.79, respectively), indicating that the isolates from BSGX and LZGX possessed higher genetic diversity. Similar result was also described in studies of populations of R. solani AG-1 IA from Jiangsu Province (Wu et al. Citation2013).
The genetic structure of a population can be affected by numerous evolutionary factors including mating system, gene flow, seed dispersal, mode of reproduction and natural selection (Cubeta & Vilgalys Citation1997; Kar et al. Citation2010). A gene flow (Nm) value greater than 1.0 is considered necessary to prevent divergence resulting from genetic drift (Wright Citation1950). The total gene flow value of 5.5810 found in this study suggests that gene flow among the south China R. solani populations is very high. The exchange of genes among populations is known to homogenize allele frequencies among populations and determines the relative effect of selection and genetic drift (Kar et al. Citation2010). Genetic differentiation becomes non-significant because more gene exchange triggers more genetic similarity. The gene flow value (Nm) was low within populations, however, and the genetic variance became significant within populations, which was confirmed by the rejection of the geographical isolation by distance model in the present study.
The high genetic variability observed in the R. solani AG-1 IA population could be regarded as an adaptive strategy for increasing the population fitness from different geographical environments, climate and co-evolution with hosts (González et al. Citation2012). Twenty-two isolates from Guangdong population and 22 isolates from Hainan population were clustered into two distinct groups, which may reflect the exchange of genetic information only within the population. The higher variation observed in the Guangxi population might be a result of the frequent change of rice germplasm in breeding programmes and the host selection pressure on the pathogen.
Acknowledgements
This work was supported by the Special Fund for Agro-scientific Research in the Public Interest of the People’s Republic of China [grant number 201403075].
References
- Anderson FM, Datta N, Shaw EJ. 1972. R factors in hospital infection. Brit Med J. 3:82–85. doi:10.1136/bmj.3.5818.82
- Bernardes-de-Assis J, Storari M, Zala M, Wang W, Jiang D, ShiDong L, Jin MS, McDonald BA, Ceresini PC. 2009. Genetic structure of populations of the rice-infecting pathogen Rhizoctonia solani AG-1 IA from China. Phytopathology. 99:1090–1099. doi:10.1094/PHYTO-99-9-1090
- Ceresini PC, Shew HD, Vilgalys RJ, Rosewich UL, Cubeta MA. 2002. Genetic structure of populations of Rhizoctonia solani AG-3 on potato in eastern North Carolina. Mycologia. 94:450–460. doi:10.2307/3761779
- Ciampi S, Böcking T, Kilian KA, Harper JB, Gooding JJ. 2008. Click chemistry in mesoporous materials: functionalization of porous silicon rugate filters. Langmuir. 24:5888–5892. doi:10.1021/la800435d
- Cubeta MA, Vilgalys R. 1997. Population biology of the Rhizoctonia solani complex. Phytopathology. 87:480–484. doi:10.1094/PHYTO.1997.87.4.480
- Dubey SC, Tripathi A, Upadhyay BK. 2012. Molecular diversity analysis of Rhizoctonia solani isolates infecting various pulse crops in different agro-ecological regions of India. Folia Microbiol. 57:513–524. doi:10.1007/s12223-012-0165-y
- Duncan S, Barton JE, O’Brien PA. 1993. Analysis of variation in isolates of Rhizoctonia solani by random amplified polymorphic DNA assay. Mycol Res. 97:1075–1082. doi:10.1016/S0953-7562(09)80508-X
- González N, Godoy-lutz G, Steadman J, Higgins R, Eskridge K. 2012. Assessing genetic diversity in the web blight pathogen Thanatephorus cucumeris (anamorph = Rhizoctonia solani) subgroups AG-1-IE and AG-1-IF with molecular markers. J Gen Plant Pathol. 78:85–98. doi:10.1007/s10327-012-0361-2
- Guleria S, Aggarwal R, Thind TS, Sharma TR. 2007. Morphological and pathological variability in rice isolates of Rhizoctonia solani and molecular analysis of their genetic variability. J Phytopathol. 155:654–661. doi:10.1111/j.1439-0434.2007.01291.x
- Hu C-J, Wei Y-W, Huang S-L, Shi G-Y, Li Y-R. 2010. Identification and characterization of fungal strains involved in rice sheath blight complex in Guangxi province. Acta Agricul Boreali Occident Sin. 19:45–51.
- Jiang L-B, Zhao K-F, Wang D-J, Wu J-C. 2012. Effects of different treatment methods of the fungicide jinggangmycin on reproduction and vitellogenin gene (Nlvg) expression in the brown planthopper Nilaparvata lugens Stål (Hemiptera: Delphacidae). Pestic Biochem Physiol. 102:51–55. doi:10.1016/j.pestbp.2011.10.009
- Kar PK, Srivastava AK, Sinha MK, Sinha AK, Prasad BC. 2010. Conservation genetics, combination of molecular biology and ecology: tropical tasar silkworm as an example. Bioscan. 3:627–634.
- Khodayari M, Safaie N, Shamsbakhsh M. 2009. Genetic diversity of Iranian AG1-IA isolates of Rhizoctonia solani, the cause of rice sheath blight, using morphological and molecular markers. J Phytopathol. 157:708–714. doi:10.1111/j.1439-0434.2009.01541.x
- Li T-D, Peng S-W, Wang Z-H, Lu G-D. 2010. Genetic diversiy analysis of Rhizoctonia solani populations from Fujian using intersimple sequences repeats (ISSR) technique. Acta Phytopathol Sin. 40:186–194.
- Liu ZL, Nickrent DL. 1990. Genetic relationships among isolates of Rhizoctonia solani anastomosis group-2 based on isozyme analysis. Can J Plant Pathol. 12:376–382. doi:10.1080/07060669009500977
- Liu ZL, Sinclair JB. 1992. Genetic diversity of Rhizoctonia solani anastomosis group 2. Phytopathology. 82:778–787. doi:10.1094/Phyto-82-778
- Mirmajlessi SM, Safaie N, Mostafavi HA, Mansouripour SM, Mahmoudy SB. 2012. Genetic diversity among crown and root rot isolates of Rhizoctonia solani isolated from cucurbits using PCR based techniques ISSR. Afr J Agric Res. 7:583–590.
- Nan P, Shi SH, Peng SL, Tian CJ, Zhong Y. 2003. Genetic diversity in Primula obconica (Primulaceae) from central and southwest China as revealed by ISSR markers. Ann Bot. 91:329–333. doi:10.1093/aob/mcg018
- Neeraja CN, Shenoy VV, Reddy CS, Sarma NP. 2003. Isozyme polymorphism and virulence of Indian isolates of the rice sheath blight fungus. Mycopathologia. 156:101–108. doi:10.1023/A:1022988710654
- Nei M. 1978. Estimation of average heterozygosity and genetic distance from a small number of individuals. Genetics. 89:583–590.
- O’Brien PA. 1994. Molecular markers in Australian isolates of Rhizoctonia solani. Mycol Res. 98:665–671. doi:10.1016/S0953-7562(09)80414-0
- Ogoshi A. 1987. Ecology and pathogenicity of anastomosis and intraspecific groups of Rhizoctonia Solani Kuhn. Annu Rev Phytopathol. 25:125–143. doi:10.1146/annurev.py.25.090187.001013
- Pan XB, Rush MC, Sha XY, Xie QJ, Linscombe SD, Stetina SR, Oard JH. 1999. Major gene, nonallelic sheath blight resistance from the rice cultivars Jasmine 85 and Teqing. Crop Sci. 39:338–346.
- Pradeep Reddy M, Sarla N, Siddiq EA. 2002. Inter simple sequence repeat (ISSR) polymorphism and its application in plant breeding. Euphytica. 128:9–17. doi:10.1023/A:1020691618797
- Rohlf FJ. 1993. NTSYS-pc: numerical taxonomy and multivariate analysis system version 1.80. Setauket, NY: Exeter Biological Software.
- Rosewich UL, Pettway RE, Mcdonald BA, Kistler HC. 1999. High levels of gene flow and heterozygote excess characterize Rhizoctonia solani AG-1 IA (Thanatephorus cucumeris) from Texas. Fungal Genet Biol. 28:148–159. doi:10.1006/fgbi.1999.1174
- Sharma M, Gupta SK, Sharma TR. 2005. Characterization of variability in Rhizoctonia solani by using morphological and molecular markers. J Phytopathol. 153:449–456. doi:10.1111/j.1439-0434.2005.01000.x
- Stodart BJ, Harvey PR, Neate SM, Melanson DL, Scott ES. 2007. Genetic variation and pathogenicity of anastomosis group 2 isolates of Rhizoctonia solani in Australia. Mycol Res. 111:891–900. doi:10.1016/j.mycres.2007.05.008
- Taheri P, Gnanamanickam S, Höfte M. 2007. Characterization, genetic structure, and pathogenicity of Rhizoctonia spp. associated with rice sheath diseases in India. Phytopathology. 97:373–383. doi:10.1094/PHYTO-97-3-0373
- Taheri P, Tarighi S. 2011. Cytomolecular aspects of rice sheath blight caused by Rhizoctonia solani. Eur J Plant Pathol. 129:511–528. doi:10.1007/s10658-010-9725-7
- Toda T, Hyakumachi M, Arora DK. 1999. Genetic relatedness among and within different Rhizoctonia solani anastomosis groups as assessed by RAPD, ERIC and REP-PCR. Microbiol Res. 154:247–258. doi:10.1016/S0944-5013(99)80022-6
- Wang L, Liu LM, Wang ZG, Huang SW. 2013. Genetic structure and aggressiveness of Rhizoctonia solani AG 1-IA, the cause of sheath blight of rice in southern China. J Phytopathol. 161:753–762. doi:10.1111/jph.12127
- Wang SB, Miao XX, Zhao WG, Huang B, Fan MZ, Li ZZ, Huang YP. 2005. Genetic diversity and population structure among strains of the entomopathogenic fungus, Beauveria bassiana, as revealed by inter-simple sequence repeats (ISSR). Mycol Res. 109:1364–1372. doi:10.1017/S0953756205003709
- Willocquet L, Fernandez L, Savary S. 2000. Effect of various crop establishment methods practised by Asian farmers on epidemics of rice sheath blight caused by Rhizoctonia solani. Plant Pathol. 49:346–354. doi:10.1046/j.1365-3059.2000.00454.x
- Wright S. 1950. Genetical structure of populations. Nature. 166:247–249. doi:10.1038/166247a0
- Wu H-F, Wang X-Y, Luo C-Q, Chen Z-Y. 2013. Genetic diversity and pathogenicity of Rhizoctonia solani isolates from Jiangsu province. Jiangsu J Agric Sci. 29:51–59.
- Wu Q, Zhang W, Pwee KH, Kumar PP. 2003. Cloning and characterization of rice HMGB1 gene. Gene. 312:103–109. doi:10.1016/S0378-1119(03)00605-X
- Yeh FC, Yang RC, Boyle TBJ, Ye ZH, Mao JX. 1997. POPGENE, the user-friendly shareware for population genetic analysis [M]. Calgary, Canada: Molecular Biology and Biotechnology Centre, University of Alberta.
- Zheng A, Lin R, Zhang D, Qin P, Xu L, Ai P, Ding L, Wang Y, Chen Y, Liu Y, et al. 2013. The evolution and pathogenic mechanisms of the rice sheath blight pathogen. Nat Commun. 4:1424. doi:10.1038/ncomms2427
- Zhou E-X, Cao J-X, Yang M, Zhu X-R. 2002. Studies on the genetic diversity of Rhizoctonia solani AG-1-IA from six provinces in the southern China. J Nanjing Agric Univ. 25:36–40.
- Zietkiewicz E, Rafalski A, Labuda D. 1994. Genome fingerprinting by simple sequence repeat (SSR)-anchored polymerase chain reaction amplification. Genomics. 20:176–183. doi:10.1006/geno.1994.1151