Abstract
This study integrated defence-related gene profiling with histological and histochemical observations to develop a detailed time-course description of Puccinia striiformis penetration and infection of susceptible ‘Fielder’ wheat and resistant ‘Moro’ that contains the Yr10 gene for stripe rust resistance. Penetration and establishment events of the pathogen within the leaf were very similar in both ‘Fielder’ and ‘Moro’ until approximately 8–12 days after inoculation (dai). Two oxidative bursts, one at 6 dai and a second at 14 dai, were recorded. Early mild up-regulation of the defence signalling genes associated with jasmonic acid pathway EDS1, PAD4 and AOS were observed as early as 4 days after inoculation (dai) in ‘Moro’ compared with ‘Fielder’. Transcripts of PR1.1, a gene associated with the salicylic acid pathway, and of a thaumatin-like protein, were differentially up-regulated from 6 to 12 dai in ‘Moro’ compared with ‘Fielder’. A toxic response in fungal tissues was observed coincident with the hypersensitive response (HR) in ‘Moro’ that terminated further growth of the pathogen. The anatomical and cytological aspects of defence expression are discussed in relation to profiling of different defence genes.
Résumé
Cette étude a intégré le profilage des gènes impliqués dans les mécanismes de défense aux observations histochimiques afin d’élaborer une description chronologique détaillée de la pénétration et de l’infection par Puccinia striiformis chez le cultivar de blé susceptible ‘Fielder’ et le cultivar résistant ‘Moro’ qui possède le gène de résistance Yr10 contre la rouille jaune. Les processus de pénétration et d’établissement de l’agent pathogène dans la feuille étaient presque en tout point semblables chez les deux cultivars, et ce, jusqu’à environ 8 à 12 jours après inoculation (jai). Deux poussées oxydatives ont été enregistrées : une première à 6 jai et une seconde à 14 jai. Une faible et précoce régulation positive des gènes de signalisation liés à la défense et associée à la voie de l’acide jasmonique, EDS1, PAD4 et AOS, a été observée dès le quatrième jour après inoculation chez le cultivar ‘Moro’, comparativement au cultivar ‘Fielder’. Transcrits de PR1.1, une protéine impliquée dans la pathogenèse, associée à la voie de l’acide salicylique, et d’une protéine semblable à la thaumatine ont été différentiellement régulées positivement de 6 à 12 jai chez le cultivar ‘Moro’, comparativement au cultivar ‘Fielder’. Une réaction toxique a été observée dans les tissus fongiques, en étroite concordance avec la réaction d’hypersensibilité chez le cultivar ‘Moro’, qui a inhibé toute croissance additionnelle de l’agent pathogène. Les aspects anatomiques et cytologiques de l’expression de la défense sont discutés en fonction du profilage de différents gènes de défense.
Introduction
Stripe rust of wheat, caused by Puccinia striiformis Westend. f. sp. tritici Erikss., is one of the most destructive diseases of wheat (Triticum aestivum L.) in the world (Chen Citation2005) and severe epidemics have frequently caused extensive yield losses. Since 1999, disease incidence and severity have increased dramatically in North America due to the occurrence of new, high temperature-adapted races and their subsequent spread (Chen Citation2005). Although fungicides can reduce losses caused by stripe rust, growing resistant cultivars is the most effective, economical and environmentally sustainable method of disease control (Line & Chen Citation1995). A wide range of disease resistance genes have been identified and deployed to control stripe rust (Chen Citation2005). Two major forms of resistance have been deployed – seedling or all stage resistance (R-gene mediated resistance) and adult plant resistance (APR) (Chen Citation2005). The wheat cultivar ‘Moro’ (CI 013740) possesses the all stage resistance gene Yr10 situated on the short arm of chromosome 1B (Chen Citation2005). A second uncharacterized gene in ‘Moro’, identified as YrMor that has a minor effect on resistance to stripe rust, is located on chromosome 4B (Chen et al. Citation1995). Races that overcome Yr10 have been reported in the Pacific Northwest of the USA and western Canada (Chen et al. Citation2009; Puchalski & Gaudet Citation2011).
Current models of host-parasite interactions in plants define two types of immunity (resistance): Pathogen Associated Molecular Patterns (PAMP) Triggered Immunity (PTI), and Effector Triggered Immunity (ETI) (Jones & Dangl Citation2006). PTI (also innate, basal or non-host immunity) is triggered following detection of PAMPs by plant recognition receptors (PRRs) and generally does not involve the hypersensitive response (HR). Adapted pathogens deliver effectors that interfere with PTI and permit dispersal within the plant. Plants have evolved ETI whereby specific receptors recognize specific effectors and trigger immunity, which most often involves HR. This model adapts the classical ‘gene-for-gene’ concept put forward by Flor (1973) that states that for every resistance (R) gene in the host, there is a corresponding interacting avirulence (Avr) gene in the pathogen in the following manner: the product of the R-gene is the NBS-LRR receptor and the product of the Avr gene is the specific pathogen effector recognized by the R-gene (Jones & Dangl Citation2006; Bent & Mackey Citation2007). Several R-gene associated-receptors have been characterized in dicots and monocots with the CC-NBS-LRR (Coiled Coil-Nucleotide Binding Site-Leucine Rich Repeats) class of receptors being the most common in monocots (Tarr & Alexander Citation2009). We have reported previously that Yr10 gene is a unique CC-NBS-LRR receptor in ‘Moro’ wheat (Laroche et al. Citation2005).
The oxidative burst is among the earliest resistance reactions in plant host-parasite interactions and occurs in two phases (Lamb & Dixon Citation1997); phase 1 is non-specific, and is activated in both compatible and incompatible interactions, whereas phase 2 is specific, activated only in incompatible interactions by avirulent races of adapted pathogens in host interactions and non-adapted pathogens in non-host interactions, and is always associated with the HR (Lamb & Dixon Citation1997; Nurnberger et al. Citation2004). Reactive oxygen species including superoxide anion (O2‒), hydrogen peroxide (H2O2), hydroxyl radical (OH‒) and singlet oxygen (1O2) are toxic intermediates originating from partial reduction of oxygen (O2). Another early plant response to pathogen invasion is autofluorescence (Lamb & Dixon Citation1997), which has been associated with the accumulation of phenolic compounds and cytoskeleton destruction (Yu et al. Citation2001).
The ‘oxidative burst’ has been recognized as a primary process of plant defence response to pathogen challenge (Alvarez et al. Citation1998) and plays an important role in the induction of defence responses, directs antimicrobial action of peroxides (Peng & Kuc Citation1992), and functions as secondary messengers for activating defence response genes, phenolics and phytoalexins (Jabs et al. Citation1997; Chamnongpol et al. Citation1998), promotes cross-linking of lignin precursors and cell wall proteins (Lamb & Dixon Citation1997), and triggers HR (Bestwick et al. Citation2001). HR may also be activated following interaction of NO with H2O2 (Delledonne et al. Citation2002). Following the oxidative burst, the phytohormones salicylic acid (SA), jasmonic acid (JA) and ethylene (ET) induce up-regulation of pathogenesis-related (PR) and related proteins (Van Loon et al. Citation2006). These plant hormones have major roles in the regulation of defence signalling networks involved in host-parasite interactions in plants (Jones & Dangl Citation2006; Bent & Mackey Citation2007). SA is involved in defence signalling in resistance reactions involving biotrophic pathogens while JA/ET is induced when necrotrophic plant pathogens are involved (Grant & Jones Citation2009). Enhanced Disease Susceptibility 1 (EDS1) and Phytoalexin Deficient 4 (PAD4) act upstream of SA and have been implicated in both basal immunity and NBS-LRR R-gene resistance (Wiermer et al. Citation2005). SA has been implicated in signalling defence responses in some monocots including wheat (Anand et al. 2007) and many of the defence pathways are similar in both monocots and dicots (Vlot et al. Citation2009). Defence responses involve the up-regulation of phenylalanine ammonium lyase (PAL) pathway leading to production of phytoalexins such as camalexin, the production of the plant hydrolases chitinase and ß-1, 3-glucanase, deposition of cell wall reinforcing materials, and the synthesis of a wide range of PR-proteins (Veronese et al. Citation2003). Currently there are more than 14 classes of PR-proteins (Van Loon et al. Citation2006).
In this study, microscopy and histochemical tests for determination of the HR and ROS were used to compare the compatible and incompatible interaction involving P. striiformis races in ‘Moro’ containing the resistance gene Yr10 (Chen & Line Citation1992; Chen et al. Citation1995) and the compatible interaction involving the same races on ‘Fielder’. Additionally, quantitative real-time polymerase chain reaction (qRT-PCR) was used to study the expression profiles of a series of defence-related SA- and JA-specific indicator genes. By linking morphological responses to regulation of gene expression, we were able to develop an integrated, time-course portrait of the host-parasite interaction of P. striiformis in susceptible ‘Fielder’ and resistant ‘Moro’. The results of these studies will permit the future identification of key regulatory factors that are precisely linked to specific stages of the resistance response in wheat to P. striiformis.
Materials and methods
Plant growth and inoculation conditions
The wheat (Triticum aestivum L.) cultivars ‘Moro’ and ‘Fielder’, and isolates 44E14 (SRC-84) and CDL-29 of P. striiformis f. sp. tritici, were used in the experiments. ‘Moro’ possesses the resistance gene Yr10 and responds with HR-resistance to inoculation with SRC-84 whereas it is susceptible to CDL-29 (Chen & Line Citation1993; Su et al. Citation2003). ‘Fielder’, historically a widely grown cultivar of soft white spring wheat, is susceptible to the predominant stripe rust races western Canada (Su et al. Citation2003) including isolates CDL-29 and SRC-84.
Wheat seeds were germinated and grown in a soil-less potting mix in 10-cm diameter plastic pots in a growth cabinet set at 16 h photoperiod (250 µmol m−2s−1 photon flux density) and 20 °C/18 °C day/night with 60% relative humidity. Stripe rust urediniospores were suspended in Solitrol mineral oil, the concentration adjusted to 2.5–3 × 105 spores mL−1, and then sprayed onto the leaves of the seedlings at the 2–3 leaf stage (12–13 days after sowing). Control plants were mock-inoculated only with Solitrol mineral oil. Treated plants were later sprayed with a fine mist of sterile water (with 0.01% Tween 20), sealed in plastic bags to maintain 100% RH, and incubated in the dark at 10 °C for 2 days. The bags were subsequently removed and plants were returned to the original growth cabinet at 15 °C. Following inoculation, wheat leaf samples were collected at 0, 1, 2, 4, 6, 8, 10, 12, 14 and 16 days after inoculation (dai) for the different studies described below.
Disease evaluation
Necrosis and chlorosis were visible at 8–10 dai and pustules were visible at about 14 dai. Plants were rated for infection type at 14–20 dai when uredinia were developing on ‘Fielder’ based on the 0–9 infection type scale according to Chen & Line (Citation1993): 0 = no visible signs or symptoms; 1 = necrotic or chlorotic flecks with no sporulation; 2 = necrotic and/or chlorotic blotches or stripes with no sporulation; 3 = necrotic and/or chlorotic blotches or stripes with only a trace of sporulation; 4, 5 and 6 = necrotic and/or chlorotic blotches or stripes with light, intermediate and moderate sporulation, respectively; and 7, 8 and 9 = abundant sporulation with necrotic and/or chlorotic blotches or stripes, chlorosis behind the sporulation area, and no chlorosis or necrosis, respectively. Individual pustules on seedlings and stripes on adult plants were recorded. On the 0–9 scale, plants with infection types 1–3 would be considered very resistant to moderately resistant, plants with infection types 4–6 would have intermediate resistance, and plants with infection types 7–9 would be moderately susceptible to very susceptible. Ranges in infection types on the same plant were designated by a dash (-). Data on rust intensity were based on the modified Cobb’s scale for cereal rusts (Peterson et al. Citation1948).
Histological studies
Leaf discs were obtained using a 5 mm cork-borer and stained with lactophenol-trypan blue (10 mL lactic acid, 10 mL glycerol, 10 g phenol, 10 mg trypan blue, dissolved in 10 mL distilled water) (Keogh et al. Citation1980). They were boiled for 2 min in the staining solution, cleared in boiling 95% ethanol 10 min, and stored in 50% glycerol. Data were collected from 5 leaf discs sampled from each of four plants per treatment. Additionally, leaf sections were fixed in formalin-acetic acid-ethanol (FAA), embedded in paraffin and stained with Pianese IIIb stain or with the triple stain (Johansen Citation1940). Leaf sections were examined with a Zeiss microscope (Zeiss Corporation, West Germany). For fluorescence microscopy, 330–385 nm and 460–490 nm excitation and emission filters, respectively, and a HBO103W/2 light source were used. For confocal microscopy, 1 cm leaf sections were placed in absolute ethanol until the chlorophyll was removed. The leaves were then rehydrated and stained with aniline blue or cotton blue, mounted in AquaMount (Polysciences, Inc., Warrington, PA, USA) and dried overnight. Slides were viewed with a Zeiss Axiophot LSM 410 confocal microscope using 488 Argon, 543 and 633 HeNe lasers. The material was optically z-sectioned and the resulting sequence of images was processed to form a 3D image. For transmission electron microscopy (TEM), small segments of leaves were fixed in 2% glutaraldehyde in 0.01 m phosphate buffer, pH 7.2, postfixed in 1% osmium tetroxide in phosphate buffer, pH 7.2, stained with 2% uranyl acetate in water, dehydrated, infiltrated and embedded in Spurr’s resin. Blocks were sectioned using a MT 7000 RMC microtome. These were stained with 2% uranyl acetate followed by lead citrate (Venable & Coggeshall Citation1965). The sections were viewed using a Hitachi H-7100 transmission electron microscope.
Histochemical localization of H2O2
Hydrogen peroxide was detected in inoculated leaves using the 3,3’- diaminobenzidine (DAB, 1 mg mL−1, Vector Laboratories, Inc., Burlingame, CA) uptake method (Thordal-Christensen et al. Citation1997). Leaf discs of 5 mm diameter made with a cork-borer from detached leaves were placed in a solution of 5 mL distilled water, 2 drops of Buffer Stock Solution and 4 drops of DAB Stock Solution according to the manufacturer’s instructions, incubated in the growth cabinet for 10 h prior to sampling to allow DAB reaction with H2O2 and peroxidase. Sections were washed 3 times by distilled water, fixed and decolourized in fixative (3 : 1 : 1 ethanol/latic acid/glycerol) for 3 days, and stored in 50% glycerol. H2O2 is visualized as a reddish-brown colouration. The numbers of DAB stained sites with associated mesophyll tissue regions were microscopically quantified from 20 leaf discs originating from four plants (reps) per treatment. Differences between treatments were established using analysis of variance and standard error values were determined for each mean. Studies were repeated once and results were combined over the two repeats.
RNA isolation and gene expression profiling
Samples for microscopy and gene profiling were harvested from the same plants to ensure consistency of experimental conditions. Leaf tissues were sampled every 2 days from 0 to 14 dai, flash frozen in liquid nitrogen and stored at −80 °C until used. Prior to isolation of RNA from leaf tissues, penetration and spread of the fungus and indication of HR and DAB staining were verified microscopically. Total RNA was extracted from about 200 mg of frozen wheat leaf samples using RNeasy Plant Mini Kit (QIAGEN, CA, USA) and residual DNA was removed using RNase-free DNase I (QIAGEN) according to the manufacturer’s instructions. The RNA concentration in each sample was measured using a DU 800 Spectrophotometer (Beckman Coulter, USA). Only RNA samples with 260/280 ratio between 1.8 and 2.0 and 260/230 ratio greater than 2.0 were used for the analysis. The integrity of RNA samples was further assessed by agarose gel electrophoresis. First strand cDNA was synthesized by using SuperScriptTM III M-MLV reverse transcriptase kit (Invitrogen, CA, USA). cDNA constructions were performed according to the manufacturers’ instructions. Total RNA (4 µg) was incubated in the presence of 50 pmol oligo (dT)20 and sterile ddH2O in 12 µL total volume at 65 °C for 5 min and cooled in ice. The synthesis was performed in 1× first strand buffer, 0.1 µmol DTT, 40 U RNaseOut (Invitrogen), 10 nmol of each dNTP and 200 U SuperScriptTM III Reverse Transcriptase in 20 µL total volume, incubated for 50 min at 45 °C. Synthesis reactions were terminated by 15 min incubation at 70 °C.
Primer pairs for qRT-PCR analyses were designed using Primer 3 software (Rozen & Skaletsky Citation2000). Sequences and general guidelines for primer design were based on the recommendations provided by QIAGEN. These primers were designed from wheat PR-protein nucleotide sequences deposited in NCBI GenBank. The gene specific primers used, their melting temperature (Tm), and the expected size of amplified fragments in base pair (bp), are listed in . Target genes were selected in order to study the expression of generalized defence responses in both incompatible and compatible interactions.
Table 1. DNA primers used to measure expression of defence-related genes using qRT-PCR.
qRT-PCR analyses were conducted in a iCycler iQ (BIO-RAD, USA) using QuantiTect SYBR Green PCR Kit (QIAGEN) as the fluorescence marker for quantitative detection of individual genes according to manufacturer’s instructions. qRT-PCR reaction mixtures contained 10 μL SYBR Green mix, 0.5 μL fluorescin (0.5 µm), 6 pm of each primer, 5 μL template (2 × diluted cDNA from leaf samples) and sterile distilled water to a total reaction volume of 20 μL. To standardize the data, the amount of target gene was normalized over the abundance of a constitutive wheat translation elongation factor 1 α-subunit (TEF1) and contig 5, a carbohydrate transmembrane transporter (). Thermal conditions were 95 °C for 13 min followed by 45 cycles of 95 °C for 30 s, 56 °C for 30 s and 72 °C for 30 s, then hold at 4 °C. Reactions for inoculated and mock-inoculated plants at the above-mentioned time points, as well as three negative controls, were performed. To detect primer dimerisation and other artefacts of amplification, a melting-curve analysis was performed immediately after completion of the qRT-PCR by increasing sample temperatures from 40 °C to 95 °C at a +0.5 °C per 10 second period with continuous measurement of fluorescence.
qRT-PCR results were analysed with the REST software (Pfaffl et al. Citation2002). Based on the data of two reference genes, TEF1 and contig 5, REST was used to analyse the fold differences in target gene expression between the control and the treated samples. Triple biological replicates for each sample were used for real-time PCR analysis and triple technical replicates were analysed for each biological replicate. The experiment was repeated twice using newly extracted RNA and synthesized cDNA samples each time, and representative results are presented.
Results
Symptoms and rust development
Initial symptoms following inoculation with strain SRC-84 consisted of chlorotic spots that were similar on both ‘Moro’ and ‘Fielder’ at 10 dai (). At 12 dai, chlorotic spots were more pronounced in ‘Moro’ and they became enlarged and coalesced from 12 to 16 dai, and necrosis developed at 16 dai (). No sporulation was observed in ‘Moro’ even after 24 dai. In ‘Fielder’ inoculated with SRC-84 and CDL-29, and in ‘Moro’ inoculated with CDL-29, yellow pustules were observed at 14–16 dai and became fully developed after 16 dai. Using infection type data (IT) recorded at 20 dai based on 0–9 scale following inoculation with SRC-84, ‘Moro’ was rated as resistant (IT = 2.8, SE ± 0.2) and ‘Fielder’ highly susceptible (IT = 8.3, SE ± 0.2). Percentage of leaf area covered with rust increased from 62% at 14 dai to 79% at 20 dai on ‘Fielder’. Upon infection with fungal strain CDL-29, both ‘Fielder’ and ‘Moro’ were similarly highly susceptible (data not shown).
Fig. 1 (Colour online) Symptoms and infection types at different plant growth stages in resistant ‘Moro’ and susceptible ‘Fielder’ after inoculation with P. striiformis strain SRC-84. Infection type is based on a scale of 0–9. M, ‘Moro’; F, ‘Fielder’; numbers at the bottom indicate infection type while those at the top reflect number of days after inoculation.
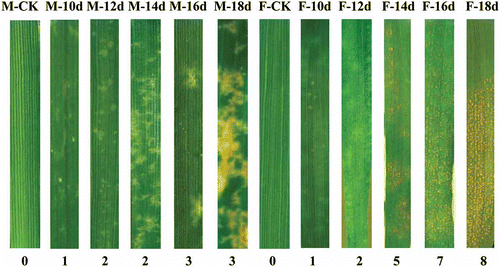
Time-course cytological observations
Percentage germination of P. striiformis urediniospores increased from 24.4% to 34.2% at 1 dai to 73.5–77.2% at 6 dai and was not statistically different between ‘Moro’ and ‘Fielder’ (P > 0.05) (). This germination spread over numerous days complicated the interpretation of infection by stripe rust in wheat. Consequently, a range of days at which different morphological events were observed is presented. After 6 dai, spores on the leaf surface had mostly disappeared. Pre-penetration and early post-penetration events including percent formation of substomatal vesicles from P. striiformis intercellular hyphae were not different in both ‘Fielder’ and ‘Moro’ from 3 to 10 dai (P > 0.05) (,) (). P. striiformis infection hyphae growth in both ‘Moro’ and ‘Fielder’ continued until approximately 8–10 dai after which hyphae ramified surrounding mesophyll cells, frequently encircling them (, , f). HR was observed among cells at 10 and 12 dai () and coincided with dark staining of intercellular hyphae, amorphous appearance of cell contents and cell collapse with darkly staining cell contents in light microscopy sections at 12–16 dai ().
Fig. 2 (Colour online) Intercellular mycelium proliferation of P. striiformis in ‘Fielder’ (a, c and e) and ‘Moro’ (b, d, and f) leading to compatible and incompatible interactions, respectively. (a, b) Similar development of intercellular hyphae (ih) in both ‘Fielder’ and ‘Moro’ observed at 10 dai. (c, d) Normal development of intercellular hyphae (ih) in ‘Fielder’ but in ‘Moro’, the hypersensitive response results in a loss of fungal organization and structure (white arrows) associated with intercellular hypha and protruding haustorial mother cells (black arrows) is observed at 12 dai. (e, f) Pustules (p) formed beneath the epidermis in ‘Fielder’ are evident at 13 dai whereas in ‘Moro’, mesophyll cells among the intercellular hyphae (black arrows) are undergoing HR with loss of cell contents and disintegration of membranes (white arrows), membrane disintegration is becoming widespread in leaf cells (inset) encircled by intercellular hyphae (ih). Sections embedded in paraffin and stained with triple stain. Bars = 20 µm.
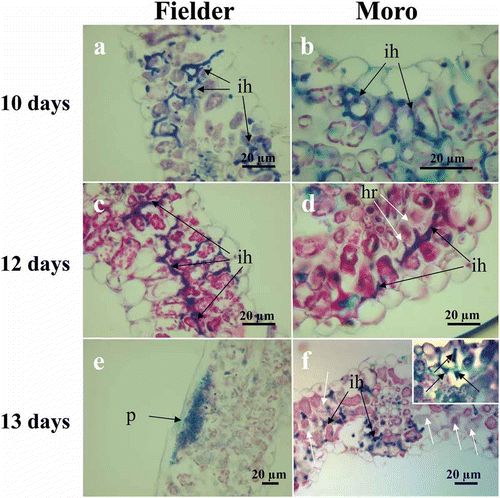
Table 2. Percentage of germinating spores, substomatal vesicles and numbers of HR cells within infection sites in resistant ‘Moro’ and susceptible ‘Fielder’ wheat following inoculation with P. striiformis strain SRC-84.
In both cultivars, haustorial mother cells were formed in the intercellular spaces adjacent to mesophyll cells following contact of intercellular hyphae with the mesophyll cells (). Haustorial development consisted of formation of a haustorial neckband and subsequent formation of a bulbous haustorium after 8 to 14 dai (, ). Multiple haustoria were observed in individual mesophyll cells of both ‘Fielder’ () and ‘Moro’ (, ). In the incompatible interaction involving ‘Moro’, formation of haustoria was similar to that in ‘Fielder’ but they soon began deteriorating shortly after enlarging () from 8–12 dai. Cytoplasmic contents within both the haustoria and haustorial mother cells developed a vacuolar and granular appearance, staining darkly in both TEM and light microscopic sections, and fungal plasma and organelle membranes became disorganized (, , , , and ). These changes were observed prior to the actual collapse of the host cells, demonstrating that cytotoxic effects were occurring in the pathogen prior to the collapse of the cell (compare , , ). Matrix substances accumulated around intercellular hyphae and began accumulating around fungal hyphae and on the exterior of mesophyll cell walls at 12–14 dai ().
Fig. 3 TEM images of strain SRC-84 of P. striiformis haustorium development in ‘Fielder’ (a, c and e) and ‘Moro’ (b, d and f). (a) Intercellular spread of hyphae (ih) and formation of a haustorial mother cell (hmc), intercellularly, adjacent to a mesophyll cells (mc) at 9 dai. (b) Haustorial complex consisting of haustorial mother cell (hmc), haustorial neckband (nb) and haustorium (ha) at 11 dai. Note the deeply staining, granular appearance of haustorium (ha) and haustorial mother cell (hmc) cytoplasm, and presence of cytoplasmic contents (cy) in the intercellular space. (c) Haustorial complex consisting of haustorial mother cell (hmc), haustorial neckband (nb) and haustorium (ha) inside a mesophyll cell (mc) at 11 dai. (d) Early stages of hypersensitive response illustrating the breakdown of haustoria (ha) prior to cell collapse at 12 dai. Of the two haustoria shown, one in the upper right is intact while the one in the lower left has lost its extrahaustorial and haustorial membranes and the contents of the haustorium are being released into the mesophyll cell (mc). Inset shows partial degradation of both membranes. (e) Multiple haustoria (ha) inside a mesophyll cell at 11 dai. Also conspicuous are numerous chloroplasts and a nucleus (n) within the mesophyll cell, and intercellular hyphae (ih) surrounding the mesophyll cell. (f) Collapse of the mesophyll cell (mc) coincident with the presence of haustoria (ha) at 12 dai. Inset shows matrix (m) substance accumulating exterior to mesophyll cells adjacent to a intercellular hyphum (h). Bars = 2.5 µm.
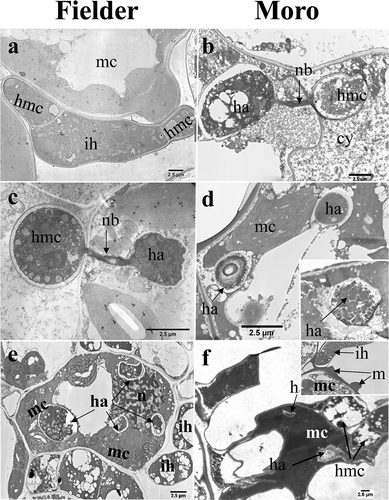
In the incompatible interaction with ‘Moro’, weak autofluorescence was initially observed at 6 dai associated with stomata and in mesophyll cell walls that had come into contact with primary hyphae, haustorial mother cells and haustoria (). Strong autofluorescence that characterized cells undergoing cell collapse and HR was first observed at 8 dai and became more generalized around the individual penetration sites at 10–12 dai (, and ). Often, the mesophyll cells undergoing HR in a single localized infection site totalled 8 or more cells at 12 dai ( and ). These results are consistent with macroscopic observations of developing chlorosis and necrosis on inoculated ‘Moro’ leaves at 12 dai (). The on-going increase in the number of cells (up to 8) exhibiting HR within leaf discs from 8 to 16 dai in ‘Moro’ () was due to ongoing proliferation of primary intercellular hyphae that develop new haustoria in cells coupled with HR in cells immediately adjacent to haustorium-containing cells (, ).
Fig. 4 (Colour online) Localization of hypersensitive cell death at individual penetration sites in resistant ‘Moro’ (a-c) and susceptible ‘Fielder’ (d) following inoculation with P. striiformis strain SRC-84. (a) Mesophyll cells (mc) in contact with primary infection hyphae, haustorial mother cells (hmc) and haustoria (h) exhibit weak autofluorescence at 6 dai. (b) Stronger autofluorescence associated with hypersensitive response (hr) among limited numbers of cells at 8 dai. (c) A substomatal vesicle (sv) is present in a larger affected area of infected mesophyll cells undergoing hypersensitive response (hr) exhibit a stronger autofluorescence at 12 dai. Collapsed cell (cc) undergoing hr. (d) Limited numbers of mesophyll cells have collapsed (cc) adjacent to pustules (pu) that exhibit weak fluorescence at 14 dai. Whole leaf sections were cleared in ethanol and stained with aniline blue. Bars = 50 µm.
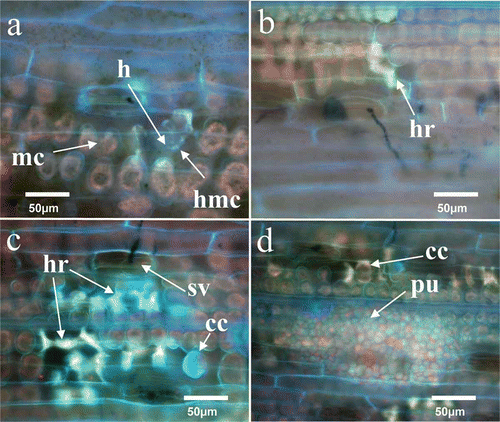
Fig. 5 Average number of necrotic cells undergoing HR per infection site exhibiting strong fluorescence from 0 to 16 dai, in resistant ‘Moro’ and susceptible ‘Fielder’ after inoculation with P. striiformis strains SRC-84 and CDL-29.
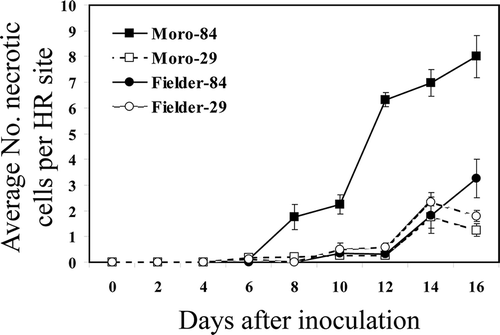
In the compatible interaction in ‘Fielder’, fluorescence associated with infection was rare and weak during early host-parasite interactions from 6 to 12 dai but increased from 12–16 dai (). This was attributed to the fluorescence associated with collapse of one or few mesophyll cells adjacent to haustoria mother cells (). Pustules also showed slight fluorescence (). Results with inoculation of ‘Moro’ with strain CDL-29 that overcomes Yr10 resistance yielded a susceptible reaction and the sequence of morphological events from germination through to sporulation was essentially the same as that observed in ‘Fielder’ treatments inoculated with this strain ().
H2O2 accumulation in the incompatible and compatible interactions
In non-inoculated ‘Moro’ and ‘Fielder’ plants, DAB staining was occasionally observed involving 1–2 cells (not shown). An initial oxidative burst was observed in both incompatible and compatible interactions from 2 to 4 dai () followed by a second, significantly higher (P ≤ 0.05) burst at 6 dai and a third at 14 dai in ‘Moro’(P ≤ 0.05). Conversely, in ‘Fielder’, the number of infected sites exhibiting DAB staining increased gradually over the time course achieving the same level as observed in ‘Moro’ after 16 dai.
Fig. 6 Average number of infection sites per leaf disc exhibiting H2O2 accumulation as visualized by DAB staining in resistant ‘Moro’ and susceptible ‘Fielder’ from 0–16 dai after inoculation with P. striiformis, strain SRC-84. Bars represent standard error. Where error bars are not visible, they occur within the space of the marker.
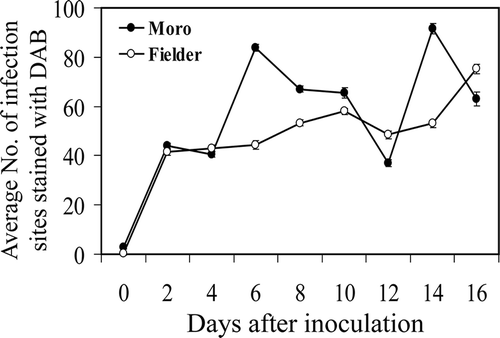
Profiling of defence-related genes
Based on inoculation results of infection studies on ‘Moro’ and ‘Fielder’ with strain SRC-84, appropriate infection levels were sufficiently high in all tests to permit interpretation of results of the profiling of defence-related genes in wheat. qRT-PCR and REST analyses demonstrated expression of defence-related genes was globally higher in ‘Moro’ than in ‘Fielder’ inoculated treatments compared with the non-inoculated controls, for most of the defence-related genes considered in this study (, ). However, the level of defence gene expression in ‘Fielder’ was frequently at lower levels and/or delayed during the course of pathogen penetration and establishment than that of ‘Moro’ despite the patterns of gene expression being similar between the two cultivars. The patterns of expression of these genes permitted placing the genes into two broad categories: (a) the upstream defence genes up-regulated early in ‘Moro’ compared with ‘Fielder’ at 2–6 dai that are subsequently down-regulated and later transiently up-regulated to a lesser extent at 8–12 dai (). These included the defence-signalling genes PAD4, EDS1 and AOS that all shared a similar expression pattern; EDS1 was differentially up-regulated in ‘Moro’ compared with ‘Fielder’ at 2 and 4 dai and PAD4 and AOS were similarly up-regulated only after 4 dai; and (b) the downstream defence genes PR1.1 and TLP1 and Glu1 that are up-regulated in ‘Moro’ compared with ‘Fielder’ from 4 to 12 dai (). The pattern of expression of these genes also became elevated in ‘Fielder’ but generally to a lesser extent and this increase in expression occurred later than in ‘Moro’ (). PAL expression was negligible throughout the time course of infection whereas expression of Chi3 and LTP1 were similar in both ‘Fielder’ and ‘Moro’ (data not shown).
Fig. 7 Relative expression levels of PAD4, EDS1 and AOS in resistant ‘Moro’ and susceptible ‘Fielder’ after inoculation with P. striiformis, strain SRC-84 from 2 to 14 dai using qRT-PCR. Values represent differences of inoculated treatments compared with the corresponding non-inoculated controls and bars represent standard errors.
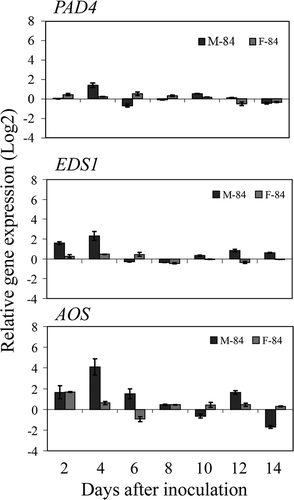
Fig. 8 Relative expression levels of PR-1.1, TLP1 and Glu1 in resistant ‘Moro’ and susceptible ‘Fielder’ after inoculation with P. striiformis, strain SRC-84 from 2 to 14 dai using qRT-PCR. Values represent differences of inoculated treatments compared with the corresponding non-inoculated controls and bars represent standard errors.
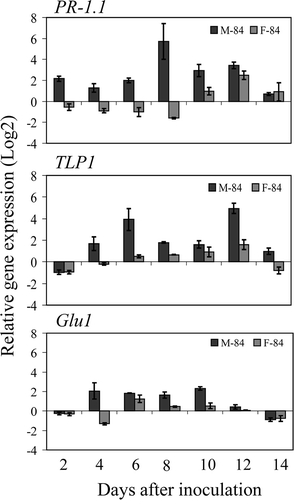
Discussion
The temporal cytological and defence-associated transcriptional events during penetration and infection of wheat by P. striiformis were clearly different in ‘Moro’ wheat containing Yr10 compared with ‘Fielder’ wheat. The early aspects of infection including spore germination, penetration, substomatal vesicle formation and intercellular spread between 2 and 8 dai were very similar in both compatible ‘Fielder’ and incompatible ‘Moro’ interactions studied. Transcriptionally, however, a very early transient expression from 2 to 4 dai of EDS1, PAD4 and AOS occurred to differing extents in the SRC-84/‘Moro’ compared with the SRC-84/‘Fielder’ interactions. Their higher expression coincided with the earliest, non-specific, phase 1 of the oxidative burst at 2 dai observed in both ‘Moro’ and ‘Fielder’ inoculated with race SRC-84. Up-regulation of a basic PR1.1, β-1,3-glucanase and a thaumatin-like protein was observed at 4 to 12 dai and generally preceded or coincided with the expression of phase 2 of the oxidative burst that was observed at 6 dai in the SRC-84/‘Moro’ interaction but not in the SRC-84/‘Fielder’ interaction. The HR in the SRC-84/‘Moro’ interaction was observed from 10 to 12 dai, occurred after the establishment of one or more haustoria in mesophyll cells and was associated with a rapidly expanding autofluorescence in mesophyll cells. The HR reaction in ‘Moro’ terminated infection and was expressed as chlorotic spots that became necrotic at 16 dai. This contrasted with extensive sporulation observed in ‘Fielder’ at 14 dai.
Light microscopy and TEM studies revealed that haustoria were numerous in ‘Moro’ and development was extensive before the onset of HR, suggesting that development of functional haustoria was required prior to induction of HR. In ‘Moro’, degradation within the fungal membranes associated with haustoria and organelles started prior to cell collapse, suggesting that a toxic response in fungal tissues occurred prior to this event. Up-regulation of PR1.1 and TLP1 and Glu1 preceded the HR from 4 to 12 dai and the toxic effects of these and other PR-proteins could have contributed to the toxic response in haustoria at 10–14 dai. Some studies have shown that occurrence HR is post-haustorial in monocots and dicots (Tani et al. Citation1975; Heath Citation1981; Niks & Dekens Citation1991) whereas in others, HR is pre-haustorial (Koch & Slusarenko Citation1990). Among wheat cultivars possessing the Lr9, Lr20 and Lr28 alleles inoculated with the leaf rust pathogen (P. triticina), and Sr6 inoculated with the stem rust pathogen (P. graminis f. sp. tritici), HR was formed after establishment of the haustorium (Skipp & Samborski Citation1974; Southerton & Deverall 2007).
Initially, HR remained restricted to cells containing haustoria at 8–10 dai and the ongoing increase in the number of cells exhibiting HR within leaf discs from 12 to 16 dai in ‘Moro’ was associated with an ongoing proliferation of intercellular hyphae coupled with a localized HR among cells immediately adjacent to haustorium-containing cells. Shortly after the HR, the haustorial mother cells became vacuolar and the cytoplasm appeared granular, indicating a transfer of a toxic response from the HR to the haustorial mother cell. Matrix substances, likely callose (Thordal-Christensen et al. Citation1997; Mellersh & Heath Citation2003), accumulated on the intercellular hyphae and on the cell walls of apparently healthy mesophyll cells adjacent to the HR-cells. Callose deposition may be triggered during PAMP induced PTI and elicitor-induced ETI (Gaudet et al. Citation2010; Luna et al. 2010).
The first differential oxidative burst, corresponding to phase 2 (Lamb & Dixon Citation1997), was observed in resistant ‘Moro’ at 6 dai and likely served as the threshold stimulus for triggering the HR that was observed from 6 to 12 dai. A single oxidative burst at 12–24 hours was reported in host-pathogen interactions in ‘Suwon 11’ wheat inoculated with an avirulent race of P. striiformis (Wang et al. Citation2007). It is noteworthy that in the compatible system involving ‘Suwon 11’, sporulation is detected within 9 dai (Wang et al. Citation2007) compared with 14–16 dai in the present study. Thus difference in profiles of the oxidative bursts between those reported by Wang et al. (Citation2007) and those in the present study is likely attributed to the growth conditions between the two studies; however other factors such as different R-gene under consideration and the races used, may also be involved. A second differential oxidative burst at 14 dai in ‘Moro’ may have coincided with the rapid expansion in the number of fluorescing mesophyll cells undergoing HR. Alternatively, the increases in DAB cells observed at 14 dai in ‘Moro’ may have been an earlier expression of the same trend observed at 16 dai in the susceptible ‘Fielder’.
Plants deploy a large repertoire of defence responses when infected by pathogens, including up-regulation of PR-proteins and phytoalexins that can have direct or indirect action on the course of pathogenesis (Van Loon et al. Citation2006). In the present study, a coordinated expression among different classes of defence-related genes, including early expression of signalling genes EDS1, PAD4 and AOS after 2–6 days was observed. EDS1 and PAD4 are key post-invasion plant defence regulators involved in basal resistance to obligate parasites in Arabidopsis (Wiermer et al. Citation2005; Lipka et al. Citation2008). AOS is a key enzyme in JA biosynthesis and its transcription levels have been associated with JA production (Laudert & Weiler Citation1998; Agrawal et al. Citation2002). These genes are also involved in a subset of R-gene-mediated resistance pathways especially in conjunction with TIR NBS-LRR R-genes that have been identified in dicot species and in some monocots such as rice, but not in cereals (Feys et al. Citation2001; Bai et al. Citation2002; Wiermer et al. Citation2005). The early up-regulation and subsequent down-regulation of EDS1, PAD4 and AOS in ‘Moro’ suggests that these genes are involved in the early signalling of basal resistance to stripe rust in wheat conferred by Yr10. The early accumulation of AOS transcripts at 24 h also suggests that JA synthesis occurs early in response to stripe rust infection, specifically before the HR-associated lesion formation in the incompatible interaction (Agrawal et al. Citation2002; Mei et al. Citation2006).
Although no morphological differences between ‘Moro’ and ‘Fielder’ were observed prior to 8–12 dai, the PR-proteins TLP, PR1.1 and Glu1 transcripts were generally up-regulated in ‘Moro’ during the early stages from 4 to 6 dai. In contrast, the elevated expression of same genes in ‘Fielder’ was detected later, between 6 and 12 dai. Transcript levels for PAL did not vary appreciably through the time course suggesting that this pathway was not critical in Yr10 resistance to P. striiformis in wheat. A sparse mycelial growth was observed following penetration in both ‘Moro’ and ‘Fielder’ between 2 and 8 dai. Following penetration, active proliferation of the fungus in mesophyll tissues would be expected, particularly in susceptible ‘Fielder’. Based on their expression pattern, the early, albeit attenuated up-regulation of signalling genes EDS1, PAD4, AOS, and the PR-proteins TLP and PR1.1, may have been sufficient to partially limit fungal growth, and subsequent down-regulation of these genes 8–12 dai may have permitted resumption of the fungal proliferation. Degradation of P. striiformis haustoria prior to observing cells collapsing in ‘Moro’ may have been as a result of the up-regulation of one or more of these genes at 10–14 dai. In the susceptible interaction in ‘Fielder’, transcript accumulation for the majority of these defence-related genes was either down-regulated significantly compared with ‘Moro’ during both the early and later stages of the host parasite interaction, or delayed. Assessment of regulation of these transcripts for several different all stage stripe rust resistance R-genes in wheat would help clarify whether the expression patterns observed for these genes are general for R-gene incompatible interactions or are specific for the Yr10 mediated interaction.
To place the P. striiformis-wheat Yr10 pathosystem in ‘Moro’ within the context of current models of host-parasite interactions (Chisholm et al. Citation2006; Jones & Dangl Citation2006; Bent & Mackey Citation2007), the first oxidative burst observed in both ‘Moro’ and ‘Fielder’ and coincident with an early expression of the defence gene EDS1, PAD4 and AOS observed at 2–6 dai were likely associated with the PTI response. Because P. striiformis is an adapted pathogen to wheat, it is able to inhibit and/or tolerate these, as well as those of TLP1 and Glu1, until they wane after 2–8 dai, thereby evading PTI. The first differential oxidative burst at 6 dai in ‘Moro’, which occurred prior to haustorium formation and subsequent HR, likely initiated ETI. ETI is triggered following recognition of a specific unknown P. striiformis f. sp. tritici elicitor by the CC-NBS-LRR protein encoding Yr-10 (Laroche et al. Citation2005). The current hypothesis regarding HR and H2O2 and associated defence responses is that there is an oxidative cell death loop whereby the initial H2O2 increase following pathogen infection activates SA synthesis which, in turn, increases levels of SA that potentiates H2O2 production, which in turn activates the synthesis of more SA and cell death in a self-amplifying loop (Overmyer et al. Citation2003; Vlot et al. Citation2009). In the present study, up-regulation of PR-1.1, an indicator of salicylic acid activation (Van Loon et al. Citation2006), and the thaumatin-like protein, coincided with the second oxidative burst that preceded the HR. The role of SA in resistance expression in wheat to stripe rust has recently been demonstrated (Yang et al. Citation2013). These and other defence reactions initiated during the oxidative burst likely contributed to the deterioration of haustoria inside the cells that was in progress during the HR. Callose deposition is a characteristic of both PTI and ETI; the collective action of these gene products leads to the final growth arrest and death of P. striiformis in ‘Moro’. In ‘Fielder’, decreased and/or delayed defence gene expression permitted P. striiformis to evade the defence response and allowed an effective infection and sporulation at 12–14 dai.
Because ‘Moro’ wheat exhibited severity levels similar to ‘Fielder’ when inoculated with the virulent isolate CDL-29, similar to the severity levels observed following infection of ‘Fielder’ with SRC-84, it is likely that all resistance reactions observed could be attributable to the sole action of Yr10; there were unlikely effects attributable to the YrMor gene in ‘Moro’ reported by Chen et al. (Citation1995). This is supported by other results in our laboratory (Laroche, unpublished results) demonstrating that silencing of Yr10 in ‘Moro’ resulted in a fully susceptible reaction following inoculation with the avirulent strain SRC-84.
In summary, studies were carried out to describe the penetration and infection processes of P. striiformis in susceptible ‘Fielder’ wheat and in Yr10 gene resistant ‘Moro’ wheat, and to assess the relative contributions of the oxidative burst, defence-gene expression and HR on the morphological development of the pathogen. We demonstrated that recognition responses in hosts occur early in the host-parasite interaction but that key defence responses that terminate pathogen development and response occurred later. These results are the first among cereal rusts to integrate detailed morphological aspects of defence reactions including oxidative burst and HR with transcript profiling of annotated genes and permitted interpretation of the Yr10/stripe rust interaction in the context of current models of host-parasite interactions. These results will also permit the future identification of non-annotated genes based on profile expression and additional key factors involved in the Yr10 mediated resistance response to P. striiformis in wheat.
Acknowledgements
We thank Dr Yuan-Yuan Wang, Dr Ye-Hong Liang, Carolyn Penniket and Thérèse Despins for assistance with the experimental work and suggestions in solving technical problems.
Funding
We thank the China Scholarship Council for financial support of Xiao-Min Wang during the stay in Canada. This study was partly supported by Agriculture and Agri-Food Canada ABIP, Alberta Innovates Biosolutions, and the 111 project of the Education Ministry of China (B07049).
References
- Agrawal GK, Rakwal R, Jwa N-S, Han K-S, Agrawal VP. 2002. Molecular cloning and mRNA expression analysis of the first rice jasmonate biosynthetic pathway gene allene oxide synthase. Plant Physiol Biochem. 40:771–782. doi:10.1016/S0981-9428(02)01429-8
- Alvarez ME, Pennell RI, Meijer P, Ishikawa A, Dixon RA, Lamb C. 1998. Reactive oxygen intermediates mediate a systemic signal network in the establishment of plant immunity. Cell. 92:773–784. doi:10.1016/S0092-8674(00)81405-1
- Anand A, Schmelz EA, Muthukrishnan S. 2003. Development of a lesion-mimic phenotype in a transgenic wheat line overexpressing genes for pathogenesis-related (PR) proteins is dependent on salicylic acid concentration. Mol Plant-Microbe Interact. 16:916–925. doi:10.1094/MPMI.2003.16.10.916
- Bai J, Pennill LA, Ning J, Lee SW, Ramalingam J, Webb CA, Zhao B, Sun Q, Nelson JC, Leach JE, Hulbert SH. 2002. Diversity in nucleotide binding site-leucine-rich repeat genes in cereals. Genome Res. 12:1871–1884. doi:10.1101/gr.454902
- Bent AF, Mackey D. 2007. Elicitors, effectors, and R genes: the new paradigm and a lifetime supply of questions. Ann Rev Phytopathol. 45:399–436. doi:10.1146/annurev.phyto.45.062806.094427
- Bestwick C, Adam A, Puri N, Mansfield J. 2001. Characterisation of and changes to pro and anti-oxidant enzyme activities during the hypersensitive reaction in lettuce (Lactuca sativa L.). Plant Sci. 161:497–506. doi:10.1016/S0168-9452(01)00427-7
- Chamnongpol S, Willekens H, Moeder W, Langebartels C, Sandermann H, Van Montagu M, Inze D, Van Camp W. 1998. Defense activation and enhanced pathogen tolerance induced by H2O2 in transgenic tobacco. Proc Natl Acad Sci. 95:5818–5823. doi:10.1073/pnas.95.10.5818
- Chen WQ, Wu LR, Liu TG, Xu SC, Jin SL, Peng YL, Wang BT. 2009. Race dynamics, diversity, and virulence evolution in Puccinia striiformis f. sp. tritici, the causal agent of wheat stripe rust in china from 2003 to 2007. Plant Dis. 93:1093–1101. doi:10.1094/PDIS-93-11-1093
- Chen X. 2005. Epidemiology and control of stripe rust [Puccinia striiformisf. sp.tritici] on wheat. Can J Plant Pathol. 27:314–337. doi:10.1080/07060660509507230
- Chen X, Line RF. 1992. Inheritance of stripe rust resistance in wheat cultivars used to differentiate races of Puccinia striiformis in North America. Phytopathology. 82:633–637. doi:10.1094/Phyto-82-633
- Chen X, Line RF. 1993. Inheritance of stripe rust (yellow rust) resistance in the wheat cultivar Carstens V. Euphytica. 71:107–113. doi:10.1007/BF00023473
- Chen X, Line RF, Jones SS. 1995. Chromosomal location of genes for resistance to Puccinia striiformis in winter wheat cultivars Heines VII, Clement, Moro, Tyee, Tres, and Daws. Phytopathology. 85:1362–1367. doi:10.1094/Phyto-85-1362
- Chisholm ST, Coaker G, Day B, Staskawicz BJ. 2006. Host-microbe interactions: shaping the evolution of the plant immune response. Cell. 124:803–814. doi:10.1016/j.cell.2006.02.008
- Delledonne M, Murgia I, Ederle D, Sbicego P, Biondani A, Polverari A, Lamb C. 2002. Reactive oxygen intermediates modulate nitric oxide signaling in the plant hypersensitive disease-resistance response. Plant Physiol Biochem. 40:605–610. doi:10.1016/S0981-9428(02)01397-9
- Feys BJ, Moisan LJ, Newman M-A, Parker JE. 2001. Direct interaction between the Arabidopsis disease resistance signaling proteins, EDS1 and PAD4. EMBO J. 20:5400–5411. doi:10.1093/emboj/20.19.5400
- Flor HH. 1971. Current status of the gene-for-gene concept. Ann Rev Phytopathol. 9:275–296. doi:10.1146/annurev.py.09.090171.001423
- Gaudet DA, Wang Y, Penniket C, Lu ZX, Bakkeren G, Laroche A. 2010. Morphological and molecular analyses of host and nonhost interactions involving barley and wheat and the covered smut pathogen Ustilago hordei. Mol Plant-Microbe Interact. 23:1619–1634. doi:10.1094/MPMI-11-09-0271
- Grant MR, Jones JDG. 2009. Hormone (dis)harmony moulds plant health and disease. Science. 324:750–752. doi:10.1126/science.1173771
- Heath MC. 1981. Resistance of plants to rust infection. Phytopathology. 71:971–974. doi:10.1094/Phyto-71-971
- Jabs T, Tschope M, Colling C, Hahlbrock K, Scheel D. 1997. Elicitor-stimulated ion fluxes and O2 from the oxidative burst are essential components in triggering defense gene activation and phytoalexin synthesis in parsley. Proc Natl Acad Sci. 94:4800–4805. doi:10.1073/pnas.94.9.4800
- Johansen DA. 1940. Plant microtechnique. New York, NY: McGraw Hill; p. 523.
- Jones JDG, Dangl JL. 2006. The plant immune system. Nature. 444:323–329. doi:10.1038/nature05286
- Keogh RC, Deverall BJ, Mcleod S. 1980. Comparison of histological and physiological responses to Phakopsora pachyrhizi in resistant and susceptible soybean. Trans Br Mycol Soc. 74:329–333. doi:10.1016/S0007-1536(80)80163-X
- Koch E, Slusarenko A. 1990. Arabidopsis is susceptible to infection by a downy mildew fungus. Plant Cell. 2:437–445. doi:10.1105/tpc.2.5.437
- Lamb C, Dixon RA. 1997. The oxidative burst in plant disease resistance. Annu Rev Plant Physiol Plant Mol Biol. 48:251–275. doi:10.1146/annurev.arplant.48.1.251
- Laroche A, Eudes F, Frick MM, Huel R, Nykiforuk CL, Conner RL, Chen Q, Kuzyk A, Soltanloo H, Gaudet D. 2005. Yr10, the wheat stripe rust resistance gene from Moro. In: Stripe rust of wheat: a plan for recovery. The ASA-CSSA-SSA International Annual Meetings, p. 232.
- Laudert D, Weiler EW. 1998. Allene oxide synthase: a major control point in Arabidopsis thaliana octadecanoid signalling. Plant J. 15:675–684. doi:10.1046/j.1365-313x.1998.00245.x
- Line R, Chen X. 1995. Successes in breeding for and managing durable resistance to wheat rusts. Plant Dis. 79:1254–1255.
- Lipka U, Fuchs R, Lipka V. 2008. Arabidopsis non-host resistance to powdery mildews. Curr Opin Plant Biol. 11:404–411. doi:10.1016/j.pbi.2008.04.004
- Luna E, Pastor V, Robert J, Flors V, Mauch-Mani B, Ton J. 2011. Callose deposition: a multifaceted plant defense response. Mol Plant-Microbe Interact. 24:183–193. doi:10.1094/MPMI-07-10-0149
- Mei C, Qi M, Sheng G, Yang Y. 2006. Inducible overexpression of a rice allene oxide synthase gene increases the endogenous jasmonic acid level, PR gene expression, and host resistance to fungal infection. Mol Plant-Microbe Interact. 19:1127–1137. doi:10.1094/MPMI-19-1127
- Mellersh DG, Heath MC. 2003. Aninvestigation into the involvement of defense signaling pathways in components of the nonhost resistance of Arabidopsis thaliana to rust fungi also reveals a model system for studying rust fungal compatibility. Mol Plant-Microbe Interact. 16:398–404. doi:10.1094/MPMI.2003.16.5.398
- Niks RE, Dekens RG. 1991. Prehaustorial and posthaustorial resistance to wheat leaf rust in diploid wheat seedlings. Phytopathology. 81:847–851. doi:10.1094/Phyto-81-847
- Nurnberger T, Brunner F, Kemmerling B, Piater L. 2004. Innate immunity in plants and animals: striking similarities and obvious differences. Immunol Rev. 198:249–266. doi:10.1111/j.0105-2896.2004.0119.x
- Overmyer K, Brosché M, Kangasjärvi J. 2003. Reactive oxygen species and hormonal control of cell death. Trends Plant Sci. 8:335–342. doi:10.1016/S1360-1385(03)00135-3
- Peng M, Kuc J. 1992. Peroxidase-generated hydrogen peroxide as a source of antifungal activity in vitro and on tobacco leaf disks. Phytopathology. 82:696–699. doi:10.1094/Phyto-82-696
- Peterson RF, Campbell AB, Hannah AE. 1948. A diagrammatic scale for estimating rust intensity on leaves and stems of cereals. Can J Res. 26c:496–500. doi:10.1139/cjr48c-033
- Pfaffl MW, Horgan GW, Dempfle L. 2002. Relative expression software tool (REST©) for group-wise comparison and statistical analysis of relative expression results in real-time PCR. Nucleic Acids Res. 30:e36. doi:10.1093/nar/30.9.e36
- Puchalski B, Gaudet DA. 2011. 2010 southern Alberta stripe rust survey. Can Plant Dis Surv. 91:69–70.
- Rozen S, Skaletsky H. 2000. Primer3 on the WWW for general users and for biologist programmers. Methods Mol Biol. 132:365–386.
- Skipp RA, Samborski DJ. 1974. The effect of the Sr6 gene for host resistance on histological events during the development of stem rust in near-isogenic wheat lines. Can J Bot. 52:1107–1115. doi:10.1139/b74-140
- Southerton S, Deverall B. 1989. Histological studies of the expression of the Lr9, Lr20 and Lr28 alleles for resistance to leaf rust in wheat. Plant Pathol. 38:190–199. doi:10.1111/j.1365-3059.1989.tb02133.x
- Su H, Conner RL, Graf RJ, Kuzyk AD. 2003. Virulence of Puccinia striiformis f. sp. tritici, cause of stripe rust on wheat, in western Canada from 1984 to 2002. Can J Plant Pathol. 25:312–319. doi:10.1080/07060660309507084
- Tani T, Yamamoto H, Onoe T, Naito N. 1975. Initiation of resistance and host cell collapse in the hypersensitive reaction of oat leaves against Puccinia coronata avenae. Physiol Plant Pathol. 7:231–242. doi:10.1016/0048-4059(75)90028-4
- Tarr DE, Alexander H. 2009. TIR-NBS-LRR genes are rare in monocots: evidence from diverse monocot orders. BMC Res Notes. 2:197. doi:10.1186/1756-0500-2-197
- Thordal-Christensen H, Zhang Z, Wei Y, Collinge DB. 1997. Subcellular localization of H2O2 in plants. H2O2 accumulation in papillae and hypersensitive response during the barley-powdery mildew interaction. Plant J. 11:1187–1194. doi:10.1046/j.1365-313X.1997.11061187.x
- Van Loon LC, Rep M, Pieterse CMJ. 2006. Significance of inducible defense-related proteins in infected plants. Ann Rev Phytopathol. 44:135–162. doi:10.1146/annurev.phyto.44.070505.143425
- Venable JH, Coggeshall R. 1965. A simplified lead citrate stain for use in electron microscopy. J Cell Biol. 25:407–408. doi:10.1083/jcb.25.2.407
- Veronese P, Ruiz MT, Coca MA, Hernandez-Lopez A, Lee H, Ibeas JI, Damsz B, Pardo JM, Hasegawa PM, Bressan RA, Narasimhan M. 2003. In defense against pathogens. Both plant sentinels and foot soldiers need to know the enemy. Plant Physiol. 131:1580–1590. doi:10.1104/pp.102.013417
- Vlot AC, Dempsey DMA, Klessig DF. 2009. Salicylic acid, a multifaceted hormone to combat disease. Ann Rev Phytopathol. 47:177–206. doi:10.1146/annurev.phyto.050908.135202
- Wang C-F, Huang L-L, Buchenauer H, Han Q-M, Zhang H-C, Kang Z-S. 2007. Histochemical studies on the accumulation of reactive oxygen species (O2− and H2O2) in the incompatible and compatible interaction of wheat—Puccinia striiformis f. sp. tritici. Physiol Mol Plant Pathol. 71:230–239. doi:10.1016/j.pmpp.2008.02.006
- Wiermer M, Feys BJ, Parker JE. 2005. Plant immunity: the EDS1 regulatory node. Curr Opin Plant Biol. 8:383–389. doi:10.1016/j.pbi.2005.05.010
- Yang Y, Zhao J, Liu P, Xing H, Li C, Wei G, Kang Z. 2013. Glycerol-3-phosphate metabolism in wheat contributes to systemic acquired resistance against Puccinia striiformis f. sp. tritici. PLoS ONE. 8:e81756.
- Yu G-X, Braun E, Wise RP. 2001. Rds and Rih mediate hypersensitive cell death independent of gene-for-gene resistance to the oat crown rust pathogen Puccinia coronata f. sp. avenae. Molecular Plant-Microbe Interact. 14:1376–1383. doi:10.1094/MPMI.2001.14.12.1376