Abstract
Susceptibility of biofilms of Clavibacter michiganensis subsp. sepedonicus, the causal agent of bacterial ring rot of potato, to three common agricultural disinfectants was tested. The MBECTM assay device was used to evaluate optimum parameters for growing artificial biofilms. These conditions were determined to be 7 days of growth at 23°C in a yeast extract-glucose-mineral salts medium. As expected, the bacteria in the biofilm state were more resistant to disinfection by chemical treatment with sodium hypochlorite, quaternary ammonium, and hydrogen peroxide when compared with planktonic cells. Artificial biofilms were also grown on five different surface materials typically found in commercial potato storage facilities (concrete, mild steel, rubber, polycarbonate and wood) using the BESTTM assay device to test the effect of surface type on biofilm susceptibility to disinfection. Sodium hypochlorite was the most effective disinfectant on the wood surface and hydrogen peroxide was best on the mild steel surface. Efficacies of the various disinfectants were not significantly different on concrete, rubber and polycarbonate surfaces. When artificially grown biofilms and those grown naturally in potato tissue were transferred to, and dried onto, coupons of the different surface materials, they were significantly more difficult to inactivate than in situ grown biofilms. The resistance of plant pathogenic bacteria in the biofilm state, particularly when spread and dried onto surfaces of agricultural machines and other equipment, to commonly used disinfectants, has important implications for disease control strategies that depend largely on strict sanitary and hygienic practices.
Résumé
La sensibilité des biofilms de Clavibacter michiganensis subsp. sepedonicus, l’agent causal de la pourriture du cerne chez la pomme de terre, à trois désinfectants agricoles courants a été testée. Le biotest MBECTM a été utilisé pour évaluer les paramètres optimaux relatifs à la croissance de biofilms artificiels. Ces conditions ont été établies à sept jours de croissance à 23 °C sur un milieu à base d’extrait de levure, de glucose et de sels minéraux. Comme nous nous y attendions, lorsque comparées aux cellules planctoniques, les bactéries à l’état de biofilms étaient plus résistantes à la désinfection chimique effectuée avec de l’hypochlorite de sodium, de l’ammonium quaternaire et du peroxyde d’hydrogène. Des biofilms artificiels ont également été cultivés sur cinq différents matériaux de surface trouvés généralement dans les entrepôts commerciaux pour pommes de terre (béton, acier doux, caoutchouc, polycarbonate et bois). Ces derniers ont été évalués avec le biotest MBECTM afin d’estimer l’influence du type de surface sur la sensibilité des biofilms à la désinfection. L’hypochlorite de sodium s’est révélé le désinfectant le plus efficace sur le bois, et le peroxyde d’hydrogène s’est avéré le plus efficace sur l’acier doux. Les taux d’efficacité des divers désinfectants n’étaient pas significativement différents sur les surfaces en béton, en caoutchouc et en polycarbonate. Quand les biofilms cultivés artificiellement et ceux cultivés naturellement sur des tissus de pomme de terre ont été transférés, et séchés, sur des coupons des différents matériaux, ils étaient significativement plus difficiles à inactiver que les biofilms cultivés in situ. La résistance des bactéries pathogènes, à l’état de biofilms, aux désinfectants utilisés couramment, particulièrement lorsque ces biofilms sont appliqués et séchés sur la machinerie agricole et les autres équipements, a d’importantes implications quant aux stratégies de lutte contre la maladie qui dépendent en grande partie de mesures sanitaires et hygiéniques strictes.
Introduction
The bacterial ring rot disease of potato is caused by the gram-positive, non-flagellated, coryneform bacterium, Clavibacter michiganensis subsp. sepedonicus (Spieck. & Kotth.) Davis et al. This pathogen is considered to be under quarantine regulations in many countries, but in Canada, it is regulated as a zero-tolerance pathogen within the seed potato certification scheme. In the years following 1931, when bacterial ring rot was first described in Canada, heavy losses were experienced by growers in all potato-producing regions of the country. Increasingly, however, the disease has been brought under control by the application of strict certification rules for seed potatoes, and diligent application of sanitary and hygienic practices on potato farms (De Boer et al. Citation1996; De Boer & Boucher Citation2011).
Vertical dissemination of C. m. sepedonicus, that is, the spread of the disease via seed tubers from one generation to the next, is mitigated by strict adherence to a zero tolerance threshold in seed certification (De Boer Citation2008). In Canada, zero tolerance in seed potato certification means that if the disease is detected in any potato crop on a seed potato farm, all potato lots within the production unit are ineligible for certification as seed. Initially, detection of ring rot depended solely on visual field inspection but since the mid-1980s, field inspection has been supplemented with laboratory tests for detection of latent (asymptomatic) infections. Indeed, over the last decade or more, most ring rot detections in Canada have been the result of laboratory tests and very few cases of ring rot have been detected as a result of field inspections for visual symptoms of the disease.
Horizontal dissemination of C. m. sepedonicus via contaminated farm equipment is considered to be a major means by which the pathogen is maintained and spread within the potato industry (De Boer & Boucher Citation2011). It is well known that the ring rot bacterium persists on the surfaces of equipment, storage bins, and transport vehicles and that disinfection of such surfaces is essential to prevent its survival and spread (Nelson Citation1978, Citation1980). Current disinfectants and recommended protocols for bacterial ring rot disinfection were developed based on the ability of antimicrobial compounds to inactivate planktonic (solitary, free-floating) forms of bacteria (Dinesen Citation1984; Secor et al. Citation1988). There is now good evidence, however, that C. m. sepedonicus does not grow as planktonic populations but rather as a biofilm in infected potato stems and tubers (Marques et al. Citation2003b).
Research over the last decade has shown that most bacteria, including plant-associated species, in natural environments normally occur as complex communities of biofilms (Fett Citation2000; Morris & Monier Citation2003; Danhorn & Fuqua Citation2007). Marques et al. (Citation2002) were the first to show that phytopathogenic bacteria also formed biofilms inside their host plants. They characterized in vitro biofilm formation by Xylella fastidiosa Wells et al, the causal agent of citrus variegated chlorosis of sweet orange and other plant hosts. Subsequently, it was found that other vascular plant pathogens, such as Xanthomonas campestris (Pammel) Dowson (Dow et al. Citation2003), Pantoea stewartii (Smith) Mergaert et al (Koutsoudis et al. Citation2006), Clavibacter michiganensis subsp. michiganensis (Smith) Davis et al. (Harding et al. Citation2011), as well as the potato pathogens Pectobacterium atrosepticum Gardan et al. (Marques et al. Citation2003a), and C. m. sepedonicus (Marques et al. Citation2003b) also have the capacity to form microbial biofilms.
Biofilms consist of communities of single or mixed species of microorganisms that are attached to each other and/or surfaces by a self-produced extracellular polysaccharide matrix. Bacteria growing as biofilms are frequently much more difficult to inactivate since they show an increased tolerance to biocides and antibiotics when compared with planktonic counterparts (Pan et al. Citation2006; Saitou et al. Citation2009). For example, Escherichia coli (Migula) Castellani & Chalmers biofilms are up to 100 times more tolerant to antibiotics and disinfectants than the corresponding planktonic cells (Spoering & Lewis Citation2001; Harrison et al. Citation2005a). Biofilms derive their extraordinary tolerance to antimicrobials from at least five contributing factors that may involve both genetic and physical components (Lewis Citation2001; Whiteley et al. Citation2001; Mah et al. Citation2003). These are: (1) The structure of a biofilm results in nutrient and oxygen gradients that restrict bacterial growth in regions of the surface-adherent community (Stewart Citation2003). Biofilms also have different growth characteristics and nutrient and drug uptake profiles from their planktonic counterparts (Olson et al. Citation2002). Many drugs and disinfectants are only active against fast-growing cells with the result that slow-growers in a biofilm are spared the effects of these otherwise lethal factors. (2) Intercellular signals can up-regulate cellular repair and defence machinery, such as the enzymes involved in removing hydrogen peroxide from cells (Bjarnsholt et al. Citation2005). (3) The extracellular polymeric matrix of biofilms is electrostatically charged and can bind to antimicrobials, thus sequestering them from the cells (Harrison et al. Citation2005b). (4) Multidrug tolerant ‘persister’ cells are dormant in the presence of an antibiotic, but not dead. When the drug is removed, these specialized survivor cells can return to an active metabolic state. This mechanism is believed to be responsible for recurrent infections in hospitals (Keren et al. Citation2004). (5) Genetic diversity acts as an ‘insurance policy’, thus improving the chance that some cells will survive any external challenge (Harrison et al. Citation2005d). As such, an assessment of anti-biofilm activity is required to analyse the efficacy of current disinfection procedures and practices for bacterial ring rot.
Until recently, a significant challenge to biofilm research was difficulty in culturing, characterizing and testing biofilms in traditional biofilm reactors. Traditional techniques can be cumbersome when many treatments or replicates are needed as they are not well-suited for rapid, high-throughput screening experiments or factorial experiments with many replicates. This changed with the introduction of the MBEC™ assay, formerly the Calgary Biofilm Device, which involves a patented, multi-well plate technology specifically designed for rapid, high throughput culturing, characterizing and testing of microbial biofilms (Allan et al. Citation2011). Additionally, planktonic cell testing can be performed simultaneously in the same plate. Up to 96 biofilms can be cultured on pegs extending from the plate lid into the wells of the plate bottom. The lid, with biofilms attached, are conveniently transferred through a series of solutions or treatments such as rinses, challenges, neutralizations, etc., culminating with the recovery of bacterial cells, which can be enumerated via standard plate count methods. This assay is now an ASTM standard method for disinfectant and biocide efficacy testing versus microbial biofilms (ASTM E2799-11). Similarly, the BEST™ assay is also a multi-well plate assay adapted for culturing and analysing biofilms on various types of surface materials (Harding et al. Citation2011).
The specific objective of this study was to employ the MBEC™ and BEST™ assays to test the susceptibility of C. m. sepedonicus in the biofilm state to several disinfectants commonly used in agriculture. We tested sensitivity to disinfectants of in situ formed biofilms on different types of surfaces that commonly occur in potato production, storage and transportation systems, such as wood, rubber, cement, steel, and polycarbonate plastic. Disinfectant efficacy was also tested against transferred biofilms (t-biofilms) because they more accurately reflect real-world circumstances in which in planta-formed biofilms from infected plant tissues are transferred to, and spread over, the surfaces of equipment, storage bins, and transport vehicles.
Materials and methods
Bacterial strains
Strains CS3, R13 and R14 of C. m. sepedonicus were used in preliminary experiments for the MBEC™ assay, while only strain R14 was used in later experiments with different surface materials in the BEST™ assay. Strain CS3 was isolated from potato in Alberta by G.A. Nelson in 1976, while strains R13 and R14 were isolated by S.H. De Boer from potato ‘Russet Burbank’ grown in B.C. in 1988 and from potato ‘Atlantic’ grown in Alberta in 1991, respectively. The strains were routinely grown on yeast extract, glucose, mineral salts medium (YGM) (De Boer & Copeman Citation1980) at 23°C. Long-term storage of strains was in 20% glycerol at −80°C.
Biofilm development and analysis
Biofilms were grown on MBEC™ assay devices (Innovotech, Edmonton, AB) according to the ASTM standard method (ASTM E2799-11). MBEC™ assay devices are polystyrene plates of the same dimensions as standard 96-well microtitre plates but have lids with 96 pegs that match corresponding wells in the plate (). Biofilms grow attached to the surface of each peg when immersed in corresponding wells filled with bacterial inoculum in a growth medium and exposed to shear force by incubation on a gyrorotary shaker. This method, formerly the Calgary Biofilm Device, is routinely used to grow and analyse biofilms (Ali et al. Citation2006; Ceri et al. Citation1999; Bardouniotis et al. Citation2001; Harrison et al. Citation2005c; Wong et al. Citation2009; Allan et al. Citation2011).
Fig. 1 (Colour online) Lids of biofilm assay devices. Left panel shows a MBEC™ lid with both balsa wood and polystyrene plastic pegs, and the right panel shows a BEST™ lid with coupons of various surface materials.
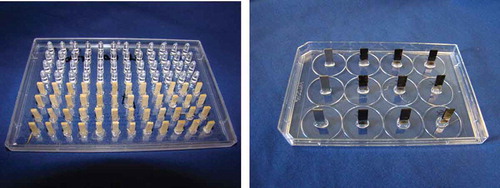
The specific method for growth and analysis of C. m. sepedonicus biofilms on the MBEC™ assay in this study was optimized by starting with aqueous suspensions of 6–7-day-old cultures of C. m. sepedonicus, which were adjusted to a turbidity level equal to 0.5 McFarland Standard units. An 88 μL aliquot of this stock suspension was diluted with 22 mL of growth medium and aliquoted at 150 μL/well in a MBEC™ assay plate. A pegged lid was sealed onto the plate with Parafilm and placed on a rotary shaker set at 150 rpm. Biofilm growth was evaluated after 3, 5, 7, and 10 days of incubation at 20, 23, and 26ºC. Growth media evaluated for biofilm development were nutrient broth (NB), YGM broth (YGMB), and Mueller-Hinton (MHB) broth. Poly-L-lysine coated pegs were evaluated in addition to untreated pegs for efficacy in supporting biofilm development. Balsa wood pegs were tested by replacing the polystyrene pegs with balsa wood ().
To measure populations of viable cells in biofilms, biofilm-containing pegs were rinsed with sterile water to remove planktonic cells and were treated in a Model 250T ultrasonic bath (VWR, Westchester, PA) to disperse biofilm bacteria into recovery broth with neutralizer. Neutralizing agents are often used in studies that measure minimum bactericidal concentrations (Buckingham-Meyer et al. Citation2007). These agents reduce toxicity from the carry-over of biologically active compounds from challenge to recovery media. A universal neutralizer was selected that would effectively neutralize across the disinfectant families evaluated while having no net negative impact on normal organism growth and recovery. This universal neutralizer recipe consisted of 1.0 gL-histidine (Sigma, USA), 1.0 gL-cysteine (Sigma), 2.0 g reduced glutathione (Sigma) in 20 mL double distilled water. This solution was sterilized by filtration through 0.22 μm diameter pore size filter (Corning Inc., Germany) and stored at −20°C.
Dispersed cells from biofilms were enumerated by serially diluting each recovery well and plating 20 μL of each dilution on agar plates specific to growth medium (NA, MH or YGM). Colony counts were transformed to log10 (count + 1, correcting for zero counts), prior to analyses.
Artificial t-biofilms of C. m. sepedonicus were prepared from colonies of C. m. sepedonicus growing on YGM medium. Colonies were applied to balsa wood coupons of the BEST™ Assay using aseptic technique and air-dried in a sterile cabinet prior to treatment with disinfectant. Natural t-biofilms were prepared from decayed vascular tissues collected from ring rot-infected tubers. These tissues were applied and dried onto balsa wood coupons of BEST™ Assay devices in a manner similar to the artificial t-biofilms.
Biofilm susceptibility to disinfectants – MBEC™ assay
Biofilms were grown on MBEC™ assay devices using the optimized conditions described above. Each treatment was performed in triplicate to evaluate efficacy of disinfectants. Mature biofilms grown on the pegs of the MBEC™ assay device were rinsed to remove any planktonic cells, and then exposed to disinfectant solutions by transferring the pegged lids to 96-well plates containing disinfectant. Three standard agricultural disinfectants, namely sodium hypochlorite, quaternary ammonium and hydrogen peroxide, were evaluated at 0.5×, 1×, 2×, and 5×, where ‘×’ represents the manufacturers’ label recommended concentrations (). Three to six replicates of sterility (non-inoculated) and growth control (no treatment) check wells were included on each plate. Exposure times were 5, 10 and 15 min on separate identical disinfectant-containing plates. Following exposure to disinfectant, biofilms were rinsed with sterile water to remove residual disinfectant and placed in universal neutralizing recovery medium (as described above) for at least 30 min (Sutton et al. Citation2002) and serially diluted and enumerated on agar plates specific to the growth medium. Disinfectant efficacy was measured as log reduction in surviving populations of biofilm cells as compared with viable populations recovered from untreated biofilms. For example, if the growth control population reached log10 5.6 whereas the disinfected biofilm population was log10 1.2, the log reduction was 5.6–1.2 = 4.4. A 3-log reduction in bacterial viability, equalling 99.9% of bacteria killed, was taken as the measure of effective disinfection. Eradication was considered achieved when no viable bacteria could be recovered after the disinfectant treatment and neutralization.
Table 1. Agricultural disinfectants used in the present study.
For comparative purposes, the efficacies of disinfectants were also tested on planktonic cells of C. m. sepedonicus. For these experiments, C. m. sepedonicus cells from overnight cultures grown in YGM broth were added to wells of 96-well plates containing disinfectants so that final disinfectant concentrations of 0.25×, 0.5×, 1× and 2× were achieved. Exposure times of 5, 10 and 15 min were performed. Following exposure to the disinfectants, the cells were transferred to neutralizer broth and enumerated as described above.
Disinfectant efficacy on various surface materials – BEST™ assay
Biofilm formation and disinfectant efficacy on different surface materials were tested using BEST™ assay devices (Innovotech, Inc.), which consisted of 12-well culture plates to which coupons of surface material could be attached to the lid (). This assay has been routinely used to culture and analyse microbial biofilms formed on a variety of surface materials (Harding et al. Citation2010, Citation2011). Coupons of concrete (13 × 5 × 4 mm), mild steel (15 × 6 × 1 mm), rubber (15 × 6 × 5 mm), polycarbonate (15 × 5 × 1 mm) and wood (15 × 5 × 3 mm) were attached so they extended vertically down from the lid into each of the plate wells and could be immersed into the liquid of individual wells. Assembled plates were sterilized by exposure to ethylene oxide for 16–18 h, or for the mild steel by incubation in dry heat (60ºC) for 72 h.
In situ- and t-biofilms were treated with disinfectants at different concentrations and exposure times on the coupons of the BEST™ assay devices in the same way as described above for the MBEC™ assay device. Four millilitres of liquid were used in the wells of the BEST™ assay devices compared with 150 μL used in the wells of the MBEC™ assay devices.
Statistics
All statistical analyses were performed using Minitab (Minitab Inc., State College, PA). A test for equal variances was performed on each data set using either Bartlett’s test for normally distributed data sets or Levene’s test for non-normal, continuous distributions. Mixed-model Analyses of Variance (ANOVA) were performed using General Linear Model (GLM) to determine significant differences for main factors and for interactions between factors. Mixed-model ANOVA by GLM was used due to the fact that a contaminated well would require removal of a data point, making the model otherwise unbalanced. Subsequently, Tukey’s Multiple Comparisons Tests were performed to determine statistically significant separations of means within each main factor.
Results
Optimizing biofilm development
Balsa wood and poly-L-lysine coated polystyrene pegs were tested for their ability to consistently achieve target biofilm populations of ≥ 103 colony forming units (CFU)/20 μL in the MBEC™ assay. Mixed-model ANOVA using GLM revealed that the 26°C temperature, the 3-day incubation interval and the R13 strain were significantly inferior factors (not shown). The remaining conditions did not result in statistically significantly different biofilm populations; however, the best biofilm development trended toward those of strain R14 grown in YGM at 23ºC for 7 days (not shown), and therefore these optimized conditions were used in subsequent analyses with both the MBEC™ and BEST™ assays.
Efficacy of disinfectants – MBEC™ assay
Reductions of C. m. sepedonicus biofilm populations were achieved by exposure to all three disinfectants, but differences between test parameters were more evident in balsa wood-grown biofilms than in biofilms grown on poly-L-lysine-coated polystyrene pegs in the MBEC™ Assay. It appeared that C. m. sepedonicus preferred the balsa wood as a biofilm growth substrate as it formed more abundant and consistent biofilm populations on the balsa wood pegs. Results generated using balsa wood pegs are presented.
ANOVA revealed that there were no significant variations between populations on the three replicate pegs of the same treatment (not shown). However, significant differences existed between disinfectant types, concentrations and exposure times. Mean separations verified that disinfectant efficacy increased with exposure time and concentration, and revealed that sodium hypochlorite was the most effective of the three when all results from all concentrations and exposure times were compared ().
Table 2. Overall log reductions of C. m. sepedonicus biofilm populations for each biocide (left panel), exposure time (centre panel) and concentration (right panel). Biofilm populations were grown using the MBEC™ Assay.
A 3-log reduction (effective disinfection) was obtained with sodium hypochlorite after 10 min at the 1× concentration and after 15 min at the 0.5× concentration (). Similarly, effective disinfection was successful using hydrogen peroxide after a 15 min exposure to the 1× concentration and a 10 min to the 2× concentration. Full eradication was achieved with 5× sodium hypochlorite after 15 min exposure, and with 5× hydrogen peroxide after 10 and 15 min exposures. The quaternary ammonium product was unable to effectively disinfect or eradicate the pathogen at any concentration or contact time evaluated.
Fig. 2 Log-reduction values in viable cell populations within C. m. sepedonicus biofilms grown on balsa wood pegs of MBEC™ assay devices after exposure to one of three agricultural disinfectants. (A) sodium hypochlorite, (B) quaternary ammonium and (C) hydrogen peroxide, at four different concentrations. The dotted horizontal line represents a 3-log reduction in viable counts, while the dashed horizontal line represents the level required for complete eradication of the bacterium as estimated by populations on growth control pegs.
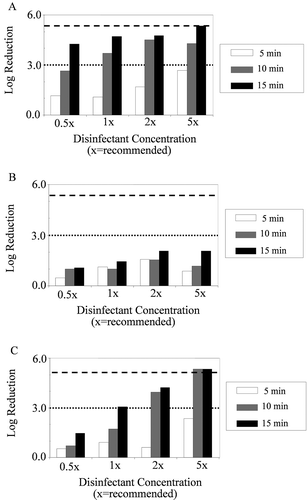
In contrast to biofilm cells, planktonic cells of C. m. sepedonicus were completely inactivated by all three disinfectants at the lowest concentration analysed (0.25×) in only 5 min of contact time (not shown), confirming that planktonic cells are much more sensitive to chemical disinfectants than their counterpart cells within a biofilm.
Efficacy of disinfectants on different surface materials – BEST™ assay
Generally, the porous surfaces (wood, concrete and rubber) were more difficult to disinfect than the metal and plastic surfaces, with balsa wood being the most difficult overall (). For example, eradication of C. m. sepedonicus biofilms was achieved with the 5× concentrations of disinfectants on all surface materials except balsa wood. Each of the three disinfectants had increased efficacy at the higher concentrations and longer exposure times on all surface materials, and 3-log reduction thresholds were achieved at the 1× concentration with all the disinfectants on all surface materials with two exceptions; quaternary ammonium for balsa-wood grown biofilms, and sodium hypochlorite on biofilms grown on mild steel (). Required exposure times to achieve a 3-log reduction, however, varied from 5 to 15 min depending on the disinfectant-surface material combination tested.
Table 3. Contact time of each of three agricultural disinfectants required to achieve effective disinfection (3-log reduction) and full eradication of C. m. sepedonicus biofilm populations grown on each of five surface materials.
Only hydrogen peroxide was able to achieve effective disinfection on all five surfaces at the 1× concentration (). Additionally, it eradicated the bacterium from mild steel and polycarbonate surfaces at the 1× concentration, but required higher concentrations to completely eradicate it from concrete and rubber, and failed to achieve total eradication on balsa wood at even the highest concentration tested. The quaternary ammonium compound was able to effectively disinfect and eradicate biofilms at the 1× concentration on all surfaces, except balsa wood. Sodium hypochlorite at the 1× concentration effectively disinfected biofilms on all surfaces, except mild steel. Sodium hypochlorite on mild steel was the only example of a non-porous surface being more difficult to disinfect. This was likely due to severe corrosion of the metal, which may have been complicated by the formation and presence of the biofilm (Beech & Sunner Citation2004; Lewandowski & Beyenal Citation2009), making the surface very difficult to disinfect.
In order to compare efficacies of the three disinfectants, an operational range of conditions was chosen for statistical analyses. The operational range included results from treatment conditions that would be most appropriate to commercial potato storage disinfection, i.e. the 1× and 2× concentrations at the 15-min exposure time. Mixed-model ANOVA performed for data within the operational range revealed that there were significant variances between biocides on balsa wood and steel, but not on concrete, rubber or polycarbonate. Mean separations for disinfectants on balsa wood and steel were performed using Tukey’s method at 95% confidence. The analysis revealed that the relative efficacies were sodium hypochlorite > hydrogen peroxide > quaternary ammonium on balsa wood, but, on steel, hydrogen peroxide and quaternary ammonium were superior to sodium hypochlorite ().
Table 4. Log reductions of C. m. sepedonicus biofilms by three agricultural disinfectants on two surfaces.
Disinfection of artificial and natural, transferred biofilms (t-biofilms) – BEST™ assay
Effective disinfection was achieved for artificial t-biofilms on balsa wood coupons with both hydrogen peroxide and sodium hypochlorite, but not with quaternary ammonium (). However, effective disinfection was only observed at concentrations greater than 1× (the manufacturer’s recommended rate) i.e., 2× for hydrogen peroxide and 5× for sodium hypochlorite. Eradication of the artificial C. m. sepedonicus t-biofilm was only achieved with hydrogen peroxide at the 5× concentration. ANOVA showed that significant differences existed for each variable, except for replicates from the same treatment. Since disinfection was only observed for one of the three disinfectants (hydrogen peroxide) within the operational range, the 5× concentration was added to the analysis of this data set. Mean separations on the data set, including 5× concentrations, revealed that average log reductions on wood coupons ranged as follows: hydrogen peroxide > sodium hypochlorite > quaternary ammonium (). Sodium hypochlorite at 5× and hydrogen peroxide at 2× and 5× were not significantly different from one another. When individual treatment concentrations were compared, sodium hypochlorite below 5× and hydrogen peroxide below 2× were not significantly different than quaternary ammonium (). It is of interest to note that, when disinfecting artificial t-biofilms, the 5× concentrations were significantly better than the 2× concentrations (), indicating that manufacturers’ label recommendations may overestimate the disinfectants’ abilities to achieve a 3-log reduction of t-biofilms.
Table 5. Comparison of three agricultural disinfectants within an operational range (1× and 2× concentrations), plus the 5× concentration, for efficacy against artificial and natural t-biofilms of C. m. sepedonicus.
Fig. 3 Log-reduction values in viable cell populations in C. m. sepedonicus a, artificial t-biofilms, and b, natural t-biofilms air-dried to balsa wood coupons of BEST™ assay devices after exposure to one of three agricultural disinfectants – sodium hypochlorite, quaternary ammonium and hydrogen peroxide, at four different concentrations. The dotted horizontal line represents a 3-log reduction in viable counts, while the dashed horizontal line represents the level required for complete eradication of the bacterium as estimated by populations on growth control pegs.
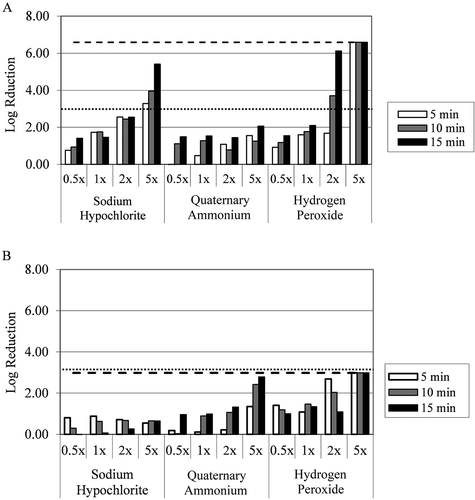
Bacterial populations in natural t-biofilms, being less dense than those of in situ or artificial t-biofilms, were approximately at log10 values of 2.98. As a result, a 3-log reduction in viable bacterial count was functionally equivalent for both effective disinfection and eradication. Disinfection and eradication of natural t-biofilms was only achieved with the 5× concentration of hydrogen peroxide (). When data within the operational range were analysed, the hydrogen peroxide and quaternary ammonium were significantly better than sodium hypochlorite (not shown). When the 5× concentrations were included in the analysis, the hydrogen peroxide and quaternary ammonium at 5× remained significantly better that all other treatments (). It appeared that the organic load (potato tuber tissue) accompanying the C. m. sepedonicus biofilms in the natural t-biofilms significantly reduced the efficacy of sodium hypochlorite.
Discussion
Effective disinfection of microorganisms is generally demonstrated via a minimum 3-log reduction (99.9% kill) in viable cell populations. For example, Health Canada defines a sanitizer as ‘a product that reduces the level of microorganisms present by significant numbers, e.g., a 3-log10 reduction (99.9%) or more, or to acceptable levels established by federal or provincial health authorities’ (http://www.hc-sc.gc.ca/dhp-mps/prodpharma/applic-demande/guide-ld/disinfect-desinfect/disin_desin-eng.php). Therefore, the impetus for preliminary experiments in this study was to establish the conditions in which a minimum of 3-log (i.e. 1000 cfu) viable cell count could be achieved for individual biofilms grown in MBEC™ and BEST™ assay devices. The optimized conditions for biofilm production using YGM broth medium incubated for 7 days at 23ºC met the required criteria for two of the three strains of C. m. sepedonicus tested. The reason for the lower growth rate of strain R13 in a biofilm community was not investigated in this study, but could have been due to an uncharacterized genetic change specific to that strain, or that became fixed during handling and/or short-term storage of the pathogen culture. It has long been known that cultures of C. m. sepedonicus maintained for extended periods on artificial media lose their virulence markedly (Snieszko & Bonde Citation1943). Strain R14, selected for use in this study, is a highly virulent strain that has been used previously for inoculation in various field experiments on bacterial ring rot of potato (e.g. De Boer & Hall Citation1996).
Many biocides have been recommended over the years for disinfection of machinery and equipment contaminated with bacteria from ring rot-infected potatoes. In the 1940s, when ring rot first became a major potato disease, recommended disinfectants included mercury chloride, phenol, and calcium iodide (Iverson & Kelly Citation1940; Starr Citation1940; Dykstra Citation1941). More recently, Secor et al. (Citation1988) included several quaternary ammonium products, sodium hypochlorite, hydrogen peroxide, as well as phenol-, iodine-, and quinone-based products, in a study of disinfectant’s efficacies against C. m. sepedonicus in aqueous suspension. All of these products were effective in 10 min treatments of planktonic cell suspensions, although effectiveness of a number of the products was significantly reduced in the presence of organic matter (Secor et al. Citation1988). The three disinfectants chosen for our study – sodium hypochlorite, quaternary ammonium, and hydrogen peroxide – are not only registered and commercially available products commonly used in agriculture but also represent three different chemistries. Both sodium hypochlorite and hydrogen peroxide are strong oxidizers, whereas quaternary ammonium is a membrane-active agent (Ioannou et al. Citation2007). All three disinfectants used in this study were found to be totally effective against planktonic cells when used at recommended rates and exposure times, a result consistent with those of Secor et al. (Citation1988); however, statistical comparisons for planktonic treatments were not performed in this study as the work focussed on disinfectant performance against microbial biofilms.
In our study on C. m. sepedonicus biofilms grown using the MBEC™ Assay, sodium hypochlorite and hydrogen peroxide appeared to be faster acting than quaternary ammonium. For example, sodium hypochlorite and hydrogen peroxide were sometimes effective at 5 min and 10 min exposure times whereas the quaternary ammonium was not (). For this reason, when statistical comparisons were performed on data sets that included all exposure times and concentrations, sodium hypochlorite had a significantly higher mean log reduction (). However, this was likely a result of its fast-acting characteristic. When comparisons from only the 15-min exposure times were evaluated, all three compounds were equally effective at manufacturers’ recommended rates.
The surface materials used in the BEST™ assay in this study were representative of many types of storage and equipment surfaces on potato farms that potentially can become contaminated with ring rot bacteria. Potato planting, harvesting, and bin-piling equipment have steel, rubber, and plastic components, while storage bins generally have wooden walls and concrete floors. Our study revealed that surface properties have a significant effect on whether effective disinfection could be achieved. Polycarbonate was the easiest surface material to disinfect, and C. m. sepedonicus biofilms could be inactivated on it with a 5-min exposure to sodium hypochlorite and hydrogen peroxide, and 10 min with the slower acting quaternary ammonium. Interestingly, sodium hypochlorite was a less effective disinfectant of mild steel than hydrogen peroxide and quaternary ammonium. The assay used in our experiments allowed the mild steel coupons to become severely corroded after treatment with sodium hypochlorite. The oxidative reaction of sodium hypochlorite on steel appeared to diminish its bactericidal activity. Additionally, corrosion of mild steel is known to be more severe when colonized by certain types of biofilm-forming bacteria which could compromise disinfectant performance (Beech & Sunner Citation2004; Lewandowski & Beyenal Citation2009). Rubber, concrete and wood all represent porous surface materials, a physical property that significantly affected the performance of the disinfectants. Wood supported biofilms with the largest cell populations and was the most difficult material to disinfect. When data from an operational range of conditions (15-min exposures of 1× and 2× concentrations) were evaluated, sodium hypochlorite performed best on wood, followed by hydrogen peroxide, and quaternary ammonium, respectively.
To our knowledge, the significance of phytobacterial biofilms for farm hygiene and sanitation has not previously been explored or researched. Vascular bacterial plant pathogens of potato, such as C. m. sepedonicus, as well as Ralstonia solanacarum and Pectobacterium atrosepticum, are not known to establish significant or rapidly growing populations outside their plant hosts. Their presence on, and transmission via, farm equipment and other surfaces in the farm environment result largely from post-harvest spread of infected plant tissues in which biofilms of the bacterial pathogens developed during the crop growing season. Although bacteria in such disrupted or t-biofilms would be expected to maintain many of the biofilm features, disruption of the polymeric matrix may affect the ease with which biocides can penetrate into the biofilm structure or cause other disturbances affecting the properties of individual bacterial cells. In other biofilm disinfection experiments, infection tolerance was shown to be a function of the size of detached biofilm clusters (Behnke et al. Citation2011). Natural biofilms of C. m. sepedonicus that form in ring rot-infected potato tissues would be expected to include other endophytic bacteria occurring in potato vascular tissue as well as plant host cell components. However, in our experiments, artificial and natural t-biofilms did not appear to differ from one another with respect to their resiliency to biocide treatment. This finding suggests that cultures of C. m. sepedonicus grown on agar medium had biofilm properties not very different from in planta growth of the bacterium, and that future experiments on t-biofilms could use culture grown cells which are far easier to obtain and more uniform than in planta grown cells requiring propagation of infected plant material under containment conditions. Further analysis is needed to verify this observation.
The t-biofilms were more difficult to inactivate than in situ biofilms, but this may have been due, in part, to the fact that t-biofilms were air-dried on the surface materials being tested, in contrast to the in situ biofilms that were tested without drying prior to disinfection. Hydration of bacterial cells, particularly in the biofilm state, may be an important factor in achieving effective disinfection or eradication, and warrants further study.
In summary, we have shown that biofilms of C. m. sepedonicus, like biofilms of other bacteria, are considerably more resistant to disinfection when compared with corresponding planktonic cells. Additionally, some manufacturers’ label recommendations, which may result from testing on planktonic cells, are not sufficient for biofilm disinfection or eradication on some surfaces. Hence, recommendations for bacterial ring rot clean-up must take into account that effective disinfection or eradication of the biofilm populations (slime from diseased plant material) on machinery and equipment surfaces will not be possible when using concentrations and/or contact times as described on manufacturers’ product labels. Washing surfaces to remove organic matter has always been a recommended practice for sanitation of storages, but would be all the more important to physically eliminate adhering C. m. sepedonicus biofilm bacteria. The results of this work verify the importance of washing, because disinfectants applied to surfaces cannot be relied upon to entirely remove all infectious units, and most disinfectants would certainly be negatively affected by additional organic load contained within dirty surfaces. Disinfection using biocides, at appropriate concentrations and adequate exposure times, remains an important component of sanitation protocols to kill pathogenic bacteria that are not physically removed by washing and cleaning of contaminated surfaces. The results of this study suggest that recommended concentrations of biocides and exposure times, along with adjuvants that prolong contact or hydration, may need to be re-visited to ensure that biofilm populations can be disinfected or eradicated. Alternatively, repeated treatments could be considered, particularly in light of the high levels of tolerance that C. m. sepedonicus biofilms were shown to possess in this study.
Acknowledgements
The authors gratefully acknowledge Rong-Cai Yang and Joe J. Harrison for helpful discussions regarding statistical analyses. Assistance with the MBEC™ and BEST™ assays was generously provided by Nick Allan, Merle Olson and Amin Omar. This work was supported by the Canadian Food Inspection Agency (Project #C0602) and Alberta Agriculture and Forestry.
References
- Ali L, Khambaty F, Diachenko G. 2006. Investigating the suitability of the Calgary biofilm device for assessing the antimicrobial efficacy of new agents. Bioresource Technol. 97:1887–1893.
- Allan ND, Omar A, Harding MW, Olson ME. 2011. A rapid, high-throughput method for culturing, characterizing and biocide efficacy testing of both planktonic cells and biofilms. In: Méndez-Vilas A, editor. Science against microbial pathogens: communicating current research and technological advances. Badajoz: Formatex Res Centre.
- Bardouniotis E, Huddleston W, Ceri H, Olson ME. 2001. Characterization of biofilm growth and biocide susceptibility testing of Mycobacterium phlei using the MBEC™ assay system. FEMS Microbiol Lett. 203:263–267.
- Beech IB, Sunner J. 2004. Biocorrosion: Towards understanding interactions between biofilms and metals. Curr Opin Biotech. 15:181–186.
- Behnke S, Parker AE, Woodall D, Camper AK. 2011. Comparing the chlorine disinfection of detached biofilm clusters with those of sessile biofilms and planktonic cells in single- and dual-species cultures. Appl Environ Microbiol. 77:7176–7184.
- Bjarnsholt T, Jensen PO, Rasmussen TB, Christophersen L, Calum H, Hentzer M, Hougen H-P, Rygaard J, Moser C, Eberl L, et al. 2005. Garlic blocks quorum sensing and promotes rapid clearing of pulmonary Pseudomonas aeruginosa infections. Microbiology. 151:3873–3880.
- Buckingham-Meyer K, Goeres DM, Hamilton MA. 2007. Comparative evaluation of biofilm disinfectant efficacy tests. J Microbiol Meth. 70:236–244.
- Ceri H, Olson ME, Stremick C, Read RR, Morck D, Buret A. 1999. The Calgary biofilm device: new technology for rapid determination of antibiotic susceptibilities of bacterial biofilms. J Clin Microbiol. 37:1771–1776.
- Danhorn T, Fuqua C. 2007. Biofilm formation by plant-associated bacteria. Annu Rev Microbiol. 61:401–422.
- De Boer SH. 2008. Managing soft rot and ring rot. In: Johnson DA, editor. Potato health management. 2nd ed. St. Paul (MN): APS Press; p. 171–181.
- De Boer SH, Boucher A. 2011. Minireview/Minisynthèse prospect for functional eradication of the bacterial ring rot disease of potato. Can J Plant Pathol. 33:297–307.
- De Boer SH, Copeman RJ. 1980. Bacterial ring rot testing with the indirect fluorescent antibody staining procedure. Amer Potato J. 57:457–465.
- De Boer SH, Hall JW. 1996. The probability of detecting clavibacter michiganensis subsp. sepedonicus by indexing seed potato lots with serological tests. J Phytopathol. 144:459–463.
- De Boer SH, Slack SA, van den Bovenkamp GW, Mastenbroek I. 1996. A role for pathogen indexing procedures in potato certification. In: De Boer S, editor. Advances in botanical research: pathogen indexing technologies. London: Academic Press; p. 217–242.
- Dinesen IG. 1984. Effect of disinfectants on Corynebacterium sepedonicum (potato ring rot). Tidsskr Planteavl. 88:413–415.
- Dow JM, Crossman L, Findlay K, He Y-Q, Feng J-X, Tang J-L. 2003. Biofilm dispersal in Xanthomonas campestris is controlled by cell-cell signaling and is required for full virulence to plants. Proc Natl Acad Sci USA. 100:10995–11000.
- Dykstra TP. 1941. Results of experiments in control of bacterial ring rot of potatoes in 1940. Amer Potato J. 18:27–55.
- Fett WF. 2000. Naturally occurring biofilms on alfalfa and other types of sprouts. J Food Prot. 63:625–372.
- Harding MH, Howard RJ, Daniels GD, Mobbs SL, Lisowski SLI, Allan ND, Omar A, Olson ME. 2011. A multi-well plate method for rapid growth, characterization and biocide sensitivity testing of microbial biofilms on various surface materials. In: Méndez-Vilas A, editor. Science against microbial pathogens: communicating current research and technological advances. Badajoz: Formatex Res Centre.
- Harding MH, Marques LLR, Howard RJ, Olson ME. 2010. Biofilm morphologies of plant pathogenic fungi. Amer J Plant Sci Biotech. 4:43–47.
- Harrison JJ, Ceri H, Roper NJ, Badry EA, Sproule KM, Turner RJ. 2005a. Persister cells mediate tolerance to metal oxyanions in Escherichia coli. Microbiology. 151:3181–3195.
- Harrison JJ, Turner RJ, Ceri H. 2005b. Persister cells, the biofilm matrix and tolerance to metal cations in biofilm and planktonic Pseudomonas aeruginosa. Environ Microbiol. 7:981–994.
- Harrison JJ, Turner RJ, Ceri H. 2005c. High-throughput metal susceptibility testing of microbial biofilms. BMC Microbiol. 5:53.
- Harrison JJ, Turner RJ, Marques LLR, Ceri H. 2005d. Biofilms: A new understanding of these microbial communities is driving a revolution that may transform the science of microbiology. Amer Sci. 93:508–515.
- Ioannou CJ, Hanlon GW, Denyer SP. 2007. Action of disinfectant quaternary ammonium compounds against Staphylococcus aureus. Antimicrob Agents Chemother. 51:296–306.
- Iverson VE, Kelly HC. 1940. Control of bacterial ring rot of potatoes with special reference to ultraviolet-light method for selecting disease-free seed stock. MT State Coll Agric Exper Sta Bull. 386:1–15.
- Keren I, Shah D, Spoering A, Kaldalu N, Lewis K. 2004. Specialized persister cells and the mechanism of multidrug tolerance in Escherichia coli. J Bacteriol. 186:8172–8180.
- Koutsoudis MD, Tsaltas D, Minogue TD, von Bodman SB. 2006. Quorum-sensing regulation governs bacterial adhesion, biofilm development, and host colonization in Pantoea stewartii subspecies stewartii. Proc Natl Acad Sci USA. 103:5983–5988.
- Lewandowski Z, Beyenal H. 2009. Mechanisms of microbially influenced corrosion. In: Flemming H-C, Murthy PS, Venkatesan R, Cooksey K, Lewandowski Z, Beyenal H, editors. Marine and industrial biofouling. Berlin: Springer.
- Lewis K. 2001. Riddle of biofilm resistance. Antimicrob Agents Chemother. 45:999–1007.
- Mah T-F, Pitts B, Pellock B, Walker GC, Stewart PS, O’Toole GA. 2003. A genetic basis for Pseudomonas aeruginosa biofilm antibiotic resistance. Nature. 426:306–310.
- Marques LLR, Ceri H, Reid DM, Olson ME. 2002. Characterization of biofilm formation by Xylella fastidiosa in vitro. Plant Dis. 86:633–638.
- Marques LLR, De Boer SH, Ceri H, Lee E, Olson ME. 2003a. Susceptibility of Erwinia carotovora biofilms to antibiotics. Phytopathology. 93:S57 (abstr.).
- Marques LLR, De Boer SH, Ceri H, Olson ME. 2003b. Evaluation of biofilms formed by Clavibacter michiganensis subp. sepedonicus. Phytopathology. 93:S57 (abstr.).
- Morris CE, Monier J-M. 2003. The ecological significance of biofilm formation by plant-associated bacteria. Annu Rev Phytopathol. 41:429–453.
- Nelson GA. 1978. Survival of Corynebacterium sepedonicum on contaminated surfaces. Amer Potato J. 55:449–452.
- Nelson GA. 1980. Long-term survival of Corynebacterium sepedonicum on contaminated surfaces and in infected potato stems. Amer Potato J. 57:595–600.
- Olson ME, Ceri H, Morck DW, Buret AG, Read RR. 2002. Biofilm bacteria: formation and comparative susceptibility to antibiotics. Can J Vet Res. 66:86–92.
- Pan Y, Breidt F, Kathariou S. 2006. Resistance of Listeria monocytogenes biofilms to sanitizing agents in a simulated food processing environment. Appl Environ Microbiol. 72:7711–7717.
- Saitou K, Furuhata K, Kawakami Y, Fukuyama M. 2009. Biofilm formation abilities and disinfectant-resistance of Pseudomonas aeruginosa isolated from cockroaches captured in hospitals. Biocontrol Sci. 14:65–68.
- Secor GA, DeBuhr L, Gudmestad NC. 1988. Susceptibility of Corynebacterium sepedonicum to disinfectants in vitro. Plant Dis. 72:585–588.
- Snieszko SF, Bonde R. 1943. Studies on the morphology, physiology, serology, longevity and pathogenicity of Corynebcterium sepedonicum. Phytopathology. 33:1032–1044.
- Spoering A, Lewis K. 2001. Biofilms and planktonic cells of Pseudomonas aeruginosa have similar resistance to killing by antimicrobials. J Bacteriol. 183:6746–6751.
- Starr GH. 1940. Experimental work for the control of ring rot of potatoes. Amer Potato J. 17:318–322.
- Stewart PS. 2003. Diffusion in biofilms. J Bacteriol. 185:1485–1491.
- Sutton SW, Proud DW, Rachui S, Brannan DK. 2002. Validation of microbial recovery from disinfectants. PDA J Pharm Sci Tech. 56:255–266.
- Whiteley M, Ott JR, Weaver EA, McLean RJ. 2001. Effects of community composition and growth rate on aquifer biofilm bacteria and their susceptibility to betadine disinfection. Environ Microbiol. 3:43–52.
- Wong HS, Townsend KM, Fenwick SG, Trengove RD, O’Handley RM. 2009. Comparative susceptibility of planktonic and 3-day-old Salmonella typhimurium biofilms to disinfectants. J Appl Microbiol. 108:2222–2228.