Abstract
Wheat leaf rust, caused by Puccinia triticina Eriks., is of worldwide concern for wheat producers. The disease has been an annual problem for Canadian wheat producers since the early days of wheat cultivation in the 1800s, and research focused on combating this disease began in the early 1900s. Significant progress was made towards understanding the epidemiology of wheat leaf rust and developing genetic resistance in many countries worldwide. This review paper focuses exclusively on the research and development done in whole, or in part, in Canada. An integrated approach to controlling wheat leaf rust consisted of research in the following areas: the early research on wheat leaf rust in Canada, breeding and commercialization of high quality rust resistant wheat cultivars, discovery and genetic analysis of leaf rust resistance genes, the population biology and genetics of the P. triticina/wheat interaction. This review summarizes the research in each of these areas and the connections between the different aspects of the research. A multi-disciplinary team approach has been key to the advancements made within these diverse research fields in Canada since the early 1900s.
Résumé
La rouille brune, causée par Puccinia triticina Eriks., préoccupe les producteurs de blé partout dans le monde. La maladie a été un problème annuel récurent pour les producteurs canadiens depuis les débuts de la culture du blé dans les années 1800, et des chercheurs se sont efforcées de trouver des moyens de lutter contre cette maladie dès les années 1900. Des progrès majeurs ont été faits dans de nombreux pays quant à la compréhension de l’épidémiologie de la rouille brune et au développement de la résistance à cette maladie. Cet article de synthèse met exclusivement l’accent sur les recherches et les progrès accomplis au Canada, en tout ou en partie. Une approche intégrée relative à la lutte contre la rouille brune a consisté en recherches dans les domaines suivants: les premières recherches sur la rouille brune au Canada; la sélection et la commercialisation de cultivars de blé de grande qualité, résistants à la rouille; la découverte et l’analyse génétique des gènes de résistance à la rouille; la biologie des populations et la génétique des interactions blé-P. triticina. Cet article présente un aperçu de la recherche qui a été faite dans chacun de ces domaines et les liens qui existent entre les différents aspects de cette recherche. Une équipe multidisciplinaire a été la clé des progrès réalisés dans ces diverses sphères de recherche au Canada depuis le début des années 1900.
Early research on wheat leaf rust in Canada
The initial focus of rust research on wheat in Canada was primarily on stem rust (caused by Puccinia graminis f. sp. tritici Eriks. & Henn.) as a consequence of the devastating losses in Western Canada during the early years of wheat production, such as the severe epidemics in 1902, 1904, 1916, 1923, 1935 and 1938 (Craigie Citation1945). The stem rust epidemic of 1916 was particularly disastrous, with the loss of approximately half of the wheat crop, estimated at over $102 million dollars at that time (Johnson Citation1961b). Leaf rust infection, while not as damaging as stem rust, was also severe during many of these early years of wheat production, including 1921, 1925, 1927, 1930, 1932 and 1935, and was moderately severe in 1923, 1924, 1931, 1934 and 1937 (Craigie Citation1939). Between 1921 and 1937, annual losses caused by leaf rust were estimated at 7% yield reduction in Manitoba (Craigie Citation1939). Losses due to the combined effects of leaf and stem rust in Manitoba and Saskatchewan were estimated at 16.8% yield loss between 1925 and 1935, representing an average annual loss of $31 million dollars at that time (Greaney Citation1936), with a current (2015 inflated) value of $532 million. The most widely grown wheat cultivars during 1910–1935 were ‘Red Fife’ (1870 until approximately 1910) and ‘Marquis’ (1911 until 1939) (McCallum & DePauw Citation2008), which were susceptible to stem and leaf rust (Dickinson Citation1976). In response to yield losses due to leaf and stem rust, the Dominion Rust Laboratory was established in Winnipeg in 1925 (Johnson Citation1961b). Research was initiated on yield loss estimates, chemical control, epidemiology, breeding for resistance, host/parasite interactions, and the fundamental biology of economically important rust fungi. Early chemical control of rust on wheat was mainly through the use of sulphur dusts (Bailey & Greaney Citation1928), while later research involved synthetic chemicals (Peturson et al. Citation1958). The production of genetically resistant cultivars, by utilizing stem rust (Sr) and leaf rust (Lr) resistance genes, was sought as an alternative to chemical control because of the cost savings, the difficulty in applying fungicides, and environmental concerns.
Breeding and commercialization of high quality rust resistant cultivars
Genetic resistance to stem rust was first introduced with the registration of the cultivar ‘Thatcher’ in 1935, and subsequently of cultivars ‘Renown’ (registered in 1937), ‘Regent’ (registered in 1939) and ‘Redman’ (registered in 1946) (Peturson Citation1958). ‘Thatcher’ was the most popular cultivar and dominated the market in Canada from 1939 (when it surpassed ‘Marquis’) until 1968, when it was overtaken by ‘Manitou’ (McCallum & DePauw Citation2008). While ‘Thatcher’ was resistant to stem rust, it was very susceptible to leaf rust. ‘Renown’ was the first leaf rust resistant cultivar to be widely grown in western Canada, having the leaf rust resistance gene Lr14a (Samborski Citation1985). This gene provided effective resistance for several years but its effectiveness was lost by 1945 due to changes in the P. triticina population (Johnson & Newton Citation1946). Leaf rust epidemics in the 1930s and 1940s were shown to substantially reduce yield, grade, and protein content of these stem rust resistant cultivars, particularly ‘Thatcher’ (Peturson & Newton Citation1939; Peturson et al. Citation1945, Citation1948). ‘Thatcher’ was nearly immune to stem rust from the 1930s to the 1950s; however, it was susceptible to race 15B that significantly increased in frequency in 1952 (Peturson Citation1958). Concurrent epidemics of leaf rust and stem rust race 15B during the 1950s caused severe losses on wheat (Peturson Citation1958). ‘Selkirk’ (registered in 1953) was resistant to stem rust race 15B, and had improved resistance to leaf rust. Its yield losses were less severe than ‘Thatcher’ under heavy leaf rust pressure (Peturson Citation1958; Samborski & Peturson Citation1960). ‘Selkirk’, containing leaf rust resistance genes Lr10 and Lr14a and heterogeneous for Lr16 (Samborski Citation1985; Martens & Dyck Citation1989), was taken up rapidly by producers and grown extensively in the rust prone area of Manitoba and eastern Saskatchewan (). However, ‘Thatcher’ remained more popular in the western prairies due to its higher yield potential in areas with lower rust epidemics (McCallum & DePauw Citation2008). Virulence in P. triticini to Lr10 increased soon after the introduction of ‘Selkirk’, whereas virulence to Lr16 started to increase 8 years after ‘Selkirk’ was widely grown (Samborski Citation1985).
Fig. 1 Distribution of wheat varieties in Western Canada in 1956. Reprinted from Searle Grain Company Limited Annual Variety Survey Report 1956.
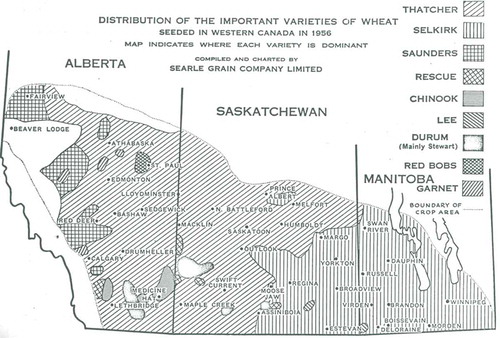
The subsequent leading cultivars in western Canada were ‘Manitou’ (1965), ‘Neepawa’ (1969) and ‘Katepwa’ (1981), all carrying Lr13 (Samborski Citation1985; Campbell & Czarnecki Citation1987) and were each the most popular from 1968 to 1972, 1973 to 1985, and 1986 to 1995, respectively. Virulence for Lr13 started to appear a few years after the introduction of ‘Manitou’ (Samborski Citation1985), and by 2002, nearly all isolates found in western Canada were virulent to this gene (McCallum & Seto-Goh Citation2005). Most of the popular cultivars developed since ‘Katepwa’ have a more diverse set of Lr genes, including the predominant cultivar from 1996–1997 ‘CDC Teal’ (Lr1, Lr13, Lr34) (Hughes & Hucl Citation1993; Liu & Kolmer Citation1997b) and the leading cultivar from 1998–2005 ‘AC Barrie’ (Lr13, Lr16) (McCaig et al. Citation1996; Kolmer Citation2001b). Other popular cultivars during this period were ‘Columbus’ (Lr13, Lr16) (Samborski & Dyck Citation1982), ‘Roblin’ (Lr1, Lr10, Lr13, Lr34) (Dyck Citation1993a), ‘Glenlea’ (Lr1, Lr13, Lr34) (Dyck et al. Citation1985), ‘AC Domain’ (Lr10, Lr12, Lr16), ‘AC Taber’ (Lr14a, Lr13, LrTb) (Liu & Kolmer Citation1997a), ‘AC Majestic’ (Lr13, Lr16), ‘AC Splendor’ (Lr13, Lr16), ‘AC Karma’ (Lr13, Lr16, LrTb) (Kolmer & Liu Citation2002), ‘Laura’ (Lr1, Lr10, Lr34), ‘Genesis’ (Lr13, Lr14a) and ‘Biggar’ (Lr13, Lr14a) (Kolmer Citation1994).
Information on the resistance genes present in current and past cultivars can be used to develop a comprehensive picture of leaf rust resistance in Canadian wheat cultivars, facilitating future resistance breeding. The genes Lr13, Lr14a, Lr16, Lr21 and Lr34 have been most widely used in Canadian cultivars (Martens & Dyck Citation1989; Kolmer et al. Citation1991; Kolmer Citation1996; McCallum & DePauw Citation2008). While virulence to Lr13, Lr14a, and to some extent to Lr16 has developed over time, Lr34 still provides effective resistance after many years of utilization. In Canada, ‘Glenlea’ (Lr1, Lr13 and Lr34) (Dyck et al. Citation1985) was the first major cultivar with Lr34 released in 1972 and it has remained moderately resistant to leaf rust since then. Over the years, the unique effectiveness and the durability of Lr34 were realized and it was subsequently incorporated into many cultivars. Most rust resistance genes are race-specific, conditioning resistance to some races of the pathogen but not others (Dyck & Kerber Citation1985). However, a few such as Lr34 are not race-specific, controlling all races equally well and have not been overcome by mutations to virulence in the pathogen (McCallum et al. Citation2011a). The proportion of the CWRS (Canadian Western Red Spring wheat, the dominant bread wheat class) area seeded to cultivars with Lr34 ranged from 20–40% from 1990 to 2009 (McCallum et al. Citation2011a). Dyck et al. (Citation1985) recognized that Lr34 conditioned resistance to stem rust as well as leaf rust in the cultivar ‘Glenlea’. This was the first report of the pleiotropic nature of Lr34, which was subsequently reported to also reduce stripe rust, stem rust and other biotrophic diseases in numerous publications worldwide (McCallum et al. Citation2012).
Other resistance genes that have maintained their effectiveness over time include Lr21 and Lr22a, isolated from Aegilops tauschii (Coss. syn. Triticum tauschii) and introgressed into bread wheat (Rowland & Kerber Citation1974). These genes have been deployed in cultivars such as ‘AC Cora’ (1994) (Lr13, Lr21), ‘AC Minto’ (1991) (Lr11, Lr13, Lr22a) (Townley-Smith et al. Citation1993a; Kolmer Citation1996), ‘McKenzie’ (1997) (Lr10, Lr13, Lr16, Lr21) (McCallum & Seto-Goh Citation2010), ‘5500HR’ (2000) (Lr22a+), ‘5600HR’ (1999) (Lr22a+) (Hiebert et al. Citation2007) and ‘Snowstar’ (Lr21+) (Humphreys et al. Citation2013). Virulence to Lr21 only recently (2011) appeared in the Canadian P. triticina population (McCallum et al. Citation2011c).
Combinations of resistance genes have often been found to confer more resistance than would be predicted from the effects of the genes in isolation. In the cultivar ‘Selkirk’, Lr13 was found to act synergistically with Lr16 and the gene combinations of Lr13+Lr30+Lr11 and Lr30+Lr3ka both resulted in more resistant seedling reactions than was expected if the genes were acting independently (Samborski & Dyck Citation1982). Many cultivars in Canada and the USA have combinations of resistance genes with Lr34 and/or Lr13 (Kolmer Citation1996). The expression levels of many other Lr genes are significantly enhanced when paired with Lr34 (German & Kolmer Citation1992) or Lr13 (Kolmer Citation1992c). Cultivar ‘Pasqua’ (1991) (Lr11, Lr13, Lr14b, Lr30, Lr34) (Dyck Citation1993b; Townley-Smith et al. Citation1993b) represents a deliberate attempt to combine multiple resistance genes into a single cultivar. It has remained highly resistant since its release in 1991 up to the present, even though Lr11, Lr13 and Lr14b became ineffective during that time and Lr30 and Lr34 only provide partial resistance when used individually (McCallum & Thomas Citation2011). The resistance gene Lr34 was also shown to enhance stem rust resistance in cultivars derived from ‘Thatcher’ (Kerber & Aung Citation1999). Furthermore, it was thought that Lr34 permitted the expression of stem rust resistance genes that were normally inhibited by a suppressor of stem rust resistance on chromosome 7D, i.e. Lr34 acted as a ‘nonsuppressor’ (Kerber & Aung Citation1995). The ‘nonsuppressing’ effect of Lr34 (or a closely linked gene) was also suggested by Dyck (Citation1987). Lr34 conferred stem rust and leaf rust resistance in ‘Glenlea’ (Dyck et al. Citation1985) and a number of other cultivars (Dyck & Samborski Citation1982). Navabi et al. (Citation2005a) found a significant contribution to both leaf and stripe rust resistance from Lr34/Yr18, or genes closely linked to this locus, in a number of CIMMYT (International Maize and Wheat Improvement Center) wheat cultivars. Hiebert et al. (Citation2011) showed that the resistance reaction to race Ug99 (TTKSK) of stem rust in the spring wheat variety ‘Peace’, associated with SrCad, was significantly lower in doubled haploid lines that also possessed Lr34.
A number of initially effective Lr genes deployed in Canada and throughout the world have become less effective because of the evolution of increased virulence in the P. triticina population. However, some Lr genes or gene combinations have proven durable over many years of wheat production. In order to understand what gene combinations conferred durable resistance, genetic analyses have been conducted on a number of durably resistant cultivars. One of these was the Australian cultivar ‘Cook’ that was found to have durable resistance to both leaf and stripe rusts because of the presence of Lr34/Yr18 interacting with other Lr genes (Navabi et al. Citation2005b). Another was the South American cultivar ‘Toropi’, whose complementary genes (LrTrp1 and LrTrp2) condition resistance to leaf and stripe rust (Barcellos Rosa Citation2012). Resistance in cultivars with multiple Lr genes (gene pyramids) has generally been durable. In Canada, the inheritance of leaf rust resistance has been investigated in many cultivars and breeding lines, including ‘Kenyon’ and ‘Buck Manantial’ (Dyck Citation1989), ‘Chinese Spring’ and ‘Sturdy’ (Dyck Citation1991), ‘Westphal 12A’ and ‘BH1146ʹ (Kolmer & Liu Citation2001), and accessions ‘V336ʹ and ‘V618ʹ from the Watkins wheat collection (Dyck & Jedel Citation1989). As changes in the races of P. triticina have rendered many of the resistance genes ineffective, the ongoing discovery of new Lr genes is needed for incorporation into future cultivars.
Wheat lines with unique leaf rust resistance genes such as Lr35 (Knox et al. Citation2000) have been released in Canada as germplasm lines for breeding. Resistance gene Lr36 from T. speltoides (Dvorak & Knott Citation1990) has been deployed in the Canadian cultivar ‘CDC Bounty’ (P. Hucl, personal communication). Durum wheat has traditionally been very resistant to P. triticina in Canada. However, races that specifically attack durum wheat have been causing significant losses in Mexico and other durum growing countries, and these races generally have been found to be virulent on Canadian durum cultivars. Efforts are underway to understand and improve resistance to these durum-attacking races in Canadian germplasm (Singh et al. Citation2013).
Genetic resistance to leaf rust has provided significant protection against losses in Canadian wheat. The results of long-term resistance breeding efforts in Canada were clearly evident when a collection of 45 Canadian wheat cultivars, released from the early 1900s to the present, were grown in side-by-side field plots for comparison (Martens et al. Citation2014). Leaf rust resistance in wheat cultivars has improved steadily over time through the incorporation of newer effective genes and the development of gene pyramids. This was reflected in the lower flag leaf infection and lower yield losses due to leaf rust of recent cultivars compared with the older more susceptible cultivars ().
Fig. 2 Relationship between year of release and average leaf rust (% of flag leaf coverage) severity over the period of 2008–2010 at Carman, Manitoba for 45 Canadian wheat cultivars. From Martens et al. (Citation2014).
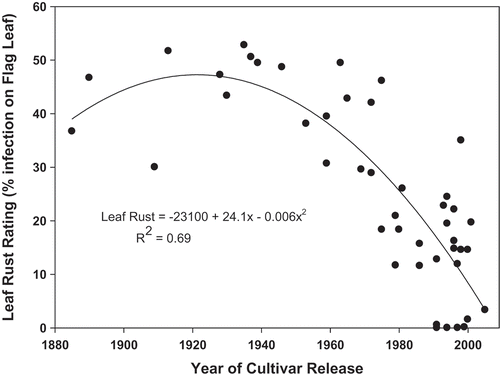
The development of ‘Thatcher’-based single Lr gene lines
Genetics of rust resistance is often complicated by the presence of multiple resistance genes in the same line. Additionally, the expression of these genes is often influenced by the genetic background of the host line. Near-isogenic lines, that contain single Lr genes in a uniformly susceptible background, greatly facilitate research to understand the genetics of host/parasite interactions. The development of near-isogenic single Lr gene lines in the ‘Thatcher’ wheat background was initiated by Dr R. G. Anderson (Anderson Citation1966) and performed mainly by Dr P. Dyck, working at the Cereal Research Centre, Agriculture and Agri-Food Canada (CRC-AAFC) (). ‘Thatcher’ was very susceptible to nearly all P. triticina virulence phenotypes, making it a highly suitable genetic background in which to incorporate these genes, although there are some very rare races avirulent on ‘Thatcher’. Dr Dyck crossed lines with unique Lr genes to ‘Thatcher’ and then used ‘Thatcher’ as the recurrent parent in backcrosses (usually to the backcross 5 generation) to create near-isogenic lines for many of the known leaf rust resistance genes. These lines are used as the official reference stocks for most of the leaf rust resistance genes (McIntosh et al. Citation1995). Many of the ‘Thatcher’ near-isogenic lines have been adopted in various parts of the world as components of differential sets for virulence surveys and genetic analysis of this pathosystem – this represents one of Canada’s most significant contributions to wheat leaf rust research globally. Because the ‘Thatcher’ near-isogenic lines simplified the genetics of differentials by placing them in a uniform background, they facilitate the careful investigation of the gene-for-gene relationship between the pathogen and the host. Furthermore, the widespread use of the same set of differential lines affords direct comparison of virulence in P. triticina populations across various laboratories. These lines have also been used extensively for tests of allelism to confirm the identity of hypothesized resistance genes in numerous cultivars (e.g. McCallum & Seto-Goh Citation2010).
Table 1. Near-isogenic lines of the wheat cultivar Thatcher with unique leaf rust resistance genes developed in Canada.
Discovery and genetic analysis of leaf rust resistance genes
The early development of rust resistant wheat cultivars in Canada highlighted the need to identify new and broadly effective sources of genetic resistance to incorporate into future cultivars. Canadian researchers have been among the world leaders in the discovery and characterization of leaf rust resistance genes. There are currently 69 recognized leaf rust resistance genes/alleles, many of which were characterized in whole or in part in Canada () (McCallum et al. Citation2012). These include Lr2a, Lr2b and Lr2c (Dyck & Samborski Citation1974), Lr3a (Dyck & Johnson Citation1983), Lr3bg and Lr3ka (Haggag & Dyck Citation1973), Lr12 and Lr13 (Dyck et al. Citation1966), Lr14a and Lr14b (Dyck & Samborski Citation1970), Lr16, Lr17a and Lr18 (Dyck & Samborski Citation1968), Lr19 (Sharma & Knott Citation1966), Lr21 and Lr22a (Rowland & Kerber Citation1974), Lr22b (Dyck Citation1979), Lr23 (McIntosh & Dyck Citation1975), Lr30 (Dyck & Kerber Citation1981), Lr32 (Kerber Citation1987, Citation1988), Lr33 (Dyck et al. Citation1987), Lr34 (Dyck Citation1987), Lr35 (Kerber & Dyck Citation1990), Lr36 (Dvorak & Knott Citation1990), Lr44 (Dyck & Sykes Citation1994), Lr52 (Hiebert et al. Citation2005), Lr53 (Marais et al. Citation2005), Lr56 (Marais et al. Citation2006), Lr59 (Marais et al. Citation2008), Lr60 (Hiebert et al. Citation2008), Lr62 (Marais et al. Citation2009), Lr63 (Kolmer et al. Citation2010), Lr64, Lr67 (Dyck et al. Citation1994; Hiebert et al. Citation2010b), Lr70 (Hiebert et al. Citation2014) and a number of Lr genes with temporary designations (Innes & Kerber Citation1994; McIntosh et al. Citation1995). These sources were developed by screening large germplasm collections of bread wheat (T. aestivum L.) and related species for leaf rust resistance, discovering the genetic basis of the resistance using defined P. triticina race collections, mapping the resistance gene to a specific chromosome arm, determining allelism with other genes in the identified region, and in some cases transferring this resistance into high quality bread wheat germplasm and cultivars.
Table 2. Leaf rust resistance genes discovered in whole or in part by Canadian researchers.
Most leaf rust resistance genes are effective from the early seedling stage throughout the life of the plant. Adult plant resistance genes, such as Lr12, Lr13, Lr22a and Lr34, are not normally expressed until the juvenile or adult plant growth stages. The genetics of adult plant resistance was first investigated in the cultivars ‘Exchange’ and ‘Frontana’ (Dyck et al. Citation1966). Field screening was critical to identify adult plant resistance in germplasm collections. Several T. aestivum germplasm collections have been screened for both seedling and adult plant leaf rust resistance, including the Watkins wheat collection (Claude et al. Citation1986; Dyck Citation1994a), Ethiopian wheat (Dyck & Sykes Citation1995), Italian collections (Jedel et al. Citation1988) and other germplasm collections (Shang et al. Citation1986; Dakouri et al. Citation2013).
Broad-spectrum leaf rust resistance was also identified in related species, which was then transferred into T. aestivum through inter-specific hybridization with closely and more distantly related species. Resistance was identified in Agropyron intermedium chromosomal translocation lines (Dyck & Friebe Citation1993), Triticum turgidum spp. dicoccoides (Dyck Citation1994b), Agropyron elongatum (Sharma & Knott Citation1966), Aegilops tauschii (Rowland & Kerber Citation1974; Kerber Citation1987; Innes & Kerber Citation1994), Ae. speltoides (Knott & Dvorak Citation1976, Citation1981), an amphiploid of Ae. speltoides × T. monoccocum (Kerber & Dyck Citation1990), and Thinopyrum intermedium through partial amphiploids with durum (Zeng et al. Citation2013). Leaf rust resistance was also successfully transferred from the durum cultivars ‘Medora’ and ‘Stewart’ to bread wheat, but results were not clear for a transfer from an autotetraploid of T. monococcum to bread wheat (Dyck & Bartos Citation1994). Sometimes, the introduction of chromosomal segments from related species caused a reduction in yield and grain quality (Knott & Dvorak Citation1976). These effects were investigated for a number of inter-specific introgressions (Knott & Dvorak Citation1981; Dyck & Lukow Citation1988). Numerous genetic tools, such as synthetic tetraploids and the use of colchicine to double the chromosomal complement, were also developed in wheat during the process of these interspecies transfers of rust resistance (Knott & Dvorak Citation1976). Two of the resistance genes transferred from Ae. tauschii (Lr21 and Lr22a) have been incorporated into many current Canadian cultivars and have provided effective resistance over time in a number of cultivars.
Molecular markers linked to many of the Lr genes provide opportunities for marker-assisted selection of leaf rust resistance. Molecular markers were developed in Canada for Lr1 (Cloutier et al. Citation2007), Lr13 (Hiebert et al. Citation2015), Lr16 (McCartney et al. Citation2005), Lr21 (J. Thomas unpublished), Lr22a (Hiebert et al. Citation2007), Lr25 and Lr29 (Procunier et al. Citation1995), Lr32 (Thomas et al. Citation2010), Lr34 (Dakouri et al. Citation2010), Lr35 (Gold et al. Citation1999), Lr52 (Hiebert et al. Citation2005), Lr60 (Hiebert et al. Citation2008), Lr67 (Hiebert et al. Citation2010b) and Lr70 (Hiebert et al. Citation2014). The various alleles at the Lr34 locus were analysed for their frequency and inheritance in Canadian (McCallum et al. Citation2011a) and international wheat germplasm (Dakouri et al. Citation2014). A potential evolutionary path for the diversity found at the Lr34 locus was also proposed by Dakouri et al. (Citation2014).
Two of the Lr genes that are common in Canadian germplasm, Lr13 and Lr16, have been the focus of recent studies. Lr13 is a race-specific adult plant resistance gene that can provide effective resistance in combination with other Lr genes. Genetic analysis and mutation studies have shown that Lr13 and Ne2 appear to be a single pleiotropic gene (Hiebert et al. Citation2015). Ne2 causes progressive necrosis in hybrids that also carry Ne1, a phenotype also referred to as hybrid necrosis. Lr16 can also confer effective resistance, particularly when combined with Lr34 (Hiebert et al. Citation2010a). Fine mapping of Lr16 has produced SNP markers based on resistance gene analogues (RGAs) that co-segregate with Lr16 in large populations (Kassa et al. Citation2014). These markers are useful for marker-assisted selection and map-based cloning of Lr16.
The leaf rust resistance gene Lr1 was cloned and sequenced by a research team led by Canadian researchers (Cloutier et al. Citation2007). It was found to belong to the coiled-coil nucleotide-binding site and leucine-rich repeat domain-containing class of resistance genes and is a member of a large gene family. However, the sequence of Lr1 was not related to either Lr10 or Lr21, the only other cloned seedling Lr genes to date. The cloned copy of Lr1 behaved in a dose-dependent manner in transgenic lines, expressing partial resistance in hemizygous lines and full resistance in homozygous lines. This correlates well with the reaction of non-transformed plants that are either heterozygous or homozygous for Lr1 in segregating populations (B. McCallum, unpublished data).
Understanding how these Lr genes interact with P. triticina avirulence genes to condition resistance requires a good understanding of the population biology of P. triticina, and detailed analysis of the host/parasite interaction using physiological and molecular genetic approaches.
Population biology and genetic analysis of Puccinia triticina
Population biology of Puccinia triticina
An initial step in understanding leaf rust epidemiology in wheat was to monitor epidemics of leaf rust and characterize the P. triticina population for its changing virulence profile. Knowledge about population diversity, race structure, and genetic lineages are extremely important for predicting new introductions and/or mutants that can overcome an effective host resistance gene. Since 1931, annual virulence surveys have been conducted in Canada through extensive field surveys during the growing season to estimate the severity and distribution of the disease. This collaborative effort involved researchers across the country. Infected leaf samples were collected annually during these surveys to obtain live rust isolates representative of the pathogen population. Purified isolates were then analysed for their virulence spectra by inoculating the above-mentioned differential wheat lines and observing the disease reactions. Detailed virulence information was used to track the evolution of new and potentially damaging races and to determine the effectiveness of the resistance genes in wheat cultivars in production as well as those that could be incorporated into future cultivars. When virulence surveys for P. triticina were initiated in Canada in 1931, virulence phenotypes or races were originally given numbers based on their reaction to the eight existing standard differentials (Newton & Johnson Citation1941), which carried resistance genes Lr1, Lr2a, Lr2c, Lr3 and Lr11. Modified race designation systems (UN or Unified Numeration and International Standard) evolved over time using Lr1, Lr2a, Lr2c and Lr3 to differentiate virulence phenotypes (Kolmer Citation1989). The use of various wheat cultivars or lines as differentials confounded the interaction between the resistance and avirulence genes due to the presence of multiple resistance genes in some lines and the effect of various genetic backgrounds. The development of the ‘Thatcher’ based near-isogenic lines simplified the host/parasite genetics of this system, since each line had a single unique resistance gene. The currently used North American race designation system uses a four letter code system to define the reaction on 16 of these ‘Thatcher’ single Lr gene near-isogenic wheat lines (Set 1: Lr1, Lr2a, Lr2c, Lr3; Set 2: Lr9, Lr16, Lr24, Lr26; Set 3: Lr3ka, Lr11, Lr17, Lr30; (Long & Kolmer Citation1989), Set 4 [subsequently added]: LrB, Lr10, Lr14a and Lr18).
As a result of ongoing annual surveys, Canada has developed a continuous historical record of the P. triticina population from 1931 to the present day. These annual surveys were published in the Canada Department of Agriculture reports (1931–1960), the Canadian Plant Disease Survey (1960–1979) and the Canadian Journal of Plant Pathology (1980–present), and were summarized for the periods of 1931–1955 (Johnson Citation1956), 1956–1987 (Kolmer Citation1989) and 1987–1997 (Kolmer Citation1999). The annual virulence surveys since 1997 (Kolmer Citation2001b; McCallum & Seto-Goh Citation2002, Citation2003, Citation2005, Citation2006a, Citation2006b, Citation2008, Citation2009; McCallum et al. Citation2004, Citation2010, Citation2011b, Citation2013) have not been summarized to date. During the period 1931–1955, distinct distributions of virulence phenotypes were found in each of the geographic regions of Canada. The regions were defined as Eastern Canada (all provinces east of Manitoba), the Prairies (Manitoba, Saskatchewan and Alberta) and British Columbia (Johnson Citation1956). Over 90% of the wheat-growing area in Canada is in the three Prairie provinces (McCallum & DePauw Citation2008). The wheat cultivars grown in the Prairies from 1931–1955 appeared to have had a strong selective effect on the virulence phenotypes in the region over time (Johnson Citation1956). A similar effect was noticed for the period 1956–1987 (Kolmer Citation1989). Selection was the strongest in this region because there was an emphasis on using genetic resistance to leaf rust in the cultivars grown in the prairies of Canada and the USA.
Cereal rust pathogens are often able to change genetically, through mutation and sexual or asexual recombination, to become virulent to resistance genes present in the host. This process was termed ‘Man-guided evolution’ (Johnson Citation1961a) because resistance genes deployed in prevalent wheat cultivars led to the appearance and selection of phenotypes with virulence to these resistance genes. Puccinia triticina populations in Canada have evolved virulence over time to many of the resistance genes used in Canadian wheat cultivars, such as Lr1, Lr10, Lr12, Lr13 and Lr14a. Lr14a was effective when initially deployed in ‘Renown’ (1937), but cultivars with this gene were susceptible by 1945 (Johnson & Newton Citation1946). When ‘Selkirk’, with Lr10, Lr14a, and heterogeneous for Lr16 (Samborski Citation1985; Martens & Dyck Citation1989), became widely grown in 1955 (McCallum & DePauw Citation2008), there was selection for virulence detected for Lr10 and Lr16. The frequency of isolates virulent to Lr10 increased rapidly from 0% in 1952 to 80% of the isolates in 1958 (Anderson Citation1961). However, isolates virulent to Lr16 were not detected until 1962, reaching a high of 56% in 1967. Virulence to Lr16 subsequently declined to near 0% (Samborski Citation1985) after ‘Selkirk’ was replaced in 1968 with ‘Manitou’ followed by ‘Neepawa’ and ‘Katepwa’ (all with Lr13). Virulence to Lr13 soon increased (Kolmer Citation1989) with the introduction of these cultivars. Virulence to Lr16 disappeared entirely in Manitoba and Saskatchewan from 1989–1994 but reappeared after 1995, reaching a high of 74% in 2001 when ‘AC Barrie’ (Lr13, Lr16) was the predominant cultivar (McCallum & Seto-Goh Citation2005; McCallum & DePauw Citation2008). After 2001, virulence to Lr16 declined again to low levels; however, ‘AC Barrie’ and single gene lines with Lr16 remained susceptible in the field, indicating that most virulence phenotypes were likely heterozygous for Lr16 virulence (McCallum & Seto-Goh Citation2005) which resulted in an intermediate infection type. Heterozygosity for virulence was found to be common for many resistance genes in the Canadian P. triticina population (Kolmer Citation1992d).
The P. triticina populations in western and eastern Canada are fairly distinct both for virulence (Kolmer Citation1991a, Citation1992a) and molecular markers (Kolmer et al. Citation1995; Kolmer Citation2001a). The western population was found to diverge significantly from the eastern population after 1937 as a consequence of the selective effect of resistant cultivars commonly grown in the Prairies, which also reduced the diversity of the prairie population (Kolmer Citation1991b). The P. triticina populations in Canada were characterized by distinct clusters of isolates that had similar virulence and molecular phenotypes within clusters (Kolmer et al. Citation1995), and demonstrated strong linkage disequilibrium between loci because of the selection for virulence and the asexual nature of the population. Linkage disequilibrium was higher in the more selected western population than in the eastern population. The eastern population was subject to less intense selection for virulence, and may have undergone some level of sexual recombination (Kolmer Citation1992b), which would have significantly reduced linkage disequilibrium (Liu & Kolmer Citation1998). Kolmer (Citation1992a) found that there were significant differences in the frequency of virulence phenotypes between Ontario and Quebec collections of P. triticina from 1990, and those from winter and spring wheat in eastern Canada. Manitoba and Saskatchewan collections were not significantly different from each other, but were different from those from eastern Canada. Some common virulence and molecular phenotypes are still found annually in both western and eastern Canada, which could be due to a common origin in the USA (McCallum & Seto-Goh Citation2006a, Citation2006b). Wang et al. (Citation2010a) analysed the P. triticina populations in Canada from 1997 to 2007 and found that the Quebec and Ontario population was distinct from that in Manitoba and Saskatchewan and the isolates formed six genetically similar clusters similar to those found in the USA.
Selection for virulence was investigated using a mixture of virulence phenotypes cultured for eight asexual uredinial generations on host lines with different combinations of resistance genes (Kolmer Citation1990). Changes in the populations observed on some lines over time were thought to be due to differences in urediniospore production between the virulence phenotypes (fitness), rather than selection for virulence per se. However, selection for virulence reduced the diversity of a randomly produced sexual population in response to resistance genes in the host cultivar (Kolmer Citation1993). Selection of a sexual population on multi-lines with various combinations of resistance genes demonstrated a predominance of virulence phenotypes heterozygous for virulence, and that unnecessary genes for virulence, in which the corresponding resistance genes were not present, were not deleterious to fitness (Kolmer Citation1995). Selection for virulence to Lr16 on wheat lines carrying this gene was demonstrated in a greenhouse study with a mixture of virulence phenotypes (Kolmer Citation1990). Because P. triticina inoculum is blown northward into Canada from the USA, the distribution of virulence phenotypes in Canada is also influenced by the Lr genes in wheat cultivars grown in the USA. Virulence to Lr24, Lr17 and other genes has appeared at relatively high levels in Canadian P. triticina populations even though these genes have not been deployed in Canadian wheat cultivars (Kolmer Citation1989, Citation1999; McCallum & Seto-Goh Citation2006b). This was hypothesized to be a consequence of the selective effect of wheat cultivars carrying those genes in the USA (). Virulence for Lr9 and Lr21 has only evolved recently in Canada (in 2006 [McCallum & Seto-Goh Citation2009] and 2011 [McCallum et al. Citation2011c], respectively), and has remained relatively low to date, whereas virulence to Lr24, Lr17 and Lr2a fluctuates over time but is relatively high in the Canadian P. triticina population (). The Canadian population of P. triticina was also tested annually for virulence to adult plant resistance genes Lr12, Lr13, Lr22a, Lr34, Lr35 and Lr37. The frequency of virulence to Lr12, Lr13 and Lr37 was very high, whereas it was normally less than 10% on Lr35, and no virulent isolates were found for Lr22a and Lr34 (McCallum & Seto-Goh Citation2005, Citation2006a, Citation2006b, Citation2008, Citation2009; McCallum et al. Citation2010, Citation2011b, Citation2013; B. McCallum, unpublished data).
Fig. 3 Virulence frequency to leaf rust resistance genes Lr2a, Lr9, Lr16, Lr17, Lr21 and Lr24 in the Canadian Puccinia triticina population 2000–2013. Data from McCallum & Seto-Goh Citation2003, Citation2004, Citation2005, Citation2006a, Citation2006b, Citation2008, Citation2009; McCallum et al. Citation2010, Citation2011b, Citation2013, and B. McCallum (unpublished data).
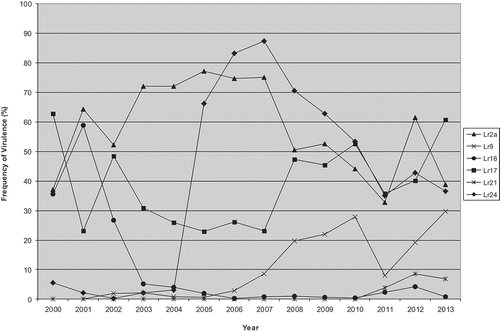
Puccinia triticina in Canada evolved new combinations of virulence relatively rapidly, resulting in a highly variable pathogen population. For example, 22 to 72 different P. triticina virulence phenotypes were identified annually in Canadian surveys from 2000 to 2013 (sample sizes varied from 97 to 420 isolates) (McCallum & Seto-Goh Citation2002, Citation2003, Citation2004, Citation2005, Citation2006a, Citation2006b, Citation2008, Citation2009; McCallum et al. Citation2010, Citation2011b, Citation2013; B. McCallum, unpublished). During this time, 305 unique virulence phenotypes were identified in Canada, based on the standard set of 16 ‘Thatcher’ near-isogenic differential wheat lines. Even more variation was found when these virulence phenotypes were tested on race-specific adult plant resistance genes for the 2000–2013 period listed above, and during earlier years (Kolmer Citation1997). Moreover, the predominant virulence phenotypes changed regularly, although there was some continuity from year to year (). This variability could have arisen from a variety of sources, including mutation, host selection, and sexual, asexual or parasexual recombination. The potential for sexual recombination in P. triticina was first investigated in Canada by Brown and Johnson (Citation1949). They determined the relative abilities of various species of Thalictrum, native to North America, to act as alternate or sexual hosts for P. triticina and made initial selfing studies for the predominant races of the time. These authors thought that while sexual reproduction of P. triticina on Thalictrum species in North America was not common, it could occasionally occur and lead to diversity in the P. triticina population. They discovered that many of the races were heterozygous for avirulence, since the selfed progeny populations segregated for virulence to many of the differentials. Canadian populations were compared with other worldwide collections of P. triticina, and some of the Canadian race groups clustered with similar groups of races from other regions (Kolmer & Liu Citation2000).
Table 3. Frequency (%) of predominant P. triticina virulence phenotypes in Canada from 2000 to 2013.
Results from more than 75 years of annual virulence surveys of P. triticina in Canada demonstrated the diverse and rapidly changing nature of these populations. Various factors have contributed to this dynamic population structure, but the primary drivers are mutation and host selection. Selection for virulence on host resistance genes has been observed in the virulence surveys over time and demonstrated in greenhouse simulations. The fact that P. triticina populations have evolved to overcome many of the resistance genes used in Canadian cultivars demonstrates that there is an ongoing need to identify and incorporate new sources of genetic resistance.
Genetic analysis of Puccinia triticina
The genetic basis of avirulence in P. triticina was investigated at great length in the 1960s and 1970s by Drs Samborski and Dyck (CRC-AAFC). Puccinia triticina genetically is a dikaryotic fungus, which has been termed a functional diploid. Populations of the pathogen that segregate for virulence were generated through a series of selfing crosses (since many virulence/avirulence loci are heterozygous in most isolates) and genetic crosses between different virulence phenotypes on Thalictrum. The parents and progeny were then screened for virulence/avirulence on host lines with single genes for resistance in the ‘Thatcher’ background. Populations from self-fertilized isolates of four different virulence phenotypes were evaluated for virulence on the eight standard wheat differential lines used in the 1960s (Samborski & Dyck Citation1968). Avirulence to most of these lines was conditioned by single dominant genes, although there were some cases of avirulence being recessive and where additional genes affected the disease response. A second study involving these eight differential wheat lines that used cross-fertilized and self-fertilized P. triticina populations (Haggag et al. Citation1973) also supported the hypothesis of avirulence being primarily conditioned by single dominant genes. Since some of these differential lines contained more than one resistance gene, the interpretation was confounded by more than one specific gene-for-gene interaction. These results were confirmed with hybrid P. triticina populations using near-isogenic backcross lines in the ‘Thatcher’ background with single resistance genes (Samborski & Dyck Citation1976). Avirulence to the adult plant resistance gene Lr22b, found in ‘Thatcher’, was also shown to be conditioned by a single dominant gene (Bartos et al. Citation1969).
Development of a genetic linkage map for P. triticina using avirulence and molecular markers (McCallum et al. Citation2004) should facilitate studies on the fundamental nature of avirulence genes. The cloning of defined avirulence genes in P. triticina should provide insight into the nature of the resistance responses and identification of the resistance genes in T. aestivum that interact with these avirulence genes. Using Simple Sequence Repeat (SSR) markers (Wang et al. Citation2006, Citation2010b) and Amplified Fragment Length Polymorphism (AFLP) markers, initial linkage groups were established in the P. triticina mapping population produced by Samborski and Dyck (Citation1968). Recent next-generation DNA sequencing methods have generated the complete genome sequences of 57 progeny of P. triticina cross Race 1 × Race 9 (Samborski & Dyck Citation1968) and over 20,000 Single Nucleotide Polymorphisms (SNPs) have been revealed, creating numerous markers, some of which are linked to potential avirulence genes (Bakkeren, McCallum, Joly, Mulock, unpublished data). In addition to asexual and sexual reproduction, P. triticina can reproduce through parasexual or somatic recombination where somatic hyphae from two different isolates can fuse and combine nuclei, ultimately producing new genotypes different from either parent (Wang & McCallum Citation2009).
Improved DNA and RNA sequencing technologies have now made possible the detailed genetic analysis of P. triticina and other cereal rusts. Initially, a large library of expressed sequence tags (ESTs) from P. triticina was developed and sequenced to reveal the expressed genes and their relative abundance in the various spore stages and in isolated haustoria of the fungus (Xu et al. Citation2011). Deep sequencing of the genome and transcriptome of a number of P. triticina isolates has allowed comparative genomics with related fungal species and the identification of genes critical to the infection process (Bakkeren et al. Citation2012; Bruce et al. Citation2014).
The Puccinia triticina/wheat interaction
The interaction between P. triticina and wheat is influenced by a number of factors, including temperature, genetic background of the host, genetics of avirulence in P. triticina, and the interaction between resistance and avirulence genes. Johnson and Newton (Citation1937) demonstrated that very high temperatures inhibited pustule development of P. triticina. Newton and Johnson (Citation1941) determined that moderate temperature rises differentially affected the reactions of resistant lines, with some becoming more resistant and others becoming more susceptible. Dyck and Johnson (Citation1983) used single resistance gene lines in the ‘Thatcher’ background to determine the temperature sensitivity, in the 10–20°C range, of the resistance genes known at that time. Not only did temperature sensitivity vary between genes, but as the temperature increased, most genes became less effective while some were unaffected and others became more effective.
The expression of host resistance can also be influenced by the growth stage of the plant. Newton and Johnson (Citation1943) were the first Canadian researchers to identify adult plant resistance to leaf rust in a number of cultivars, including ‘Thatcher’ and ‘Marquis’. This resistance was not expressed at the seedling stage but was expressed as the plant matured to the jointing and flag leaf stages. Resistance in the cultivars ‘Exchange’ and ‘Selkirk’ was found to increase from the seedling to the adult plant stage but declined as the plant matured (Samborski & Ostapyk Citation1959). The adult plant resistance in ‘Thatcher’ and ‘Marquis’ was later determined to be a single recessive gene (Bartos et al. Citation1969). This gene was subsequently found to be allelic to Lr22 that was transferred from Ae. tauschii, and was designated Lr22b while the Ae. tauschii allele received the Lr22a designation (Dyck Citation1979). Adult plant resistance was also genetically characterized in ‘Exchange’ (Lr12) and ‘Frontana’ (Lr13) (Dyck et al. Citation1966), and PI250 413 (Lr67) (Dyck & Samborski Citation1979; Hiebert et al. Citation2010b). The most important of the adult plant resistance genes, Lr34, was isolated from the cultivars ‘Terenzio’, ‘Lageadinho’ and ‘PI58548’ (Dyck Citation1987). It has subsequently been found to be widely distributed in Canadian germplasm and is one of the most common resistance genes worldwide (McCallum et al. Citation2011a; Dakouri et al. Citation2013), conferring durable leaf rust resistance. Analysis of adult plant resistance genes is time and space consuming, because of the need to grow plants to maturity to determine rust reaction phenotypes. Recently, a technique was developed to inoculate separate tillers on a single plant with different races, improving the efficiency of adult plant resistance gene phenotyping by permitting the same plant to be used for testing multiple races (Rosa et al. Citation2014).
In the wheat–P. triticina pathosystem, most Lr genes are single dominant genes, and most avirulence genes are inherited as single dominant genes (Samborski Citation1985). However, both avirulence and resistance for a number of gene pair combinations demonstrated incomplete dominance. Puccinia triticina isolates heterozygous for virulence to a particular resistance gene often produce intermediate reactions on host plants carrying this gene (Samborski Citation1963; Kolmer & Dyck Citation1994). Similarly, many resistance genes confer an intermediate level of resistance when the host line is heterozygous (Kolmer & Dyck Citation1994).
Physiology of the Puccinia triticina/wheat interaction
Physiology of the P. triticina/wheat interaction was first investigated by using detached wheat leaves, inoculated with P. triticina and floated on solutions containing benzimidizole (Samborski et al. Citation1958) with various additives (Samborski & Forsyth Citation1960). Some enzyme inhibitors and metabolites prevented the development of rust pustules. A subsequent study analysing host metabolism determined that most fungus inhibiting compounds were found to also affect the host (Samborski et al. Citation1961). Significant changes in the physiology of leaf rust-infected wheat leaves, compared with uninfected leaves, included an increase in ribonuclease activity (Rohringer et al. Citation1961), which was also higher in the susceptible or compatible interaction than in the resistant or incompatible interaction.
The interactions of the ‘Thatcher’ near-isogenic lines for Lr2a, Lr3, Lr9 and LrB with various races of P. triticina were analysed microscopically to determine the timing and extent of resistance responses, such as lignin and callose deposition and host cell death (Wang et al. Citation2013). These observations were correlated to the development and growth of the fungus within the host tissues to understand how these resistance genes slow down and sometimes stop the growth of the rust fungus within the host leaves. Future studies should determine how these are coordinated to condition resistance which will hopefully lead to more durable forms of genetic resistance.
Gene expression and silencing in the Puccinia triticina/wheat interaction
Gene expression in the P. triticina/wheat interaction was analysed using Lr1 interacting with Avr1 (Fofana et al. Citation2007). Significant differences were found between compatible and incompatible interactions using microarrays with 7728 ESTs hybridized with RNA from compatible or incompatible interactions involving host lines with Lr1 and P. triticina isolates with or without Avr1, sampled at different time points after inoculation. A similar but more comprehensive analysis conducted using the Affymetrix GeneChip® wheat genome array comprising more than 60,000 probe sets revealed the central role of COI1, the primary component of jasmonic acid signalling, and the shift towards carbon conservation 24 hours after infection (Kumar et al. Citation2014).
Another approach to better understand the P. triticina/wheat interaction has focused on the biology and genetics of the pathogen. To obtain a gene catalogue, an EST library was developed from various spore stages of P. triticina and from the P. triticina/wheat interaction including isolated haustoria of the fungus (Hu et al. Citation2007a; Xu et al. Citation2011). These ESTs have proven to be a rich source from which SSR markers can be derived (Wang et al. Citation2010b). Since ESTs are derived from expressed genes, a correlation with gene expression was tentatively made and dramatic differences in the transcriptomes of the various spore and infection stages were found. Several candidate pathogenicity genes were identified based on comparative analyses with other model pathogens (Hu et al. Citation2007b).
Since genetic manipulations such as gene deletion and genetic transformation are as yet not feasible in P. triticina, performing functional gene studies is challenging. To address this challenge, a novel Host Induced Gene Silencing (HIGS) approach was developed (Panwar et al. Citation2013a, Citation2013b), where pathogen gene sequences are expressed in the host but post-transcriptional gene silencing produces a suppressed fungal gene phenotype. Disease severity has been significantly reduced by silencing sequences targeted to predicted P. triticina pathogenicity genes. When these genes were effectively silenced, disease symptoms were reduced after leaf, stem or stripe rust fungal inoculations. This confirmed the role of these fungal genes in pathogenicity but also showed that this approach could possibly lead to alternative ways for crop protection (Bakkeren et al. Citation2012; Panwar et al. Citation2013a). Through collaboration with Canadian researchers, the complete draft genome sequence of P. triticina race 1 (BBBD) was publicly released in 2010 (http://www.broadinstitute.org). This resource has supported the P. triticina genetic mapping and has allowed comprehensive analyses of pathogenicity and virulence factors of this fungus (Fellers et al. Citation2013; Bruce et al. Citation2014).
Proteomics of the Puccinia triticina/wheat interaction
A relatively new tool for analysing pathosystems at the molecular level is proteomics. Unlike genomics methods, proteomics measures proteins directly, along with changes in their abundance and post-translational modification status both in space and time. This is a tall order, and most progress has been made in model-organisms fueled by advances in mass spectrometry and bioinformatics. However, the wheat-rust pathosystem has no suitable model for comparison to facilitate progress. The first forays into rust proteomics, although the term had not yet been coined, were made at the Cereal Research Centre by Kim et al. (Citation1982) and Howes et al. (Citation1982). They compared the proteomes of resting and germinated urediospores, respectively, of P. graminis f. sp. tritici by 2-D gel electrophoresis, and demonstrated that there were race-specific differences in the protein patterns on the gels. However, at that time they were unable to identify any of the proteins, and the significance of their discovery remains unknown.
The first recent publication of the proteome of an incompatible interaction between P. triticina and the wheat cultivar ‘Thatcher’ highlighted more of the difficulties still being faced (Rampitsch et al. Citation2006). At the time, P. triticina Race 1 had not yet been sequenced, necessitating protein identification through cross-species database searching, and manual de novo sequencing of peptides for which no credible match could be found. In addition, using 2-D gels of whole unenriched tissue resulted in superficial proteome coverage. All of the important biochemical events occur at levels far below the sensitivity of the approach available at the time. This prompted research into enriched haustoria (Song et al. Citation2011), which revealed many more proteins. It was clear that more work would be needed to provide details on the biochemistry of this pathosystem. This work led to the production of monoclonal antibodies to enable purification of Race 1 (BBBD) haustoria to near-homogeneity (Rampitsch et al. Citation2015). The isolation of the haustorial proteome of this race can now be used in conjunction with the sequenced genome to identify proteins and genes which could be involved in pathogenesis. These antibodies were used to compare the proteomes of haustoria from different races of P. triticina, which could be the key to identifying the proteins associated with the virulence differences between the races (Rampitsch et al. Citation2013).
Summary
Research on wheat leaf rust in Canada has been conducted for more than a century, generating tremendous knowledge and resources. Progress has been made on controlling wheat leaf rust through an integrated approach with the development of leaf rust resistant cultivars, identification of novel resistance sources, understanding virulence dynamics of the P. triticina population, and analysing the host/parasite interaction. Resistant cultivars were developed for Canadian farmers through the use of available effective genes, the incorporation of genes from related species into adapted wheat backgrounds (e.g. ‘Thatcher’), and gene pyramiding. Monitoring virulence in the P. triticina population in Canada annually has been crucial for the early detection of virulence to resistance genes used in cultivars, and has demonstrated the selective effect of resistance genes used in Canada and the USA on the pathogen population. A multidisciplinary team of researchers has determined the underlying genetic basis for many resistance and avirulence genes, and the complex interaction between these genes. The body of knowledge generated by pioneering Canadian rust scientists has been capitalized upon by successive generations of dedicated scientists in Canada and around the world, to further understand the P. triticina/wheat system towards even more effective and durable genetic control of leaf rust in the future.
Acknowledgements
We thank Pat Seto-Goh, Barbara Mulock, Elsa Reimer and Winnie McNabb for excellent technical assistance on the recent wheat leaf rust pathology portions of this research. Thanks also to Mira Popovic, Jadwiga Budzinski, Sasanda Nilmalgoda and Ghassan Mardli, Eva Beimcik, Leslie Bezte, Rob Linning, Abdulsalam Dakouri and David Joly for their contributions to this research.
Additional information
Funding
References
- Anderson RG. 1961. The inheritance of leaf rust resistance in seven varieties of common wheat. Can J Plant Sci. 41:342–359.
- Anderson RG. 1966. Studies on the inheritance of resistance to leaf rust of wheat. Hereditas. 2:144–155.
- Bailey DL, Greaney FJ. 1928. Dusting with sulphur for the control of leaf and stem rust of wheat in Manitoba. Sci Agric. 8:409–432.
- Bakkeren G, Song X, Panwar V, Linning R, Wang X, Rampitsch C, McCallum B, Fellers J, Saville B. 2012. Functional genomic approaches in cereal rusts. Can J Plant Pathol. 34:3–12.
- Barcellos Rosa S. 2012. The genetics of leaf rust resistance in the durably resistant wheat cultivar ‘Toropi’ [Ph.D. dissertation]. Winnipeg: University of Manitoba.
- Bartos P, Dyck PL, Samborski DJ. 1969. Adult-plant leaf rust resistance in Thatcher and Marquis wheat: a genetic analysis of the host-parasite interaction. Can J Bot. 47:267–269.
- Brown AM, Johnson T. 1949. Studies on variation in pathogenicity in leaf rust of wheat, Puccinia triticina Erikss. Can J Res. 27c:191–202.
- Bruce M, Neugebauer KA, Joly DL, Migeon P, Cuomo CA, Wang S, Akhunov E, Bakkeren G, Kolmer JA, Fellers JP. 2014. Using transcription of six Puccinia triticina races to identify the effective secretome during infection of wheat. Front Plant Sci. 4:520. doi:10.3389/flps.2013.00520
- Campbell AB, Czarnecki E. 1987. Katepwa hard red spring wheat. Can J Plant Sci. 67:229–230.
- Claude PP, Dyck PL, Evans LE. 1986. An evaluation of 391 spring wheat introductions for resistance to stem rust and leaf rust. Can J Plant Pathol. 8:132–139.
- Cloutier S, McCallum BD, Loutre C, Banks TW, Wicker T, Feuillet C, Keller B, Jordan MC. 2007. Leaf rust resistance gene Lr1, isolated from bread wheat (Triticum aestivum L.) is a member of the large psr567 gene family. Plant Mol Biol. 65:93–106.
- Craigie JH. 1939. Economic diseases of field crops in Manitoba. Bot Plant Path Sci Service. 574:5–37.
- Craigie JH. 1945. Epidemiology of stem rust in Western Canada. Sci Agric. 25:285–401.
- Dakouri A, McCallum B, Walichnowski A, Cloutier S. 2010. Fine-mapping of the leaf rust Lr34 locus in Triticum aestivum (L.) and characterization of large germplasm collections support the ABC transporter as essential for gene function. Theor Appl Genet. 121:373–384.
- Dakouri A, McCallum BD, Cloutier S. 2014. Haplotype diversity and evolutionary history of the Lr34 locus of wheat. Mol Breed. 33:639–655.
- Dakouri A, McCallum BD, Radovanovic N, Cloutier S. 2013. Molecular and phenotypic characterization of seedling and adult plant leaf rust resistance in a world wheat collection. Mol Breed. 32:663–677.
- Dickinson FL. 1976. Prairie wheat. Three centuries of wheat varieties in Western Canada. Winnipeg (Canada): Canada Grains Council.
- Dvorak J, Knott DR. 1990. Location of a Triticum speltoides chromosome segment conferring resistance to leaf rust in Triticum aestivum. Genome. 33:892–897.
- Dyck PL. 1979. Identification of the gene for adult plant leaf rust resistance in Thatcher. Can J Plant Sci. 59:499–501.
- Dyck PL. 1987. The association of a gene for leaf rust resistance with the chromosome 7D suppressor of stem rust resistance in common wheat. Genome. 29:467–469.
- Dyck PL. 1989. The inheritance of leaf rust resistance in wheat cultivars Kenyon and Buck Manantial. Can J Plant Sci. 69:1113–1117.
- Dyck PL. 1991. Genetics of adult plant leaf rust resistance in ‘Chinese Spring’ and ‘Sturdy’ wheats. Crop Sci. 31:309–311.
- Dyck PL. 1993a. Inheritance of leaf rust and stem rust resistance in ‘Roblin’ wheat. Genome. 36:289–293.
- Dyck PL. 1993b. The inheritance of leaf rust resistance in the wheat cultivar Pasqua. Can J Plant Sci. 73:903–906.
- Dyck PL. 1994a. Genetics of leaf rust resistance in 13 accessions of the Watkins wheat collection. Euphytica. 80:151–155.
- Dyck PL. 1994b. The transfer of leaf rust resistance from Triticum turgidum ssp. dicoccoides to hexaploid wheat. Can J Plant Sci. 74:671–673.
- Dyck PL, Bartos P. 1994. Attempted transfer of leaf rust resistance from Triticum monococcum and durum wheat to hexaploid wheat. Can J Plant Sci. 74:733–736.
- Dyck PL, Friebe B. 1993. Evaluation of leaf rust resistance from wheat chromosomal translocation lines. Crop Sci. 33:687–690.
- Dyck PL, Jedel PE. 1989. Genetics of resistance to leaf rust in two accessions of common wheat. Can J Plant Sci. 69:531–534.
- Dyck PL, Johnson R. 1983. Temperature sensitivity of genes for resistance in wheat to Puccinia recondita. Can J Plant Pathol. 5:229–234.
- Dyck PL, Kerber ER. 1981. Aneuploid analysis of a gene for leaf rust resistance derived from the common wheat cultivar Terenzio. Can J Genet Cytol. 23:405–409.
- Dyck PL, Kerber ER. 1985. Resistance of the race specific type. In: Roelfs AP, Bushnell WR, editors. The Cereal Rusts. Orlando: Academic Press; p. 469–500.
- Dyck PL, Kerber ER, Aung T. 1994. An interchromosomal reciprocal translocation in wheat involving leaf rust resistance gene Lr34. Genome. 37:556–559.
- Dyck PL, Kerber ER, Lukow OM. 1987. Chromosome location and linkage of a new gene (Lr33) for reaction to Puccinia recondita in common wheat. Genome. 29:463–466.
- Dyck PL, Lukow OM. 1988. The genetic analysis of two interspecific sources of leaf rust resistance and their effect on the quality of common wheat. Can J Plant Sci. 68:633–639.
- Dyck PL, Samborski DJ. 1968. Host-parasite interactions involving two genes for leaf rust resistance in wheat. In: Finlay KW, Shepherd KW, editors. Proceedings of the 3rd international wheat genetics symposium. Canberra, Australia 3: Australian Academy of Science; p. 245–250.
- Dyck PL, Samborski DJ. 1970. The genetics of two alleles for leaf rust resistance at the Lr14 locus in wheat. Can J Genet Cytol. 12:689–694.
- Dyck PL, Samborski DJ. 1974. Inheritance of virulence in Puccinia recondita on alleles at the Lr2 locus for resistance in wheat. Can J Genet Cytol. 16:323–332.
- Dyck PL, Samborski DJ. 1979. Adult plant leaf rust resistance in PI 250413 an introduction of common wheat. Can J Plant Sci. 59:329–332.
- Dyck PL, Samborski DJ. 1982. The inheritance of resistance to Puccinia recondita in a group of common wheat cultivars. Can J Genet Cytol. 24:273–283.
- Dyck PL, Samborski DJ, Anderson RG. 1966. Inheritance of adult-plant leaf rust resistance derived from the common wheat varieties Exchange and Frontana. Can J Genet Cytol. 8:665–671.
- Dyck PL, Samborski DJ, Martens JW. 1985. Inheritance of resistance to leaf rust and stem rust in the wheat cultivar Glenlea. Can J Plant Pathol. 7:351–354.
- Dyck PL, Sykes EE. 1994. Genetics of leaf rust resistance in three spelt wheats. Can J Plant Sci. 74:231–233.
- Dyck PL, Sykes EE. 1995. The inheritance of stem rust and leaf rust resistance in some Ethiopian wheat collections. Euphytica. 81:291–297.
- Fellers J, Soltani B, Bruce M, Linning R, Cuomo C, Szabo L, Bakkeren G. 2013. Conserved loci of leaf and stem rust fungi of wheat share synteny interrupted by lineage-specific influx of repeat elements. BMC Genomics. 14:60.
- Fofana B, Banks TW, McCallum BD, Strelkov SE, Cloutier S. 2007. Temporal gene expression profiling of the wheat leaf rust pathosystem using cDNA microarray reveals differences in compatible and incompatible defense pathways. Int J Plant Genot. Article ID 17542, 13 pages doi:10.1155/2007/17542
- German SE, Kolmer JA. 1992. Effect of gene Lr34 in the enhancement of resistance to leaf rust of wheat. Theor Appl Genet. 84:97–105.
- Gold J, Harder D, Townley-Smith F, Aung T, Procunier J. 1999. Development of a molecular marker for rust resistance genes Sr39 and Lr35 in wheat breeding lines. Electron J Biotechnol. 2:35–40.
- Greaney FJ. 1936. Cereal rust losses in Western Canada. Sci Agric. 16:608–614.
- Haggag MEA, Dyck PL. 1973. The inheritance of leaf rust resistance in 4 common wheat varieties possessing genes at or near the Lr3 locus. Can J Genet Cytol. 15:127–134.
- Haggag MEA, Samborski DJ, Dyck PL. 1973. Genetics of pathogenicity in three races of leaf rust on four wheat cultivars. Can J Genet Cytol. 15:73–82.
- Hiebert C, Thomas J, McCallum BD. 2005. Locating the broad-spectrum wheat leaf rust resistance gene Lr52 (LrW) to chromosome 5B by a new cytogenetic method. Theor Appl Genet. 110:1453–1457.
- Hiebert CW, Fetch TG, Zegeye T, Thomas JB, Somers DJ, Humphreys DG, McCallum BD, Cloutier S, Singh D, Knott DR. 2011. Genetics and mapping of seedling resistance to Ug99 stem rust in Canadian wheat cultivars ‘Peace’ and ‘AC Cadillac’. Theor Appl Genet. 122:143–149.
- Hiebert CW, McCallum BD, Thomas JB. 2014. Lr70, a new gene for leaf rust resistance mapped in common wheat accession KU3198. Theor Appl Genet. 127:2005–2009.
- Hiebert CW, Thomas JB, McCallum BD. 2010a. Stacking pairs of disease resistance genes in wheat populations using telocentric chromosomes. Mol Breed. 26:681–692.
- Hiebert CW, Thomas JB, McCallum BD, Humphreys DG, DePauw RM, Hayden MJ, Mago R, Schnippenkoetter W, Spielmeyer W. 2010b. An introgression on wheat chromosome 4DL in RL6077 (Thatcher*6/PI 250413) confers adult plant resistance to stripe rust and leaf rust (Lr67). Theor Appl Genet. 121:1083–1091.
- Hiebert CW, Thomas JB, McCallum BD, Somers DJ. 2008. Genetic mapping of the wheat leaf rust resistance gene Lr60 (LrW2). Crop Sci. 48:1020–1026.
- Hiebert CW, Thomas JB, Somers DJ, McCallum BD, Fox SL. 2007. Microsatellite mapping of adult-plant leaf rust resistance gene Lr22a in wheat. Theor Appl Genet. 115:877–884.
- Hiebert CW, Zhang P, McCallum BD, Thomas JB, Hoxha S, McIntosh RA. 2015. Genetic analysis of Lr13 and Ne2. In: Proceedings of the 14th international cereal rusts and powdery mildews conference; 2015 July 6–8. Helsingor (Denmark).
- Howes NK, Kim WK, Rohringer R. 1982. Detergent-soluble polypeptides extracted from urediospores of four physiologic races of Puccinia graminis f.sp. tritici. Physiol Plant Pathol. 21:361–366.
- Hu G, Kamp A, Linning R, Naik S, Bakkeren G. 2007b. Complementation of Ustilago maydis MAPK mutants by a wheat leaf rust Puccinia triticina homolog: Potential for functional analyses of rust genes. Mol Plant-Microbe Interact. 20:637–647.
- Hu G, Linning R, McCallum BD, Banks T, Cloutier S, Butterfield Y, Liu J, Kirkpatrick R, Stott J, Yang G, et al. 2007a. Generation of a wheat leaf rust, Puccinia triticina, EST database from stage-specific cDNA libraries. Mol Plant Pathol. 8:451–467.
- Hughes GR, Hucl P. 1993. CDC Teal hard red spring wheat. Can J Plant Sci. 73:193–197.
- Humphreys DG, Townley-Smith TF, Czarnecki E, Lukow OM, McCallum B, Fetch T, Gilbert J, Menzies J, Brown D, Fox SL. 2013. Snowstar hard white spring wheat. Can J Plant Sci. 93:143–148.
- Innes RL, Kerber ER. 1994. Resistance to wheat leaf rust and stem rust in Triticum tauschii and inheritance in hexaploid wheat of resistance transferred from T. tauschii. Genome. 37:813–822.
- Jedel P, Perriro P, Dyck PL, Martens JW. 1988. Resistance to Puccinia recondita and Puccinia graminis in Triticum accessions from the National Research Council Germplasm Institute, Bari, Italy. In: Zwatz B, editor. Proceedings of the 7th European Mediterranean Cereal Rusts Conference; 1988 Sep 5–9. Vienna (Austria): Federal Institute of Plant Protection.
- Johnson T. 1956. Physiologic races of leaf rust of wheat in Canada 1931 to 1955. Can J Agri. Sci. 36:371–379.
- Johnson T. 1961a. Man-guided evolution in plant rusts: through his modification of the host plants of the cereal rusts, man is also modifying the rusts. Science. 133:357–362.
- Johnson T. 1961b. Rust research in Canada and related plant disease investigations. Publ No 1098, Contr No 51. Res Station, Winnipeg: Res Branch, Can Dept Agric; Ottawa, Canada 69 pp.
- Johnson T, Newton M. 1937. The effect of high temperatures on uredial development in cereal rusts. Can J Res. 15:425–432.
- Johnson T, Newton M. 1946. The occurrence of new strains of Puccinia triticina in Canada and their bearing on varietal reaction. Scient Agricul. 26:468–478.
- Kassa MT, You FM, McCallum B, Hiebert C, Cabral A, Panwar V, Menzies J, Pozniak C, Sharpe A, Fobert P, et al. 2014. Fine mapping of leaf rust resistance gene Lr16. Plant and Animal Genome XXII, January 11–15, 2014. San Diego (CA).
- Kerber ER. 1987. Resistance to leaf rust in hexaploid wheat: Lr32, a third gene derived from Triticum tauschii. Crop Sci. 27:204–206.
- Kerber ER. 1988. Telocentric mapping in wheat of the gene Lr32 for resistance to leaf rust. Crop Sci. 28:178–179.
- Kerber ER, Aung T. 1995. Confirmation of nonsuppressor mutation of stem rust resistance in ‘Canthatch’ common wheat. Crop Sci. 35:743–744.
- Kerber ER, Aung T. 1999. Leaf rust resistance gene Lr34 associated with nonsuppression of stem rust resistance in wheat cultivar Canthatch. Phytopathology. 89:518–521.
- Kerber ER, Dyck PL. 1990. Transfer to hexaploid wheat of linked genes for adult-plant leaf rust and seedling stem rust resistance from an amphiploid of Aegilops speltoides x Triticum monoccocum. Genome. 33:530–537.
- Kim WK, Howes NK, Rohringer R. 1982. Detergent-soluble polypeptides in germinated urediospores and differentiated urediosporelings of wheat stem rust. Can J Plant Pathol. 4:328–333.
- Knott DR, Dvorak J. 1976. Alien germplasm as a source of resistance to disease. Annu Rev Phytopathol. 14:211–235.
- Knott DR, Dvorak J. 1981. Agronomic and quality characteristics of wheat lines with leaf rust resistance derived from Triticum speltoides. Can J Genet Cytol. 23:475–480.
- Knox RE, Campbell HL, DePauw RM, Clarke JM, Gold JJ. 2000. Registration of P8810-B5B3A2A2 white-seeded spring wheat germplasm with Lr35 leaf and Sr39 stem rust resistance. Crop Sci. 40:1512–1513.
- Kolmer JA. 1989. Virulence and race dynamics of Puccinia recondita f.sp. tritici in Canada during 1956–1987. Phytopathology. 79:349–356.
- Kolmer JA. 1990. Selection of virulence phenotypes in a heterogeneous asexual population of Puccinia recondita f.sp. tritici. Phytopathology. 80:1377–1381.
- Kolmer JA. 1991a. Evolution of two distinct populations of Puccinia recondita f.sp. tritici in Canada. Phytopathology. 81:316–322.
- Kolmer JA. 1991b. Phenotypic diversity in two populations of Puccinia recondita f.sp. tritici in Canada during 1931–1987. Phytopathology. 81:311–315.
- Kolmer JA. 1992a. Diversity of virulence phenotypes and effect of host sampling between and within populations of Puccinia recondita f.sp. tritici in Canada. Plant Dis. 76:618–621.
- Kolmer JA. 1992b. Effect of sexual recombination in two populations of the wheat leaf rust fungus, Puccinia recondita. Can J Bot. 70:359–363.
- Kolmer JA. 1992c. Enhanced leaf rust resistance in wheat conditioned by resistance gene pairs with Lr13. Euphytica. 61:123–130.
- Kolmer JA. 1992d. Virulence heterozygosity and gametic phase disequilibria in two populations of Puccinia recondita (wheat leaf rust fungus). Heredity. 68:505–513.
- Kolmer JA. 1993. Selection in a heterogeneous population of Puccinia recondita f.sp. tritici. Phytopathology. 83:909–914.
- Kolmer JA. 1994. Genetics of leaf rust resistance in three western Canada spring wheats. Plant Dis. 78:600–602.
- Kolmer JA. 1995. Selection of Puccinia recondita f.sp. tritici virulence phenotypes in three multilines of Thatcher wheat lines near isogenic for leaf rust resistance genes. Can J Bot. 73:1081–1088.
- Kolmer JA. 1996. Genetics of resistance to wheat leaf rust. Annu Rev Phytopathol. 34:435–455.
- Kolmer JA. 1997. Virulence in Puccinia recondita f. sp. tritici isolates from Canada to genes for adult plant resistance to wheat leaf rust. Plant Dis. 81:267–271.
- Kolmer JA. 1999. Virulence dynamics, phenotypic diversity, and virulence complexity in two populations of Puccinia triticina in Canada from 1987 to 1997. Can J Bot. 77:333–338.
- Kolmer JA. 2001a. Molecular polymorphism and virulence phenotypes of the wheat leaf rust fungus Puccinia triticina in Canada. Can J Bot. 79:917–926.
- Kolmer JA. 2001b. Physiologic specialization of Puccinia triticina in Canada in 1998. Plant Dis. 85:155–158.
- Kolmer JA, Anderson JA, Flor JM. 2010. Chromosome location, linkage with simple sequence repeat markers, and leaf rust resistance conditioned by gene Lr63 in wheat. Crop Sci. 50:2392–2395.
- Kolmer JA, Dyck PL. 1994. Gene expression in the Triticum aestivum-Puccinia recondita f. sp. tritici gene-for-gene system. Phytopathology. 84:437–440.
- Kolmer JA, Dyck PL, Roelfs AP. 1991. An appraisal of stem and leaf rust resistance in North American hard red spring wheats and the probability of multiple mutations to virulence in populations of cereal rust fungi. Phytopathology. 81:237–239.
- Kolmer JA, Liu JQ. 2000. Virulence and molecular polymorphism in international collections of the wheat leaf rust fungus Puccinia triticina. Phytopathology. 90:427–436.
- Kolmer JA, Liu JQ. 2001. Simple inheritance of partial resistance to leaf rust in two wheat cultivars. Plant Pathol. 50:546–551.
- Kolmer JA, Liu JQ. 2002. Inheritance of leaf rust resistance in the wheat cultivars AC Majestic, AC Splendor, and AC Karma. Can J Plant Pathol. 24:327–331.
- Kolmer JA, Liu JQ, Sies M. 1995. Virulence and molecular polymorphism in Puccinia recondita f. sp. tritici in Canada. Phytopathology. 85:276–285.
- Kumar S, Wang Z, Banks TW, Jordan MC, McCallum BD, Cloutier S. 2014. Lr1-mediated leaf rust resistance pathways of transgenic wheat lines revealed by a gene expression study using the Affymetrix GeneChip® wheat genome array. Mol Breed. 34:127–141.
- Liu J, Kolmer JA. 1997a. Genetics of leaf rust resistance in Canadian spring wheats AC Domain and AC Taber. Plant Dis. 81:757–760.
- Liu JQ, Kolmer JA. 1997b. Inheritance of leaf rust resistance in wheat cultivars Grandin and CDC Teal. Plant Dis. 81:505–508.
- Liu JQ, Kolmer JA. 1998. Molecular and virulence diversity and linkage disequilibria in asexual and sexual populations of the wheat leaf rust fungus, Puccinia recondita. Genome. 41:832–840.
- Long DL, Kolmer JA. 1989. A North American system of nomenclature for Puccinia recondita f.sp tritici. Phytopathology. 79:525–529.
- Marais F, Marais A, McCallum B, Pretorius Z. 2009. Transfer of leaf rust and stripe rust resistance genes Lr62 and Yr42 from Aegilops neglecta Req. ex Bertol. to common wheat. Crop Sci. 49:871–879.
- Marais GF, McCallum B, Marais AS. 2006. Leaf rust and stripe rust resistance genes derived from Aegilops sharonensis. Euphytica. 149:373–380.
- Marais GF, McCallum B, Marais AS. 2008. Wheat leaf rust resistance gene Lr59 derived from Aegilops peregrina. Plant Breed. 127:340–345.
- Marais GF, Pretorius ZA, Wellings CR, McCallum B, Marais AS. 2005. Leaf rust and stripe rust resistance genes transferred to common wheat from Triticum dicoccoides. Euphytica. 143:115–123.
- Martens G, Lamari L, Grieger A, Gulden RH, McCallum B. 2014. Comparative yield, disease resistance and response to fungicide for forty-five historic Canadian wheat cultivars. Can J Plant Sci. 94:371–381.
- Martens JW, Dyck PL. 1989. Genetics of resistance to rust in cereals from a Canadian perspective. Can J Plant Pathol. 11:78–85.
- McCaig TN, DePauw RM, Clarke JM, McLeod JG, Fernandez MR, Knox RE. 1996. AC Barrie hard red spring wheat. Can J Plant Sci. 76:337–339.
- McCallum B, Mulock B, Somers D, Bakkeren G. 2004. Inheritance of avirulence in the wheat leaf rust fungus Puccinia triticina. In: Proceedings of the 11th international cereal rusts and powdery mildews conference, 1.46. Cereal Rusts and Powdery Mildews Bulletin. [cited Feb. 10, 2016]. Available from: www.crpmb.org/icrpmc11/abstracts.htm
- McCallum BD, DePauw RM. 2008. A review of wheat cultivars grown in the Canadian prairies. Can J Plant Sci. 88:649–677.
- McCallum BD, Hiebert CW, Huerta-Espino J, Cloutier S. 2012. Wheat leaf rust. In: Sharma I, editor. Disease resistance in wheat. Wallingford (UK): CABI Publishers; p. 33–62.
- McCallum BD, Humphreys DG, Somers DJ, Dakouri A, Cloutier S. 2011a. Allelic variation for the rust resistance gene Lr34/Yr18 in Canadian wheat cultivars. Euphytica. 183:261–274.
- McCallum BD, Seto-Goh P. 2002. Physiologic specialization of wheat leaf rust (Puccinia triticina) in Canada in 1999. Can J Plant Pathol. 24:205–210.
- McCallum BD, Seto-Goh P. 2003. Physiologic specialization of wheat leaf rust [Puccinia triticina] in Canada in 2000. Can J Plant Pathol. 25:91–97.
- McCallum BD, Seto-Goh P. 2004. Physiologic specialization of Puccinia triticina, the cause of wheat leaf rust, in Canada in 2001. Can J Plant Pathol. 26:109–120.
- McCallum BD, Seto-Goh P. 2005. Physiologic specialization of wheat leaf rust (Puccinia triticina) in Canada in 2002. Can J Plant Pathol. 27:90–99.
- McCallum BD, Seto-Goh P. 2006a. Physiologic specialization of Puccinia triticina, the causal agent of wheat leaf rust, in Canada in 2003. Can J Plant Pathol. 28:208–213.
- McCallum BD, Seto-Goh P. 2006b. Physiologic specialization of Puccinia triticina, the causal agent of wheat leaf, in Canada in 2004. Can J Plant Pathol. 28:566–576.
- McCallum BD, Seto-Goh P. 2008. Physiologic specialization of Puccinia triticina in Canada in 2005. Can J Plant Pathol. 30:124–132.
- McCallum BD, Seto-Goh P. 2009. Physiologic specialization of Puccinia triticina, the causal agent of wheat leaf rust, in Canada in 2006. Can J Plant Pathol. 31:80–87.
- McCallum BD, Seto-Goh P. 2010. The inheritance of leaf rust resistance in the wheat cultivars ‘Superb’, ‘McKenzie’ and ‘HY644ʹ. Can J Plant Pathol. 32:387–395.
- McCallum BD, Seto-Goh P, Xue A. 2010. Physiological specialization of Puccinia triticina in Canada in 2007. Can J Plant Pathol. 32:229–236.
- McCallum BD, Seto-Goh P, Xue A. 2011b. Physiologic specialization of Puccinia triticina, the causal agent of wheat leaf rust, in Canada in 2008. Can J Plant Pathol. 33:541–549.
- McCallum BD, Seto-Goh P, Xue A. 2011c. The detection of virulence to Lr21 in Canada during 2011. Can J Plant Sci. 92:605.
- McCallum BD, Seto-Goh P, Xue A. 2013. Physiologic specialization of Puccinia triticina, the causal agent of wheat leaf rust, in Canada in 2009. Can J Plant Pathol. 35:338–345.
- McCallum BD, Thomas J. 2011. Effectiveness of wheat leaf rust gene combinations derived from the cultivar Pasqua. In: Proceedings of the international plant and animal genome conference XIX; 2011 Jan 15–19. San Diego (CA).
- McCartney CA, Somers DJ, McCallum BD, Thomas J, Humphreys DG, Menzies JG, Brown PD. 2005. Microsatellite tagging of the leaf rust resistance gene Lr16 on wheat chromosome 2BS. Mol Breed. 15:329–337.
- McIntosh RA, Dyck PL. 1975. Cytogenetical studies in wheat. VII. Gene Lr23 for reaction to Puccinia recondita in Gabo and related cultivars. Aust J Biol Sci. 28:201–212.
- McIntosh RA, Wellings CR, Park RF. 1995. Wheat rusts: an atlas of resistance genes. Canberra, Australia: CSIRO Publishing; 200 p.
- Navabi A, Singh RP, Huerta-Espino J, Tewari JP. 2005a. Phenotypic association of adult-plant resistance to leaf and stripe rusts in wheat. Can J Plant Pathol. 27:396–403.
- Navabi A, Tewari JP, Singh RP, McCallum B, Laroche A, Briggs KG. 2005b. Inheritance and QTL analysis of durable resistance to stripe and leaf rusts in Australian wheat cultivar Cook. Genome. 48:97–107.
- Newton M, Johnson T. 1941. Environmental reaction of physiologic races of Puccinia triticina and their distribution in Canada. Can J Res. 19c:121–133.
- Newton M, Johnson T. 1943. Adult plant resistance in wheat to physiologic races of Puccinia triticina Erikss. Can J Res. 21c:10–17.
- Panwar V, McCallum B, Bakkeren G. 2013a. Endogenous silencing of Puccinia triticina pathogenicity genes through in planta-expressed sequences leads to suppression of rust diseases on wheat. Plant J. 73:521–532.
- Panwar V, McCallum B, Bakkeren G. 2013b. Host-induced gene silencing of wheat leaf rust fungus Puccinia triticina pathogenicity genes mediated by the Barley stripe mosaic virus. Plant Mol Biol. 81:595–608.
- Peturson B. 1958. Wheat rust epidemics in Western Canada in 1953, 1954, and 1955. Can J Plant Sci. 38:16–28.
- Peturson B, Forsyth FR, Lyon CB. 1958. Chemical control of cereal rusts. II. Control of leaf rust of wheat with experimental chemicals under field conditions. Phytopathology. 48:655–657.
- Peturson B, Newton M. 1939. The effect of leaf rust on the yield and quality of Thatcher and Renown wheat in 1938. Can J Res. 17c:380–387.
- Peturson B, Newton M, Whiteside AGO. 1945. The effect of leaf rust on the yield and quality of wheat. Can J Res. 23c:105–114.
- Peturson B, Newton M, Whiteside AGO. 1948. Further studies on the effect of leaf rust on the yield, grade, and quality of wheat. Can J Res. 26c:65–70.
- Procunier JD, Townley-Smith TF, Fox S, Prashar S, Gray M, Kim WK, Czarnecki E, Dyck PL. 1995. PCR-based RAPD-DGGE markers linked to leaf rust resistance genes Lr29 and Lr25 in wheat (Triticum aestivum L.). J Genet Breed. 49:87–91.
- Rampitsch C, Beimcik E, McCallum B, Fan T, Gunel A, Bakkeren G. 2013. Comparative proteomics of monoclonal-antibody enriched haustoria from three races of Puccinia triticina. Asilomar CA: 27th Fungal Genetics Seminar.
- Rampitsch C, Bykova NV, McCallum BD, Beimcik E, Ens W. 2006. Analysis of the wheat and Puccinia triticina (leaf rust) proteomes during a susceptible host-pathogen interaction. Proteomics. 6:1897–1907.
- Rampitsch C, Günel A, Beimcik E, Mauthe WM. 2015. The proteome of monoclonal antibody-purified haustoria from Puccinia triticina Race-1. Proteomics. 15:1307–1315.
- Rohringer R, Samborski DJ, Person CO. 1961. Ribonuclease activity in rusted wheat leaves. Can J Bot. 39:775–784.
- Rosa SB, McCallum BD, Brûlé-Babel A, Seto-Goh P. 2014. Double artificial inoculation of Puccinia triticina for the study of wheat leaf rust resistance. Can J Plant Pathol. 36:83–88.
- Rowland GG, Kerber ER. 1974. Telocentric mapping hexaploid wheat of genes for leaf rust resistance and other characters derived from Aegilops squarrosa. Can J Genet Cytol. 16:137–144.
- Samborski DJ. 1963. A mutation in Puccinia recondita Rob. ex. Desm. f. sp. tritici to virulence on transfer, Chinese spring x Aegilops umbellulata Zhuk. Can J Bot. 41:475–479.
- Samborski DJ. 1985. Wheat leaf rust. In: Roelfs AP, Bushnell WR, editors. The cereal rusts. New York, USA: Academic Press; p. 39–59.
- Samborski DJ, Dyck PL. 1968. Inheritance of virulence in wheat leaf rust on the standard differential wheat varieties. Can J Genet Cytol. 10:24–32.
- Samborski DJ, Dyck PL. 1976. Inheritance of virulence in Puccinia recondita on six backcross lines of wheat with single genes for resistance to leaf rust. Can J Bot. 54:1666–1671.
- Samborski DJ, Dyck PL. 1982. Enhancement of resistance to Puccinia recondita by interactions of resistance genes in wheat. Can J Plant Pathol. 4:152–156.
- Samborski DJ, Forsyth FR. 1960. Inhibition of rust development on detached wheat leaves by metabolites, antimetabolites, and enzyme poisons. Can J Bot. 38:467–476.
- Samborski DJ, Forsyth FR, Person C. 1958. Metabolic changes in detached wheat leaves floated on benzimidazole and the effect of these changes on rust reaction. Can J Bot. 36:591–601.
- Samborski DJ, Ostapyk W. 1959. Expression of leaf rust resistance in Selkirk and Exchange wheats at different stages of plant development. Can J Bot. 37:1153–1155.
- Samborski DJ, Peturson B. 1960. Effect of leaf rust on the yield of resistant wheats. Can J Plant Sci. 40:620–622.
- Samborski DJ, Rohringer R, Person C. 1961. Effect of rust-inhibiting compounds on the metabolism of wheat leaves. Can J Bot. 39:1019–1027.
- Shang HS, Dyck PL, Samborski DJ. 1986. Inheritance of resistance to Puccinia recondita in a group of resistant accessions of common wheat. Can J Plant Pathol. 8:123–131.
- Sharma D, Knott DR. 1966. The transfer of leaf-rust resistance from Agropyron to Triticum by irradiation. Can J Genet Cytol. 8:137–143.
- Singh A, Pandey M, Knox R, Ammar K, Clarke J, Clarke F, Singh R, Pozniak C, DePauw R, McCallum B, et al. 2013. Identification and mapping of leaf, stem and stripe rust resistance quantitative trait loci and their interactions in durum wheat. Mol Breed. 31:405–418.
- Song X, Rampitsch C, Soltani B, Mauthe W, Linning R, Banks T, McCallum B, Bakkeren G. 2011. Proteome analysis of wheat leaf rust fungus, Puccinia triticina, infection structures enriched for haustoria. Proteomics. 11:944–963.
- Thomas J, Nilmalgoda S, Hiebert C, McCallum B, Humphreys G, DePauw R. 2010. Genetic markers and leaf rust resistance of the wheat gene Lr32. Crop Sci. 50:2310–2317.
- Townley-Smith TF, Czarnecki EM, Campbell AB, Dyck PL, Samborski DJ. 1993a. AC Minto hard red spring wheat. Can J Plant Sci. 73:1091–1094.
- Townley-Smith TF, Czarnecki EM, Campbell AB, Dyck PL, Samborski DJ. 1993b. Pasqua hard red spring wheat. Can J Plant Sci. 73:1095–1098.
- Wang X, Bakkeren G, McCallum B. 2010a. Virulence and molecular polymorphisms of the wheat leaf rust fungus Puccinia triticina in Canada from 1997 to 2007. Botany. 88:575–589.
- Wang X, Leclerc C, Bakkeren G, McCallum BD. 2006. Development of simple sequence repeat markers for the genetic analysis of leaf rust (Puccinia triticina) in wheat. Can J Plant Pathol. 28:368.
- Wang X, McCallum B. 2009. Fusion body formation, germ tube anastomosis, and nuclear migration during the germination of urediniospores of the wheat leaf rust fungus, Puccinia triticina. Phytopathology. 99:1355–1364.
- Wang X, McCallum BD, Fetch T, Bakkeren G, Marais GF, Saville BJ. 2013. Comparative microscopic and molecular analysis of Thatcher near-isogenic lines with wheat leaf rust resistance genes Lr2a, Lr3, LrB or Lr9 upon challenge with different Puccinia triticina races. Plant Pathol. 62:698–707.
- Wang X, Mulock B, Guus B, McCallum B. 2010b. Development of EST-derived simple sequence repeat markers for wheat leaf rust fungus, Puccinia triticina Eriks. Can J Plant Pathol. 32:98–107.
- Xu J, Linning R, Fellers J, Dickinson M, Zhu W, Antonov I, Joly DL, Donaldson ME, Eilam T, Anikster Y, et al. 2011. Gene discovery in EST sequences from the wheat leaf rust fungus Puccinia triticina sexual spores, asexual spores and haustoria, compared to other rust and corn smut fungi. BMC Genomics. 12:161.
- Zeng J, Cao W, Fedak G, Sun S, McCallum B, Fetch T, Xue A, Zhou Y. 2013. Molecular cytological characterization of two novel durum –Thinopyrum intermedium partial amphiploids with resistance to leaf rust, stem rust and Fusarium head blight. Hereditas. 150:10–16.