Abstract
Net blotch, caused by Pyrenophora teres, is an economically important disease of barley. The pathogen has two morphologically similar but genetically distinct forms: P. teres f. teres (Ptt) and P. teres f. maculata (Ptm), which cause net form net blotch (NFNB) and spot form net blotch (SFNB), respectively. The virulence of a collection of 39 Ptt and 27 Ptm isolates collected from western Canada was evaluated by inoculating these isolates onto sets of barley differential hosts. One week following inoculation, the second and third leaves of each plant were rated for disease severity on scales of 1–10 (for Ptt) or 1–9 (for Ptm). Plants rated <5 and ≥5 were scored as resistant and susceptible to Ptt, respectively, while plants rated 1–3 and >3 were scored as resistant and susceptible to Ptm. The experiment was repeated. Cluster analysis revealed 16 and 13 distinct pathotype groups, respectively, among the 39 and 27 representative Ptt and Ptm isolates. The barley differentials CI 5791 and CI 9820 were resistant to all isolates of Ptt except one, whilst the differential CI 9214 was resistant to all isolates of Ptm except two. Therefore, the differential lines CI 5791 and CI 9820, for Ptt, and CI 9214, for Ptm, can still be considered as potentially useful sources of resistance for Canadian barley breeding programmes.
Résumé
La rayure réticulée, causée par Pyrenophora teres, est une maladie de l’orge importante sur le plan économique. L’agent pathogène possède deux formes morphologiquement semblables, mais génétiquement distinctes, P. teres f. teres (Ptt) et P. teres f. maculata (Ptm), qui causent la tache en réseau de la rayure réticulée (TRRR) et la forme maculée de la rayure réticulée (FMRR), respectivement. La virulence d’une collection de 39 isolats de Ptt et de 27 isolats de Ptm, collectés dans l’Ouest canadien, a été évaluée en inoculant ces derniers à des gammes d’hôtes différentiels d’orge. Une semaine après l’inoculation, les deuxièmes et troisièmes feuilles de chaque plant ont été cotées en fonction de la gravité de la maladie sur une échelle de 1 à 10 pour Ptt ou de 1 à 9 pour Ptm. Les plants dont la cote variait de < 5 et ≥ 5 étaient considérés comme résistants et réceptifs à Ptt, respectivement, tandis que les plants cotés de 1 à 3 et > 3 étaient considérés comme résistants et réceptifs à Ptm, respectivement. L’expérience a été répétée. L’analyse typologique a révélé 16 et 13 groupes distincts de pathotypes, respectivement, parmi les 39 et les 27 isolats représentatifs de Ptt et de Ptm. Les différentiels d’orge CI 5791 et CI 9820 étaient résistants à tous les isolats de Ptt, sauf un, tandis que le différentiel CI 9214 était résistant à tous les isolats de Ptm, sauf deux. Par conséquent, les lignées différentielles CI 5791 et CI 9820, pour Ptt, et CI 9214, pour Ptm, peuvent encore être considérées comme utiles en tant que sources de résistance quant aux programmes canadiens de sélection de l’orge.
Introduction
Net blotch, caused by the heterothallic ascomycete Pyrenophora teres Drechs. (anamorph: Drechslera teres [Sacc.] Shoem.) (McDonald Citation1963; Smedegard-Petersen Citation1978), is an economically important disease of barley (Hordeum vulgare L.) across western Canada (Tekauz Citation1990; Xi et al. Citation1999). Pyrenophora teres has two morphologically similar but genetically distinct forms: P. teres f. teres (Ptt) and P. teres f. maculata (Ptm), which cause the net (NFNB) and spot (SFNB) forms of net blotch, respectively (Rau et al. Citation2007; Liu et al. Citation2011; Ellwood et al. Citation2012). Both Ptt and Ptm are stubble-borne pathogens and likely go through regular cycles of sexual reproduction in western Canada (Akhavan et al. Citation2015), with sexual hybridization occurring on the stubble (Piening Citation1961, Citation1968; Duczek et al. Citation1999) followed by multiple cycles of asexual reproduction during the growing season (van den Berg & Rossnagel Citation1991; Peever & Milgroom Citation1994). Therefore, both forms of the pathogen have mixed reproduction and an outcrossing mating system, falling into the category of pathogens with a relatively high risk of being able to rapidly adapt to major host resistance genes and fungicides with single modes of action (McDonald & Linde Citation2002). It was demonstrated that Ptt and Ptm are capable of hybridization under laboratory conditions (Campbell et al. Citation1999; Campbell & Crous Citation2003; Jalli Citation2011). However, many studies have shown that these two forms are classified in two divergent groups (Rau et al. Citation2003; Leisova-Svobodova et al. Citation2014). Yield losses of 10–40% for NFNB, and up to 44% for SFNB, are typical in severe outbreaks, but P. teres has the potential to cause a total loss in susceptible cultivars under conditions that favour disease development (Steffenson et al. Citation1991; Mathre Citation1997; Jayasena et al. Citation2007; Murray & Brennan Citation2010). Losses can be reduced by crop rotation, fungicide application and the deployment of resistant cultivars, which are the three main components of integrated net blotch management in barley (Tekauz Citation1990; Turkington et al. Citation2011; McLean et al. Citation2012). However, the application of fungicides increases input costs, and long crop rotation is not always practical on many farms in western Canada, resulting in an increased interest in the use of resistant cultivars for net blotch management. Currently, very few cultivars exhibiting NFNB resistance are available, while most cultivars have intermediate to moderately resistant reactions to SFNP (Alberta Agriculture and Rural Development Citation2015). Therefore, incorporating resistance to the net blotch pathogen in barley cultivars remains a priority for barley breeding programmes in western Canada.
Tekauz (Citation1990) and Liu and Friesen (Citation2010) reported that the occurrence of SFNB in Canada and in the Northern Great Plains of the USA has been increasing. Neupane et al. (Citation2015) suggested that this increase likely results from changes in the virulence of local pathogen populations. Khan (Citation1982), Bockelman et al. (Citation1983), Karki and Sharp (Citation1986), Wu et al. (Citation2003) and Grewal et al. (Citation2008) also reported the occurrence of various degrees of pathogenic diversity among Ptm isolates. In contrast, McLean et al. (Citation2010) reported low pathogenic variation among isolates in a local Ptm population collected from Victoria, Australia. More recently, however, McLean et al. (Citation2014) identified 33 pathotypes among 60 isolates collected across Australia using 16 barley differential genotypes. The evolution of virulent pathotypes of P. teres is a primary challenge for the deployment of durable net blotch resistant barley cultivars (Tekauz Citation1990; McLean et al. Citation2014), and an updated knowledge of the pathogenic diversity and virulence spectrum of local pathogen populations is essential for successful incorporation of resistance genes into commercial cultivars. The pathogenic diversity present in a local pathogen population represents a challenge to barley breeders, since a particular resistance source may be effective only toward a limited number of pathotypes (McLean et al. Citation2012). For example, it was shown that barley lines with the Rpt6 resistance gene resulted in a resistant reaction against Ptm isolates collected from Finland, but not to Ptm isolates from Australia or the Czech Republic (Manninen et al. Citation2006). Moreover, since P. teres has the potential to undergo sexual recombination in western Canada (Akhavan et al. Citation2015), there is a likelihood that new virulent pathotypes may emerge and overcome the currently used sources of resistance (Jayasena et al. Citation2004). Khan (Citation1982) reported that resistance against Ptm in the barley cultivar ‘Beecher’ was overcome by the development of new virulent pathotypes in Western Australia. The virulence of Ptt and Ptm isolates varies on differential host lines, and has been examined in many studies. Different pathotypes, also called virulence phenotypes, have been observed in the populations of both forms, both globally and within particular areas (Liu et al. Citation2011).
In the last comprehensive assessment of pathogenic diversity among Ptt and Ptm populations from western Canada, Tekauz (Citation1990) classified 182 Ptt isolates into 10 main pathotype groups (A–J) with one to nine subgroups in each group (45 distinct virulence profiles in total). Tekauz (Citation1990) also classified 42 Ptm isolates into seven main pathotype groups (P–V) with one to five subgroups in each group (20 distinct virulence profiles in total). Liu et al. (Citation2012) suggested that western Canada has a higher percentage of virulent Ptt isolates (Tekauz Citation1990) than North Dakota by comparing the virulence of isolates on three differentials, CI 5791, ‘Heartland’ and CI 9214, shared between the two studies.
Canadian barley is produced mainly in the provinces of Alberta, Saskatchewan and Manitoba (Statistics Canada Citation2015), and both NFNB and SFNB are among the most important foliar diseases of barley in this region (Turkington et al. Citation2011). However, there has been only one comprehensive study that assessed pathogenic diversity of Ptt and Ptm populations collected from western Canada, which was conducted using a population collected in 1985 (Tekauz Citation1990). In the current study, the virulence of a set of isolates collected from 2009–2012 was evaluated on the same differential sets previously developed and used to characterize the Ptt and Ptm isolates collected in 1985 (Tekauz Citation1990), enabling a direct comparison of pathogenic diversity with the earlier collection. The objective of this study was to evaluate the virulence and pathotype composition of western Canadian populations of Ptt and Ptm, and to determine if there have been any changes over the past three decades.
Materials and methods
Fungal isolation and preparation of inoculum
Previously, a cluster analysis using simple sequence repeat (SSR) DNA markers was performed using the methods and primers described by Liu et al. (Citation2012) on a collection of 126 Ptt and 91 Ptm isolates collected from across western Canada (Alberta, Saskatchewan and Manitoba) (Akhavan et al. Citation2014a, Citation2014b). In the current study, 36 Ptt and 21 Ptm isolates were selected as representatives of these different clades to assess pathogenic variation. Three Ptt and six Ptm isolates were added from another collection made in 2012. The origins of the Ptt isolates included the provinces of Alberta (17 isolates), Saskatchewan (12) and Manitoba (10). The Ptm isolates originated from Alberta (8 isolates), Saskatchewan (10) and Manitoba (9). Reference isolates of Ptt (WRS102) and Ptm (WRS857) also were included for comparison (Supplementary Table 1).
To obtain single-spore isolates from barley leaves, leaf sections (about 10 mm × 5 mm) were surface-sterilized in 50% ethanol for 15 s and in 2% sodium hypochlorite for 30 s, flushed with sterile water and placed on moistened filter paper in 9 cm-diameter plastic Petri dishes (Tekauz Citation1990). To induce fungal sporulation on the leaves, the dishes were incubated at 20°C ± 0.5°C with a 12 h photoperiod under a mixture of common fluorescent lamp light and a black light blue fluorescent lamp emitting near ultraviolet light at 368 nm. After 3–5 days, single conidia of P. teres were transferred onto 10% V-8 juice agar (V-8 juice, 100 mL; CaC03 3 g; Difco agar 20 g; distilled water 900 mL) supplemented with 50 mg L−1 kanamycin, and incubated as above to produce new colonies (Tekauz Citation1990). Isolates were stored as mycelial plugs (0.5 cm in diameter) in liquid nitrogen or at −80°C in an ultra-low temperature freezer. Identification of the isolates as P. teres was based on the morphological characteristics of the conidiophores and conidia, and species-specific PCR analysis. The form of P. teres (Ptt or Ptm) was confirmed by form-specific PCR as described by Akhavan et al. (Citation2015).
To produce conidia for use as inoculum, the protocol described by Lamari & Bernier (Citation1989) as modified by Aboukhaddour et al. (Citation2013) for the closely related fungus Pyrenophora tritici-repentis (Died.) Drechs (anamorph: Drechslera tritici-repentis (Died.) Shoem.) was used. Briefly, isolates were removed from storage and the mycelial plugs were placed on fresh 10% V-8 juice agar in 9 cm-diameter Petri dishes, with one plug (isolate) per dish. The Petri dishes were then stored in darkness at room temperature for 5–7 days, until the colonies reached 4–5 cm in diameter. Approximately 10 mL of sterile distilled water was then added to each Petri dish, and the fungal colonies were flattened with a sterile glass tube. The water was decanted and the Petri dishes were incubated overnight under fluorescent light at room temperature, followed by 24 h incubation in darkness at 15°C to induce sporulation. Spore suspensions were prepared by adding 4–5 mL of sterile distilled water to the sporulating colonies and gently removing the conidia from the conidiophores with a sterile inoculation loop or an art brush.
Virulence assessments
The virulence of the Ptt isolates was evaluated on seedlings of nine barley differential genotypes, including the two-rowed ‘Norbert’, TR 473, CI 5791 and CI 9820, and the six-rowed ‘Heartland’, ‘OAC 21’, ‘Steptoe’, BT 201 and CI 9214. Similarly, the virulence of the Ptm isolates was evaluated on seedlings of 11 barley differential genotypes, including the two-rowed ‘Betzes’, ‘Herta’, ‘Norbert’, TR 473, CI 5791 and CI 9820, and the six-rowed ‘Bonanza’, ‘Heartland’, ‘OAC 21ʹ, ‘Steptoe’ and CI 9214. These differential sets were developed by Tekauz (Citation1990) to evaluate collections of Ptt and Ptm isolates made in the 1980s. For each isolate-host genotype combination, two replicates (each consisting of three barley plants in 10 cm-diameter plastic pots) were included. The entire test was conducted twice on two different occasions. If the results were inconsistent between the two runs of the experiment for a particular isolate or host genotype, the inoculations were repeated as necessary to clarify the host reaction.
Plants were grown in growth cabinets maintained at 20°C ± 2°C with 18 h light and 6 h dark for 2 weeks, and were inoculated at the two to three leaf stage. Fungal spore concentrations were measured with a Fuchs Rosenthal Counting Chamber (Hausser Scientific, Blue Bell, PA), and manually adjusted to 1 × 104 conidia per mL for Ptt, and 2 × 103 conidia per mL for Ptm. The spore suspensions were applied at a rate of 10 mL per pot (Tekauz Citation1990) with a pressurized atomizer connected to an air-line. The inoculated plants were covered with plastic bags for 24 h and maintained at almost 100% relative humidity, following which the bags were removed and the plants returned to the previous growth conditions. One week following inoculation, the second and third leaves of each seedling were rated on the 1–10 and 1–9 disease severity scales developed by Tekauz (Citation1985) for Ptt and Ptm, respectively.
Analysis of data
For each barley differential-isolate interaction, the average disease severity was calculated by averaging the arithmetic mean of reactions in the two experiments (two leaves per plant, three plants per replicate, and two replicates per experiment). Barley differentials rated as <5 and ≥5, for Ptt, and 1–3 and >3, for Ptm, were scored as resistant and susceptible, respectively. Data were transformed into a 0–1 matrix with 0 as resistant and 1 as susceptible. The similarity matrix was then used to perform cluster analysis by the unweighted pair-group method using the arithmetic means (UPGMA) procedure and simple similarity coefficient. The qualitative data program of NTSYSpc v. 2.2 (Exeter Software, New York, NY) was used to construct the similarity matrix. The SAHN program of NTSYS-pc was employed to perform the UPGMA analysis, and dendrograms were produced using the Tree Plot program of the same software package (McLean et al. Citation2014). A SSR dendrogram also was produced using the same procedure and programs.
For ease of comparison between the results from the current study and those of Tekauz (Citation1990), the isolates were grouped based on the relative number of resistant (r) and susceptible (s) reactions on the nine Ptt and 11 Ptm differentials as described by Tekauz (Citation1990). For the Ptt population, these groups ranged from 9r/0s, designated as group A, to 1r/8s, designated as group J, and each isolate was further designated with a number (1–11) based on the combination of specific susceptible or resistance reactions on each of the nine barley genotypes. For the Ptm population, groups ranged from 11r/0s, designated as group L, to 0r/11s, designated as group W, and each isolate was further designated with a number (1–6) according to the combination of specific reactions of each of the 11 barley genotypes (Tekauz Citation1990).
Results
Virulence of Pyrenophora teres f. teres
The reaction of each of the nine differential genotypes to each of the 39 Ptt isolates, along with their pathotype designations and relative virulence, is summarized in . Based on the reaction of the nine barley lines, the UPGMA cluster analysis using simple similarity coefficient revealed 16 pathotype groups among the 39 Ptt isolates, with a mean of 0.41 pathotypes per isolate (). Two pathotype groups (C04 and E09), which comprised 43% of the isolates, were found to be predominant in the collection, while nine isolates (22.5%) had virulence profiles distinct from all other isolates. The average disease severity for the 39 isolates on the nine differential genotypes was 4.5. Variation in virulence ranged from that of an isolate (MB14) which was virulent on eight of the nine differentials, with an average disease severity rating of 6.5, to an avirulent isolate (MB11) with an average rating of 2.8. Among the host genotypes tested, BT 201 and ‘OAC 21ʹ were the most susceptible to Ptt, with average disease ratings of almost 8.0 and 7.5, respectively. With the exception of the avirulent isolate MB11, all Ptt isolates examined in this study were virulent on BT 201. None of the barley differential hosts were resistant to all 39 isolates, although CI 5791 and CI 9820 were resistant to all isolates except MB14, with average ratings of 2.6 and 2.7, respectively. MB14 from Manitoba, which was the most virulent isolate, could overcome the resistance and cause mild symptoms of disease on the most resistant differential CI 5791. The percentage of Ptt isolates virulent on each differential genotype is shown in . Seven pathotypes (B2, C6, D9, D10, E10, E11 and I3) were identified for the first time in the Ptt population from western Canada, while pathotypes G, H and J, previously reported by Tekauz (Citation1990), were not identified among the Ptt isolates studied ().
Table 1. Pathogenic variation of 39 Canadian isolates of Pyrenophora teres f. teres (net form net blotch of barley) on nine barley differentials based on a 1–10 scale. Regular and bold font numbers denote resistant (<5) and susceptible (≥5) phenotypes, respectively.
Fig. 1 Pathogenic similarity of 39 Pyrenophora teres f. teres (net form net blotch of barley) isolates recovered from barley crops in western Canada; two pathotype groups, highlighted in boxes, were found to be predominant. The dendrogram was produced using the unweighted pair-group method using the arithmetic means (UPGMA) procedure and simple similarity coefficient with NTSYSpc ver. 2.2.
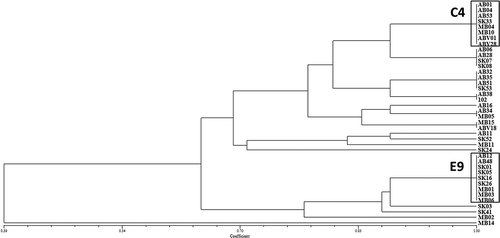
Fig. 2 a, Percentage of western Canadian Pyrenophora teres f. teres isolates virulent on nine barley differential genotypes; BT 201 (97.4%, 38 isolates), ‘OAC 21ʹ (84.6%, 33 isolates), ‘Norbert’ (35.9%, 14 isolates), TR 473 (33.3%, 13 isolates), ‘Steptoe’ (30.8%, 12 isolates), CI 9214 (28.2%, 11 isolates), ‘Heartland’ (12.8%, 5 isolates), CI 5791 (2.6%, 1 isolate), CI 9820 (2.6%, 1 isolate). b, Percentage of western Canadian Pyrenophora teres f. maculata isolates virulent on 11 barley differential genotypes; ‘Betzes’ (92.6%, 25 isolates), ‘Herta’ (88.9%, 24 isolates), TR 473 (85.2%, 23 isolates), ‘Norbert’ (85.2%, 23 isolates), ‘Bonanza’ (85.2%, 23 isolates), ‘Steptoe’ (81.5%, 22 isolates), CI 9820 (77.8%, 21 isolates), CI 5791 (70.4%, 19 isolates), ‘OAC 21ʹ (55.6%, 15 isolates), ‘Heartland’ (51.9%, 14 isolates), CI 9214 (7.4%, 2 isolates).
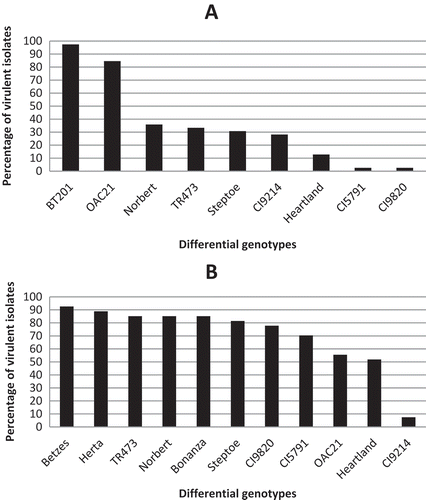
Fig. 3 a, Comparison of the main pathotype groups (A–J) found among Pyrenophora teres f. teres (net form net blotch of barley) populations from western Canada in 1985 (Tekauz Citation1990) versus 2009–2011 (current study). b, Comparison of the main pathotype groups (L–W) found among the Pyrenophora teres f. maculata (spot form net blotch of barley) populations from western Canada in 1985 (Tekauz Citation1990) versus 2009–2011 (current study).
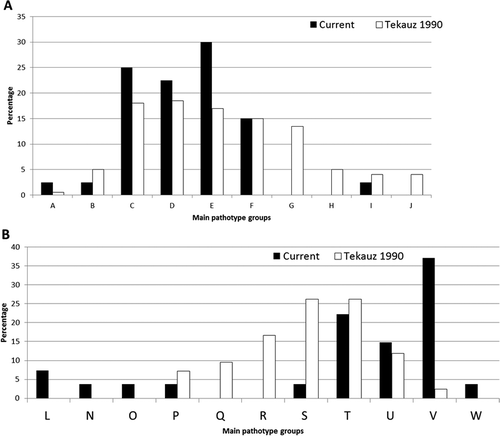
Virulence of Pyrenophora teres f. maculata
In the case of Ptm, the reaction of the 11 differential host genotypes to each of the 27 isolates, along with their pathotype designations and relative virulence, is summarized in . The UPGMA cluster analysis revealed 13 pathotype groups (), with two of these groups (V1 and T1) comprising 52% of the isolates and therefore predominant among the Ptm isolates examined; nine isolates (33%) had distinct virulence profiles. Variation in virulence ranged from a Manitoba isolate (MBV25) that was virulent on 10 of the 11 differentials (with an average disease rating of 6.1) and a Saskatchewan isolate (SK60) that was virulent on all 11 differentials (and caused an average disease rating of 5.7) to an avirulent isolate from Alberta (AB57) with an average disease rating of 2.2. The percentage of virulent Ptm isolates on each differential genotype is shown in . While pathotypes Q and R, which were previously reported by Tekauz (Citation1990), were not identified in the current study, eight new Ptm pathotypes (L1, N1, O1, P3, T6, U3, U4 and W1) were found for the first time in western Canada (). Among the differential genotypes tested, ‘Herta’, ‘Betzes’, ‘Norbert’ and TR 473 were the most susceptible, while the differential CI 9214 was resistant to all but two isolates, SK60 from Saskatchewan and MB22 from Manitoba. For both Ptt and Ptm, no clear trends were observed with respect to the geographic distribution of pathotypes in Alberta, Saskatchewan and Manitoba.
Table 2. Pathogenic variation of 27 Canadian isolates of Pyrenophora teres f. maculata (spot form net blotch of barley) on 11 barley differentials based on a 1–9 scale. Regular and bold font numbers denote resistant (1–3) and susceptible (>3) phenotypes, respectively.
Fig. 4 Pathogenic similarity of Pyrenophora teres f. maculata (spot form net blotch of barley) isolates recovered from barley crops in western Canada; two pathotype groups, highlighted in boxes, were found to be predominant. The dendrogram was produced using the unweighted pair-group method using the arithmetic means (UPGMA) procedure and simple similarity coefficient with NTSYSpc ver. 2.2.
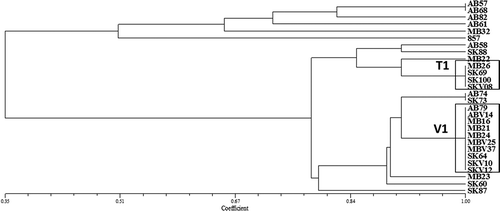
Comparative virulence and molecular polymorphism
Previously, a cluster analysis using simple sequence repeat (SSR) DNA markers was performed on a collection of 126 Ptt and 91 Ptm isolates from across western Canada (Alberta, Saskatchewan and Manitoba) (Akhavan et al. Citation2015). Among the isolates tested in the current study, 36 Ptt and 21 Ptm isolates were selected as representatives of the different clusters to assess variation in virulence. For these isolates, a UPGMA analysis was conducted using simple similarity coefficient on the combined Ptt and Ptm virulence datasets (). The isolates clustered in two distinct groups, with 35 Ptt and 6 Ptm isolates in the first group and 1 Ptt and 15 Ptm isolates in the second group. The results obtained through a similar analysis using SSR data (), however, indicated that the Ptt and Ptm isolates clustered into two divergent groups conforming to each form. Moreover, the dendrograms produced for these 36 Ptt and 21 Ptm isolates using the SSR and virulence datasets had a very low consensus index (CIc = 0.07).
Fig. 5 Pathogenic similarity of 36 P. teres f. teres (Ptt) and 21 P. teres f. maculata (Ptm) isolates collected from western Canada. Isolates clustered in two distinct groups with 35 Ptt and 6 Ptm isolates in the first group and 1 Ptt and 15 Ptm isolates in the second group. The dendrogram was produced using the unweighted pair-group method using the arithmetic means (UPGMA) procedure and simple similarity coefficient with NTSYSpc ver. 2.2.
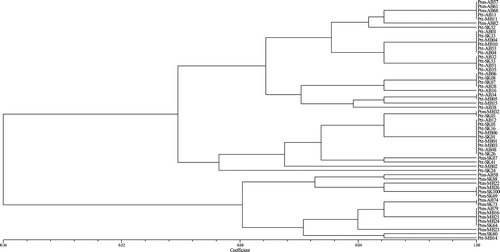
Fig. 6 Genetic similarity of 36 P. teres f. teres (Ptt) and 21 P. teres f. maculata (Ptm) isolates collected from western Canada. Cluster analysis revealed that all isolates clustered in two distinct divergent groups conforming to either Ptt or Ptm, with no intermediate cluster between the two forms. The dendrogram was produced using the unweighted pair-group method using the arithmetic means (UPGMA) procedure and simple similarity coefficient with NTSYSpc ver. 2.2.
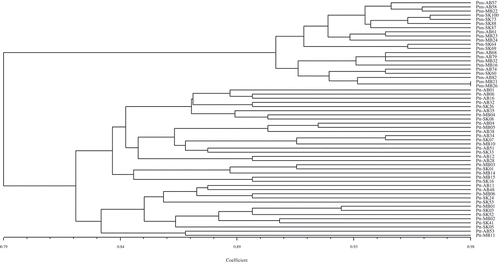
Discussion
Barley cultivars with resistance to Ptt and Ptm represent effective and practical tools for the management of net blotch disease in western Canada. van den Berg & Rossnagel (Citation1991) showed that even a moderately susceptible cultivar can provide adequate control of SFNB compared with a susceptible cultivar. However, the emergence of new pathotypes in local Ptt and Ptm populations is a great threat to the durability of the currently used sources of resistance. Up to date knowledge of the extent of variability in the virulence of pathogen populations is essential for successful deployment of any resistant cultivar. McDonald & Buchannon (Citation1962) first reported physiological specialization in P. teres in Canada. Steffenson & Webster (Citation1992) found 13 pathotypes among 91 Ptt isolates from California using 22 differential barley genotypes. Wu et al. (Citation2003) identified 15 pathotypes from a collection of 23 Ptt isolates collected from 12 different barley-growing regions of the world. In Australia, Gupta and Loughman (Citation2001) identified eight virulence groups among 74 Ptt isolates collected from different barley fields in Western Australia. Bouajila et al. (Citation2012) found 23 pathotypes among 85 isolates of Ptt collected in Tunisia. Cromey & Parks (Citation2003) characterized 11 pathotypes among 29 New Zealand isolates of Ptt using 31 barley differential genotypes. Liu et al. (Citation2012) showed significant differences in the virulence of isolates representing a population of Ptt collected from North Dakota, and also reported that the host genotypes CI 5791, ‘Algerian’ and ‘Heartland’ showed a high level of seedling resistance to all of the isolates tested. Working with Ptm populations collected from the Mediterranean region and the USA, Karki & Sharp (Citation1986) found that almost 10 of the 20 differential genotypes they used showed differential reactions. Gupta et al. (Citation2012) also reported a high pathogenic diversity in Ptm populations collected in Australia.
In the present study, 39 Ptt and 27 Ptm isolates were selected as representatives of Ptt and Ptm populations from across western Canada and assessed for virulence on sets of differential hosts, revealing relatively high levels of pathogenic diversity. In all, 16 Ptt and 13 Ptm pathotypes were identified. For Ptt, this level of pathogenic diversity (0.41 pathotype/isolate) was higher than that reported by Tekauz (Citation1990) in western Canada (0.24 pathotype/isolate), Steffenson & Webster (Citation1992) in California (0.14 pathotype/isolate), or by Cromey & Parks (Citation2003) in New Zealand (0.38 pathotype/isolate), but lower than that reported by Liu et al. (Citation2012) in North Dakota (0.65 pathotype/isolate), Wu et al. (Citation2003) in a global collection of Ptt (0.65 pathotype/isolate) and Jonsson et al. (Citation1997) in Sweden (0.48 pathotype/isolate). Douiyssi et al. (Citation1998) showed that each of 15 Ptt isolates from Morocco was unique in its virulence spectrum on 38 barley genotypes. For Ptm, the level of pathogenic diversity identified in the current study (0.48 pathotype/isolate) was equal to that reported by Tekauz (Citation1990) in western Canada, but slightly lower than reported by McLean et al. (Citation2014) for Australian isolates (0.55 pathotype/isolate). As noted by Liu et al. (Citation2012), the pathogenic diversity and the virulence spectrum reported in different studies are dependent mainly on the pathogen population structure, as well as the genotypes included in each differential set and their differential capacity. The use of distinct differential sets makes it challenging to compare the specific pathotype composition in different regions.
The development of resistant cultivars is an effective strategy for net blotch management, and is a high priority for all western Canadian barley-breeding programmes. However, a thorough knowledge of the variation in the local populations of a pathogen is required for the development of such resistant cultivars (Jalli Citation2011; Liu et al. Citation2012). It also was shown that some cultivars resistant to one form of net blotch are not resistant to the other form (Bockelman et al. Citation1983), which suggests that there are distinct host–pathogen interactions associated with each form of P. teres, and that resistance breeding efforts should focus on each form individually (Liu et al. Citation2011). This is currently the case in western Canada, where candidate cultivars are evaluated for each of the two forms independently (Prairie Recommending Committee for Oat and Barley Citation2015). Resistance against Ptt was reported in many barley genotypes and the inheritance and chromosomal location of the related genes were studied. Dominant and recessive major genes and quantitative trait loci (QTLs) on different barley chromosomes were shown to be involved in conferring resistance to various isolates of Ptt (Abu Qamar et al. Citation2008; Ho et al. Citation1996; Friesen et al. Citation2006; Liu et al. Citation2011, Citation2015; O’Boyle et al. Citation2011; Grewal et al. Citation2012; König et al. Citation2013). Recently, Liu et al. (Citation2015) showed that the sensitivity to a Ptt proteinaceous necrotrophic effector (NE) which mapped to barley chromosome 6H accounted for 31% of the disease variation, and suggested that the barley–Ptt pathosystem follows, at least partially, a necrotrophic effector-triggered susceptibility (NETS) model. For Ptm, McLean et al. (Citation2009, Citation2010) characterized 13 lines with combinations of major and minor genes controlling seedling, adult or all-stage resistance against SFNB. In these sources, SFNB resistance is related mostly to genes with major or minor effects, located on a single or multiple chromosomes (Williams et al. Citation1999, Citation2003; McLean et al. Citation2012). Wang et al. (Citation2015) used association mapping to investigate for quantitative trait loci (QTL) controlling SFNB resistance at both the seedling and adult plant growth stages, and found 29 significant QTL; 22 of the QTL influenced resistance at both plant growth stages, while 2 and 5 QTL controlled resistance only at the seedling or adult growth stages, respectively. Neupane et al. (Citation2015) assessed the resistance of more than 2000 barley accessions against four geographically diverse Ptm isolates and found only 15 resistant barley accessions against all isolates tested. In addition, Neupane et al. (Citation2015) reported isolate-specific susceptibility in several of the barley accessions they tested. Employing the same set of barley genotypes used by Neupane et al. (Citation2015), Tamang et al. (Citation2015) reported a total of 8–13 quantitative trait loci (QTL) associated with SFNB resistance against four different isolates from the USA, New Zealand, Australia and Denmark.
The Ptt isolates in this study caused average disease severities that ranged from 2.8 to 6.5 on the barley differentials, which was similar to the range of severities reported by Liu et al. (Citation2012) for a collection of Ptt isolates from North Dakota (2.6–6.2). Disease severities caused by the Ptm isolates ranged from 2.2 to 6.1. Among the genotypes included in the Ptt differential set, BT 201 and ‘OAC 21ʹ were the most susceptible, with average disease ratings of almost 8.0 and 7.5, respectively. In contrast, the genotypes CI 5791 and CI 9820 were resistant to all Ptt isolates except one, with average ratings of 2.6 and 2.7, respectively. Line CI 5791, with resistance mapped to chromosome 6 H (Friesen et al. Citation2014), also was found to be the most resistant among a set of barley differentials assessed against Ptt isolates from North Dakota (Liu et al. Citation2012).
Using the pathotyping procedure reported by Tekauz (Citation1990), two Ptt pathotype groups (C4 and E9) comprising 43% of the isolates were predominant in western Canada. Tekauz (Citation1990) previously found that pathotypes Cl and Gl were common in this region. The four most virulent pathotypes identified in this study included five isolates in total: three from Manitoba, one from Saskatchewan and one from central Alberta. Similarly, Tekauz (Citation1990) found that the Ptt isolates in the three most virulent pathotypes were primarily from Manitoba and Saskatchewan, with only one from Alberta. MB14, an isolate from Manitoba, was the most virulent and the only isolate of Ptt pathotype I identified in the current study. Comparison of the present results with those of Tekauz (Citation1990) suggests that the virulence profile of Ptt in western Canada has changed in the past three decades (). Pathotypes G, H and J, which were previously reported by Tekauz (Citation1990), were no longer identified, while seven new pathotypes (B2, C6, D9, D10, E10, E11 and I3) not reported by Tekauz (Citation1990) were found. Nevertheless, the sources of resistance in CI 5791 and CI 9820, which have been used extensively in western Canadian breeding programmes (Tekauz Citation1990), are still effective against all current pathotypes excluding pathotype I of Ptt.
For Ptm, two Ptm pathotype groups (V1 and T1) comprising 52% of the isolates were found to be predominant in western Canada. In contrast, Tekauz (Citation1990) previously reported that pathotypes T1 and S1 were the most common in this region. The two most virulent Ptm pathotypes identified in this study included 11 isolates in total, five from Manitoba, four from Saskatchewan and two from central Alberta. Tekauz (Citation1990) reported that the most virulent Ptm pathotypes in his study originated from Athabasca, Alberta, followed by isolates from all three provinces. As was the case for Ptt, it seems that the virulence profile of Ptm in western Canada also has changed over the past three decades (). While pathotypes Q and R (previously reported by Tekauz Citation1990) were not identified, eight new Ptm pathotypes (L1, N1, O1, P3, T6, U3, U4 and W1) were found for the first time in western Canada. The differential CI 9214, with the Rpt4 gene mapped to chromosome 7H (Williams et al. Citation2003), was resistant to all but two isolates, indicating that the resistance in this genotype, which has been employed for a long time in western Canadian breeding programmes (Tekauz Citation1990), is still for the most part effective against Ptm. Gupta et al. (Citation2012) and McLean et al. (Citation2010) previously documented differences in the virulence of isolates between the eastern and western states of Australia. In the current study, however, no clear geographic trends were observed with respect to the pathotype distribution of either Ptt or Ptm in western Canada.
The differential sets developed by Tekauz (Citation1990) for both Ptt and Ptm still seem effective for assessing variability in the virulence of western Canadian isolates of these pathogens. Afanasenko et al. (Citation2009) developed an international barley differential set (IBDS) for Ptt, and proposed it for global use to profile virulence patterns in Ptt. Tekauz et al. (Citation2011) assessed the utility of the IBDS in evaluating the virulence of nine Canadian Ptt isolates and found this differential set was effective in uncovering pathogenic variability in this collection. However, five barley genotypes had similar reaction profiles to the Canadian isolates, suggesting some redundancy. More recently, Liu et al. (Citation2012) also developed a set of 22 barley differential lines and identified 0.65 pathotype/isolate, which is higher than found in the current study (0.41 pathotype/isolate). Therefore, in future assessments of the virulence of western Canadian Ptt populations, it may be useful to add the genotypes with the highest differentiating capacity from the IBDS differential set (Afanasenko et al. Citation2009) or those of Liu et al. (Citation2012) to the differentials of Tekauz (Citation1990). McLean et al. (Citation2014) assessed the ability of 16 barley genotypes to uncover the extent of pathogenicity in an Australian Ptm population and characterized 33 pathotypes among 60 isolates, but also found that some of the differentials exhibited similar reactions. McLean et al. (Citation2009, Citation2012, Citation2014) also suggested the inclusion of five additional barley genotypes (CI 3576, CI 9776, CI 9819, CI 9831 and ‘Haruna Nijo’) in their differential set to develop a standard Ptm differential set for international use, as these genotypes could effectively differentiate pathotypes among non-Australian Ptm isolates. Thus, adding the genotypes with the highest differentiating capacity from the differential set proposed by McLean et al. (Citation2012) to that of Tekauz (Citation1990) also may result in better pathotype discrimination among western Canadian Ptm populations. Among the differentials used in this study, the resistance against Ptt has been characterized in CI5791 (Friesen et al. Citation2014), ‘Steptoe’ (Steffenson et al. Citation1996) and CI 9214 (Gupta et al. Citation2004), and against Ptm in CI 9214 (Williams et al. Citation2003). Differential sets that include genotypes that are well characterized with respect to their genetic background would lead to a more informative assessment of virulence diversity among the pathogen populations. This reinforces the need to study the genetic basis of resistance in all genotypes employed in a differential set, such as the one used in the current study or those suggested by other researchers (Liu et al. Citation2012; McLean et al. Citation2014).
The P. teres isolates examined in this study clustered into mixed groups based on UPGMA analysis of the combined Ptt and Ptm virulence datasets (), but into groups conforming to each form of the fungus based on the SSR data (). The dendrograms produced with the virulence and SSR datasets also had a very low consensus index. Collectively, these observations suggest that variability in the SSR sites is not likely an indication of variability in virulence, and that these two types of variation are independent. Therefore, the selection of a subset of isolates based on their SSR variability for the purposes of assessing variation in virulence may not be any more effective than a random selection of representative isolates.
The fairly high level of variability in the virulence of Ptt and Ptm populations from western Canada, combined with the fact that considerably greater diversity was found in Ptt relative to an earlier study (Tekauz Citation1990), suggests the need for the ongoing incorporation of novel sources of resistance into commercial barley cultivars in this region, and continued monitoring of pathogen populations. Resistance sources should be evaluated at both the adult plant and seedling stages (Liu et al. Citation2012). Moreover, the current findings confirm the conclusion of Tekauz (Citation1990) that the virulence spectrum of P. teres in western Canada is broad and changes rapidly. The observation that the differentials CI 5791 and CI 9820 were resistant to all isolates of Ptt except one, while the differential CI 9214 was resistant to all isolates of Ptm except two, suggests that the resistance in these genotypes is still (for the most part) effective. Nonetheless, the identification of a Ptt isolate virulent on CI 5791 and CI 9820, and two Ptm isolates virulent on CI 9214, indicates that producers should not rely exclusively on host resistance as the sole strategy for net blotch management, but rather use resistance together with other strategies such as crop rotation and the judicious application of fungicides.
Supplemental Material
Download MS Word (17.9 KB)Acknowledgements
The authors would like to thank Ms Colleen Kirkham, Mr Meconnen Beyene, Ms Noryne Rauhala, Ms Jackie Busaan (Agriculture and Agri-Food Canada) and Dr Krishan Kumar (Alberta Agriculture and Forestry) for assistance with the collection of isolates, and Ms Ileana S. Strelkov, Ms Nikki-Karyssa Scott, Ms Kelley Dunfield, Dr Reem Aboukhaddour and Dr Tiesen Cao (University of Alberta) for technical advice and insight.
Supplemental material
Supplemental data for this article can be accessed online here: http://dx.doi.org/10.1080/07060661.2016.1159617
Additional information
Funding
References
- Aboukhaddour R, Turkington TK, Strelkov SE. 2013. Race structure of Pyrenophora triciti-repentis (tan spot of wheat) in Alberta, Canada. Can J Plant Pathol. 35:256–268.
- Abu Qamar M, Liu ZH, Faris JD, Chao S, Edwards MC, Lai Z, Franckowiak JD, Friesen TL. 2008. A region of barley chromosome 6H harbors multiple major genes associated with net type net blotch resistance. Theor Appl Genet. 117:1261–1270.
- Afanasenko OS, Jalli M, Pinnschmidt HO, Filatova O, Platz GJ. 2009. Development of an international standard set of barley differential genotypes for Pyrenophora teres f. teres. Plant Pathol. 58:665–676.
- Akhavan A, Turkington TK, Kebede B, Tekauz A, Kutcher R, Kirkham C, Xi K, Kumar K, Tucker J, Strelkov SE. 2015. Prevalence of mating type idiomorphs in Pyrenophora teres f. teres and P. teres f. maculata populations from the Canadian prairies. Can J Plant Pathol. 37:52–60.
- Akhavan A, Turkington TK, Kebede B, Tekauz A, Xi K, Kutcher R, Tucker J, Kirkham C, Kumar K, Rennie D, Strelkov SE. 2014a. Genetic structure of Pyrenophora teres f. teres (net form net blotch of barley) populations from the Canadian prairies as revealed by simple sequence repeats analysis. Can J Plant Pathol. 36:125. (abstr.).
- Akhavan A, Turkington TK, Kebede B, Tekauz A, Xi K, Kutcher R, Tucker J, Kirkham C, Kumar K, Rennie D, Strelkov SE. 2014b. Genetic diversity of the spot form of the net blotch pathogen of barley (Pyrenophora teres f. maculata) on the Canadian prairies as revealed by simple sequence repeats analysis. Can J Plant Pathol. 36:125–126. (abstr.).
- Alberta Agriculture and Rural Development. 2015. Varieties of Cereal and Oilseed Crops for Alberta – 2015. Government of Alberta, Agriculture and Rural Development [Internet]. [cited 2015 Sept 11]. Available from: http://www1.agric.gov.ab.ca/$department/deptdocs.nsf/all/agdex4069
- Bockelman HE, Sharp EL, Bjarko ME. 1983. Isolates of Pyrenophora teres from Montana and the Mediterranean region that produce spot-type lesions on barley. Plant Dis. 67:696–697.
- Bouajila A, Zoghlami N, Al Ahmed M, Baum M, Ghorbel A, Nazari K. 2012. Pathogenicity spectra and screening for resistance in barley against Tunisian Pyrenophora teres f. teres. Plant Dis. 96:1569–1575.
- Campbell GF, Crous PW. 2003. Genetic stability of net × spot hybrid progeny of the barley pathogen Pyrenophora teres. Australas Plant Pathol. 32:283–287.
- Campbell GF, Crous PW, Lucas JA. 1999. Pyrenophora teres f. maculata, the cause of Pyrenophora leaf spot of barley in South Africa. Mycol Res. 103:257–267.
- Cromey MG, Parks RA. 2003. Pathogenic variation in Dreschlera teres in New Zealand. N Z Plant Prot. 56:251–256.
- Douiyssi A, Rasmusson DC, Roelfs AP. 1998. Responses of barley cultivars and lines to isolates of Pyrenophora teres. Plant Dis. 82:316–321.
- Duczek LJ, Sutherland KA, Reed SL, Bailey KL, Lafond GP. 1999. Survival of leaf spot pathogens on crop residues of wheat and barley in Saskatchewan. Can J Plant Pathol. 21:165–173.
- Ellwood SR, Syme RA, Moffat CS, Oliver RP. 2012. Evolution of three Pyrenophora cereal pathogens: recent divergence, speciation and evolution of non-coding DNA. Fungal Genet Biol. 49:825–829.
- Friesen TL, Faris JD, Lai Z, Steffenson BJ. 2006. Identification and chromosomal location of major genes for resistance to Pyrenophora teres in a doubled-haploid barley population. Genome. 49:855–859.
- Friesen TL, Koladia V, Brueggeman RS, Faris JD. 2014 June 3–6. A CI5791 × Tifang RI population shows major dominant net form net blotch resistance located on barley chromosomes 6H and 3H in CI5791 and Tifang, respectively. Paper presented at: 1st International Workshop on Barley Leaf Diseases, Salsomaggiore Terme, Italy.
- Grewal TS, Rossnagel BG, Scoles GJ. 2008. The utility of molecular markers for barley net blotch resistance across geographic regions. Crop Sci. 48:2321–2333.
- Grewal TS, Rossnagel BG, Scoles GJ. 2012. Mapping quantitative trait loci associated with spot blotch and net blotch resistance in a doubled-haploid barley population. Mol Breed. 30:267–279.
- Gupta S, Loughman R. 2001. Current virulence of Pyrenophora teres on barley in Western Australia. Plant Dis. 85:960–966.
- Gupta S, Loughman R, Antuono MD, Bradley J. 2012. Characterisation and diversity of Pyrenophora teres f. maculata isolates in Western Australia. Australas Plant Pathol. 41:31–40.
- Gupta S, Wielinga C, Li CD, Cakir M, Platz G, Loughman R, Lance R, Appels R. 2004. Gene distribution and SSR markers linked with net type net blotch resistance in barley. In: Spunar J, Janikova J, editors. Proceedings of the 9th International Barley Genetics Symp.; 2004 June 20–26; Brno, Czech Republic. Publisher: Agricultural Research Institute Kromeriz Ltd., Kromeriz, Czech Republic p. 668–673.
- Ho KM, Tekauz A, Choo TM, Martin RA. 1996. Genetic studies on net blotch resistance in a barley cross. Can J Plant Sci. 76:715–719.
- Jalli M. 2011. Sexual reproduction and soil tillage effects on virulence of Pyrenophora teres in Finland. Ann Appl Biol. 158:95–105.
- Jayasena KW, George E, Loughman R, Hardy G. 2004. First record of the teleomorph stage of Drechslera teres f. maculata in Australia. Australas Plant Pathol. 33:455–456.
- Jayasena KW, Van Burgel A, Tanaka K, Mejewski J, Loughman R. 2007. Yield reduction in barley in relation to spot-type net blotch. Australas Plant Pathol. 36:429–433.
- Jonsson R, Bryngelsson T, Gustafsson M. 1997. Virulence studies of Swedish net blotch isolates Drechslera teres and identification of resistant barley lines. Euphytica. 94:209–218.
- Karki CB, Sharp EL. 1986. Pathogenic variation in some isolates of Pyrenophora teres f. sp. maculata on barley. Plant Dis. 70:684–687.
- Khan TN. 1982. Occurrence and pathogenicity of Drechslera teres isolates causing spot-type symptoms on barley in Western Australia. Plant Dis. 66:423–425.
- König J, Perovic D, Kopahnke D, Ordon F. 2013. Development of an efficient method for assessing resistance to the net type of net blotch Pyrenophora teres f. teres in winter barley and mapping of quantitative trait loci for resistance. Mol Breed. 32:641–650.
- Lamari L, Bernier CC. 1989. Evaluation of wheat lines and cultivars for reaction to tan spot (Pyrenophora tritici-repentis) based on lesion types. Can J Plant Pathol. 11:49–56.
- Leisova-Svobodova L, Minarikova V, Matusinsky P, Hudcovicova M, Ondreickova K, Gubis J. 2014. Genetic structure of Pyrenophora teres net and spot populations as revealed by microsatellite analysis. Fungal Biol. 118:180–192.
- Liu Z, Ellwood SR, Oliver RP, Friesen TL. 2011. Pyrenophora teres: profile of an increasingly damaging barley pathogen. Mol Plant Pathol. 121:1–19.
- Liu Z, Friesen TL. 2010. Identification of Pyrenophora teres f. maculata, causal agent of spot type net blotch in North Dakota. Plant Dis. 94:480.
- Liu Z, Holmes DJ, Faris JD, Chao S, Brueggeman RS, Edwards MC, Friesen TL. 2015. Necrotrophic effector‐triggered susceptibility (NETS) underlies the barley–Pyrenophora teres f. teres interaction specific to chromosome 6H. Mol Plant Pathol. 16:188–200.
- Liu ZH, Zhong S, Stasko AK, Edwards MC, Friesen TL. 2012. Virulence profile and genetic structure of a North Dakota population of Pyrenophora teres f. teres, the causal agent of net form net blotch of barley. Phytopathology. 102:539–546.
- Manninen OM, Jalli M, Kalendar R, Schulman A, Afanasenko O, Robinson J. 2006. Mapping of major spot-type and net-type net-blotch resistance genes in the Ethiopian barley line CI 9819. Genome. 49:1564–1571.
- Mathre DE. 1997. Compendium of Barley diseases. 2nd ed. St. Paul (MN): APS Press.
- McDonald B, Linde C. 2002. Pathogen population genetics, evolutionary potential, and durable resistance. Annu Rev Phytopathol. 40:349–379.
- McDonald WC. 1963. Heterothallism in Pyrenophora teres. Phytopathology. 53:771–773.
- McDonald WC, Buchannon KW. 1962. The inheritance of variability in Pyrenophora teres. Barley Newslett. 6:40.
- McLean MS, Howlett BJ, Hollaway GJ. 2009. Epidemiology and control of spot form of net blotch (Pyrenophora teres f. maculata) of barley: a review. Crop Pasture Sci. 60:303–315.
- McLean MS, Howlett BJ, Turkington TK, Platz GJ, Hollaway GJ. 2012. Spot form of net blotch resistance in a diverse set of barley lines in Australia and Canada. Plant Dis. 96:569–576.
- McLean MS, Keiper FJ, Hollaway GJ. 2010. Genetic and pathogenic diversity in Pyrenophora teres f. maculata in barley crops of Victoria, Australia. Australas Plant Pathol. 39:319–325.
- McLean MS, Martin A, Gupta S, Sutherland MW, Hollaway GJ, Platz GJ. 2014. Validation of a new spot form of net blotch differential set and evidence for hybridisation between the spot and net forms of net blotch in Australia. Australas Plant Pathol. 43:223–233.
- Murray GM, Brennan JP. 2010. Estimating disease losses to the Australian barley industry. Australas Plant Pathol. 39:85–96.
- Neupane A, Tamang P, Brueggeman RS, Friesen TL. 2015. Evaluation of a barley core collection for spot form net blotch reaction reveals distinct genotype-specific pathogen virulence and host susceptibility. Phytopathology. 105:509–517.
- O’Boyle P, Brooks W, Steffenson B, Stromberg E, Griffey C. 2011. Genetic characterization of barley net blotch resistance genes. Plant Dis. 95:19–23.
- Peever TL, Milgroom MG. 1994. Genetic structure of Pyrenophora teres populations determined with random amplified polymorphic DNA markers. Can J Bot. 72:915–923.
- Piening L. 1961. The occurrence of Pyrenophora teres on barley straw in Alberta. Can Plant Dis Surv. 41:299–300.
- Piening L. 1968. Development of barley net blotch from infested straw and seed. Can J Plant Sci. 48:623–625.
- Prairie Recommending Committee for Oat and Barley. 2015. Operating procedures, Prairie Recommending Committee for Oat and Barley, Prairie Grain Development Committee [Internet]. [cited [2015 Sept 11]. Available from: http://www.pgdc.ca/committees_ob.html
- Rau D, Attene G, Brown AHD, Nanni L, Maier FJ, Balmas V, Saba E, Schäfer W, Papa R. 2007. Phylogeny and evolution of mating-type genes from Pyrenophora teres, the causal agent of barley ‘net blotch’ disease. Curr Genet. 51:377–392.
- Rau D, Brown AHD, Brubaker CL, Attene G, Balmas V, Saba E, Papa R. 2003. Population genetic structure of Pyrenophora teres Drechs., the causal agent of net blotch in Sardinian landraces of barley (Hordeum vulgare L.). Theor Appl Genet. 106:947–959.
- Smedegard-Petersen V. 1978. Genetics of heterothallism in Pyrenophora graminea and P. teres. Trans Br Mycol Soc. 70:99–102.
- Statistics Canada. 2015. Table 001-0010 - Estimated areas, yield, production and average farm price of principal field crops, in metric units, annual, CANSIM (database) [Internet]. [cited 2015 Sept 11]. Available from: http://www5.statcan.gc.ca/cansim/a26?lang=eng&retrLang=eng&id=0010010&&pattern=&stByVal=1&p1=1&p2=31&tabMode=dataTable&csid=
- Steffenson BJ, Hayes PM, Kleinhofs A. 1996. Genetics of seedling and adult plant resistance to net blotch (Pyrenophora teres f. teres) and spot blotch (Cochliobolus sativus) in barley. Theor Appl Genet. 92:552–558.
- Steffenson BJ, Webster RK. 1992. Pathotype diversity of Pyrenophora teres f. teres on barley. Phytopathology. 82:170–177.
- Steffenson BJ, Webster RK, Jackson LF. 1991. Reduction in yield loss using incomplete resistance to Pyrenophora teres f. teres in barley. Plant Dis. 75:96–100.
- Tamang P, Neupane A, Mamidi S, Friesen T, Brueggeman R. 2015. Association mapping of seedling resistance to spot form net blotch in a worldwide collection of barley. Phytopathology. 105:500–508.
- Tekauz A. 1985. A numerical scale to classify reactions of barley to Pyrenophora teres. Can J Plant Pathol. 7:181–183.
- Tekauz A. 1990. Characterization and distribution of pathogenic variation in Pyrenophora teres f. teres and P. teres f. maculata from Western Canada. Can J Plant Pathol. 12:141–148.
- Tekauz A, Desjardins M, Kleiber F. 2011. Evaluating the Pyrenophora teres international standard barley differential set with Canadian isolates of the pathogen. Dundee, Scotland, UK: 4th International Workshop on Barley Leaf Blights (IWBLB).
- Turkington TK, Tekauz A, Xi K, Kutcher HR. 2011. Foliar diseases of barley: don’t rely on a single strategy from the disease management toolbox. Prairie Soils Crops. 4:142–150.
- van den Berg CGJ, Rossnagel BG. 1991. Epidemiology of spot-type net blotch on spring barley in Saskatchewan. Phytopathology. 81:1446–1452.
- Wang X, Mace ES, Platz GJ, Hunt CH, Hickey LT, Franckowiak JD, Jordan DR. 2015. Spot form of net blotch resistance in barley is under complex genetic control. Theor Appl Genet. 128:489–499.
- Williams KJ, Lichon A, Gianquitto P, Kretschmer JM, Karakousis A, Manning S, Langridge P, Wallwork H. 1999. Identification and mapping of a gene conferring resistance to the spot form of net blotch (Pyrenophora teres f. maculata) in barley. Theor Appl Genet. 99:323–327.
- Williams KJ, Platz GJ, Barr AR, Cheong J, Willsmore K, Cakir M, Wallwork H. 2003. A comparison of the genetics of seedling and adult plant resistance to the spot form of net blotch (Pyrenophora teres f. maculata). Aust J Agric Res. 54:1387–1394.
- Wu HL, Steffenson BJ, Zhong S. 2003. Genetic variation for virulence and RFLP markers in Pyrenophora teres. Can J Plant Pathol. 25:82–90.
- Xi K, Burnett PA, Turkington TK, Tekauz A, Helm JH. 1999. Determining quantitative resistance of barley cultivars at the seedling stage to net blotch caused by Pyrenophora teres. Can J Plant Pathol. 21:284–290.