Abstract
Arabidopsis has been the model dicot plant system to study a variety of processes including immunity. One type of immunity, termed systemic acquired resistance (SAR), is deployed upon detection of avirulent pathogens by a plant. SAR deployment is dependent on the small molecule salicylic acid (SA) and confers immunity against a broad range of biotrophic pathogens. In this process, SA is perceived by NPR1, a key transcriptional co-activator. Recruitment of SA by NPR1 leads to changes in the conformation of NPR1, which frees a C-terminal transactivation domain from the auto-inhibitory effect of the N-terminal BTB/POZ domain. During this unfolding process, NPR1 is itself recruited by TGA2 and together they form an enhanceosome capable of activating downstream genes, including the SAR marker gene PR1. NPR1 is conserved in sequence and function in both dicot and monocot species. As such, it is portable from one plant species to another. In Arabidopsis, NPR1 is part of a multigene family containing six members, labelled NPR1 to NPR6, which show no redundancy with respect to NPR1 function in immunity. Arabidopsis NPR1 and NPR2 share 63% amino acid sequence identity and yet NPR2 is not a functional NPR1 with respect to immune functions. When looking across plant species, the per cent amino acid identity between Arabidopsis NPR1 and potential NPR1 orthologues tends to be below the 63% shared between NPR1 and NPR2. In this paper, we will show that relying on sequence identity alone to identify NPR1 orthologues can be misleading.
Résumé
Arabidopsis est la plante dicotylédone modèle qui a permis d’étudier une variété de processus, y compris l’immunité. Un type d’immunité, la résistance systémique acquise (RSA), entre en action lorsqu’une plante détecte des agents pathogènes avirulents. L’entrée en action de la RSA dépend de la petite molécule de l’acide salicylique (AS) et confère une immunité contre une vaste gamme d’agents pathogènes biotrophes. Durant ce processus, l’AS est perçu par NPR1, un coactivateur transcriptionnel essentiel. Le recrutement de l’AS par ce coactivateur provoque des changements dans la conformation de ce dernier, ce qui libère un domaine de transactivation de la région C-terminale de l’effet auto-inhibiteur du domaine de la région N-terminale BTB/POZ. Au cours de ce processus, NPR1 est lui-même recruté par le facteur de transcription TGA2 et, ensemble, ils forment un enhanceosome capable d’activer des gènes en aval, y compris le gène PR1, marqueur de la RSA. NPR1 est conservé en séquence et fonctionne tant chez les espèces dicotylédones que monocotylédones. En tant que tel, il est transférable d’une espèce de plantes à une autre. Chez Arabidopsis, NPR1 fait partie d’une famille multigénique de six membres, marqués NPR1 à NPR6, qui n’affichent aucune redondance quant aux fonctions qu’assure NPR1 sur le plan de l’immunité. Chez Arabidopsis, NPR1 et NPR2 partagent une identité de séquence d’acides aminés de 63 % et, malgré cela, NPR2 n’est pas un coactivateur NPR1 fonctionnel en ce qui a trait aux fonctions immunitaires. Si l’on considère l’ensemble des espèces de plantes, le taux d’identité des acides aminés chez Arabidopsis, entre NPR1 et les possibles orthologues de NPR1, tend à se situer sous les 63 % partagés par NPR1 et NPR2. Dans cet article, nous montrerons que le fait de se fier uniquement à l’identité de séquence pour identifier les orthologues de NPR1 risque d’être trompeur.
Introduction
Crop protection is at the heart of our sustainable food, feed and fibre production. With a steadily growing human population, projected to reach 9 billion by 2050, food security is a global and vital challenge that must be addressed. Every year, crop yield is lost due to weed competition, insect herbivory and diseases caused by pathogens. Here in the developed world, we are privileged and are accustomed to having high crop yield and high crop quality in modern agriculture. These yield and quality traits are only possible through the combined use of improved cultivars, fertilizers and agrochemicals. In conventional agriculture, these chemicals include herbicides, insecticides, fungicides and antibiotics. There are other parts of the world which are not so fortunate and, according to the FAO publication The State of Food Insecurity in the World (2012), 870 million people worldwide are chronically undernourished, and most of them (850 million) live in developing countries. Agricultural growth is the key to reducing hunger and malnutrition. High crop quality and high crop yield will continue to be required to sustain our growing world population.
Fig. 1 Phylogenetic tree of Arabidopsis and soybean NPR1-like proteins. The tree is divided into three clades, NPR1/NPR2, NPR3/NPR4 and NPR5/NPR6, based on the Arabidopsis NPR1-like proteins. NPR1 and the true soybean NPR1 orthologues appear inside white oval shapes. See text for more details on the figure.
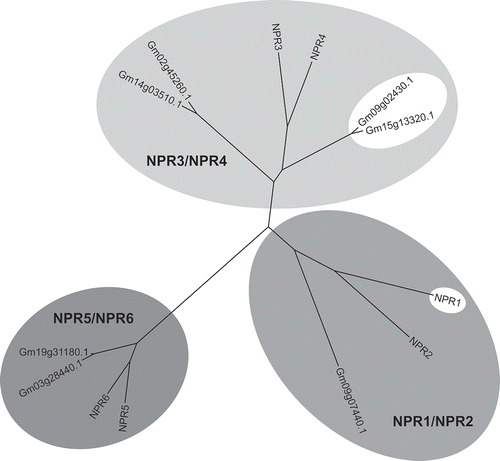
To add to the challenges faced by agriculture, regulatory bodies and consumers are demanding stricter regulatory safety margins, environmentally safer agrochemicals and practices, and low-cost goods, while at the same time the spectre of weed, pest and pathogen resistance to agrochemicals is mounting. As such, to ensure global food security, it is imperative to adopt a holistic approach to agriculture by not excluding or limiting the number of management tools at our disposal. These tools could include biocontrol agents and chemicals that stimulate plant immunity. The development of chemicals that stimulate plant immunity has been of great interest to us. We have approached this paradigm by studying a certain type of plant immunity termed systemic acquired resistance.
Systemic acquired resistance
Systemic acquired resistance (SAR) is a broad-spectrum and ‘whole-plant’ resistance response that is analogous to the innate immune system found in animals (Vlot et al. 2009). The term ‘broad-spectrum’ indicates that once induced, SAR will give rise to immunity against viral, bacterial and fungal pathogens. The term ‘systemic’ or ‘whole-plant’ relates to the fact that once the immune response is triggered in any part of the plant, immunity will be deployed throughout the plant. SAR is the most agronomically relevant type of plant immunity, and its deployment requires the build-up or at least the presence of endogenous salicylic acid (SA) (Vlot et al. 2009). In some plants, such as the model plant Arabidopsis, SA concentrations rise from a basal level to a high level during the deployment of SAR (Vlot et al. 2009). In others, such as rice, the basal level of SA is already high and does not rise significantly during SAR deployment (Vlot et al. 2009). Nevertheless, despite the absence of an increase in SA concentrations in rice, SA is required for SAR deployment. SAR is naturally triggered by perception, in one part of a plant, of an avirulent pathogen, which is detected by the plant. SAR can also be artificially triggered by systemically treating a plant with SA or other chemical inducers, such as 2,6-dichloroisonicotinic acid (INA) (Vernooij et al. 1995) and benzo(1,2,3)thiadiazole-7-carbothioic acid S-methyl ester (BTH) (Lawton et al. 1996). A hallmark of SAR deployment is the induction of the pathogenesis-related1 (PR1) gene by both avirulent pathogens and SAR-inducing chemicals. PR1 serves as the SAR marker-gene (Ward et al. 1991).
NPR1: the salicylic acid-binding transcription co-activator
The NPR1 protein is the central regulator of plant immunity, orchestrating SA-dependent resistance against biotrophic pathogens and necrotrophs with biotrophic phases (Cao et al. 1997; Ryals et al. 1997). NPR1 was first identified and is best characterized in the model system Arabidopsis, but it is found in all monocot and dicot plant species, including all economically important crops. We have recently identified and characterized NPR1 as the salicylic acid receptor in plants. Binding of SA to NPR1 requires the transition metal copper (Wu et al. 2012). Both the NPR1 protein and SA were known to be key components of plant immunity to pathogens. However, it took one and a half decades to link the two as the receptor-ligand pair. The discovery was very difficult to make since it required methods commonly used in the pharmaceutical industry but unknown in the plant biology field. In addition, we discovered that NPR1 is also the receptor for BTH (Wu et al. 2012), a synthetic compound that like SA stimulates a plant’s own immune response (Lawton et al. 1996). BTH is the active ingredient in Actigard®, a commercial product sold in North America. It was developed by a Syngenta legacy company without knowledge of its receptor or target protein in the plant. In Canada, because of human health concerns, BTH can only be used on tomato and tobacco. Interestingly, INA does not appear to be a ligand of NPR1. At least, we can conclude that if INA binds to NPR1, it would do so at a site different from the SA-binding site, since INA cannot displace radioactive SA. This could indicate that INA activates SAR through a mode of action that is different from that of SA.
Mechanistic description of SA perception by NPR1 and PR1 activation
Mechanistically, after perception of SA by NPR1, the NPR1 protein will ‘open up’ and release the C-terminal transactivation domain from the auto-inhibition by the N-terminal BTB/POZ domain (Rochon et al. 2006; Wu et al. 2012). NPR1 does not contain a DNA-binding domain and requires interaction with a DNA-binding protein for its recruitment to a promoter. As such, NPR1 is not a transcription factor, but a transcription co-activator. Recruitment to the SAR marker gene PR1 is effected by the TGA2-clade of transcription factors and occurs in vivo only after perception by SA (Subramaniam et al. 2001; Zhang et al. 2003). In vitro, NPR1 can interact with TGA2 without SA (Boyle et al. 2009). This observation may be due to the polydispersity of NPR1 protein preparations, in which some of the NPR1 molecules could be in an ‘open’ state. NPR1 contains two protein-protein interaction domains of which we are aware. These are the BTB/POZ domain in the N-terminus (Aravind & Koonin 1999) and the ankyrin repeats located in the central portion of the protein (Cao et al. 1997; Ryals et al. 1997). The ankyrin repeats are the domain responsible for the stable formation of the TGA/NPR1 complex. However, the BTB/POZ domain also contacts the TGA factors, and specifically in the case of TGA2, the domain interacts with the N-terminus of TGA2 (Boyle et al. 2009). TGA2 is recruited to PR1 both pre- and post-SA treatment and at its core, TGA2 is a transcriptional repressor. The repression domain is located in the N-terminus of TGA2. The function of the TGA2 repression domain is negated by the binding of the BTB/POZ domain of NPR1. Indeed, when one deletes the repression domain from TGA2, the NPR1 BTB/POZ becomes obsolete with respect to PR1 induction and can be deleted from the protein without consequences (Boyle et al. 2009).
Defining NPR1 orthologues in crops and the pitfall of percentage sequence identity
NPR1 is found in all crops of commercial significance, whether, like the model plant Arabidopsis, they are dicots, such as soybean (Sandhu et al. 2009; Pandey et al. 2011), or monocots, such as rice (Yuan et al. 2007). Functionally, NPR1 are conserved from species to species. For example, Arabidopsis NPR1 will function when transformed in rice (Chern et al. 2001). The reverse is also true, where rice (Yuan et al. 2007) or soybean (Sandhu et al. 2009) NPR1 will function in Arabidopsis and complement the npr1 mutation. However, the NPR1 amino acid sequence is not identical in all plants. There is a high sequence diversity among the members of the NPR1 superfamily. Within a plant species, NPR1 is part of a multigene family. For example, in the model system Arabidopsis, there are six members in the family, NPR1 to NPR6 (Liu et al. 2005). Two other Arabidopsis NPR1-like proteins, NPR3 and NPR4, have been shown to play a role in SA-dependent immunity (Fu et al. 2012). However, these proteins serve to degrade NPR1 and are therefore negative regulators of immunity. Given the universality of NPR1 and the fact that it has paralogues within a given species, it has become important to identify which NPR1-like gene encodes the real NPR1 protein. This will allow for a faster and more efficient translation of the knowledge garnered from Arabidopsis. Furthermore, the sequence diversity amongst the members of the NPR1 superfamily will lead to a greater understanding of what makes an NPR1, from a secondary-structure perspective.
A typical approach to identify an NPR1 orthologue, or any orthologous gene for that matter, is to perform a BLAST search and select the NPR1-like sequence that is the closest in identity to the Arabidopsis NPR1. A more sophisticated approach is to compare the sequence identity of the potential orthologue by performing a pair-wise comparison of the full-length sequences using an alignment software such as Clustal (Larkin et al. 2007; www.clustal.org). Clustal can then be used to create a more visually appealing rendering of the data by producing a ‘phylogenetic’ tree, if more than two sequences are used in a multiple alignment experiment. The data in have been produced by extracting all the NPR1-like sequences found in Arabidopsis and soybean using a protein-protein BLAST search with default parameters (blast.ncbi.nlm.nih.gov). The amino acid sequences from the matches were then extracted and subjected to a multiple alignment using ClustalX version 2.1 (Larkin et al. 2007). The per cent identity resulting from the pair-wise comparisons, performed by Clustal, is reported in .
Table 1. Soybean orthologues of the Arabidopsis NPR identified by per cent identity and complementation.
The top section of reports on the pair-wise comparison between the six NPR1 family members found in Arabidopsis. One can see that NPR1 is most similar to NPR2, with 63% amino acid sequence identity. NPR3 is most similar to NPR4, with 74% identity. NPR5 is most similar to NPR6, with 85% identity. Despite the high per cent sequence identity between NPR1 and NPR2, NPR2 is not a functional NPR1, since loss-of-function in a single gene (NPR1) is sufficient to abolish PR1 gene activation and SAR deployment (Cao et al. 1997; Ryals et al. 1997).
The bottom section of reports on the pair-wise comparison between Arabidopsis NPR1 to NPR6 and the seven NPR1-like sequences found in soybean. We chose soybean as a comparative organism because its genome is sequenced and available publicly (www.plantgdb.org). The soybean NPR1-like sequence with the highest sequence identity to Arabidopsis NPR1 is Gm09g07440.1, with 52% identity. One could therefore conclude that Gm09g07440.1 is the likely soybean orthologue of NPR1. However, 52% is below the per cent identity found between Arabidopsis NPR1 and NPR2. Furthermore, Arabidopsis NPR2 is also 51% identical to this gene. Therefore, is Gm09g07440.1 an NPR1 or an NPR2 orthologue? Continuing the analysis reveals that Gm09g02430.1 and Gm15g13320.1 are the closest members to Arabidopsis NPR3 and NPR4, with per cent identity between 61 and 63%. Again, which is the NPR3 or NPR4 orthologue? And so on for the orthologues of NPR5 and NPR6. Interestingly, two different research groups have demonstrated using a combination of genetic complementation tests, in the Arabidopsis npr1-1 mutant background (Sandhu et al. 2009), and virus-induced gene silencing (VIGS) in soybean (Pandey et al. 2011), that Gm09g02430.1 and Gm15g13320.1 are indeed the orthologues of Arabidopsis NPR1 despite being more closely related, in terms of sequence identity, to NPR3 and NPR4. Gm09g02430.1 and Gm15g13320.1 are only 38% identical to Arabidopsis NPR1, yet they are the true NPR1 orthologues.
We next performed a ‘phylogenetic’ analysis of the Arabidopsis and soybean NPR1-like proteins, which is depicted in Fig. 1. Once again, we used ClustalX version 2.1, which is not regarded as a specialist phylogenetic analysis program, but is the typical go-to software used by non-specialists, including ourselves. These trees are often used by biochemists to identify enzymes with related functions. The tree of Fig. 1 shows three distinct clades, which we identified as NPR1/NPR2, NPR3/NPR4 and NPR5/NPR6. Remarkably, Gm09g02430.1 and Gm15g13320.1, which are the true NPR1 orthologues based on complementation of the npr1-1 Arabidopsis mutant, are in the same clade as NPR3 and NPR4.
Conclusion
It may not be possible to distinguish an NPR1 orthologue from another species simply by comparing their amino acid sequences with that of the Arabidopsis NPR1. A functional test is needed in which the NPR1-like genes will be transformed in one of the Arabidopsis npr1 mutant backgrounds for a complementation test. Alternatively, a loss-of-function mutant in the potential crop NPR1 gene could be used to identify potential NPR1 orthologues by testing the effect of the mutation on the activation of the crop PR1 gene or the deployment of the crop SA-dependent immunity. However, we do not believe that this can substitute for a complementation test, since a loss-of-function in an NPR1-like gene only indicates that this gene is involved in the activation of PR1 or in the deployment of SA-dependent immunity. It does not de facto indicate that the gene is an NPR1 orthologue. Finally, the simple analysis presented here indicates that much work is still needed to pinpoint the key amino acids that truly define an NPR1.
Acknowledgement
We thank the Natural Sciences and Engineering Research Council of Canada (Discovery and Discovery Accelerator Supplement Programs), the Ontario Genomics (Pre-commercialization Business Development Fund) and the Canada Foundation for Innovation for their financial support to CD. CD is a founding member of Brock University’s Advanced Biomanufacturing Center, which supports CD and XK.
References
- Aravind L, Koonin EV. 1999. Fold prediction and evolutionary analysis of the POZ domain: structural and evolutionary relationship with the potassium channel tetramerization domain. J Mol Biol. 285:1353–1361.
- Boyle P, Le SE, Rochon A, Shearer HL, Murmu J, Chu JY, Fobert PR, Despres C. 2009. The BTB/POZ domain of the Arabidopsis disease resistance protein NPR1 interacts with the repression domain of TGA2 to negate its function. Plant Cell. 21:3700–3713.
- Cao H, Glazebrook J, Clarke JD, Volko S, Dong X. 1997. The Arabidopsis NPR1 gene that controls systemic acquired resistance encodes a novel protein containing ankyrin repeats. Cell. 88:57–63.
- Chern MS, Fitzgerald HA, Yadav RC, Canlas PE, Dong X, Ronald PC. 2001. Evidence for a disease-resistance pathway in rice similar to the NPR1-mediated signaling pathway in Arabidopsis. Plant J. 27:101–113.
- Fu ZQ, Yan S, Saleh A, Wang W, Ruble J, Oka N, Mohan R, Spoel SH, Tada Y, Zheng N, Dong X. 2012. NPR3 and NPR4 are receptors for the immune signal salicylic acid in plants. Nature. 486:228–232.
- Larkin MA, Blackshields G, Brown NP, Chenna R, McGettigan PA, McWilliam H, Valentin F, Wallace IM, Wilm A, Lopez R, et al. 2007. Clustal W and Clustal X version 2.0. Bioinformatics. 23:2947–2948.
- Lawton KA, Friedrich L, Hunt M, Weymann K, Delaney T, Kessmann H, Staub T, Ryals J. 1996. Benzothiadiazole induces disease resistance in Arabidopsis by activation of the systemic acquired resistance signal transduction pathway. Plant J. 10:71–82.
- Liu G, Holub EB, Alonso JM, Ecker JR, Fobert PR. 2005. An Arabidopsis NPR1-like gene, NPR4, is required for disease resistance. Plant J. 41:304–318.
- Pandey AK, Yang C, Zhang C, Graham MA, Horstman HD, Lee Y, Zabotina OA, Hill JH, Pedley KF, Whitham SA. 2011. Functional analysis of the Asian soybean rust resistance pathway mediated by Rpp2. Mol Plant-Microbe Interact. 24:194–206.
- Rochon A, Boyle P, Wignes T, Fobert PR, Despres C. 2006. The coactivator function of Arabidopsis NPR1 requires the core of its BTB/POZ domain and the oxidation of C-terminal cysteines. Plant Cell. 18:3670–3685.
- Ryals J, Weymann K, Lawton K, Friedrich L, Ellis D, Steiner HY, Johnson J, Delaney TP, Jesse T, Vos P, Uknes S. 1997. The Arabidopsis NIM1 protein shows homology to the mammalian transcription factor inhibitor I kappa B. Plant Cell. 9:425–439.
- Sandhu D, Tasma IM, Frasch R, Bhattacharyya MK. 2009. Systemic acquired resistance in soybean is regulated by two proteins, orthologous to Arabidopsis NPR1. BMC Plant Biol. 9:105.
- Subramaniam R, Desveaux D, Spickler C, Michnick SW, Brisson N. 2001. Direct visualization of protein interactions in plant cells. Nat Biotechnol. 19:769–772.
- Vernooij B, Friedrich L, Ahl Goy P, Staub T, Kessmann H, Ryals J. 1995. 2,6-dichloroisonicotinic acid-induced resistance to pathogens without the accumulation of salicylic acid. Mol Plant-Microbe Interact. 8:228–234.
- Vlot AC, Dempsey DA, Klessig DF. 2009. Salicylic acid, a multifaceted hormone to combat disease. Annu Rev Phytopathol. 47:177–206.
- Ward ER, Uknes SJ, Williams SC, Dincher SS, Wiederhold DL, Alexander DC, Hl-Goy P, Metraux JP, Ryals JA. 1991. Coordinate gene activity in response to agents that induce systemic acquired resistance. Plant Cell. 3:1085–1094.
- Wu Y, Zhang D, Chu JY, Boyle P, Wang Y, Brindle ID, De Luca V, Despres C. 2012. The Arabidopsis NPR1 protein is a receptor for the plant defense hormone salicylic acid. Cell Rep. 1:639–647.
- Yuan Y, Zhong S, Li Q, Zhu Z, Lou Y, Wang L, Wang J, Wang M, Li Q, Yang D, He Z. 2007. Functional analysis of rice NPR1-like genes reveals that OsNPR1/NH1 is the rice orthologue conferring disease resistance with enhanced herbivore susceptibility. Plant Biotechnol J. 5:313–324.
- Zhang Y, Tessaro MJ, Lassner M, Li X. 2003. Knockout analysis of Arabidopsis transcription factors TGA2, TGA5, and TGA6 reveals their redundant and essential roles in systemic acquired resistance. Plant Cell. 15:2647–2653.