Abstract
The occurrence of stripe rust (Puccinia striiformis Westend.) of cereal in central Alberta was first documented nearly a century ago. Since the late 1990s, outbreaks of wheat stripe rust caused by P. striiformis f. sp. tritici (Pst) were frequently reported and barley stripe rust caused by P. striiformis f. sp. hordei (Psh) was regularly observed. The major source of stripe rust inoculum for Alberta is considered to be from the Pacific Northwest (PNW) region of the USA. However, this pathogen was found to have the potential to survive on winter wheat in central Alberta and then spread to other winter and spring cereals the following growing season. During 2007–2011, 165 field collections of stripe rust samples were identified to forma specialis and pathotype using wheat and barley differentials. Overlapping virulence was observed in both Pst and Psh isolates that were virulent on highly susceptible wheat and barley cultivars and lines. Potential hybridization between the two formae speciales is considered to be the source of isolates capable of overcoming multiple wheat and barley resistance genes. The predominant Pst races were similar between central Alberta and the PNW, while the predominant Psh races appeared to be different between the two regions. Wheat genes in differential lines were found to show more resistance in central Alberta trap nurseries than in southern Alberta and the PNW. Low to moderate disease pressure in central Alberta was sufficient to cause significant yield losses in susceptible spring wheat cultivars, while there was limited to no yield reduction in resistant or intermediate cultivars in this region. Winter wheat is generally more susceptible than spring wheat and, thus, yield losses in winter wheat are more likely to occur than susceptible spring wheat in this region. Considerably more two-row hulled barley lines were resistant than six-row and hulless lines screened in international nurseries. For management of this disease, an integrated approach is discussed in relation to central Alberta conditions.
Résumé
L’incidence de la rouille jaune (Puccinia striiformis Westend.) des céréales dans le centre de l’Alberta a été documentée il y a près d’un siècle. Depuis la fin des années 1990, les flambées de rouille jaune du blé, causées par P. striiformis f. sp. tritici (Pst), ont fréquemment été rapportées et la rouille jaune de l’orge, causée par P. striiformis f. sp. hordei (Psh), a couramment été observée. La source majeure d’inoculum de rouille jaune en Alberta semble provenir de la région du Nord-Ouest du Pacifique (N.-O. P.) des États-Unis. Toutefois, on a constaté que cet agent pathogène pouvait survivre sur le blé d’hiver cultivé dans le centre de l’Alberta pour, la saison suivante, infecter les autres céréales d’hiver et de printemps. De 2007 à 2011, 165 collections d’échantillons de rouille jaune prélevés dans les champs ont été assimilées à une forma specialis et à un pathotype à partir de différentiels de blé et d’orge. Un chevauchement de la virulence a été observé tant chez les isolats de Pst que de Psh qui étaient virulents à l’égard des lignées et des cultivars de blé et d’orge hautement réceptifs. La possible hybridation des deux formae speciales est considérée comme la source des isolats qui ont la capacité d’inactiver les multiples gènes de résistance du blé et de l’orge. Les races de Pst prédominantes du centre de l’Alberta et du N.-O. P. se ressemblaient, tandis que les races de Psh prédominantes différaient d’une région à l’autre. Dans les lignées différentielles, les gènes de blé se sont avérés plus résistants dans les pépinières-appâts du centre de l’Alberta que dans le sud de l’Alberta et dans le N.-O. P. Dans le centre de l’Alberta, un risque de maladie faible à modéré a été suffisant pour engendrer des pertes importantes de rendement chez les cultivars réceptifs de blé de printemps, tandis qu’il n’y en a pas eu, ne fût-ce que de très faibles, chez les cultivars résistants ou moyennement résistants de la région. En règle générale, le blé d’hiver est plus réceptif que le blé de printemps et, par conséquent, dans cette région, les pertes de rendement risquent davantage de se produire chez le blé d’hiver que chez le blé de printemps réceptif. Lors d’examens en pépinières internationales, beaucoup plus de lignées d’orge à deux rangs à grain vêtu se sont avérées résistantes que de lignées à six rangs à grains nus. En ce qui a trait à la gestion de cette maladie, nous examinons une approche intégrée qui tient compte des conditions propres au centre de l’Alberta.
Introduction
Stripe rust, caused by Puccinia striiformis Westend., has traditionally been considered to be a problem only in soft white wheat (Triticum aestivum L.) in southern Alberta due to its late maturity and production under irrigated conditions (Su et al. Citation2003), despite the fact that this disease was first documented in 1918 in central Alberta (Fraser & Conners Citation1925). Stripe rust was rarely a concern in central Alberta until the late 1990s, when outbreaks of the disease caused production issues on wheat. In contrast, barley stripe rust has also been regularly observed, but currently has a limited impact. Infection on triticale (× Triticosecale) breeding plots has also occasionally been observed in this region (Xi et al. Citation2011).
Stripe rust severities of up to 100% were observed in wheat nurseries when disease outbreaks occurred in Alberta in 2005 (McCallum et al. Citation2006). This disease was also observed in barley (Hordeum vulgare L.) breeding plots and commercial fields in central Alberta the same year (Xi et al. Citation2011). A combination of factors likely contributed to the 2005 epidemic, including the use of susceptible cereal cultivars, severe stripe rust in the Pacific Northwest of the USA (PNW) that resulted in a large amount of inoculum coming into Alberta based on wind trajectory events (Turkington et al. Citation2009), local overwintering inoculum in winter wheat (Gehl, personal communication) and changes in pathogen virulence (Puchalski et al. Citation2013). Mild winters in central Alberta can be favourable for survival and development of the stripe rust pathogen. Aggressive races that are adapted to warmer conditions have been reported in the USA (Milus et al. Citation2006, Citation2009) and these races are considered to be responsible for recent outbreaks of stripe rust in western Canada (Fetch et al. Citation2011).
Central Alberta is epidemically close to the north end of the Puccinia pathway of stripe rust in North America. In this paper, the central Alberta region is defined by the area where the occurrence of stripe rust of wheat and barley has been regularly observed and the economic impact to cereal production has yet to be determined. This area completely surrounds the Edmonton capital region and includes the central part of the Calgary–Edmonton Corridor. This region consists of at least five census divisions (CD). In CD 7, 8, 9, 10 and 11, wheat and barley are the major crops, accounting for 32% (0.88 M ha) of all wheat acreage and 34% (0.43 M ha) of barley acreage being harvested in the province for 2011. Approximately 31% (2.78 M tonne) of wheat and 35% (1.59 M tonne) of barley production in Alberta occurs in these CDs (Anon. Citation2011a). Wheat stripe rust caused an estimated average yield loss of 1–5% in Alberta based on a recent report (Wellings Citation2011). Yield losses up to 70% in individual wheat commercial fields were observed in southern Alberta during the 1980s (Conner & Kuzyk Citation1988). The severity and impact of stripe rust is generally less in central Alberta due to the late arrival of inoculum than in southern Alberta. With this low to moderate disease pressure on wheat in central Alberta, yield losses were assumed to be insignificant. However, grain yield losses of 45% in the susceptible spring wheat cultivar ‘AC Crystal’ were observed in a research plot at Lacombe in central Alberta in 2000. Subsequent evaluations in 2012 and 2013 also demonstrated significant yield losses in susceptible spring wheat cultivars in experimental plots in Lacombe, AB (K. Xi, unpublished observations).
Successful management of stripe rust requires an understanding of factors such as the source of inoculum, race structure and frequency, and host resistance. Since 2007, research has been conducted to understand the distribution and frequency of virulent pathotypes in relation to wheat and barley resistance genes in central Alberta. With this information, management using resistant cultivars can be complemented with chemical control and cultural methods suitable for central Alberta conditions. This paper will compare race virulence, distribution and disease severity between central Alberta, southern Alberta and the PNW. It will also summarize research progress in the understanding of the potential overwintering of inoculum and the significance of a green bridge between winter and spring wheat crops for inoculum transmission and disease development. Disease management strategies are discussed in relation to cultivar resistance, pathogen variation, fungicide application and environmental factors pertinent to central Alberta conditions.
Pst virulence study
The occurrence and geographic distribution of wheat stripe rust during the early years in Alberta have been extensively documented (Fraser & Conners Citation1925; Johnson & Newton Citation1928; Sanford & Broadfoot Citation1932; Newton & Johnson Citation1936; Tyner Citation1947; Peturson et al. Citation1953; Anon. Citation1964, Citation1970). More recently, outbreaks of stripe rust resulted in high yield losses in soft white spring wheat in southern Alberta in 1981 and 1986 (Conner & Kuzyk Citation1988). By the late 1990s, stripe rust epidemics were not just limited to southern Alberta on irrigated soft white spring wheat, but also occurred throughout central Alberta, southern Saskatchewan and southern Manitoba (McCallum & Fetch Citation2001). Wheat stripe rust was also observed in Saskatchewan and Manitoba during the growing seasons of 2005 and 2006 (McCallum et al. Citation2006, Citation2007a). Wheat stripe rust was also observed in the Peace River region of Alberta in 2013 (R. Howard, personal communication).
Wheat stripe rust is primarily caused by Puccinia striiformis f. sp. tritici (Pst) worldwide. The pathogen is variable in virulence which has rendered cultivar resistance ineffective after resistant wheat cultivars were grown (Wan et al. Citation2004; Chen Citation2007a). Having studied pathogen specialization and changes in P. striiformis virulence in the USA when epidemics occurred in the 1990s, Chen et al. (Citation2010) and Markell & Milus (Citation2008) concluded that the old population of races in the USA has been replaced by a new population as a result of new pathotypes migrating into the USA since 2000. A portion of the current population of Pst arose from recombination, but the ‘post-2000’ population still dominates in the USA (Chen et al. Citation2010).
Pathogen specialization and virulence of P. striiformis in Canada was initially studied by Newton et al. (Citation1933), who identified two physiological races collected from the east border region of Saskatchewan. Newton & Johnson (Citation1936) were able to differentiate races between North America and Europe collections using the differential wheat line ‘Chinese 166’, which has the Yr1 gene. They concluded that races from Olds, Alberta were virulent on ‘Chinese 166’, but the European races were not. Meaningful studies on pathogen virulence were not resumed in Alberta until after the stripe rust epidemics of the late 1990s. Su et al. (Citation2003) differentiated 57 isolates collected during 1984 to 2002 from western Canada into 39 Pst races based on 24 wheat cultivar differentials. From the samples collected before 2000, races virulent on ‘Lee’ (Yr7, Yr22, Yr23) and ‘Owens’ (Yr7?) were primarily from Creston, BC. Most of the races collected from the prairies before 2000 were avirulent on the two cultivars, whereas all isolates after 2000 were found to be virulent on both cultivars. In addition, new races detected since 2000 were virulent on both ‘Compare’ (Yr8, Yr19) and ‘Clement’ (Yr9, YrCle).
In two more recent studies (Kumar et al. Citation2012; Holtz et al. Citation2013a), 165 field collections of Puccinia striiformis, primarily from central Alberta during 2007–2011, were identified to be Pst or Psh based on virulence on wheat and barley differential seedlings. Wheat differentials based mainly on the ‘Avocet’ background isogenic lines separated 105 Pst isolates into 21 pathotypes, with 48 isolates (45%) being identified as the most dominant type (Pathotype 7). This pathotype was virulent on 12 wheat differentials including Null, YrA, Yr2, Yr6, Yr7, Yr8, Yr9, Yr17, Yr26, Yr27, Yr31 and Yr32 (YrCV). The second dominant pathotype (14 isolates) was represented by nine isolates (8.6%) that were virulent on 16 wheat differentials. Pathotype 10 consisted of seven isolates that were virulent on 11 wheat differentials. Two pathotypes were represented by six isolates, and three pathotypes consisted of three, four and five isolates. Four pathotypes were represented by two isolates that were virulent on 13–20 wheat differentials. Nine pathotypes each consisted of a single isolate virulent on 9–20 wheat differentials. Differentials Yr5 and Yr15 were resistant to all pathotypes and YrSP was susceptible to only one of the pathotypes tested. Differentials Yr1, Yr10, Yr24 and Yr28 were resistant to 83–92% of the isolates. Differentials YrA, Yr2, Yr6, Yr7, Yr8, Yr9, Yr17, Yr26, Yr27, Yr31 and YrCV were susceptible to 97–100% of the isolates. When 67 of the Pst isolates were tested on seven US wheat cultivars, ‘Moro’, ‘Hyak’ and ‘Tyee’ were resistant to 80–90% of the isolates and ‘Paha’ was resistant to two-thirds of isolates, while ‘Produra’, ‘Stephens’ and ‘Yamhill’ were susceptible to all 67 isolates.
Based on the 12 ‘Avocet’ isogenic lines, there were significantly high correlations in mean virulence frequency among isolate collections from central Alberta and the two US studies (Chen Citation2010, Citation2011) (). The close correlations between central Alberta and these US locations are not surprising, given the potential for long-distance transport of stripe rust inoculum from the PNW of the US and prairie cereal-growing region. Despite being significant, correlations for virulence frequency were much lower between Lacombe, Alberta and other international locations including Chile, China, Pakistan and Turkey (Sharma-Poudyal et al. Citation2013).
Table 1. Correlations of Puccinia striiformis f. sp. tritici virulence frequency by inoculation at the seedling stage under controlled conditions, and disease severity resulting from natural infection at the adult plant stage in trap nurseries, 2007–2012.
Pst field trap nursery
Trap nurseries have been used for studying pathogen variation and evaluating effective genes available at the adult plant stage in multiple regions (Chen et al. Citation2002; Bux et al. Citation2011; Safavi et al. Citation2013; Dalvand et al. Citation2014). In the present study, trap nurseries were set up across central Alberta including Lacombe, Edmonton, Trochu and Olds, and in international locations including Creston, BC, Pullman, WA, Mt. Vernon, WA, and Quito, Ecuador from 2007 to 2012. The Creston site was included in the international nurseries because this site is geographically closer and epidemically similar to the PNW compared with the central Alberta sites. Twenty wheat isogenic lines and one susceptible check (Null) with the ‘Avocet’ background were used in central Alberta and the international trap nurseries. Wheat stripe rust development was scored on the differentials at the adult plant stage when the plants were nearly mature using percentage disease severity (Peterson et al. Citation1948). Low levels of disease development occurred in a few tests that were excluded from the analyses. Consequently, data from 13 and 8 location-year tests in central Alberta and international sites, respectively, were retained for analyses. Statistics for the retained tests are listed in . The levels of disease in the retained tests suggest that there was sufficient but variable disease development among location-years. Although a correlation in mean disease severity between central Alberta and international nurseries was significant (R2 = 0.46**), this coefficient from the trap nurseries was considerably lower () than those in virulence frequency for North America (R2 = 0.96**, R2 = 0.84**). Highly significant correlations in virulence frequency based on the wheat differentials used can be indicative of a close association in race distribution between the two regions, while a low correlation in disease severity may have been caused by different levels of disease severity, different races and variation in expression of disease resistance at the adult plant stage under variable environmental conditions.
Cluster analyses grouped individual genes based on wheat stripe rust reactions at the adult plant stage based on location-years for the two regions. These analyses separated the 21 wheat isogenic lines into two major groups based on disease severity for central Alberta or other locations, respectively ( and ). The group with higher disease severity can be viewed as a group of wheat differential lines for which genes are susceptible or a resistance gene was absent, while the groups with low disease severity can be viewed as a group of wheat differential lines where the genes were effective in conferring resistance to the current stripe rust pathotypes. As such, YrA, Yr6, Yr7 and Yr9 were ineffective genes and the rest were effective genes for central Alberta (). Genes including YrA, Yr2, Yr6, Yr7, Yr9, Yr18, Yr24, Yr26 (YrUnk), Yr28, Yr29 and Yr31 were ineffective ones, while the rest were effective genes for the Creston and international sites ().
Fig. 1 Cluster of wheat differentials based on stripe rust severity at 13 station-years of data in central Alberta during 2007, 2008, 2010 to 2012.
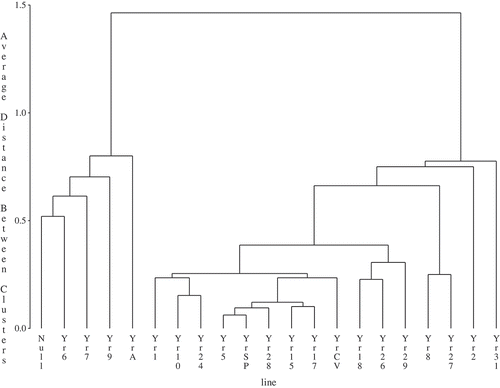
Fig. 2 Cluster of wheat differentials based on stripe rust severity at 8 station-years of data from international and Creston nurseries during 2007, 2008 and 2010.
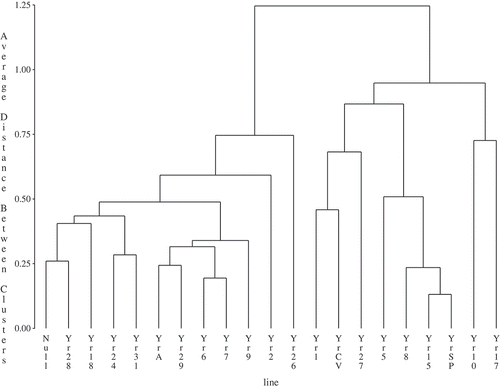
There were more effective genes in central Alberta than other locations based on the analyses. Among the 20 wheat genes screened in the two regions, Yr1, Yr5, Yr8, Yr10, Yr15, Yr17, Yr27, YrCV and YrSP were consistently resistant, while YrA, Yr6, Yr7, Yr9 were consistently susceptible. The rest of the genes including Yr2, Yr18, Yr24, Yr26 (YrUnk), Yr28, Yr29 and Yr31 were variable in disease reactions, depending on the region. It is recognized that inoculum dispersal and disease intensity tend to decrease with increasing distance from the source of inoculum (Mundt et al. Citation2010). Though yet to be thoroughly tested, we anticipate that the relationship between disease gradient and host reactions will apply over a wide range of spatial scales, such as the stripe rust pathway along the PNW and central Alberta. The difference in expression of gene reactions between the two regions was likely caused by the effect of a disease gradient over space and time along the stripe rust pathway. The late arrival of inoculum and possibly a lower spore density in central Alberta results in either incomplete or a fewer number of infection cycles given the relatively short growing season occurring in this region. In addition, late arrival of inoculum means the host is at the adult plant stage where an increase in adult-plant resistance comes into play. Thus, low stripe rust severity that is typically observed in central Alberta may be due to a number of pathogen and host factors, as well as being located near the end of the stripe rust Puccinia pathway compared with higher severity typically observed in the PNW, which traditionally is the source region for Alberta epidemics.
Expression of the genes Yr1, Yr5, Yr10, Yr15 and YrSp at the adult stage in the trap nurseries was consistent with reactions at the seedling stage (). Virulence for Yr1 in ‘Chinese 166’ was first detected in isolates from wheat and grass from Olds, Edmonton and Lethbridge, Alberta in the 1930s (Newton & Johnson Citation1936) and then in 2000 in Creston, British Columbia (Su et al. Citation2003). Pathotypes virulent on Yr1 were recently detected at a low frequency in the samples primarily collected from central Alberta (Kumar et al. Citation2012; Holtz et al. Citation2013a). Line (Citation2002) considered the virulence on ‘Chinese 166’ originated from native grasses, as a race originally collected from triticale on the coast of California. The ‘Avocet’ line with Yr1 showed 90% severity in Pullman, 2008 and Ecuador, 2010 (data not shown), while an average of 5% disease severity was observed in central Alberta trap nurseries. This virulence pathotype and its variants appear to occur in central Alberta, despite being uncommon. Consequently, Yr1 may not be suitable for deployment in cultivars grown in North America including central Alberta.
Genes Yr5 and Yr15 have been highly resistant in most parts of the world (Sharma-Poudyal et al. Citation2013) with only trace levels of disease in the international trap nurseries (). Gene Yr10 is among the few seedling resistance genes employed in winter wheat in western Canada (Randhawa et al. Citation2012). Virulent race(s) for Yr10 were first detected in 1972 in the US (Chen et al. Citation2010). Data from the current trap nurseries showed that despite being classified in the resistant group for both nurseries in central Alberta and abroad, the cultivars/lines with this gene displayed high levels of disease severity at Creston and the PNW. Furthermore, recent tests showed an increase in virulence frequency from 8% (Kumar et al. Citation2012) to 19% (Holtz et al. Citation2013a) in Alberta. Virulence towards YrA, Yr2, Yr6 and Yr7 was widespread in the trap nurseries in Alberta and abroad. These genes are commonly present in bread wheat cultivars from the CIMMYT breeding programme (Dubin & Johnson Citation1989). Despite being classified into the resistant category in the Alberta trap nurseries with a mean of 12% disease severity, Yr2 may have a limited value for stripe rust resistance in central Alberta, given that it is globally ineffective (Stubbs Citation1988). Pathotypes virulent on ‘Fielder’ were also virulent on ‘Heines Kolben’, indicating that there was a common resistance gene, probably Yr6, in the two cultivars (Line Citation2002). A pathotype virulent on ‘Owens’ that may carry Yr7 was first detected in Creston, BC in 1989 (Su et al. Citation2003). Pst pathotypes displayed high levels of virulence frequency for Yr7 and this line was highly susceptible in field nurseries ().
Virulence towards Yr8 and Yr9 were separately detected in western Canada. Su et al. (Citation2003) reported that virulence for Yr9 in ‘Clement’ was detected in the 1984 sample from southern Alberta and for Yr8 in ‘Compair’ was detected in the 1991 and 1993 samples from Creston, BC. The combination of virulence towards the Yr8 and Yr9 was found in western USA in 2000 (Chen et al. Citation2002). Pst pathotypes virulent on both Yr8 and Yr9 are considered to be a characteristic distinctive of a group of invasive populations into North America around 2000 (Markell & Milus Citation2008). Although recent studies in Alberta and the USA confirmed high virulence frequency towards both genes of Yr8 and Yr9 at the seedling stage (Kumar et al. Citation2012; Holtz et al. Citation2013a), there was a substantial difference in disease severity between the two genes in the trap nurseries. This difference in disease severity resulted in their separation into the resistant and susceptible group for both regions ( and ).
There was also a discrepancy in Yr17 reaction between seedling inoculation and trap nurseries (). Yr17 is considered to be a temperature-dependent gene for stripe rust resistance (Bariana & McIntosh Citation1994). In addition to other factors, temperature differences might have been the major factor causing the discrepancy in stripe rust reactions between the seedling tests and trap nurseries. The pathotypes virulent for Yr17 are widespread in the USA (Chen et al. Citation2010). Similarly, virulence frequency for Yr17 from Alberta samples was determined to be nearly 100% (Kumar et al. Citation2012; Holtz et al. Citation2013a). However, this gene was classified to be resistant at the adult plant stage in both central Alberta and the international nurseries ( and ). Virulence towards this gene based on field reactions developed in NW Europe and Australia. Having analysed disease severity of wheat stripe rust in Denmark from 1985 to 1999, Hovmøller (Citation2001) concluded that Yr17 became ineffective in that country. The occurrence of stripe rust was only associated with the resistance in a French wheat VPM1 or in the wheat varieties in Australia (Murray et al. Citation2005; Wellings et al. Citation2007).
Of the 70 stripe rust resistance genes that have been reported in wheat (McIntosh et al. Citation2008), several genes including Yr18 and Yr29 tested in the present study confer adult plant resistance (APR) (McIntosh et al. Citation1995; Singh Citation2007). While Yr31 has been defined as a seedling resistance gene (Dalvand et al. Citation2014), it has also been considered to be an APR gene (Khlestkina et al. Citation2007; Luo et al. Citation2009). Likewise, in the present study, the differential line with Yr31 showed APR gene reactions similar to Yr18 and Yr29 that are typical APR genes (). Thus, more studies are needed to define Yr31. While classified into the susceptible group in the international nurseries (), the three genes were all classified into the resistant group in the central Alberta nurseries (). The two genes, Yr18 and Yr29, are often involved in an APR complex linkage in two pairs, Yr18/Lr34 (Kolmer & Liu Citation2002) and Yr29/Lr46 (William et al. Citation2003), for resistance to stripe as well as leaf rust. The two pairs of linked genes appear to be utilized globally for resistance to both rusts (Singh Citation2007). Although Yr18 alone was found to be ineffective in the area where stripe rust was severe (Ma & Singh Citation1996; McCallum et al. Citation2007b), the resistance conferred from this gene is considered to be durable and likely provides some level of protection to minimize yield reduction (Ma & Singh Citation1996). Thus, the level of resistance in Yr18 may be adequate for the low to moderate stripe rust pressure central Alberta has typically experienced.
While low levels of virulence towards gene Yr24 were identified based on seedling inoculation (), this gene was classified to have a susceptible response at the adult plant stage in trap nurseries at the international locations (), indicating that this seedling resistance gene was ineffective against the predominant pathotypes in North America. Li et al. (Citation2006) suggested that Yr24 and Yr26 are the same genes based on SSR analyses. We designated an isogenic line to be YrUnk in the present study. It was initially believed to possess Yr26, but the gene was later found not to be Yr26 and was different from any known Yr genes (Sharma-Poudyal et al. Citation2013). Virulence towards YrUnk was high in frequency (100%) based on seedling inoculations, while disease severity was also high in the international trap nurseries. In contrast, lines with YrUnk had relatively low disease severity in the trap nurseries in central Alberta (). The drastic difference in virulence frequency between Yr24 and YrUnk suggests they are not the same gene (Kumar et al. Citation2012).
A high frequency of virulence for Yr27 was detected in the present study () due perhaps to the long presence of this gene in Canadian wheat cultivars. ‘Selkirk’ wheat is generally known as a source of Yr27 and is of Canadian origin, although the selection for stripe rust resistance was not intended (McDonald et al. Citation2004). ‘Garnet’ wheat in Manitoba was used to select for ‘McMurachy’ which became part of the pedigree for ‘Selkirk’ (National Plant Germplasm System, USDA-ARS, www.ars-grin.gov). This gene was classified into the resistant group in both regions ( and ), suggesting that it can offer protection for Alberta wheat cultivars provided that disease pressure is low. Similar to Yr24, only a minority of pathotypes from Alberta were virulent for Yr28 at the seedling stage (). Yr28 was found to be resistant in the central Alberta trap nurseries (), but was highly susceptible at other locations (). Singh et al. (Citation2000) reported that this gene conferred both seedling and adult plant resistance, but offered insufficient protection due to being overcome by changes in pathogen virulence. Singh and Rajaram (Citation1994) suggested that this gene be used by combining other slow-rusting genes like Yr18 for durable resistance.
Line YrCV was frequently susceptible at the seedling stage and showed moderate disease severity at the adult plant stage compared with YrSP (), although both differential lines were classified into the resistant group in the central Alberta trap nurseries and the international locations ( and ). A close examination showed that in the trap nurseries, YrCV had high levels of disease severity in Pullman, Mt. Vernon and Ecuador (data not shown). As a result, this gene may not be suitable for central Alberta due to the high level of virulence frequency in this region. The wheat differential ‘Carstens V’ with the YrCV gene was resistant, but later was found to be susceptible to the stripe rust pathogen in Europe (Stubbs Citation1985). Depending on the North American races challenged, one to three genes, YrCV1 to YrCV3, have been identified in ‘Carstens V’ (Chen & Line Citation1993). ‘Spaldings Prolific’ (YrSP) has been resistant to the majority of Albertan pathotypes in several studies (Su et al. Citation2003; Kumar et al. Citation2012; Holtz et al. Citation2013a), and also exhibited resistance in the field nurseries in North America (). This line possesses a single gene, YrSP, resistant to stripe rust races of North America origin (Allen & Purdy Citation1970).
The virulence frequency of P. striiformis for individual resistance genes was similar when samples were analysed from the collections of 13 countries in Europe, Asia, Australia and America (Sharma-Poudyal et al. Citation2013). Widespread deployment of the same resistance genes over large wheat-growing regions contributed to identical and similar virulence frequencies in the Pst populations. However, races virulent for a number of genes including Yr1, Yr5, Yr8 and YrSP have been identified in Australia, but none of these resistance genes had been deployed in commercial cultivars in that region (Wellings Citation2007). Wellings (Citation2007) considered mutation as a possible reason for the occurrence of pathotypes with virulence on these genes. Most important wheat leaf rust races have either evolved through mutations in existing populations or migrated from other unknown areas (Huerta-Espino et al. Citation2011). Somatic recombination is considered to be another potential mechanism for the development of genetic variation in P. striiformis (Stubbs Citation1985). It is possible that new stripe rust races have evolved through either mechanism in the area where resistance genes are absent or deployed only on an insignificant scale. The recent discovery by Jin et al. (Citation2010) demonstrated that Berberis chinensis Poir. was an alternate host for Pst to complete sexual reproduction. Under greenhouse conditions, 18 Berberis species in the epidemics regions in China were identified as alternate hosts of Pst (Zhao et al. Citation2013). It is quite possible that sexual reproduction via an alternate host could facilitate new Pst races to arise.
There was substantial discrepancy between virulence frequency following inoculations at the seedling stage under controlled conditions and disease severity at the adult plant stage in field nurseries. Yr8 is an example where seedling resistance was shown to be overcome, but resistance at the adult plant stage was still evident (). This is indicative of the differential expression of resistance genes between the seedling and adult plant stage. Most seedling resistance genes in the wheat differentials are presently ineffective due to evolution of pathogenic races (Kang et al. Citation2010). An alternative interpretation is that residual resistance may play a role in maintaining a moderate level of resistance after a major gene is partially overcome by virulent pathotypes of P. striiformis (Danial et al. Citation1994). Virulence frequency at the seedling stage was generally higher than disease severity in central Alberta nurseries. This discrepancy may be interpreted from a point of view of epidemiology. Migrating uredinospores are likely to arrive late in the growing season, resulting in fewer infection cycles in central Alberta than southern Alberta and the PNW. Thus, this disease does not have sufficient time to fully develop and a low disease severity results by the end of the season in central Alberta. However, late arriving inoculum can be sampled and the virulence of pathotypes determined based on disease reactions at the seedling stage using greenhouse inoculations (Kumar et al. Citation2013; Holtz et al. Citation2013a). As a result, pathotypes can be identified, but this likely does not take epidemiological significance into consideration for central Alberta conditions. The hypothesis about the disassociation between virulence pathotypes and disease severity can be tested by repeated sampling and spore trapping in central Alberta. In light of the substantial differences observed between virulence frequency and disease severity, either type of data alone should be interpreted with caution. The determination of virulence frequency serves as a prediction for potential changes in pathotypes for central Alberta. Furthermore, on-going trap nurseries should be used to monitor the stripe rust situation under field conditions. Consequently, the use of both approaches should be complementary to each other in characterizing stripe rust situations in relation to host resistance for central Alberta.
Psh virulence study
Based on early studies, the causal agents of barley stripe rust were likely to be grouped into those causing wheat stripe rust due to a limited understanding of the pathogen and few available tools for classification. Early reports showed that Canadian isolates, including those from barley cultivars, were not considered to be the barley stripe rust pathogen, but were suggested to be the wheat stripe rust pathogen or related forms (Newton et al. Citation1933). In North and South America, the causal agent of barley stripe rust was not systematically studied until the mid-1970s when outbreaks of barley stripe rust were reported near Bogota, Colombia, causing losses of 30–70% in grain yield (Dubin & Stubbs Citation1986). There have been a few studies on the physiological specialization of Psh since barley stripe rust was first observed in Texas. The dominant Psh race with its variants was considered to have originated from European race 24 (Dubin & Stubbs Citation1986). Marshall & Sutton (Citation1995) identified three races in south-central USA, based on a total of 273 isolates tested. By 2009, Wan & Chen (Citation2012) identified 82 Psh pathotypes in the USA using 12 barley differentials. Severe stripe rust has been regularly observed in barley breeding plots in Lacombe, and sporadic stripe rust has been observed in barley commercial fields since 2000 (Xi et al. Citation2011). There was an unconfirmed report on outbreaks of barley stripe rust in commercial fields in southern Alberta in 2011 when epidemics of wheat stripe rust also occurred (Shaw, personal communication). Given the potential impact in Alberta, barley stripe rust was for the first time studied as intensively as wheat (Kumar et al. Citation2012; Holtz et al. Citation2013a, Citation2013b).
In Alberta, information on the virulence dynamics of Psh in relation to host resistance is rather limited. Sixty isolates collected in Alberta were recently identified to 21 Psh pathotypes, with virulence on 2–9 of the 12 barley differentials (Kumar et al. Citation2012; Holtz et al. Citation2013a). Eighteen isolates (30%) were represented by two Psh pathotypes (Pathotypes 2 and 18) with virulence on 8 and 4 barley differentials, respectively. Pathotype 2 was virulent on the differentials including ‘Topper’, ‘Hiproly’, ‘Varunda’, ‘Abed Binder 12’, ‘Trumpf’, ‘Mazurka’, ‘Bigo’ and ‘Bancroft’. Pathotype 18 was virulent on differentials including ‘Topper’, ‘Hiproly’, ‘Abed Binder 12’ and ‘Trumpf’. The remaining pathotypes were each represented by one to five isolates. Barley differentials ‘Heils Franken’, ‘Emir’ and ‘Astrix’ were resistant to all Psh pathotypes (). Barley differentials ‘I 5’, ‘Varunda’ and ‘Bancroft’ were resistant to 55–90% of Psh isolates, while ‘Topper’, ‘Hiproly’, ‘Abed Binder 12’, ‘Trumpf’, ‘Mazurka’ and ‘Bigo’ were susceptible to 57–100% of isolates (). Eighteen Psh isolates grouped into five pathotypes which were unique as they exhibited virulence towards wheat isogenic lines, YrA, Yr1, Yr6, YrUnk, Yr31 and a wheat cultivar differential, ‘Stephens’ (Yr3a, YrS, YrSte). The wheat isogenic line ‘Avocet S’ (Null) and winter wheat ‘AC Bellatrix’ were susceptible to most Psh isolates (Holtz et al. Citation2013a).
Table 2. Correlations of virulence frequency of Puccinia striiformis f. sp. hordei between central Alberta and the USA based on seedling inoculation.
Despite being significant, correlations were considerably lower in Psh than Pst virulence frequency between the central Alberta (Lacombe) and USA studies (Chen Citation2010, Citation2011) ( and ). Psh pathotypes with a relatively narrow spectrum of virulence tended to be more predominant (Chen Citation2010, Citation2011). The low selection pressure from the barley cultivars with narrow range of sources of resistance resulted in the dominance of the Psh with a narrow virulence spectrum (Chen Citation2007a). Line (Citation2002) held a similar view that the lack of resistant barley commercial cultivars in North America and susceptibility of wild Hordeum species were the major factors contributing to less diversity in Psh virulence. However, more samples are needed for analyses before a reliable comparison of Psh from both countries can be made.
Climatic conditions especially summer temperatures in Alberta appear to provide a better environment for Psh to oversummer than in the USA, as barley stripe rust is favoured by cool conditions around 15°C (Tekauz Citation2003), which is lower than the temperature range for Pst (Roelfs et al. Citation1992). Preliminary results showed that temperatures for spore germination and infection initiation were considerably lower for Psh than Pst when the recent collections of the two formae speciales from Alberta were compared (Holtz et al. Citation2011, Citation2013c). Studies are needed to determine if the low summer temperature in central Alberta played a role in the enhanced diversity and virulence for Psh.
Barley stripe rust was monitored using the 12 barley differentials in the central Alberta and international trap nurseries under natural infection conditions. None of the barley differentials showed substantial disease except for the susceptible checks in the nurseries. Similar to the Pst situation, we observed the difference between Psh virulence frequency at the seedling stage and disease severity in the field nurseries. No data from the nurseries are reported in this paper.
Forma specialis and relationship between Pst and Psh
Eriksson first suggested the subdivision of the stripe rust pathogen into five formae speciales in P. glumarum (Eriksson 1894 cited from Chen Citation2005). Newton et al. (Citation1933) disagreed with the concept of the subdivision due to overlapping host range between wheat and barley. In their study, an isolate from ‘O.A.C. 21’ barley sampled from Olds, Alberta was virulent on wheat differential seedlings. In a subsequent test, three of nine barley varieties at the seedling stage were susceptible to four physiological forms of Pst by inoculation (Newton & Johnson Citation1936). Line (Citation2002) reported that the rust on barley observed in western states of the USA was always identified as Pst. Until 1975 near Bogota, Columbia, Dubin & Stubbs (Citation1986) identified Psh race 24 and its variants as the cause of the outbreaks of barley stripe rust. Puccinia striiformis has been frequently found on H. jubatum since the first discovery of stripe rust in Alberta. Furthermore, it was only recently that isolates from H. jubatum in Alberta have been identified to Pst or Psh (Holtz et al. Citation2013a).
Based on pathogenicity phenotyping, there now seems to be general consensus among researchers for the division between Pst and Psh (Chen et al. Citation1995; Kumar et al. Citation2012; Holtz et al. Citation2013a, Citation2013b), which are the most economically important formae speciales in P. striiformis. Overlapping pathogenicity between the two formae speciales has been previously reported in North America (Chen et al. Citation1995). Based on seedling inoculation tests, the majority of Pst and Psh pathotypes from the Alberta collections were virulent on highly susceptible wheat and barley cultivars/genotypes, such as ‘AC Bellatrix’ wheat, ‘Mahigan’ and HB522 barley (Kumar et al. Citation2012; Holtz et al. Citation2013a). Recently, the concept for the subdivision into forma specialis has been again questioned as a result of the overlapping host range, particularly between wheat and barley (Hovmøller et al. Citation2011). DNA markers were used to separate the two formae speciales, where Chen et al. (Citation1995) clarified the relationships of P. striiformis f. sp. hordei, P. striiformis f. sp. tritici and P. striiformis f. sp. poae, based on random-amplified polymorphic DNA (RAPD) analyses. They found that Psh and Pst were more closely related to each other than they were to other formae speciales such as P. striiformis f. sp. poae, which was reclassified as P. pseudostriiformis (Tollenaar Citation1967; Liu & Hambleton Citation2010). Holtz et al. (Citation2013b) assessed the genetic diversity of P. striiformis using 11 simple sequence repeat (SSR) markers and examining a length polymorphism in the ribosomal DNA (rDNA) intergenic spacer 1 (IGS1) region. Using a total of 261 isolates primarily sampled from Alberta, they separated genotypes belonging to Pst from Psh isolates with the exception of the two US Pst pathotypes. One group of isolates collected mainly from barley in Alberta was identified to be Psh. This group of Psh isolates is considered to be the result of hybridization between Pst and Psh. This is because these isolates were virulent to barley as well as a number of wheat lines and cultivars, but genetically more closely related to Pst than Psh based on SSR markers (Holtz et al. Citation2013b). The significance of these hybrid isolates in epidemics remains to be determined.
A pathogen, commonly referred to as barley grass stripe rust (BGSR), causing natural infection on certain barley cultivars and lines was identified as pseudo-hordei (Psp-h) in Australia (Wellings et al. Citation2000a, Citation2000b). This form differs from both Pst and Psh in that it was avirulent to most wheat differentials evaluated with the exception of Yr1 in cultivar ‘Chinese 166’. Further studies demonstrated the unique molecular phenotypes of BGSR isolates compared with Australian Pst pathotypes (Keiper et al. Citation2003). Psp-h was found to be more closely related to Pst than Psh based on SSR markers (Spackman et al. Citation2010). Holtz et al. (Citation2013b) concluded that hybrid isolates from Alberta are not the Australian Psp-h isolates, as no unique alleles to either Pst or Psh were detected in these isolates. Safavi et al. (Citation2013) reported the occurrence of P. striiformis isolates virulent for Yr1 in some provinces of Iran. Psh virulence for the Yr1 wheat differential in the ‘Avocet’ background was also observed in the two Alberta studies (Kumar et al. Citation2012; Holtz et al. Citation2013a). Regarding the similarity in virulence towards Yr1 from Psp-h in Australia (Wellings Citation2011) and from Alberta Psh (Kumar et al. Citation2012), Safavi et al. (Citation2013) concluded that the isolates including Alberta Psh could be Psp-h because they were virulent on Yr1. A direct comparison using molecular markers may determine the classification of forma specialis in these isolates. Psh was more diverse than Pst within Alberta (Holtz et al. Citation2013a). The lack of diversity for Pst was interpreted as the replacement of pre-2000 genotypes with the more aggressive post-2000 genotypes (Holtz et al. Citation2013a).
Wheat genetic resistance used in western Canada
It has been known for a long time that winter wheat is more susceptible than spring wheat in Alberta and British Columbia. Screening at the Provincial School of Agriculture, Olds, AB in 1928 showed that the majority of 64 spring wheat varieties were resistant to stripe rust (Sanford & Broadfoot Citation1929). Sanford & Broadfoot (Citation1932) concluded that stripe rust did not represent an economic problem in Alberta, mainly because spring wheat varieties commonly grown were resistant. Foster (Citation1937) reported that there was a range of disease reactions in 26 winter wheat varieties evaluated at Sidney, BC. ‘Egyptian Amber’ was severely diseased and ‘Baldwin’ was immune to stripe rust in the test.
Wheat stripe rust has been an annual production problem under irrigation in southern Alberta since the early 1980s, as a result of a susceptible cultivar ‘Fielder’, a soft white spring wheat being widely grown in the region (Conner & Kuzyk Citation1988). The resistant cultivar ‘Owens’ that may carry Yr7 was licensed in the mid-1980s, but had a limited acreage in the region due to poor agronomic performance (Conner & Kuzyk Citation1988). The reaction of ‘Owens’ to stripe rust races is identical to that of ‘Thatcher’ (H. Su & R.L. Conner, unpublished data, cited from Su et al. Citation2003). The resistance can be traced back to ‘Marquis’, an ancestor of ‘Thatcher’, which carries Yr7 (McIntosh et al. Citation1995). The development of stripe rust was slowed down and this was attributed to the use of resistant ‘AC Reed’ (Su et al. Citation2003). This is a soft white spring wheat which was grown on more than 90% of the wheat acreage in southern Alberta before 2000 (Sadasivaiah et al. Citation1993; Su et al. Citation2003). The current dominant soft white spring wheat is ‘AC Andrew’ and it is resistant to stripe rust (McCallum & DePauw Citation2008). Genetic resistance to stripe rust was known to have been incorporated into ‘AC Reed’ (Sadasivaiah et al. Citation1993) and ‘AC Andrew’ (Sadasivaiah et al. Citation2004). Randhawa et al. (Citation2012) reported that the two cultivars were negative for the molecular markers of any resistance genes that were identified in the western Canadian cultivars.
By 2010, increasing virulence towards winter wheat cultivar ‘Radiant’ (Canada Western Red Winter, CWRW) carrying Yr10 was observed in southern Alberta (Puchalski & Gaudet Citation2011). Although virulence frequency towards Yr10 was relatively low (), stripe rust severity of 25% was found in ‘Radiant’ in a recent field test located in central Alberta (K. Xi, unpublished data). Thus, this gene may no longer be effective if stripe rust epidemics occur in central Alberta. Several winter wheat cultivars including ‘Emerson’ (CWRW) and ‘McClintock’ (CWRW) are resistant to stripe rust in Alberta and they are known to possess the resistance gene Yr17 (Graf et al. Citation2013). Spring wheat cultivars with a few known genes for stripe rust resistance including Yr17, Yr18 or Yr36 have been grown in western Canada (Randhawa et al. Citation2012). The presence of Yr17 in the Canada Western Red Spring (CWRS) wheat cultivar ‘CDC Stanley’ has been demonstrated (Randhawa et al. Citation2012). In the present study, this cultivar was susceptible at the seedling stage and moderately resistant at the adult plant stage ().
Yr18 was widespread among all of the Canadian spring wheat classes except Canada Western Soft White Spring (CWSWS), Canada Western Amber Durum (CWAD) and triticale (McCallum & DePauw Citation2008). This gene may have been introduced into wheat cultivars by western Canadian wheat breeding programmes due to its tight linkage with the Lr34 gene for leaf rust resistance (McCallum & DePauw Citation2008). All Canada Western Extra Strong (CWES) varieties are derived from crosses with ‘Glenlea’ which carries Yr18 (McCallum & DePauw Citation2008). McCallum et al. (Citation2007b) reported that many of the 28 spring wheat cultivars commonly grown in western Canada were moderately resistant when screened in international stripe rust nurseries. The most popular ‘AC Barrie’ (CWRS) was susceptible (McCallum et al. Citation2007b), while the susceptible ‘AC Crystal’ [Canada Prairie Spring (CPS)] was one of the leading CPS cultivars from 2000 to 2005 and in 2010 in western Canada (McCallum & DePauw Citation2008; Randhawa et al. Citation2012). No resistance genes have been detected in these two susceptible cultivars (McCallum & DePauw Citation2008). With only a few stripe rust resistance genes in western Canadian wheat cultivars, more genes need to be incorporated to improve resistance. Genes such as Lr67/Yr46 could be good candidates to use (Fetch et al. Citation2011), as like the Lr34/Yr18 locus, they condition resistance to both leaf and stripe rust (Hiebert et al. Citation2010).
Recent screening experiments at the seedling stage showed that there were few detectable all-stage resistances in 64 wheat or 10 triticale cultivars to Pst isolates found in Alberta, with no cultivars being resistant to all isolates (Holtz et al. Citation2013c). The lack of all-stage resistance in western Canadian wheat cultivars to stripe rust suggests that the adult plant resistance plays a major role under field conditions. Furthermore, the majority of CWRS, CWES and CWRW generic wheat were susceptible to Psh at the seedling stage, particularly for a group of isolates that likely resulted from hybridization between Psh and Pst. This is suggestive of the lack of all-stage resistance in both wheat and barley to hybrid isolates of P. striiformis. The reactions of both crops at the adult plant stage to these hybrid isolates remains to be determined.
Barley resistance in western Canada
Information on stripe rust genetic resistance in barley cultivars and germplasm lines is limited compared with the vast literature on wheat genetics and resistance. Chen et al. (Citation1994) published the first genetic study on barley stripe rust in North America. In that study, they mapped two quantitative trait loci (QTL) that conferred resistance to stripe rust in a six-row spring barley line. Subsequently, Chen & Line (Citation1999, Citation2002) identified at least 20 resistance genes in 18 barley genotypes. Most of these genes were found to be recessive (Chen & Line Citation1999, Citation2002; Yan & Chen Citation2007). Recessive genes in some of these barley genotypes were reported earlier in Europe (Nover & Scholz Citation1969). Research including mapping populations of barley resistance to stripe rust has been carried out (Hayes et al. Citation2001). A QTL for high temperature adult plant (HTAP) resistance to Psh was identified in the two-row feed barley cultivar ‘Bancroft’ (Rossi et al. Citation2006; Yan & Chen Citation2008). The barley cultivar ‘Baronesse’ has a moderate level of non-race-specific HTAP resistance to stripe rust and is one of the most widely grown cultivars in the USA (Anon. Citation2009a).
To aid in the development of resistant barley that is agronomically suitable for western Canada, spring barley lines were recently screened for resistance to American Psh pathotypes in international nurseries at Pullman and Mt. Vernon, WA, and Ecuador (Xi et al. Citation2013). A total of 1357 entries, mainly consisting of hulled two-row, hulled six-row and hulless barley from the Field Crop Development Centre (FCDC) breeding programme in Lacombe, Alberta, were evaluated during 2007 to 2009. Over 40% of the hulled two-row types of barley lines were resistant compared with only 9% and 7% of the six-row and hulless type lines, respectively. Moreover, significant correlations between infection type (IT) and disease severity (DS) suggest that either can be used for an assessment of stripe rust reaction without a significant loss of information. Since both IT and DS assessments had high loadings on the first principal component compared with the rest of components, and the two assessments were significantly correlated, the first principal component values alone can be used for the selection of resistant barley lines (Xi et al. Citation2013).
While substantial numbers of advanced lines and germplasm from the FCDC breeding programme were resistant, among 30 cultivars primarily from this programme that were screened, only the two-row cultivar ‘Seebe’ (Helm et al. Citation1996) was consistently resistant in the international nurseries (Xi et al. Citation2011). Information on the genetic resistance in these FCDC barley lines is largely unavailable. Further work is needed to investigate the genetic resistance of the FCDC barley lines identified as resistant phenotypes in the international nurseries. Cross infection of barley by Pst and Psh pathotypes representative of the pathogen population found in Alberta was investigated using seedling inoculation of 47 barley cultivars (M. Holtz, unpublished observations). These barley cultivars represented advanced lines from the barley breeding programmes in western Canada. The majority of cultivars were susceptible to all Psh isolates and no cultivar was resistant to all Psh isolates. Most two-row barley cultivars were resistant to all Pst isolates, whereas the majority of 6-row barleys were susceptible to multiple Pst isolates.
Currently, barley stripe rust severity has been low in Alberta. Lower stripe rust severity in barley than in wheat in Alberta may be attributed to a number of factors. The small winter barley acreage in the US PNW may result in fewer Psh urediniospores migrating to Alberta. In addition, there was a larger acreage seeded to two-row barley than six-row barley varieties in western Canada (Anon. Citation2011b). Observations suggest that two-row cultivars were generally more resistant than six-row cultivars in commercial fields. However, differences between two- and six-row cultivars needs to be experimentally determined where both Psh or Pst, as well as the hybrid isolates, are used to screen barley cultivars at the adult plant stage.
Puccinia striiformis overwintering in central Alberta
Stripe rust is wind-dispersed via airborne urediniospores in a relatively cool temperature environment (Line Citation2002). The pathogen is an obligate parasite that only infects and survives in a living host (Agrios Citation1978). The fungus relies on migrating urediniopores for overwintering or oversummering to complete its life cycle. This allows the pathogen to overwinter in warmer southern regions and oversummer in cooler northern regions for infection and sporulation. During the summer, high levels of stripe rust have often been observed in winter wheat in southern Alberta (Conner et al. Citation1988) and central Alberta (Xi et al. Citation2011). Overwintering of stripe rust was observed in winter wheat in late April in southern Alberta and likely contributed to seedling infections in spring wheat from 2011 to 2013 (Puchalski et al. Citation2013). Overwintering of stripe rust may be one of the factors causing the breakdown of the resistance gene Yr10 in winter wheat ‘Radiant’ in Alberta. The breakdown of winter wheat resistance to leaf rust (P. triticina) in the southern USA was faster than in spring wheat (Long et al. Citation1992). McDonald & Linde (Citation2002) suggested a combination of several factors including high gene/genotype flow and large effective population sizes of the pathogen over the wheat crop for a long period of time may explain the more rapid breakdown of resistance in winter wheat versus spring wheat.
There seem to be conflicting views as to whether P. striiformis overwinters in winter wheat in Alberta, and the role viable overwintering inoculum plays for disease development in subsequent crops in Alberta. The earliest observation on overwintering of stripe rust in western Canada was made as soon as stripe rust was found in this region. Sanford & Broadfoot (Citation1929) reported that stripe rust could survive at least some winters on grasses in Alberta. Later, Sanford & Broadfoot (Citation1932) concluded that it was unlikely that stripe perpetuated from one season to another by dormant mycelium. Similarly, stripe rust on heavily infected wheat late in October was found not to develop in April and May the next year (Anon. Citation1932). This was perhaps because the low ambient winter temperatures that prevailed in western Canada were believed to kill the fungus, thus preventing overwintering (Rapilly Citation1979). The PNW is considered to be the major source of inoculum that migrates to and cause epidemics in Alberta (Newton & Johnson Citation1936; Su et al. Citation2003; Chen Citation2005). However, stripe rust epidemics on soft white spring wheat were attributed to overwintering inoculum in the Lethbridge area of Alberta (Conner et al. Citation1988). Epidemics in 2006 and 2011 were also attributed to overwintering of the pathogen in southern Alberta (D. Gaudet, unpublished results, cited from Randhawa et al. Citation2012). While the cause of the epidemics in southern Saskatchewan in 2012 was unknown, high inoculum loads observed in winter wheat in the autumn of 2011 may have contributed to the disease outbreak in 2012 (Gehl, personal communication).
Weather conditions play an important role in the overwintering of inoculum associated with stripe rust epidemics in Alberta. A comparison of winter ambient temperatures showed that outbreaks of stripe rust were inversely related to negative degree days in December and January (Conner et al. Citation1988). The amount of snow cover during the winter could directly affect pathogen survival in southern Alberta (Conner et al. Citation1988). Sufficient snow cover is essential for winter wheat to overwinter in Alberta (Anon. Citation2012). As an obligate pathogen, survival of the fungus is contingent on survival of the host. Conditions that result in winter kill to the host will also result in the mortality of the fungus, whereas survival of living tissue represents a potential opportunity for the fungus to survive. The potential for overwintering of P. striiformis on winter wheat was studied in Lacombe, Alberta during 2007–2011 using a combination of laboratory and field experiments (Kumar et al. Citation2013). Experimental results demonstrated that under central Alberta conditions, snow cover was more important than ambient temperature for winter survival of the fungus due to the biotrophic relationship between the fungus and winter wheat. Persistent snow cover was found to be critical for the survival of overwintering inoculum as viable pustules, and urediniospores rarely survived after snow melt. Kumar et al. (Citation2013) found that approximately 4–20% of urediniospores sampled from pustules on winter wheat during January to April of each year were viable. Latent mycelia were intermittently observed on winter wheat sampled from January to May in each of 2008 and 2010. The fungus was detected using PCR intermittently from winter to early spring (Kumar et al. Citation2013).
The role of overwintering inoculum in causing stripe rust was also determined in Lacombe by comparison of disease severity in spring wheat and barley, and between winter wheat and spring wheat (Kumar et al. Citation2013). There was consistently higher stripe rust severity in spring wheat or barley seeded near winter wheat compared with the same crops seeded near spring wheat for all years of field testing during 2008 to 2011. This is indicative of the potential for overwintering of inoculum on winter wheat that contributed to elevated disease severity in spring crops seeded near winter wheat compared with spring wheat. Kumar et al. (Citation2013) recognized that in addition to the overwintering inoculum, there might be the early arrival of exogent inoculum that caused early infection and thus, increased disease severity in the spring crops. Although the relative importance of overwintering inoculum versus exogenous inoculum was not determined in these studies, the importance of overwintering inoculum in epidemics should not be overlooked. The role winter wheat crop plays in inoculum transmission and subsequent stripe rust in spring crops can be described as a ‘green bridge’ phenomenon.
Winter cereals are considered to be the cause of a ‘green bridge’ for pest and disease problems in Saskatchewan (Anon. Citation2010a). Spring and winter wheat crops overlap in central Alberta, and thus, a green bridge may be present in this region. Average maturity dates for susceptible spring wheat cultivars ‘AC Crystal’ and ‘AC Barrie’ are 10 and 5 September, respectively, in Lacombe (Anon. Citation2012). Additionally, spring wheat matures later and earlier seeding of winter wheat is required in central Alberta than in southern Alberta (Anon. Citation2007, Citation2012). Substantial numbers of commercial winter wheat fields were seeded around 1 September 2011, based on surveys conducted in central Alberta (Xi et al. Citation2011). The overlapping of spring wheat maturity dates for spring wheat with the seeding dates for winter wheat suggests that green tissue from both crops may exist at the same time. Furthermore, volunteer spring wheat and foxtail barley bearing stripe rust pustules were extensively present in the same autumn period (K. Xi, unpublished observations). Surveys in central Alberta also showed that nearly all winter wheat fields seeded before 5 September were infected by stripe rust, while very few winter wheat fields seeded after this date were infected (Xi et al. Citation2011). The difference in disease incidence between the two winter wheat seeding dates is suggestive of inoculum transmission from overlapping crops during early September. These obervations suggest that there is a potential green bridge for inoculum survival and its transmission in central Alberta.
Integrated control strategies
An integrated approach using appropriate cultural practices and cultivar resistance could help to limit the risk of stripe rust infection. Brown et al. (Citation2001) cited several examples of successful control by earlier planting in areas prone to annual epidemics of barley stripe rust in the mountains of Bolivia. As airborne disseminated inoculum, stripe rust can spread quickly to a large area in a short period of time, and thus cultural practices such as crop rotation are not considered to be suitable for stripe rust management. However, disease management is possible by the choice of not planting spring wheat adjacent to winter wheat where stripe rust was observed the previous autumn and there were indications that the pathogen has successfully overwintered. Moreover, planting winter wheat adjacent to infected spring wheat can also increase the risk of autumn infections in the emerging winter wheat crop in central Alberta. Stripe rust can be variable in occurrence and impact in central Alberta given the distance from exogenous inoculum sources, e.g. the PNW, variability in the development of stripe rust in these source locations, and variable dispersal and transport of inoculum. In addition, the potential for overwintering in central Alberta represents an important source of local inoculum. Thus, the choice of field planting location may be effective for disease management in this region. Specifically, spring wheat should not be planted adjacent to winter wheat fields, so that the impact of a green bridge for transmission of stripe rust inoculum from winter wheat to spring wheat can be minimized. Delaying winter wheat seeding until spring wheat has fully matured while controlling volunteer spring wheat and grassy hosts may also break the green bridge effect. Avoiding early planting of winter wheat is recommended to escape stripe rust infection in the PNW region (http://striperust.wsu.edu/index.html). However, in central Alberta late seeding of winter wheat may limit plant development in the autumn, leading to winter survival issues and subsequent yield reductions the following year (Anon. Citation2012).
Fungicide application represents an effective short-term strategy when resistant varieties are unavailable. Fungicides may be used in combination with cultivar resistance to enhance stripe rust control if there is a risk for of severe disease development. Seed treatment has been extensively tested in the PNW (Chen & Wood Citation2006; Chen Citation2007b) and used in Australia (Anon. Citation2009b) for management of stripe rust at the seedling stage and thus may reduce the amount of inoculum available for disease development later in the growing season. The active ingredient flutriafol is registered for application as an ‘in furrow’ treatment in Australia (Anon. Citation2009b). This treatment provides an alternative to an early foliar-applied fungicide to moderately susceptible or moderately resistant varieties. Several foliar-applied fungicides have been registered for control of stripe rust in cereals in Alberta (Anon. Citation2013b). Conner & Kuzyk (Citation1988) demonstrated in southern Alberta that the foliar fungicides propiconazole, triadimefon and fenpropimorph when applied before head emergence provided sufficient control of stripe rust and leaf rust and increased yield by 16–79% in soft white spring wheat. In contrast, the foliar fungicides oxycarboxin, chlorothalonil, mancozeb and the seed treatment fungicide triadimenol had limited effectiveness in controlling these rusts and did not reduce yield losses. A recent test in southern Alberta showed that while no significant increase in yield due to stripe rust control was observed on susceptible ‘AC Barrie’, foliar-applied Folicur® (tebuconazole) increased height, maturity, test weight and thousand kernel weight (Gaudet et al. Citation2013).
Information on fungicide efficacy in relation to spray timing and varietal response for stripe rust control is limited in central Alberta. At Lacombe in 2012 under low to moderate stripe rust pressure caused by natural infection, Tilt® (propiconazole) was foliar-applied at the early heading stage to seven spring wheat cultivars belonging to different classes and three barley cultivars/lines in a field experiment (K. Xi, unpublished data). A single fungicide application significantly reduced stripe rust severity in the susceptible wheat cultivars. The fungicide application also increased grain yield by 15 to 23% and thousand kernel weight (TKW) by 8–10% in the three susceptible wheat varieties. In contrast there was no reduction of disease severity or significant yield response for resistant or intermediate resistant wheat cultivars. Results from this trial suggest that the use of resistant or intermediate cultivars of spring wheat provides adequate protection, which is similar to the benefit of foliar fungicide application on susceptible cultivars exposed to low to moderate stripe rust pressure in central Alberta. No significant yield or TKW increase was observed in resistant ‘Seebe’ barley or a susceptible barley line H98077001, while susceptible ‘Mahigan’ barley showed significant (~10%) TKW increase. The lack of substantial barley stripe rust development in the test might have caused the difference in TKW response between the susceptible barley cultivars/lines. Currently, fungicide efficacy tests using both spring wheat and barley cultivars/lines are being repeated in central Alberta.
Stripe rust in wheat was substantially less severe in 2012 compared with 2011 in central Alberta. The fungicide test in 2012 in central Alberta demonstrated that despite low to moderate levels of stripe rust in susceptible spring wheat cultivars (40–60% disease severity), yield losses could be substantial without effective control measures. In central Alberta, there is a late onset of disease, while there are preharvest interval restrictions for many fungicides (Anon. Citation2013b), which may result in the ability to only use one spray during the season. Given the short spray window and rapid disease development in susceptible spring wheat, we recommend fungicides be applied at the first sign of pustules on susceptible spring wheat cultivars if disease occurs at or before the early heading stage. Timely scouting followed by fungicide application at the appropriate plant growth stage is critical for effective stripe rust management. Depending on the year and conditions, stripe rust appearance in susceptible spring wheat may occur prior to the flag leaf emergence stage and under these conditions an immediate fungicide application will help to limit further inoculum production from within the spring wheat field. A subsequent application of fungicide from flag leaf emergence to anthesis will help to limit the impact of stripe rust on the upper canopy leaves when disease pressure is high and/or the risk of exogenous inoculum is elevated. Control measures should also be employed on susceptible winter wheat as stripe rust is generally more severe in winter wheat than spring wheat in central Alberta. Stripe rust has been found to typically appear at the early flowering stage in winter wheat during early to mid-June and reach a maximum disease severity in late July in central Alberta (K. Xi, unpublished observations). The efficacy of foliar fungicide or seed treatment should be evaluated against autumn and spring/summer stripe rust development in winter wheat under central Alberta conditions.
Concluding remarks
Stripe rust is becoming increasingly important for cereal production in Alberta due to increasingly mild winters, use of susceptible cultivars, and the potential for successful overwintering of inoculum with adequate snow cover. In some cases, the observed high disease levels were the result of resistance breakdown, such as the case with the Yr10 gene due to changes in pathogen virulence. A long-term strategy for stripe rust management will require the deployment of both seedling resistance and APR in winter and spring wheat in order to reduce overall levels of stripe rust inoculum over-wintering. Given the relatively low levels of disease severity in central Alberta, the resistance genes, especially those APR genes such as Yr18 in many spring wheat cultivars, may provide satisfactory protection against stripe rust. These resistance genes may potentially have a longer lifespan in this region due to relatively lower stripe rust severities and late arrival of inoculum. In central Alberta, field trap nurseries are essential to determine the performance of resistance genes in the cultivars under field conditions. Data from central Alberta also suggest that virulence identification or field trap nurseries when used individually can provide an adequate picture of the stripe rust pathogen. In central Alberta, the use of annual pathogen virulence testing and trap nurseries will provide comprehensive information on pathogen variability that can be used to update annual varietal recommendations and identify effective genes for breeding purposes. To improve the methodology for evaluating APR to stripe rust in wheat, adult plant stage testing for stripe rust resistance needs to be evaluated under controlled environmental conditions (Pretorius et al. Citation2007).
Given that both wheat and barley are major crops in central Alberta, hybrid populations of Pst and Psh may need to be closely monitored. Seedling inoculation and PCR markers should be used to identify pathogen phenotype and genotype, and field tests should examine the epidemiological significance of these Pst/Psh-hybrids. Although barley stripe rust severity in Alberta has generally been lower than wheat stripe rust, disease outbreaks occurred in individual commercial fields and yield losses were demonstrated using highly susceptible barley cultivars/lines in experimental plots (K. Xi, unpublished data). The growth of highly susceptible cultivars should be avoided to limit the potential for development of barley stripe rust and the production of inoculum, while barley cultivars from western Canada breeding programmes should be evaluated for stripe rust reactions.
Despite relatively low stripe rust severity in central Alberta, field tests demonstrated that it can still cause significant yield losses in susceptible spring wheat in this region. In addition, large acreages of wheat have been seeded to susceptible spring wheat such as ‘AC Barrie’ and ‘AC Crystal’ in western Canada (McCallum & DePauw Citation2008). Consequently, the economic impact of this disease in both winter and spring wheat may have been underestimated for this region. Winter wheat has generally showed higher levels of stripe rust than spring wheat due to overwintering inoculum and only a few resistant cultivars are available. Thus, yield losses due to stripe rust in winter wheat have likely been higher than spring wheat. Fungicide application provides a last defence for yield protection when susceptible wheat cultivars are grown. Moreover, in winter wheat, fungicide application or seed treatment can limit disease development in the early spring, and thus stripe rust inoculum levels for spring cereal crops. However, for spring wheat with a late onset of disease and the short growing season in central Alberta, there can be a relatively narrow window for foliar fungicide application. As a result, to improve the efficacy and benefit of fungicide, there is a need for the implementation of a monitoring and disease alert system for the entire region and diligent scouting of individual fields for disease onset and development. The efficacy of seed treatment and in-furrow application using systemic fungicides should be evaluated to reduce early season disease development and inoculum production.
The use of stripe rust resistant winter and spring wheat cultivars is the most effective disease management strategy. Stripe rust reactions for spring and winter wheat cultivars from western Canadian wheat breeding programmes have been annually updated (Anon. Citation2013a). Proximity to infected winter cereal fields may also increase the risk of stripe rust in adjacent spring cereal crops. Avoiding planting susceptible spring wheat adjacent to winter wheat fields may help to break the green bridge effect by limiting inoculum availability and delaying disease appearance and development. Ultimately, an integrated approach using resistance and appropriate cultural practices plus the judicial use of fungicide will help to minimize stripe rust damage in central Alberta.
Acknowledgements
The authors wish to thank S. Albers, M. Aljarrah, C. Bergen, J. Busaan, L. Langford, B. Smith and L. Vandermaar for valuable assistance. This project was supported by Alberta Crop Industry Development Fund.
References
- Agrios GN. 1978. Plant Pathology. 2nd ed. New York, Academic Press; p. 703.
- Allan RE, Purdy LH. 1970. Reaction of F2 seedlings of several crosses of susceptible and resistant wheat selections to Puccinia striiformis. Phytopathology. 60:1368–1372. doi:10.1094/Phyto-60-1368
- Anonymous. 1932. I. Diseases of cereal crops. Can Plant Dis Surv. 12:1–23.
- Anonymous. 1964. New and noteworthy diseases. Can Plant Dis Surv. 44:1.
- Anonymous. 1970. Diseases of cereal crops. Can Plant Dis Surv. 50:8–12.
- Anonymous. 2007. Agronomic management of winter wheat in Alberta. AGDEX. 112:10–11.
- Anonymous. 2009a. Baronesse variety accounts for 58 percent of all barley plantings in 2009. Washington’s Barley Variety – 2009 CROP, Posted Online July 10, 2009. www.nass.usda.gov/Statistics_by_State/Washington/Publications/Accessed Oct 17, 2013.
- Anonymous. 2009b. Cereal seed dressing and in-furrow fungicides for 2009, Farmnote, Department of Agriculture and Food. Accessed on July 29, 2013 at http://www.agric.wa.gov.au/PC_93297.html.
- Anonymous. 2010a. Introduction to plant disease. June 2010. Saskatchewan Ministry of Agriculture. http://www.agriculture.gov.sk.ca/Default.aspx?DN=c3743d36-98ee-4e12-ba54-6454467cd781.
- Anonymous. 2011a. Alberta agriculture statistics yearbook, 2011. 37th Ed. Edmonton, AB: Alberta Agriculture and Rural Development, Statistics and Data Development Branch. AGDEX. 853–10.
- Anonymous. 2011b. 2011. Canadian Wheat Board Variety Survey. http://www.growwinterwheat.ca//documents/CWB2011VarietySurvey.pdf.
- Anonymous. 2012. Winter wheat in the parkland area of Alberta. AGDEX. 112:11–1.
- Anonymous. 2013a. Varieties of cereal and oilseed crops for Alberta. AGDEX. 100:32.
- Anonymous. 2013b. Crop protection 2013. Edmonton, AB: Alberta Agriculture and Rural Development, Information Management Division. AGDEX. 606–1.
- Bariana HS, McIntosh RA. 1994. Characterization and origin of rust and powdery mildew resistance genes in VPM1 wheat. Euphytica. 76:53–61. doi:10.1007/BF00024020
- Brown Jr. WM, Hill JP, Velasco VR. 2001. Barley yellow rust in North America. Annu Rev Phytopathol. 39:367–384. doi:10.1146/annurev.phyto.39.1.367
- Bux H, Ashraf M, Chen XM, Mumtaz AS. 2011. Effective genes for resistance to stripe rust and virulence of Puccinia striiformis f. sp. tritici in Pakistan. Afr J Biotech. 10:5489–5495.
- Chen FQ, Prehn D, Hayes PM, Mulrooney D, Corey A, Vivar H. 1994. Mapping genes for resistance to barley stripe rust (Puccinia striiformis f. sp. hordei). Theor Appl Genet. 88:215–219. doi:10.1007/BF00225900
- Chen XM. 2005. Epidemiology and control of stripe rust [Puccinia striiformis f. sp. tritici] on wheat. Can J Plant Pathol. 27:314–337. doi:10.1080/07060660509507230
- Chen XM. 2007a. Challenges and solutions for stripe rust control in the United States. Aust J Agri Res. 58:648–655. doi:10.1071/AR07045
- Chen XM. 2007b. Fungicide seed treatments for reducing yield losses caused by stripe rust on spring wheat, 2006. Plant Dis Manag Rept. 1:ST006.
- Chen XM 2010. Race summary of Puccinia striiformis f. sp. tritici (wheat stripe rust) and P. striiformis f. sp. hordei (barley stripe rust) in the United States in 2010. http://striperust.wsu.edu/races/stripe-rust-race-data.html.
- Chen XM 2011. Race summary of Puccinia striiformis f. sp. tritici (wheat stripe rust) and P. striiformis f. sp. hordei (barley stripe rust) in the United States in 2011. http://striperust.wsu.edu/races/stripe-rust-race-data.html.
- Chen XM, Line RF. 1993. Inheritance of stripe rust (yellow rust) resistance in the wheat cultivar Carstens V. Euphytica. 71:107–113. doi:10.1007/BF00023473
- Chen XM, Line RF, Leung H. 1995. Virulence and polymorphic DNA relationships of Puccinia striiformis f. sp. hordei to other rusts. Phytopathology. 85:1335–1342. doi:10.1094/Phyto-85-1335
- Chen XM, Line RF. 1999. Recessive genes for resistance to Puccinia striiformis f. sp. hordei in barley. Phytopathology. 89:226–232. doi:10.1094/PHYTO.1999.89.3.226
- Chen XM, Line RF. 2002. Identification of genes for resistance to Puccinia striiformis f. sp. hordei in 18 barley genotypes. Euphytica. 129:127–145. doi:10.1023/A:1021585907493
- Chen XM, Moore M, Milus EA, Long DL, Line RF, Marshall D, Jackson L. 2002. Wheat stripe rust epidemics and races of Puccinia striiformis f. sp. tritici in the United States in 2000. Plant Dis. 86:39–46. doi:10.1094/PDIS.2002.86.1.39
- Chen XM, Wood AD. 2006. Fungicide seed treatments for reducing yield losses caused by stripe rust on spring wheat, 2006. Plant Dis Manag Rept. 1:ST006.
- Chen XM, Penman L, Wan A, Cheng P. 2010. Virulence races of Puccinia striiformis f. sp. tritici in 2006 and 2007 and development of wheat stripe rust and distributions, dynamics, and evolutionary relationships of races from 2000 to 2007 in the United States. Can J Plant Pathol. 32:315–333. doi:10.1080/07060661.2010.499271
- Conner RL, Kuzyk AD. 1988. Effectiveness of fungicides in controlling stripe rust, leaf rust, and black point in soft white spring wheat. Can J Plant Pathol. 10:321–326. doi:10.1080/07060668809501706
- Conner RL, Thomas JB, Kuzyk AD. 1988. Overwintering of stripe rust in southern Alberta. Can Plant Dis Surv. 68:153–155.
- Danial DL, Stubbs RW, Parlevliet JE. 1994. Evolution of virulence patterns in yellow rust races and its implications for breeding for resistance in wheat in Kenya. Euphytica. 80:165–170.
- Dalvand M, Afshari F, Aeini M. 2014. Virulence survey of Puccinia striiformis, the causal agent of wheat yellow rust, by trap nursery 2010–2012. Arch Phytopath Plant Prot. 47:1508–1513. doi:10.1080/03235408.2013.848119
- Dubin HJ, Stubbs RW. 1986. Epidemic spread of barley stripe rust in South America. Plant Dis. 70:141–144. doi:10.1094/PD-70-141
- Dubin HJ, Johnson R. 1989. Postulated genes for resistance to stripe rust in selected CIMMYT and related wheats. Plant Dis. 73:472–475. doi:10.1094/PD-73-0472
- Fetch T, McCallum B, Menzies J, Rashid K, Tenuta A. 2011. Rust diseases in Canada. Prairie Soils Crops J. 4:86–96.
- Foster VR. 1937. Disease of cereal crops - wheat. Can Plant Dis Surv. 17:3.
- Fraser WP, Conners IL. 1925. The Uredinales of the prairie provinces of Western Canada. Trans Roy Soc Can. 19:275–308.
- Gaudet DA, Puchalski B, Randhawa H, Despins T, Laroche A 2013. Fungicide control of stripe rust in western Canada. 34th Annual meeting of the plant pathology society of Alberta - Brooks, AB November 4–6, 2013.
- Graf RJ, Beres BL, Laroche A, Gaudet DA, Eudes F, Pandeya RS, Badea A, Randhawa HS. 2013. Emerson hard red winter wheat. Can J Plant Sci. 93:741–748. doi:10.4141/cjps2012-262
- Hayes PM, Castro A, Corey AE, Filichkin T, Rossi C, Sandoval JS, Vales I, Vivar HE, Von Zitzewitz J. 2001. Collaborative stripe rust resistance gene mapping and deployment efforts. In: He V, McNab A, editors. Breeding barley in the new millennium: proceedings of an international symposium. Mexico, D.F.: CIMMYT; p. 47–60.
- Helm JH, Cortez MJ, Jedel PE, Salmon DF, Stewart WM. 1996. Registration of ‘Seebe’ barley. Crop Sci. 36:808. doi:10.2135/cropsci1996.0011183X003600030055x
- Hiebert CW, Thomas JB, McCallum BD, Humphreys DG, DePauw RM, Hayden MJ, Mago R, Schnippenkoetter W, Spielmeyer W. 2010. An introgression on wheat chromosome 4DL in RL6077 (Thatcher*6/PI 250413) confers adult plant resistance to stripe rust and leaf rust (Lr67). Theor Appl Genet. 121:1083–1091. doi:10.1007/s00122-010-1373-y
- Holtz MD, Kumar K, Xi K. 2011. Adaptation of Puccinia striiformis f.sp. tritici and f.sp. hordei to different temperatures. Can J Plant Pathol. 33:259. (abstr.).
- Holtz MD, Kumar K, Xi K. 2013a. Virulence phenotypes of Puccinia striiformis in Alberta from 2009–2011. Can J Plant Pathol. 35:241–250. doi:10.1080/07060661.2013.775184
- Holtz MD, Kumar K, Zantinge JL, Xi K. 2013b. Genetic diversity of Puccinia striiformis from cereals in Alberta, Canada. Plant Pathol. doi:10.1111/ppa.12094
- Holtz MD, Kumar K, Xi K 2013c. Aggressiveness of temperature adaptation in distinct genetic groups of Puccinia striiformis f. sp. tritici in North America. 34th Annual meeting of the plant pathology society of Alberta - Brooks, AB November 4–6, 2013.
- Hovmøller MS. 2001. Disease severity and pathotype dynamics of Puccinia striiformis f. sp. tritici in Denmark. Plant Pathol. 50:181–189. doi:10.1046/j.1365-3059.2001.00525.x
- Hovmøller MS, Sørensen CK, Walter S, Justesen AF. 2011. Diversity of Puccinia striiformis on cereals and grasses. Annu Rev Phytopathol. 49:197–217. doi:10.1146/annurev-phyto-072910-095230
- Huerta-Espino J, Singh RP, German S, McCallum BD, Park RF, Chen WS, Bhardwaj C, Goyeau H. 2011. Global status of wheat leaf rust caused by Puccinia triticina. Euphytica. 179:143–160. doi:10.1007/s10681-011-0361-x
- Jin Y, Szabo LJ, Carson M. 2010. Century-old mystery of Puccinia striiformis life history solved with the identification of Berberis as an alternate host. Phytopathology. 100:432–435. doi:10.1094/PHYTO-100-5-0432
- Johnson T, Newton M. 1928. The occurrence of yellow stripe rust in western Canada. Sci Agric. 8:464. (abstr.).
- Kang ZS, Zhao J, Han JD, Zhang HC, Wang XJ, Wang CF, Han QM, Guo J, Huang LL 2010. Status of wheat rust research and control in China. In Borlaug Global Rust Initiative, Technical Workshop, 30–31 May, 2010, St Petersburg, Russia.
- Keiper FJ, Hayden MJ, Park RF, Wellings CR. 2003. Molecular genetic variability of Australian isolates of five cereal rust pathogens. Mycol Res. 107:545–556. doi:10.1017/S0953756203007809
- Khlestkina EK, Roder MS, Unger O, Meinel A, Borner A. 2007. More precise map position and origin of a durable non-specific adult plant disease resistance against stripe rust (Puccinia striiformis) in wheat. Euphytica. 153:1–10. doi:10.1007/s10681-006-9182-8
- Kolmer J, Liu JQ. 2002. Inheritance of leaf rust resistance in the wheat cultivars AC Majestic, AC Splendor, and AC Karma. Can J Plant Pathol. 24:327–331. doi:10.1080/07060660209507017
- Kumar K, Holtz MD, Xi K, Turkington TK. 2012. Virulence of Puccinia striiformis on wheat and barley in central Alberta. Can J Plant Pathol. 34:551–561. doi:10.1080/07060661.2012.722130
- Kumar K, Holtz MD, Xi K, Turkington TK. 2013. Overwintering potential of the stripe rust pathogen (Puccinia striiformis) in central Alberta. Can J Plant Pathol. 35:304–314. doi:10.1080/07060661.2013.809385
- Li GQ, Li ZF, Yang WY, Zhang Y, He ZH, Xu SC, Singh RP, Qu YY, Xia XC. 2006. Theor Appl Genet. 112:1434–1440. doi:10.1007/s00122-006-0245-y
- Line FR. 2002. Stripe rust of wheat and barley in North America: A retrospective historical review. Annu Rev Phytopathol. 40:5–118. doi:10.1146/annurev.phyto.40.020102.111645
- Liu M, Hambleton S. 2010. Taxonomic study of stripe rust, Puccinia striiformis sensu lato, based on molecular and morphological evidence. Fungal Biol. 114:881–899. doi:10.1016/j.funbio.2010.08.005
- Long DL, Roelfs AP, Roberts JJ. 1992. Virulence of Puccinia recondita f. sp. tritici in the United States during 1988–1990. Plant Dis. 76:495–499. doi:10.1094/PD-76-0495
- Luo PG, Hu XY, Zhang HY, Ren ZL. 2009. Genes for resistance to stripe rust on chromosome 2B and their application in wheat breeding. Progress Nat. Sci. 19:9–15. doi:10.1016/j.pnsc.2008.02.017
- Ma H, Singh RP. 1996. Contribution of adult plant resistance gene Yr18 in protecting wheat form yellow rust. Plant Dis. 80:66–69. doi:10.1094/PD-80-0066
- Markell SG, Milus EA. 2008. Emergence of a novel population of Puccinia striiformis f. sp. tritici in eastern United States. Phytopathology. 98:632–639. doi:10.1094/PHYTO-98-6-0632
- Marshall D, Sutton RL. 1995. Epidemiology of stripe rust, virulence of Puccinia striiformis f. sp. hordei, and yield loss in barley. Plant Dis. 79:732–737. doi:10.1094/PD-79-0732
- McCallum B, Fetch T. 2001. The appearance of wheat stripe rust in Manitoba and Saskatchewan in 2000. Can J Plant Pathol. 23:192. (abstr.).
- McCallum B, Fetch T Jr., Seto-Goh P, Xi K, Turkington TK. 2006. Stripe rust of wheat and barley in Manitoba, Saskatchewan and Alberta in 2005. Can Plant Dis Surv. 86:50.
- McCallum BD, Pearse PG, Fetch J,T, Gaudet D, Tekauz A, Seto-Goh P, Xi K, Turkington TK. 2007a. Stripe rust of wheat and barley in Manitoba, Saskatchewan and Alberta in 2006. Can Plant Dis Surv. 87:66.
- McCallum BD, Chen X, Shorter S, Sadasivaiah RS, Tewari Jr. P. 2007b. Stripe rust reaction of 28 Canadian wheat cultivars cereal rust in Canada. Can J Plant Pathol. 29:401–407. doi:10.1080/07060660709507486
- McCallum BD, DePauw RM. 2008. A review of wheat cultivars grown in the Canadian prairies. Can J Plant Sci. 88:649–677. doi:10.4141/CJPS07159
- McDonald BA, Linde C. 2002. Pathogen population genetics, evolutionary potential, and durable resistance. Annu Rev Phytopathol. 40:349–379. doi:10.1146/annurev.phyto.40.120501.101443
- McDonald DB, McIntosh RA, Wellings CR, Singh RP, Nelson JC. 2004. Cytogenetical studies in wheat XIX. Location and linkage studies on geneYr27 for resistance to stripe (yellow) rust. Euphytica. 136:239–248. doi:10.1023/B:EUPH.0000032709.59324.45
- McIntosh RA, Wellings CR, Park RF. 1995. Wheat rust – an Atlas of resistance genes. Chapter 4 Canberra, Australia: CSIRO Publications,; p. 162.
- McIntosh RA, Devos KM, Dubcovsky J, Rogers WJ, Morris CF, Appels R, Somers DJ, Anderson OA 2008. Catalogue of gene symbols for wheat: 2008 supplement, http://www.shigen.nig.ac.jp/wheat/komugi/genes/symbolClassList.jsp
- Milus EA, Seyran E, McNew R. 2006. Aggressiveness of Puccinia striiformis f. sp. tritici isolates in the south-central United States. Plant Dis. 90:847–852. doi:10.1094/PD-90-0847
- Milus EA, Kristensen K, Hovmøller MS. 2009. Evidence for increased aggressiveness in a recent widespread strain of Puccinia striiformis f. sp. tritici causing stripe rust of wheat. Phytopathology. 99:89–94. doi:10.1094/PHYTO-99-1-0089
- Mundt CC, Sackett KE, Wallace LD, Cowger C, Dudley JP. 2010. Aerial dispersal and multiple-scale spread of epidemic disease. EcoHealth. 6:546–552. doi:10.1007/s10393-009-0251-z.
- Murray G, Wellings C, Simpfendorfer S, Cole C. 2005. Stripe rust, understanding the disease in wheat. 5617 January, 2005. State of New South Wales: Department of Primary Industries.
- Newton M, Johnson T, Brow AM. 1933. Stripe rust in Canada. Phytopathology. 23:25–26.
- Newton M, Johnson T. 1936. Stripe rust, Puccinia glumarum, in Canada. Can J Res C. 14:89–108. doi:10.1139/cjr36c-008
- Nover I, Scholz F. 1969. Genetic studies on the resistance of barley to yellow rust (Puccinia striiformis West.). Theor Appl Genet. 39:150–155.
- Peterson RF, Campbell AB, Hannah AE. 1948. A diagrammatic scale for estimating rust intensity of leaves and stem of cereals. Can J Res Sect C. 26:496–500. doi:10.1139/cjr48c-033
- Peturson B, Green GJ, Johnson T, Brown AM. 1953. Rust nurseries in Canada in 1953. Can Plant Dis Surv. 33:14–18.
- Pretorius ZA, Pienaar L, Prins R. 2007. Greenhouse and field assessment of adult plant resistance in wheat to Puccinia striiformis f. sp. tritici. Aust Plant Pathol. 36:552–559. doi:10.1071/AP07058
- Puchalski B, Gaudet DA. 2011. 2010 southern Alberta stripe rust survey. Can Plant Dis Surv. 91:68–69.
- Puchalski B, Gaudet D, Randhawa H, Wogsberg S, Kundrik K, Frick M, Despins T, Laroche A 2013.The occurrence of stripe rust in Southern Alberta 2012-2013. 34th Annual meeting of the plant pathology society of Alberta - Brooks, AB November 4–6, 2013.
- Randhawa H, Puchalski BJ, Frick M, Goyal A, Despins T, Graf RJ, Laroche A, Gaudet DA. 2012. Stripe rust resistance among western Canadian spring wheat and triticale varieties. Can J Plant Sci. 92:713–722. doi:10.4141/cjps2011-252
- Rapilly F. 1979. Yellow rust epidemiology. Annu Rev Phytopathol. 17:59–73. doi:10.1146/annurev.py.17.090179.000423
- Roelfs AP, Singh RP, Saari EE. 1992. Rust diseases of wheat. Concepts and methods of disease management. Mexico, D.F.: CIMMYT.
- Rossi C, Cuesta-Marcos A, Vales I, Gomez-Pando L, Orjeda G, Wise R, Sato K, Hori K, Capettini F, Vivar H, et al.. 2006. Mapping multiple disease resistance genes using a barley mapping population evaluated in Peru, Mexico, and the USA. Mol Breed. 18:355–366. doi:10.1007/s11032-006-9043-0
- Sadasivaiah RS, Thomas JB, Conner RL. 1993. Registration of ‘AC Reed’ spring wheat. Crop Sci. 33:879. doi:10.2135/cropsci1993.0011183X003300040059x
- Sadasivaiah RS, Perkovic SM, Pearson DC, Postman B, Beres BL. 2004. Registration of AC Andrew wheat. Crop Sci. 44:696–697. doi:10.2135/cropsci2004.6960
- Safavi SA, Afshari F, Yazdansepas A. 2013. Effective and ineffective resistance genes to wheat yellow rust during six years monitoring in Ardabil. Arch Phytopath Plant Prot. http://dx.doi.org/10.1080/03235408.2012.752142.
- Sanford GB, Broadfoot WC. 1929. Stripe rust in Alberta. Sci Agric. 9:337–345.
- Sanford GB, Broadfoot WC. 1932. Epidemiology of stripe rust in western Canada. Sci Agric. 13:77–96.
- Sharma-Poudyal D, Chen XM, Wan AM, Zhan GM, Kang ZS, Cao SQ, Jin SL, Morgounov A, et al. 2013. Virulence characterization of international collections of the wheat stripe rust pathogen, Puccinia striiformis f.sp. tritici. Plant Dis. 97:379–386. doi:10.1094/PDIS-01-12-0078-RE
- Singh PR, Rajaram S. 1994. Genetics of adult plant resistance to stripe rust in ten spring bread wheats. Euphytica. 72:1–7.
- Singh RP. 2007. Increasing genetic yield and mitigating effects of key biotic and abiotic constraints to wheat production in India through international wheat resources and partnerships. J Wheat Res. 1:13–18.
- Singh RP, Nelson JC, Sorrells ME. 2000. Mapping Yr28 and other genes for resistance to stripe rust in wheat. Crop Sci. 40:1148–1155. doi:10.2135/cropsci2000.4041148x
- Spackman ME, Ogbonnaya FC, Brown JS. 2010. Hypervariable RAPD, ISSR and SSR markers generate robust taxonomic groups among Puccinia striiformis formae speciales of importance to Australian agriculture. Austral Plant Pathol. 39:226–233. doi:10.1071/AP09087
- Stubbs RW. 1985. Stripe rust. In: Ap R, Bushnell WR, editors. The cereal rusts. Vol. II. Diseases, distribution, epidemiology, and control. Orlando: Acad. Int. Maize Wheat Improve. Cent. Mexico; p. 61–101.
- Stubbs RW. 1988. Pathogenicity analysis of yellow (stripe) rust of wheat and its significance in a global context. In: Nw S, Rajaram S, editors. Breeding strategies for resistance to the rusts of wheat. Mexico, D.F.: CIMMYT.
- Su H, Conner RL, Graf RJ, Kuzyk AD. 2003. Virulence of Puccinia striiformis f. sp. tritici, cause of stripe rust on wheat, in western Canada from 1984 to 2002. Can J Plant Pathol. 25:312–319. doi:10.1080/07060660309507084
- Tekauz A. 2003. Disease of Field Crops in Canada. In: Kl B, Bd G, Rk G, Raa M, editors,. 2nd ed. The Canadian Phytopathological Society; University Extension Press, University of Saskatchewan, p. 34.
- Tollenaar H. 1967. A comparison of Puccinia striiformis f. sp. poae on bluegrass with P. striiformis f. sp. tritici and f. sp. dactylidis. Phytopathology. 57:418–420.
- Turkington TK, McCallum BD, Jr. T F, Xi K, Kumar K, Guadet D, Oo O, Weiss R, Soroka J. 2009. Wind trajectories and cereal rust risk, western Canada, 2007. Can J Plant Pathol. 31:147. (abstr.).
- Tyner LE 1947. 27th Annual Report of the Can Plant Dis. Survey. p. 2.
- Wan AM, Chen XM. 2012. Virulence, frequency, and distribution of races of Puccinia striiformis f. sp. tritici and P. striiformis f. sp. hordei identified in the United States in 2008 and 2009. Plant Dis. 96:67–74. doi:10.1094/PDIS-02-11-0119
- Wan AM, Zhao ZH, Chen XM, He ZH, Jin SL, Jia QZ, Yao G, Yang JX, Wang BT, Li GB, et al.. 2004. Wheat stripe rust epidemics and virulence of Puccinia striiformis f. sp. tritici in China in 2002. Plant Dis. 88:896–904. doi:10.1094/PDIS.2004.88.8.896
- Wellings C, Edwards J, Thompson R 2007. Stripe rust - an update on the Yr17 pathotype. BSW DPI Profitable & Sustainable Primary Industries at www.dpi.nsw.gov.au.
- Wellings CR. 2007. Puccinia striiformis in Australia: A review of the incursion, evolution, and adaptation of stripe rust in the period 1979–2006. Austr J Agr Res. 58:567–575. doi:10.1071/AR07130
- Wellings CR. 2011. Global status of stripe rust: A review of historical and current threats. Euphytica. 179:129–141. doi:10.1007/s10681-011-0360-y
- Wellings CR, Burdon JJ, McIntosh RA, Wallwork H, Raman H, Murray GM. 2000a. A new variant in Puccinia striiformis causing stripe rust on barley and wild Hordeum in Australia. Plant Pathol. 49:803. doi:10.1046/j.1365-3059.2000.00506.x
- Wellings CR, Read B, Moody D 2000b. Stripe rust affecting barley in Australia—current and potential threats. In Proceedings of 8th International Barley Genetics Symposium. Adelaide, Australia, September 2000. III, p. 197–199.
- William M, Singh RP, Huerta-Espino J, Ortiz IS, Hoisington D. 2003. Molecular marker mapping of leaf rust resistance, gene Lr46 and its association with stripe rust resistance, gene Yr29 in wheat. Phytopathology. 93:153–159. doi:10.1094/PHYTO.2003.93.2.153
- Xi K, Holtz M, Kumar K, Aljarrah M, Helm J, Juskiw P, Nyachiro J, Salmon D, Spaner D, Turkington TK, et al. 2011. Control of cereal stripe rust in Alberta using genetic resistance. Alberta Crop Industry Development Fund Project #2007F028R. Final Report.
- Xi K, Chen XM, Capettini F, Falconi E, Yang RC, Helm JH, Holtz MD, Juskiw P, Kumar K, Nyachiro J, Turkington TK. 2013. Multivariate analysis of stripe rust assessment and reactions of barley in multi-location nurseries. Can J Plant Sci. 93:209–219. doi:10.4141/cjps2012-051
- Yan GP, Chen XM. 2007. Molecular mapping of the rps1.a recessive gene for resistance to stripe rust in BBA 2890 barley. Phytopathology. 97:668–673. doi:10.1094/PHYTO-97-6-0668
- Yan GP, Chen XM. 2008. Identification of a quantitative trait locus for high-temperature adult-plant resistance against Puccinia striiformis f. sp. hordei in ‘Bancroft’ barley. Phytopathology. 98:120–127. doi:10.1094/PHYTO-98-1-0120
- Zhao J, Wang L, Wang ZY, Chen XM, Zhang HC, Yao JN, Zhan GM, Huang LL, Kang ZS. 2013. Identification of eighteen Berberis species as alternate hosts of Puccinia striiformis f. sp. tritici and virulence variation in the pathogen isolates from natural infection of barberry plants in China. Phytopathology. “First Look” paper. http://dx.doi.org/10.1094/PHYTO-09-12-0249-R. posted 03/20/2013.