Abstract
Pyrenophora teres f. teres (Ptt) and Pyrenophora teres f. maculata (Ptm), the causal agents of the net and spot forms of net blotch of barley, respectively, can be managed in western Canada with fungicides containing propiconazole and pyraclostrobin. Given the potential for development of fungicide resistance, the sensitivity of a collection of Ptt and Ptm isolates to propiconazole and pyraclostrobin was evaluated using microtitre plate bioassays. The concentration of propiconazole needed to inhibit fungal growth by 50% (EC50) was 1.5 mg L−1 for Ptt and 2.3 mg L−1 for Ptm, while the EC50 of pyraclostrobin was 0.015 mg L−1 for Ptt and 0.024 mg L−1 for Ptm. Subsequently, 39 Ptt and 27 Ptm isolates were screened with discriminatory doses of 5 mg propiconazole L−1 and 0.15 mg pyraclostrobin L−1. Inhibition of growth as a result of propiconazole was 12–95% for Ptt and 48–92% for Ptm; growth inhibition as a result of pyraclostrobin was 40–100% and 24–100%, respectively. Two Ptt isolates were insensitive to propiconazole, while one Ptm isolate was insensitive to pyraclostrobin. The latter also showed decreased sensitivity to propiconazole. The identification of net blotch isolates insensitive to these fungicides emphasizes the need for farmers to employ integrated crop management strategies to avoid fungicide resistance build-up.
Résumé
Pyrenophora teres f. teres (Ptt) et Pyrenophora teres f. maculata (Ptm), les agents causaux respectifs des formes réticulée et helminthosporienne de la tache réticulée de l’orge, peuvent être maîtrisés dans l’Ouest canadien avec des fongicides à base de propiconazole et de pyraclostrobine. Compte tenu de la capacité de ces agents à développer une résistance aux fongicides, la sensibilité d’une collection d’isolats de Ptt et de Ptm au propiconazole et à la pyraclostrobine a été évaluée par essais biologiques au moyen de microtitrage sur plaque. La concentration de propiconazole requise pour inhiber de 50% la prolifération fongique (EC50) était de 1.5 mg L−1 pour Ptt et de 2.3 mg L−1 pour Ptm, tandis que l’EC50 pour la pyraclostrobine était de 0.015 mg L−1 pour Ptt et de 0.024 mg/l−1 pour Ptm. Par la suite, 39 isolats de Ptt et 27 de Ptm ont été criblés avec des doses discriminatoires de 5 mg L−1 de propiconazole et de 0.15 mg L−1 de pyraclostrobine. L’inhibition de la prolifération engendrée par le propiconazole était de 12 à 95% chez Ptt et de 48 à 92% chez Ptm; l’inhibition de la prolifération engendrée par la pyraclostrobine était de 40 à 100% et de 24 à 100%, respectivement. Deux isolats de Ptt étaient insensibles au propiconazole, tandis qu’un isolat de Ptm l’était à la pyraclostrobine. Ce dernier a également affiché une sensibilité réduite au propiconazole. L’identification des isolats de tache réticulée insensibles à ces fongicides souligne l’importance pour les producteurs d’utiliser des stratégies de gestion intégrée des cultures afin d’entraver le développement de la résistance aux fongicides.
Introduction
The necrotrophic fungus Pyrenophora teres Drechs. (anamorph: Drechslera teres [Sacc.] Shoem.) has two morphologically identical but genetically distinct forms: P. teres f. teres (Ptt) and P. teres f. maculata (Ptm), which incite the net form of net blotch (NFNB) and spot form of net blotch (SFNB) of barley (Hordeum vulgare L.), respectively (McDonald Citation1963; Smedegard-Petersen Citation1978). These are among the most economically important foliar diseases across the major barley growing regions of the world, including western Canada with 2.5 million hectares planted to barley in 2016 (Tekauz Citation1990; Steffenson Citation1997; Liu et al. Citation2011; Statistics Canada Citation2016). Yield losses of 10–40% are typical in severe cases of NFNB, although Ptt has the potential to cause total yield loss (Steffenson et al. Citation1991; Steffenson Citation1997; Murray & Brennan Citation2010). Likewise, yield losses of up to 44% were reported for SFNB (Jayasena et al. Citation2007). Crop rotation, fungicide application and the deployment of resistant cultivars can be used to manage net blotch of barley in western Canada (van den Berg & Rossnagel Citation1991; Turkington et al. Citation2004, Citation2005, Citation2006, Citation2011, Citation2012, Citation2015). However, many western Canadian farmers have adopted a canola-cereal-canola rotation, which is not sufficient to manage barley leaf spot diseases such as net blotch (Mathre Citation1997; Tekauz Citation2003; Turkington et al. 2011, 2015). Moreover, many two and six row barley cultivars, especially malting types, are susceptible to net blotch (Alberta Government Citation2016a). As a consequence, many farmers routinely use fungicides as their main tool for managing barley leaf diseases (Turkington et al. 2011, 2015; Poole & Arnaudin Citation2014). Most fungicides are registered for use on barley from stem elongation to head emergence, but also are registered for tank mixing with herbicides and may be applied at earlier crop growth stages (Turkington et al. Citation2015; Alberta Government Citation2016a). When weather conditions are conducive to disease development and the barley cultivar is susceptible, many farmers will follow an application of fungicide that is tank-mixed with herbicide(s) with a second fungicide-only application between flag leaf and head emergence (Turkington et al. Citation2015; Alberta Government Citation2016a).
Both Ptt and Ptm are stubble-borne pathogens, producing asexual conidia and sexual pseudothecia, which produce ascospores on overwintered infected crop debris (Piening Citation1961, Citation1968; van den Berg & Rossnagel Citation1991; Peever & Milgroom Citation1994; Duczek et al. Citation1999). Based on a 1:1 ratio of the two mating types for Ptt and Ptm in western Canada (Akhavan et al. Citation2015), it is likely that both forms of the net blotch pathogen undergo regular cycles of sexual reproduction in this region. Moreover, Ptt and Ptm populations in western Canada are genetically diverse, with almost 90% of the population consisting of distinct haplotypes (Akhavan et al. Citation2016b). These diverse Ptt and Ptm populations with mixed reproduction and an outcrossing mating system fall into the category of pathogens that have a greater likelihood of quickly adapting to fungicides with a single mode of action (McDonald & Linde Citation2002), ultimately resulting in a reduction or loss of fungicide effectiveness.
The fungicides propiconazole and pyraclostrobin are commonly applied to manage net blotch on barley in western Canada (Alberta Government Citation2016b). Propiconazole has been used on various crops including barley since the early 1990s as the foliar fungicide Tilt (propiconazole, 250 g L−1; Syngenta Canada Inc.) (Xue et al. Citation1994; Thomas Citation1997). Propiconazole is a triazole belonging to the demethylation inhibitors (DMIs) group. This group of fungicides inhibits 14α–sterol demethylase, an enzyme used by fungi to synthesize ergosterol, which in turn is needed for fungal cell membrane development (Dahl et al. Citation1987; reviewed in Parker et al. Citation2014). Excessive use of DMIs has been reported to select for insensitivity in cereal pathogens such as Ptt (Mair et al. Citation2016), Blumeria graminis DC Speer f. sp. hordei Marchal (Délye et al. Citation1998), Zymoseptoria tritici (Desm.) Quaedvlieg & Crous [formerly known as Mycosphaerella graminicola (Fuckel) Schroeter] (Leroux et al. Citation2007; Bean et al. Citation2009; Cools & Fraaije Citation2013), and Fusarium graminearum Schwabe [teleomorph: Gibberella zeae (Schwein.) Petch] (Talas & McDonald Citation2015), increasing the level of insensitivity within these pathogen populations (Kuck et al. Citation2012). Insensitivity to fungicides usually results from point mutations in the gene(s) encoding the proteins targeted by specific fungicides. Alterations in the CYP51 gene in plant pathogens were reported to be one of the major mechanisms resulting in reduced sensitivity towards DMIs (reviewed in Parker et al. Citation2014). Mair et al. (Citation2016) reported the occurrence of insensitivity among Ptt isolates collected from Australia against multiple DMI compounds. This insensitivity was correlated with both a mutation in the Cyp51A gene and overexpression of both the Cyp51A and Cyp51B genes, which are paralogues of the Cyp51 gene. Rallos and Baudoin (Citation2016) also reported that CYP51 over-expression and target-site mutation contributed to insensitivity in Erysiphe necator.
Pyraclostrobin is classified within the strobilurin or quinone outside inhibitors (QoI) group of fungicides, and is the active ingredient in the fungicide Headline 250 EC (BASF Canada, Mississauga, ON, Canada). Products containing pyraclostrobin have been registered and utilized by western Canadian farmers for cereal leaf spot management since the early 2000s (BASF Citation2003; Government of Canada: Health Canada Pest Management Regulatory Agency Citation2011). Marzani (Citation2011) investigated the effectiveness of several QoI fungicides on Ptt isolate growth and reported that pyraclostrobin was the most active QoI, resulting in high growth inhibition. Strobilurins have a single site of activity and prevent mitochondrial respiration in fungi by binding to the Qo site of cytochrome bc1, interfering with electron transfer in complex III (Sauter et al. Citation1999; Bartlett et al. Citation2002; Grasso et al. Citation2006). The Fungicide Resistance Action Committee (FRAC Citation2016) has labelled strobilurins as compounds at a high risk of selecting for insensitivity in pathogen populations.
In the current study, a microtitre bioassay was used to assess the level of fungicide sensitivity. This method is more efficient relative to other more conventional techniques, such as the agar radial growth bioassay (Tremblay et al. Citation2003). The microtitre bioassay is an approved method of assessing fungicide sensitivity for P. teres (FRAC Citation2006a, Citation2009) and other species of Pyrenophora (FRAC Citation2006b) to DMI, QoI and succinate dehydrogenase inhibitor (SDHI) fungicides. It involves measurement of the optical density of fungal tissues in the presence of different concentrations of chemicals in a 96-well microplate, followed by comparison of the growth of the same isolate in non-amended controls (Pijls et al. Citation1994; Tremblay et al. Citation2003). For DMIs, discriminatory doses from near the half-maximal effective concentration (EC50) value (Smith et al. Citation1991; Peever & Milgroom Citation1992, Citation1993) to 100 times greater than the baseline EC50 (Villani & Cox Citation2011) have been employed. The inhibition of fungal growth for a single fungicide concentration close to the EC50 value was shown to be an appropriate response to measure with DMI fungicides (Smith et al. Citation1991) and was also shown in P. teres to be highly correlated with EC50 values determined with a series of concentrations (Peever & Milgroom Citation1992, Citation1993). However, Romero & Sutton (Citation1997) suggested that a discriminatory dose slightly higher than both the mean EC50 value and the highest detected EC50 is more useful for monitoring sensitivity to DMI fungicides. For QoIs, discriminatory doses from near the EC50 value (Mondal et al. Citation2005) to almost 70 times greater than the baseline EC50 (Fraser et al. Citation2016) have been typically employed.
Information on the sensitivity of local isolates of P. teres to fungicides used commercially is required in the formulation of integrated net blotch management strategies. Although both propiconazole and pyraclostrobin fungicides have been applied extensively in barley fields in western Canada, no information is available on the fungicide sensitivity of P. teres populations to these compounds. The objectives of the current study were to: (i) determine the EC50 of propiconazole (DMI) and pyraclostrobin (QoI) for a set of Ptt and Ptm isolates from western Canada; (ii) test the null hypothesis of a lack of significant differences between the EC50 values obtained for Ptt and Ptm; (iii) quantify the degree of sensitivity to propiconazole and pyraclostrobin in a representative collection of Ptt and Ptm isolates from commercial barley crops across western Canada; and (iv) test the null hypothesis of a lack of significant differences in propiconazole and pyraclostrobin sensitivity among Ptt and Ptm isolates from the three western Canadian provinces of Alberta, Saskatchewan and Manitoba.
Materials and methods
Fungal isolation and preparation of inoculum
A total of 39 Ptt and 27 Ptm isolates collected from commercial fields and research plots across western Canada (Alberta, Saskatchewan and Manitoba) were studied. These included 36 Ptt and 21 Ptm isolates representing different clades identified in earlier genetic analyses (Akhavan et al. Citation2016b), plus an additional three isolates of Ptt and six isolates of Ptm from another collection made in 2012. All 39 Ptt and 27 Ptm isolates also were previously characterized for their virulence on a selection of barley genotypes and differentials (Akhavan et al. Citation2016a). The collection included 17 Ptt isolates from Alberta, 12 from Saskatchewan, and 10 from Manitoba, as well as eight Ptm isolates from Alberta, 10 from Saskatchewan, and nine from Manitoba (Supplementary Table 1). Two previously collected isolates of Ptt (WRS858 and WRS102) and one of Ptm (WRS857), which were obtained from Dr A. Tekauz (Agriculture and Agri-Food Canada, Winnipeg, MB), were also included for comparison in experiments to test the sensitivity of Ptt and Ptm isolates to propiconazole and pyraclostrobin. These three isolates were collected prior to registration of both propiconazole and pyraclostrobin in Canada. Isolate WRS102 was originally collected from Saskatchewan (Metcalfe et al. Citation1970), while WRS858 and WRS857 were collected from Manitoba (Tekauz & Mills Citation1974; Ho et al. Citation1996).
Table 1. The effective concentration of propiconazole (mg L−1) required to inhibit fungal growth by 50% (EC50) in eight isolates each of Pyrenophora teres f. teres (Ptt) and P. teres f. maculata (Ptm).
To obtain single-spore isolates, segments (10 mm × 5 mm) were cut from barley leaves with symptoms of NFNB or SFNB, and soaked in 50% ethanol for 15 s and in 2% sodium hypochlorite for 30 s, flushed with sterile water and placed on moistened filter paper in 9 cm-diameter plastic Petri dishes (Tekauz Citation1990). To enhance fungal sporulation on the leaves, the Petri dishes were incubated at 20 ± 0.5°C in a growth chamber with a 12 h photoperiod under a mixture of common fluorescent lamp light and a black light blue fluorescent lamp emitting near ultraviolet light at 368 nm. Following 3–5 days of incubation, single conidia of P. teres were transferred onto 10% V-8 juice agar (V-8 juice, 100 mL; CaCO3 3 g; Difco agar 20 g; distilled water 900 mL) amended with 50 mg L−1 kanamycin, and incubated as above to form new colonies (Tekauz Citation1990). Single-spore isolates were preserved at −80°C in an ultra-low temperature freezer, either as 0.5 cm-diameter mycelial plugs in 50% sterile glycerol or as conidial suspensions in 25% sterile glycerol. Identification of the isolates as P. teres was based on the morphological features of the conidiophores and conidia and species-specific PCR analysis. The form of P. teres (Ptt or Ptm) was confirmed by form-specific PCR as described by Akhavan et al. (Citation2015). To validate the results of the form-specific PCR, isolates were inoculated onto the barley cultivar ‘Steptoe’ as described previously by Tekauz (Citation1990).
Conidial suspensions were produced as per the technique developed by Lamari & Bernier (Citation1989) for Pyrenophora tritici-repentis (Died.) Drechs., as modified by Aboukhaddour et al. (Citation2013). Briefly, the isolates were removed from a −80°C ultra-low temperature freezer, and the mycelial plugs were transferred onto fresh 10% V-8 juice agar supplemented with 50 mg L−1 kanamycin in 9 cm-diameter Petri dishes, with one plug in the centre of each Petri dish. Alternatively, for those isolates preserved as a frozen conidial suspension, approximately 100 µL of thawed spore suspension was transferred onto the same medium. The Petri dishes were incubated in darkness at room temperature for 5–7 days, until the colonies were 4–5 cm in diameter. Approximately 10 mL of sterile distilled water was then added to each Petri dish, and the fungal mycelium was flattened with the bottom of a sterile glass tube. The water was decanted and the Petri dishes were incubated overnight under fluorescent light at room temperature, followed by 24 h incubation in darkness at 15°C to encourage sporulation. The spore suspensions were prepared by adding 4–5 mL of sterile distilled water to the fungal colonies, and then gently dislodging the conidia from the conidiophores with the help of a sterile inoculation loop or an artist’s brush.
Calculation of the mean EC50
Independent experiments were run to determine EC50(s) for propiconazole and pyraclostrobin. Experiments were conducted using a completely randomized design (CRD) with three replicate wells assigned for each treatment. There were three replicate control wells per isolate that were not amended with fungicide. All experiments were repeated.
Fungal spore concentrations were estimated using a Fuchs Rosenthal Counting Chamber (Hausser Scientific, Blue Bell, PA) and manually adjusted to 1 × 104 conidia per mL for both Ptt and Ptm. An aliquot of 100 µL of the spore suspension, containing approximately 1000 spores, was transferred into each well of a 96-well microtitre plate (Thermo Scientific, Waltham, MA, Catalog # 167008), which contained 100 µL of the fungicide solution prepared in 2× concentrated potato dextrose broth (PDB; Difco Laboratories, Detroit, MI). The combined solution was mixed thoroughly. Kanamycin (100 mg L−1) and streptomycin (20 mg L−1) were added to the concentrated media to avoid bacterial contamination. Technical grade propiconazole and pyraclostrobin fungicides were purchased from Sigma-Aldrich Corporation (USA) and were diluted to achieve end concentrations. The EC50 of propiconazole was determined based on eight different concentrations of the fungicide (0, 0.25, 0.5, 1, 2, 3, 4 and 5 mg L−1) and eight isolates each of Ptt and Ptm. These isolates were randomly selected from the full collection of 39 Ptt and 27 Ptm isolates. The EC50 value of pyraclostrobin was determined based on eight different concentrations of the fungicide (0, 0.0015, 0.0075, 0.015, 0.075, 0.15, 0.45 and 1.05 mg L−1) and 12 isolates each of Ptt and Ptm, with four isolates of each form randomly selected from each province.
The endpoint optical density (OD) of samples in the 96-well microtitre plates was measured at 405 nm, on a Molecular Devices SpectraMax M3 microtitre plate reader (Sunnyvale, CA), immediately after filling the plates. Plates were then incubated in darkness at 20°C for 7 days. Following incubation, the OD was measured and final values for absorbance were obtained by subtracting the values of the initial reading from those of the final reading. Subsequently, for each isolate-concentration replicate, growth inhibition was calculated using the equation: 1 – [(absorption for each treatment/absorption of non-amended control)], and then presented as a percentage.
Since some fungi exhibit in vitro insensitivity to the QoIs based on an alternative respiratory pathway using alternative oxidase 1 (AOX), a preliminary assessment was conducted to determine if the addition of salicylhydroxamic acid (SHAM), which blocks this pathway (Wood & Hollomon Citation2003; Miguez et al. Citation2004), was required. The pyraclostrobin sensitivity of three isolates each of Ptt and Ptm was assessed in the presence or absence of 100 mg L−1 SHAM (99%; Sigma-Aldrich, St. Louis, MO). Although a paired t-test indicated no significant difference for the results obtained in the presence or absence of this chemical (results not shown), all pyraclostrobin bioassays were conducted in the presence of 100 mg L−1 SHAM to avoid any potential alternative respiration, which could confound sensitivity assessments.
Evaluation of the sensitivity of Ptt and Ptm isolates
As in the case for the EC50 assessment, independent experiments were performed for each fungicide and for each pathogen form, employing a completely randomized design (CRD) with three replicate wells per isolate × concentration combination. All experiments were repeated. There were three replicate control wells per isolate with no fungicide amendment. Each of the 39 Ptt and 27 Ptm isolates was tested using the microtitre bioassay procedure, described previously, at a discriminatory dose of 5.0 mg L−1 for propiconazole, and 0.15 mg L−1 for pyraclostrobin. For both fungicides, these concentrations provided overall growth inhibitions of almost 90% for Ptt and 86% for Ptm when averaged over the representative isolates used in the calculation of mean EC50 values (). The dose response curve was not, however, used directly to calculate the average EC50 values, so as to not violate the assumptions of normality and homogeneity of variance required to pool the data from individual isolates. Therefore, average EC50 values were estimated as described in the data analysis section below. The discriminatory dose of propiconazole (5 mg L−1) was 2.6× greater than the average EC50 values calculated for the Ptt and Ptm isolates, and was 1.4 times greater than the highest calculated EC50 value among the 16 examined P. teres isolates which was 3.69 mg L−1 for Ptm isolate SKV12. The discriminatory dose of pyraclostrobin (0.15 mg L−1) was 7.7× greater than the average EC50 values calculated for Ptt and Ptm, and was 2.5 times greater than the highest calculated EC50 value among the 24 examined P. teres isolates which was 0.061 mg L−1 for Ptm isolate MBV37.
Fig. 1 Dose response curve of the mean fungal growth inhibition of representative Pyrenophora teres f. teres and P. teres f. maculata isolates from western Canada in response to propiconazole (a) and pyraclostrobin (b). Eight and 12 isolates each of Ptt and Ptm, respectively, were employed in the experiments with propiconazole (16 isolates in total) and pyraclostrobin (24 isolates in total). Three replicate wells were included for each combination of isolate and concentration. Experiments were conducted twice, and the mean fungal growth inhibitions were averaged. Growth inhibition is relative to non-amended control treatments.
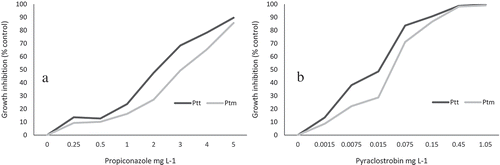
Fungal isolates were grouped as sensitive to propiconazole and pyraclostrobin if growth was reduced by >70%, intermediate if growth was reduced by 30–70%, and insensitive if growth was reduced <30% (Thaher Citation2011; Bowness et al. Citation2016). For each isolate replicate, per cent growth inhibition was calculated as described above and averaged over the three replicates and the two experiments. All isolates for which reduced sensitivity was observed were examined further by treatment with higher discriminatory doses of each fungicide. In the case of propiconazole, all isolates with growth inhibition values of <50% at 5.0 mg L−1 were also evaluated against discriminatory doses of 10 mg L−1, 20 mg L−1 and 100 mg L−1 of the fungicide. In the case of pyraclostrobin, isolates with growth inhibition values of <50% at 0.15 mg L−1 were further tested against a new discriminatory dose of 0.45 mg L−1.
Data analysis
For each isolate, the EC50 value was independently estimated by probit analysis (Finney Citation1971; Stammler et al. Citation2012) with SPSS statistical software (IBM SPSS Statistics for Windows, Version 23.0, IBM Corporation, Armonk, NY). The maximum likelihood procedure was used for linear regression analysis to fit the regression of the response versus the concentration (Marzani Citation2011). Briefly, a log10-transformed fungicide concentration was used to linearize fungal growth inhibition (as a proportion of the control) and a regression line was fitted. The resulting linear regression equation was used to calculate the fungicide concentration at which fungal growth was inhibited by 50% (EC50) for each isolate. Paired t-tests (PROC TTEST) were performed with SAS 9.3 (SAS Institute Inc., Cary, NC) to determine if the EC50 values calculated for a set of isolates in an experiment were significantly (P < 0.05) different from the EC50 values calculated for the same set when the entire test was repeated. Since there was no significant difference between the estimated values, the final EC50 values of the eight Ptt and eight Ptm isolates (for propiconazole) and 12 Ptt and 12 Ptm isolates (for pyraclostrobin) were calculated by averaging the arithmetic means of the calculated EC50 values from the two experiments. For analysis of the fungal growth inhibition data, the assumptions of normality and variance homogeneity of the residuals were tested using Shapiro–Wilk and Kolmogorov–Smirnov tests, and Leaven’s test, respectively (PROC UNIVARIATE) in SAS 9.3 (SAS Institute). The growth inhibition data were then subjected to a one-way analysis of variance (PROC GLM) to test the null hypothesis of a lack of significant difference in the propiconazole and pyraclostrobin sensitivities of Ptt and Ptm isolates from Alberta, Saskatchewan and Manitoba.
Results
Determination of EC50 of propiconazole for Ptt and Ptm isolates
The mean EC50 of propiconazole for the Ptt isolates was 1.5 mg L−1 and ranged from 0.8 mg L−1 for isolate MB15 to 3.0 mg L−1 for isolate AB38. Similarly, the mean EC50 of propiconazole for the Ptm isolates was 2.3 mg L−1, and ranged from 1.1 mg L−1 for isolate MB22 to 3.7 mg L−1 for isolate SKV12 (). A two-tailed t-test indicated that the difference between the EC50 values obtained for Ptt and Ptm was just above the P = 0.05 level of significance (P = 0.07); therefore, we failed to reject the null hypothesis of a lack of significant difference between the propiconazole EC50 values obtained for Ptt and Ptm.
Assessing propiconazole sensitivity of Ptt and Ptm isolates
No significant differences were observed in the propiconazole sensitivity of Ptt or Ptm isolates collected from each of the western Canadian provinces of Alberta, Saskatchewan or Manitoba (P = 0.25 for Ptt and P = 0.15 for Ptm). Growth inhibition values in response to propiconazole (5 mg L−1) among the 39 Ptt isolates ranged from 12.4% for isolate AB48 to 95.2% for isolate AB28. A mean growth inhibition of 78.7% was obtained for all of the Ptt isolates combined. Two isolates, AB48 and AB11, collected from the Lacombe and Bentley areas in central Alberta, had a growth inhibition of <30%. Another four isolates had growth inhibition values of 30–70%, while the growth inhibition of the remaining 33 isolates was 70–100% (, ). Growth inhibition for the Ptt reference isolates WRS102 and WRS858 was 84.8% and 92.0%, respectively. The isolates AB48 and AB11 were designated as insensitive to the fungicide, based on the low growth inhibition observed, and also were assessed at discriminatory doses of 10 mg L−1, 20 mg L−1 and 100 mg L−1 propiconazole. Both isolates had a growth inhibition of <50% at the 10 mg L−1 dose, but growth inhibition at 20 mg L−1 was >50%. Neither AB48 nor AB11 grew at a dose of 100 mg L−1 propiconazole.
Table 2. Propiconazole sensitivity of Pyrenophora teres f. teres (Ptt) and P. teres f. maculata (Ptm) isolates collected in 2009–2012 from Alberta, Saskatchewan and Manitoba, Canada.
Fig. 2 Frequency distribution of inhibition of fungal growth in 39 Pyrenophora teres f. teres and 27 P. teres f. maculata isolates from western Canada in response to 5 mg L−1 propiconazole in potato dextrose broth. Inhibition of fungal growth is expressed as a percentage relative to a control treatment in which no propiconazole was included.
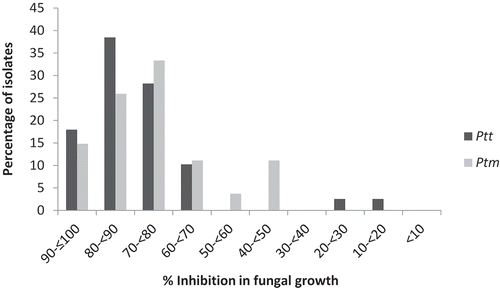
For the Ptm isolates, growth inhibition in response to propiconazole (5 mg L−1) ranged from 48.2% for isolate SK60 to 92.1% for isolate ABV14. The mean growth inhibition was 75.0% over all of the Ptm isolates examined. No isolates had growth inhibition values of <30%, while growth inhibition ranged from 30–70% for seven isolates and from 70–100% for the remaining 20 (, ). Growth inhibition for the Ptm reference isolate WRS857 was 73.2%. Three isolates with growth inhibition values of 48–49% (MB26, SK64 and SK60) also were evaluated at discriminatory doses of 10 mg L−1, 20 mg L−1 and 100 mg L−1 propiconazole. Only SK64 from Dollard, Saskatchewan, had a growth inhibition of <50% at 10 mg L−1 or 20 mg L−1 propiconazole, but did not grow at a dose of 100 mg L−1.
Determination of EC50 of pyraclostrobin for Ptt and Ptm isolates
The mean EC50 of pyraclostrobin for the Ptt isolates was 0.015 mg L−1, and ranged from 0.002 mg L−1 for isolate MB03 to 0.059 mg L−1 for isolate SK24. In the case of the Ptm isolates, the mean EC50 of pyraclostrobin was 0.024 mg L−1, ranging from 0.008 mg L−1 for isolate SK69 to 0.061 mg L−1 for isolate MBV37 (). A two-tailed t-test indicated that the difference between the EC50 values obtained for Ptt and Ptm was just above the P = 0.05 level of significance (P = 0.06). Therefore, as was the case for propiconazole, we failed to reject the null hypothesis of a lack of significant difference between the pyraclostrobin EC50 values obtained for Ptt and Ptm.
Table 3. The effective concentration of pyraclostrobin (mg L−1) required to inhibit fungal growth by 50% (EC50) in 12 isolates each of Pyrenophora teres f. teres and P. teres f. maculata.
Assessing pyraclostrobin sensitivity of Ptt and Ptm isolates
No significant differences were detected in the pyraclostrobin sensitivity of Ptt or Ptm originating from Alberta, Saskatchewan or Manitoba (P = 0.11 for Ptt, and P = 0.20 for Ptm). Growth inhibition values in response to pyraclostrobin (0.15 mg L−1) for the 39 Ptt isolates ranged from 40.5% for isolate ABV01 to 100% for isolates ABV28, SK01 and MB05. No isolates had a growth inhibition of <30%, four had a growth inhibition of 30–70%, and 35 had a growth inhibition of 70–100%. The mean growth inhibition for all of the Ptt isolates was 89%. The Ptt reference isolates WRS102 and WRS858 had growth inhibition values of 84% and 99%, respectively. The sensitivity of two isolates with growth inhibition values of 40.5% and 44.9% (ABV01 and SK24, respectively) also was evaluated at a discriminatory dose of 0.45 mg L−1 pyraclostrobin, but growth of both was inhibited by >50% at this concentration of fungicide.
In the case of the 27 Ptm isolates, growth inhibition ranged from 23.8% for isolate SK64 to 100% for isolate AB79. One isolate (SK64) had a growth inhibition value of <30%, five isolates had a growth inhibition of 30–70%, and 21 isolates had a growth inhibition of 70–100% (, ). The mean growth inhibition over all of the Ptm isolates was 84.6%. Growth of the Ptm reference isolate WRS857 was completely inhibited (100%). Isolate SK64 was designated as insensitive to pyraclostrobin based on its low growth inhibition, and this isolate, along with three others (SK100, MB16 and MB22) that had growth inhibition values of 38–49%, were evaluated under a higher discriminatory dose of 0.45 mg L−1 pyraclostrobin. At this concentration, all of the isolates were inhibited by >50%. Isolate SK64 also showed decreased sensitivity to propiconazole (see above).
Table 4. Pyraclostrobin sensitivity of Pyrenophora teres f. teres (Ptt) and P. teres f. maculata (Ptm) collected in 2009–2012 from Alberta, Saskatchewan and Manitoba, Canada.
Fig. 3 Frequency distribution of inhibition of fungal growth in 39 Pyrenophora teres f. teres and 27 P. teres f. maculata isolates from western Canada in response to 0.15 mg L−1 pyraclostrobin in potato dextrose broth. Inhibition of fungal growth is expressed as a percentage relative to a control treatment in which no pyraclostrobin was included. Experiments were performed in the presence of 100 mg L−1 salicylhydroxamic acid (SHAM).
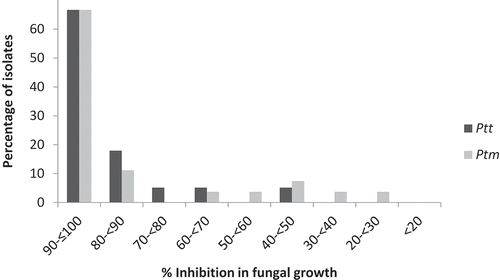
Discussion
Propiconazole and pyraclostrobin are commonly used to manage net blotch of barley and other cereal leaf diseases in western Canada. To evaluate the sensitivity of Ptt and Ptm to these fungicides, the first step was to establish an EC50 for each compound. In fungicide sensitivity screening, isolates with no history of exposure to the fungicidal compounds are typically used to calculate a baseline EC50. However, since there were no P. teres isolates collected before the registration of propiconazole or pyraclostrobin, randomly selected Ptt and Ptm isolates from across western Canada were used to estimate the baseline EC50 of these fungicides in this study. The EC50 values calculated based on the sensitivity of these isolates, which may have been exposed to propiconazole or pyraclostrobin in the past, could be higher than the EC50 values obtained for isolates that had never been exposed to these products. Nonetheless, this study provides important information on the current fungicide sensitivity of P. teres populations in western Canada, serving as a baseline against which to compare future assessments. To our knowledge, this is the first evaluation of the sensitivity of Ptt and Ptm to propiconazole and pyraclostrobin in this important barley growing region.
Ascospores and conidia are the most important primary inocula in the disease cycle of both Ptt and Ptm in western Canada, and are responsible for primary infection of barley tissues (Piening Citation1961, Citation1968; van den Berg & Rossnagel Citation1991; Duczek et al. Citation1999). Conidia are also the main inoculum source for most secondary infections. Therefore, conidia were used in this study to calculate the EC50 values, and also to assess the sensitivity of a collection of P. teres isolates to propiconazole and pyraclostrobin.
The EC50 of propiconazole for the Ptt isolates ranged from 0.8 to 3.0 mg L−1 with a mean value of 1.5 mg L−1, while the EC50 for the Ptm isolates ranged from 1.1 to 3.7 mg L−1 with a mean of 2.3 mg L−1 (). Using an agar radial growth procedure, Campbell & Crous (Citation2002) calculated mean EC50 values of 1.2 and 0.4 mg L−1 propiconazole for two Ptt populations (with a range of 0.004–7.693 mg L−1 for individual isolates), and of 3.0 and 1.4 mg L−1 for two Ptm populations (with a range of 0.138–5.772 mg L−1) collected from four separate fields in South Africa. The wider range of EC50 values reported by Campbell & Crous (Citation2002) suggests the occurrence of more variability in the propiconazole sensitivity of South African versus Canadian isolates of P. teres. Direct comparisons are difficult to make, however, since the EC50 values in the current study were determined in a microtitre plate bioassay using conidial suspensions, as opposed to the mycelial growth that was measured by Campbell & Crous (Citation2002).
In the case of pyraclostrobin, the EC50 values for individual Ptt isolates ranged from 0.002 to 0.059 mg L−1 with a mean of 0.015 mg L−1. The EC50 values for Ptm isolates ranged from 0.008 to 0.061 mg L−1 pyraclostrobin with a mean of 0.024 mg L−1 (). Marzani (Citation2011) calculated a mean EC50 of 0.1–0.22 mg L−1 pyraclostrobin for wild-type Ptt isolates, and of 0.28–0.69 mg L−1 for mutant Ptt isolates with increased pyraclostrobin insensitivity. Marzani et al. (Citation2013) observed similar EC50 values for some of the mutant and wild-type isolates, suggesting that isolates were highly sensitive to pyraclostrobin. While the EC50 values reported by Marzani (Citation2011) were substantially higher than those reported in this study, those estimates were based on a modified radial growth bioassay compared with the microtitre procedure employed in the current study, making direct comparisons difficult.
Only two of the 39 Ptt isolates, AB48 and AB11, both of which were collected in central Alberta, had a growth inhibition of <30% and thus were regarded as propiconazole insensitive when tested at a discriminatory dose of 5 mg L−1 propiconazole. These two isolates were closely related genetically, as revealed previously by simple sequence repeat marker analysis (Akhavan et al. Citation2016a). The growth inhibition of both isolates remained <50% even when the discriminatory dose was doubled to 10 mg L−1 propiconazole. While no Ptm isolates were designated as insensitive to propiconazole, the growth inhibition of isolates SK60 and SK64 was only 48.2% and 48.4%, respectively. Further testing with a discriminatory dose of 10 mg L−1 propiconazole showed that inhibition of the growth of SK64 remained <50%. When isolates AB48, AB11 and SK64 were evaluated at an even higher discriminatory dose of 20 mg L−1 propiconazole, only SK64 had a growth inhibition of <50%. On the basis of these results, isolate SK64 appears to be the most insensitive to propiconazole among all Ptt and Ptm isolates evaluated. None of the isolates grew on 100 mg L−1 propiconazole, a concentration almost 53× greater than the mean EC50 values calculated for Ptt and Ptm. This suggests that it is unlikely that any of the P. teres isolates examined possess extreme or qualitative insensitivity to propiconazole. Alterations in the CYP51 gene in many plant pathogens (reviewed by Parker et al. Citation2014), including Ptt (Mair et al. Citation2016), have been reported to be the main mechanism for increased insensitivity towards DMIs. Azole insensitivity is increasing in pathogen populations as a result of the selection pressure imposed by the excessive application of triazole fungicides (Parker et al. Citation2014). Interestingly, isolate SK60 was previously reported to be one of the two Ptm isolates virulent on barley breeding line CI 9214, which is classified as highly resistant to SFNB (Akhavan et al. Citation2016a).
None of the Ptt isolates out of the 39 examined had a growth inhibition of <30% when tested at a discriminatory dose of 0.15 mg L−1 pyraclostrobin (, ). Marzani (Citation2011) found that among the QoI fungicides they tested on a collection of Ptt isolates, pyraclostrobin and picoxystrobin were highly inhibitory, whilst the efficacy of other compounds was less pronounced. In the current study, only one isolate of Ptm (SK64) had a growth inhibition of <30% in response to 0.15 mg L−1 pyraclostrobin (, ). This is the same isolate that also exhibited increased insensitivity to propiconazole, suggesting that this isolate is tolerant to both products. However, when evaluated against a higher discriminatory dose of 0.45 mg L−1 pyraclostrobin, growth of all isolates was inhibited by >50%. Since this dose was only 23× the average EC50, it appears that none of the isolates in the current study had qualitative insensitivity to pyraclostrobin. In contrast, such extreme insensitivity to pyraclostrobin has been reported previously in western Canada for both Ascochyta rabiei on chickpea and Mycosphaerella pinodes on field pea (Gossen & Anderson Citation2004; Chang et al. Citation2007; Wise et al. Citation2008, Citation2009; Thaher Citation2011; Bowness et al. Citation2016). Two isolates of A. rabiei had an EC50 value almost 704× greater than that of baseline isolates (Wise et al. Citation2009). Similarly, the mean EC50 of M. pinodes pyraclostrobin-insensitive isolates was nearly 1500× that of sensitive controls (Bowness et al. Citation2016). Mutations (G143A and F129L) in the mitochondrial target gene cytochrome b have been found to be associated with the insensitivity of many plant pathogens to QoIs (Grasso et al. Citation2006; Sierotzki et al. Citation2007). The G143A mutation, which is stronger and more frequent, results in qualitative insensitivity to the fungicide, while the F129L mutation results in moderate insensitivity (Gisi et al. Citation2000; Sierotzki et al. Citation2007). In P. teres, only the F129L mutation has been reported to confer pyraclostrobin insensitivity (Semar et al. Citation2007; Sierotzki et al. Citation2007; Marzani et al. Citation2013). The current findings are consistent with those of other studies (Sierotzki et al. Citation2007; Marzani et al. Citation2013) which reported there was no qualitative or extreme insensitivity to pyraclostrobin in the net blotch fungus.
No significant differences were observed in the propiconazole or pyraclostrobin sensitivity of the Ptt and Ptm isolates from Alberta, Saskatchewan and Manitoba. Recently, Akhavan et al. (Citation2016b) reported that Ptt and Ptm isolates from these provinces are genetically similar, with a large fraction of the genetic differentiation occurring within as opposed to between provinces.
Campbell & Crous (Citation2002) found that Ptm isolates were less sensitive to propiconazole, tebuconazole, triadimenol, bromuconazole and flusilazole than were Ptt isolates, and concluded that Ptm may have developed more insensitivity as a result of a different evolutionary path. Scott et al. (Citation1992) reported that two applications of propiconazole were required to reliably control SFNB caused by Ptm, while only one application was sufficient for control of NFNB caused by Ptt. Similar, but weaker trends (P < 0.10) were found in the current study for propiconazole or pyraclostrobin sensitivity, where slightly higher EC50 values were observed for Ptm versus Ptt.
Propiconazole has been used for the control of diseases of cereals and other crops in western Canada since the early 1990s (Xue et al. Citation1994; Thomas Citation1997), while pyraclostrobin has been applied commercially to cereals only since the early 2000s (BASF Citation2003; Government of Canada: Health Canada Pest Management Regulatory Agency Citation2011). In western Canada, barley stubble probably contains both the sexual and asexual stages of the net blotch pathogens, and with fungicide use by barley farmers, fungal populations are frequently exposed to these products. Therefore, the routine application of these site-specific compounds on the genetically diverse populations of Ptt and Ptm poses a high risk for the development of insensitivity to both fungicides, particularly since the sensitivity to fungicides such as propiconazole has a high heritability rate (Talas & McDonald Citation2015). Moreover, no fitness penalty was observed for mutant Ptt isolates with increased insensitivity to strobilurins (Marzani Citation2011). Campbell & Crous (Citation2002) also suggested that sexual recombination could increase the rate of insensitivity of Ptm isolates toward DMI fungicides in South Africa. If the pathogen develops insensitivity, then with many asexual cycles during the following growing season, the insensitive individual may multiply and constitute a significant portion of a whole new emerging P. teres population. The high gene flow reported among the provincial populations of both forms of the net blotch pathogen in western Canada (Akhavan et al. Citation2016b) suggests there is a risk of the transfer of fungicide insensitivity from one population to another. In Europe, it was shown that the frequency of M. graminicola haplotypes with insensitivity to QoI fungicides (due to the G143A mutation) increased rapidly via strong fungicide selection pressure, and that the pathogen then spread in a west-to-east direction via wind dispersal of ascospores (Torriani et al. Citation2009).
The identification of Ptt and Ptm isolates insensitive to propiconazole, and of a Ptm isolate with increased insensitivity to both propiconazole and pyraclostrobin, suggest the need for increased fungicide stewardship in western Canada. Farmers should avoid relying exclusively on a single or the same few fungicide active ingredients, and employ other strategies to reduce the risk of fungicide insensitivity developing in Ptt and Ptm populations. Fungicide mixtures, comprising QoIs and DMIs or the novel SDHI formulations, were found to have great efficacy for net blotch disease management (Marzani Citation2011). Ideally, a broad combination of control methods, in addition to the application of fungicides, should be used to manage net blotch of barley.
In the current study, we assessed the sensitivity of Ptt and Ptm for the DMI fungicide propiconazole and the QoI fungicide pyraclostrobin. Our research mainly focused on detecting insensitivity and comparing differences between the two forms of the pathogen. Given the occurrence of insensitive isolates, our results underscore the need for continued monitoring of the fungicide sensitivity of P. teres populations in western Canada. In addition, future studies are needed to investigate the genetic mechanisms underlying these insensitivities, and to also evaluate the relative fitness of insensitive isolates; this research will help with the early detection of fungicide insensitivity, and allow for more extensive evaluation of insensitivity development in western Canadian populations of Ptt and Ptm.
Supplemental meterial
Supplemental data for this article can be accessed online here: http://dx.doi.org/10.1080/07060661.2017.1282541.
Supplemental Table 1
Download MS Word (14.2 KB)Acknowledgements
The authors would like to thank Dr Andy Tekauz, Mr James Tucker, Ms Colleen Kirkham, Mr Meconnen Beyene, Ms Noryne Rauhala, Ms Jackie Busaan (Agriculture and Agri-Food Canada), Dr Kequan Xi, and Dr Krishan Kumar (Alberta Agriculture and Forestry) for assistance with the collection of isolates, and Dr Tiesen Cao, and Dr Urmila Basu (University of Alberta) for technical advice and insight. Finally, the authors thank the Western Grains Research Foundation, Alberta Barley Commission, Rahr Malting Inc., Canadian Wheat Board, Agriculture and Agri-Food Canada, and the University of Alberta for financial support of this research.
Additional information
Funding
References
- Aboukhaddour R, Turkington TK, Strelkov SE. 2013. Race structure of Pyrenophora triciti-repentis (tan spot of wheat) in Alberta, Canada. Can J Plant Pathol. 35:256–268.
- Akhavan A, Turkington TK, Askarian H, Tekauz A, Xi K, Tucker JR, Kutcher HR, Strelkov SE. 2016a. Virulence of Pyrenophora teres populations in western Canada. Can J Plant Pathol. 38:183–196.
- Akhavan A, Turkington TK, Kebede B, Tekauz A, Kutcher HR, Kirkham C, Xi K, Kumar K, Tucker JR, Strelkov SE. 2015. Prevalence of mating type idiomorphs in Pyrenophora teres f. teres and P. teres f. maculata populations from the Canadian prairies. Can J Plant Pathol. 37:52–60.
- Akhavan A, Turkington TK, Kebede B, Xi K, Kumar K, Tekauz A, Kutcher HR, Tucker JR, Strelkov SE. 2016b. Genetic structure of Pyrenophora teres f. teres and P. teres f. maculata populations from western Canada. Eur J Plant Pathol. 146:325–335.
- Alberta Government. 2016a. Varieties of cereal and oilseed crops for Alberta – 2016. Government of Alberta, agriculture and forestry. [ cited 2016 Jun 01]. Available from: http://www1.agric.gov.ab.ca/$department/deptdocs.nsf/all/agdex4069
- Alberta Government. 2016b. Crop protection 2016, Government of Alberta, agriculture and forestry. [ cited 2016 May 01]. Available from: http://www1.agric.gov.ab.ca/$department/deptdocs.nsf/all/agdex32/$file/606-1.pdf?OpenElement
- Bartlett DW, Clough JM, Godwin JR, Hall AA, Hamer M, Parr‐Dobrzanski B. 2002. The strobilurin fungicides. Pest Manag Sci. 58:649–662.
- BASF. 2003. Headline fungicide label. [Online] [ cited 2015 Jan 19]. Available from: https://agro.basf.ca/West/Products/Product_Labels/Headline.pdf
- Bean TP, Cools HJ, Lucas JA, Hawkins ND, Ward JL, Shaw MW, Fraaije BA. 2009. Sterol content analysis suggests altered eburicol 14α-demethylase (CYP51) activity in isolates of Mycosphaerella graminicola adapted to azole fungicides. FEMS Microbiol Lett. 296:266–273.
- Bowness R, Gossen BD, Chang K-F, Goswani R, Willenborg CJ, Holtz M, Strelkov SE. 2016. Sensitivity of Mycosphaerella pinodes to pyraclostrobin fungicide. Plant Dis. 100:192–199.
- Campbell GF, Crous PW. 2002. Fungicide sensitivity of South African net- and spot-type isolates of Pyrenophora teres to ergosterol biosynthesis inhibitors. Australas Plant Pathol. 31:151–155.
- Chang KF, Ahmed HU, Hwang SF, Gossen BD, Strelkov SE, Blade SF, Turnbull GD. 2007. Sensitivity of field populations of Ascochyta rabiei to chlorothalonil, mancozeb and pyraclostrobin fungicides and effect of strobilurin fungicides on the progress of ascochyta blight of chickpea. Can J Plant Sci. 87:937–944.
- Cools HJ, Fraaije BA. 2013. Update on mechanisms of azole resistance in Mycosphaerella graminicola and implications for future control. Pest Manag Sci. 69:150–155.
- Dahl C, Biemann HP, Dahl J. 1987. A protein kinase antigenically related to pp60v-src possibly involved in yeast cell cycle control: positive in vivo regulation by sterol. Proc Natl Acad Sci USA. 84:4012–4016.
- Délye C, Bousset L, Corio-Costet MF. 1998. PCR cloning and detection of point mutations in the eburicol 14a-demethylase (CYP51) gene from Erysiphe graminis f. sp. hordei a recalcitrant fungus. Curr Genet. 34:399–403.
- Duczek LJ, Sutherland KA, Reed SL, Bailey KL, Lafond GP. 1999. Survival of leaf spot pathogens on crop residues of wheat and barley in Saskatchewan. Can J Plant Pathol. 21:165–173.
- Finney DJ. 1971. Probit analysis. 3rd ed. Cambridge (UK): Cambridge University Press.
- FRAC. 2006a. PYRNTE monitoring method Syngenta 2006 V1. Fungicide Resistance Action Committee. Online publication. [Online] [ cited 2016 Oct 06]. Available from: http://www.frac.info/docs/default-source/monitoring-methods/approved-methods/pyrnte-monitoring-method-syngenta-2006-v1.pdf?sfvrsn=4
- FRAC. 2006b. PYRNTR monitoring method Syngenta 2006 V1. Fungicide Resistance Action Committee. Online publication. [Online] [ cited 2016 Oct 06]. Available from: http://www.frac.info/docs/default-source/monitoring-methods/approved-methods/pyrntr-monitoring-method-syngenta-2006-v1.pdf?sfvrsn=4
- FRAC. 2009. PYRNTE monitoring methods update Feb 2009. Fungicide Resistance Action Committee. Online publication. [Online] [ cited 2016 Oct 06]. Available from: http://www.frac.info/docs/default-source/monitoring-methods/approved-methods/prynte-monitoring-methods-update-feb-2009.pdf?sfvrsn=4
- FRAC. 2016. FRAC Code List 2016: Fungicides sorted by mode of action. Fungicide Resistance Action Committee. Online publication. [Online] [ cited 2016 May 15]. Available from: http://www.frac.info/docs/default-source/publications/frac-code-list/frac-code-list-2016.pdf?sfvrsn=2
- Fraser M, Hwang SF, Ahmed HU, Akhavan A, Stammler G, Barton W, Strelkov SE. 2016. Sensitivity of Leptosphaeria maculans to pyraclostrobin in Alberta, Canada. Can J Plant Sci. doi:10.1139/CJPS-2015-0382)
- Gisi U, Chin KM, Knapova G, Farber R, Kung R, Mohr U, Parisi S, Sierotzki H, Steinfeld U. 2000. Recent developments in elucidating modes of resistance to phenylamide, DMI and strobilurin fungicides. Crop Prot. 19:863–872.
- Gossen BD, Anderson KL. 2004. First report of resistance to strobilurin fungicides in Didymella rabiei. Can J Plant Pathol. 26:411.
- Government of Canada: Health Canada Pest Management Regulatory Agency. 2011. ARCHIVED - Pyraclostrobin seed treatment - proposed registration decision PRD2011-15 - health Canada consultation document. [Online] [ cited 2016 May 15]. Available from: http://www.hc-sc.gc.ca/cps-spc/pest/part/consultations/_prd2011-15/prd2011-15-eng.php
- Grasso V, Palermo S, Sierotzki H, Garibaldi A, Gisi U. 2006. Cytochrome b gene structure and consequences for resistance to Qo inhibitor fungicides in plant pathogens. Pest Manage Sci. 62:465–472.
- Ho KM, Tekauz A, Choo TM, Martin RA. 1996. Genetic studies on net blotch resistance in a barley cross. Can J Plant Sci. 76:715–720.
- Jayasena KW, Van Burgel A, Tanaka K, Majewski J, Loughman R. 2007. Yield reduction in barley in relation to spot-type net blotch. Australas Plant Pathol. 36:429–433.
- Kuck KH, Leadbeater A, Gisi U. 2012. FRAC: Mode of action classification and resistance risk. In: Kraemer W, Schirmer U, Jeschke P, Witschel M, editors. Modern crop protection compounds. 2nd ed. Berlin: Wiley-VCH Verlag GmbH & Co. KGaA; p. 539–557.
- Lamari L, Bernier CC. 1989. Evaluation of wheat lines and cultivars to tan spot [Pyrenophora tritici-repentis] based on lesion type. Can J Plant Pathol. 11:49–56.
- Leroux P, Albertini C, Gautier A, Gredt M, Walker AS. 2007. Mutations in theCYP51 gene correlated with changes in sensitivity to sterol 14α-demethylation inhibitors in field isolates of Mycosphaerella graminicola. Pest Manage Sci. 63:688–698.
- Liu Z, Ellwood SR, Oliver RP, Friesen TL. 2011. Pyrenophora teres: Profile of an increasingly damaging barley pathogen. Mol Plant Pathol. 121:1–19.
- Mair WJ, Deng W, Mullins JG, West S, Wang P, Besharat N, Ellwood SR, Oliver RP, Lopez-Ruiz FJ. 2016. Demethylase inhibitor fungicide resistance in Pyrenophora teres f. sp. teres associated with target site modification and inducible overexpression of Cyp51. Front Microbiol. 7:1279.
- Marzani QA. 2011. Fungicide resistance and efficacy for control of Pyrenophora teres and Mycosphaerella graminicola on barley and wheat. [ Doctoral dissertation]. University of Nottingham, Nottingham, UK.
- Marzani QA, Swarbrick P, Rossall S. 2013. Correlation of the F129L mutation in Pyrenophora teres, the pathogen of net blotch of barley, with the efficacy of QoI fungicides. IOSR-JAVS. 3:66–72.
- Mathre DE. 1997. Compendium of barley diseases. 2nd ed. St. Paul (MN): APS Press; p. 90.
- McDonald B, Linde C. 2002. Pathogen population genetics, evolutionary potential, and durable resistance. Annu Rev Phytopathol. 40:349–379.
- McDonald WC. 1963. Heterothallism in Pyrenophora teres. Phytopathology. 53:771–773.
- Metcalfe DR, Buchannon KW, McDonald WC, Reinbergs E. 1970. Relationships between the ‘Jet’ and ‘Milton’ genes for resistance to loose smut and genes for resistance to other barley diseases. Can J Plant Sci. 50:423427.
- Miguez M, Reeve C, Wood PM, Hollomon DW. 2004. Alternative oxidase reduces the sensitivity of Mycosphaerella graminicola to QoI fungicides. Pest Manage Sci. 60:3–7.
- Mondal SN, Bhatia A, Shilts T, Timmer LW. 2005. Baseline sensitivities of fungal pathogens of fruit and foliage of citrus to azoxystrobin, pyraclostrobin, and fenbuconazole. Plant Dis. 89:1186–1194.
- Murray GM, Brennan JP. 2010. Estimating disease losses to the Australian barley industry. Australas Plant Pathol. 39:85–96.
- Parker JE, Warrilow AG, Price CL, Mullins JG, Kelly DE, Kelly SL. 2014. Resistance to antifungals that target CYP51. J Biol Chem. 7:143–161.
- Peever TL, Milgroom MG. 1992. Inheritance of triadimenol resistance in Pyrenophora teres. Phytopathology. 82:821–828.
- Peever TL, Milgroom MG. 1993. Genetic correlations in resistance to sterol biosynthesis-inhibiting fungicides in Pyrenophora teres. Phytopathology. 83:1076–1082.
- Peever TL, Milgroom MG. 1994. Genetic structure of Pyrenophora teres populations determined with random amplified polymorphic DNA markers. Can J Bot. 72:915–923.
- Piening L. 1961. The occurrence of Pyrenophora teres on barley straw in Alberta. Can Plant Dis Surv. 41:299–300.
- Piening L. 1968. Development of barley net blotch from infested straw and seed. Can J Plant Sci. 48:623–625.
- Pijls CFN, Shaw MW, Parker A. 1994. A rapid test to evaluate in vitro sensitivity of Septoria tritici to flutriafol, using a microtitre plate reader. Plant Pathol. 43:726–732.
- Poole NF, Arnaudin ME. 2014. The role of fungicides for effective disease management in cereal crops. Can J Plant Pathol. 36:1–11.
- Rallos LEE, Baudoin AB. 2016. Co-occurrence of two allelic variants of CYP51 in Erysiphe necator and their correlation with over-expression for DMI resistance. Plos One. 11:e0148025.
- Romero RA, Sutton TB. 1997. Sensitivity of Mycosphaerella fijiensis, causal agent of black Sigatoka of banana, to propiconazole. Phytopathology. 87:96–100.
- Sauter H, Steglich W, Anke T. 1999. Strobilurins: Evolution of a new class of active substances. Angew Chem Int Ed. 38:1328–1349.
- Scott DB, van Niekerk HA, Paxton TG. 1992. Effect of propiconazole on necrotrophic fungi and yield of barley genotypes differing in susceptibility to Rhyncosporium secalis. Crop Prot. 11:243–247.
- Semar M, Strobel D, Koch A, Klappach K, Stammler G. 2007. Field efficacy of pyraclostrobin against populations of Pyrenophora teres containing the F129L mutation in the cytochrome b gene. J Plant Dis Prot. 114:117–119.
- Sierotzki H, Frey R, Wullschleger J, Palermo S, Karlin S, Godwin J, Gisi U. 2007. Cytochrome b gene sequence and structure of Pyrenophora teres and P. tritici-repentis and implications for QoI resistance. Pest Manage Sci. 63:225–233.
- Smedegard-Petersen V. 1978. Genetics of heterothallism in Pyrenophora graminea and P. teres. Trans Br Mycol Soc. 70:99–102.
- Smith FD, Parker DM, Köller W. 1991. Sensitivity distribution of Venturia inaequalis to the sterol demethylation inhibitor flusilazole: Baseline sensitivity and implications for resistance monitoring. Phytopathology. 81:392–396.
- Stammler G, Taher K, Koch A, Haber J, Liebmann B, Bouagila A, Yahyaoui A, Nasraoui B. 2012. Sensitivity of Mycosphaerella graminicola isolates from Tunisia to epoxiconazole and pyraclostrobin. Crop Prot. 34:32–36.
- Statistics Canada. 2016. Table 001-0010 - Estimated areas, yield, production and average farm price of principal field crops, in metric units, annual, CANSIM (database). [ cited 2016 Oct 11]. Available from: http://www5.statcan.gc.ca/cansim/a26?lang=eng&id=10010#F2
- Steffenson BJ. 1997. Net blotch. In: Mather DE, editor. Compendium of barley diseases. 2nd ed. St. Paul (MN): American Phytopathological Society; p. 28–31.
- Steffenson BJ, Webster RK, Jackson LF. 1991. Reduction in yield loss using incomplete resistance to Pyrenophora teres f. teres in barley. Plant Dis. 75:96–100.
- Talas F, McDonald BA. 2015. Significant variation in sensitivity to a DMI fungicide in field populations of Fusarium graminearum. Plant Pathol. 64:664–670.
- Tekauz A. 1990. Characterization and distribution of pathogenic variation in Pyrenophora teres f. teres and P. teres f. maculata from Western Canada. Can J Plant Pathol. 12:141–148.
- Tekauz A. 2003. Diseases of barley. In Bailey KL, Gossen BD, Gugel RK, Morrall RAA, editors. Diseases of field crops in Canada. 3rd ed. Saskatoon (SK): Canadian Phytopathological Society; p. 30–53.
- Tekauz A, Mills JT. 1974. New types of virulence in Pyrenophora teres in Canada. Can J Plant Sci. 54:731–734.
- Thaher N. 2011. Fungicide insensitivity in Ascochyta rabiei in Saskatchewan. [ M.Sc. thesis]. Guelph: University of Guelph.
- Thomas P. 1997. Canola growers’ manual. Winnipeg: Canola Council of Canada.
- Torriani SF, Brunner PC, McDonald BA, Sierotzki H. 2009. QoI resistance emerged independently at least 4 times in European populations of Mycosphaerella graminicola. Pest Manage Sci. 65:155–162.
- Tremblay DM, Talbot BG, Carisse O. 2003. Sensitivity of Botrytis squamosa to different classes of fungicides. Plant Dis. 87:573–578.
- Turkington TK, Kutcher HR, Clayton GW, O’Donovan JT, Johnston AM, Harker KN, Xi K, Stevenson FC. 2004. Impact of seedbed utilization and fungicide application on severity of net blotch [Pyrenophora teres] and production of barley. Can J Plant Pathol. 26:533–547.
- Turkington TK, O’Donovan JT, Edney MJ, Juskiw PE, McKenzie RH, Harker KN, Clayton GW, Xi K, Lafond GP, Irvine RB, Brandt S. 2012. Effect of crop residue, nitrogen rate and fungicide application on malting barley productivity, quality, and foliar disease severity. Can J Plant Sci. 92:577–588.
- Turkington TK, O’Donovan JT, Harker KN, Xi K, Blackshaw RE, Johnson EN, Peng G, Kutcher HR, May WE, Lafond GP, et al. 2015. The impact of fungicide and herbicide timing on foliar disease severity, and barley productivity and quality. Can J Plant Sci. 95:525–537.
- Turkington TK, Tekauz A, Xi K, Kutcher HR. 2011. Foliar diseases of barley: Don’t rely on a single strategy from the disease management toolbox. Prairie Soils Crops. 4:142–150.
- Turkington TK, Xi K, Clayton GW, Burnett PA, Klein-Gebbinck H, Lupwayi NZ, Harker KN, O’Donovan JT. 2006. Impact of crop management on leaf diseases in Alberta barley fields, 1995-1997. Can J Plant Pathol. 28:441–449.
- Turkington TK, Xi K, Tewari JP, Lee HK, Clayton GW, Harker KN. 2005. Cultivar rotation as a strategy to reduce leaf diseases under barley monoculture. Can J Plant Pathol. 27:283–290.
- van den Berg CGJ, Rossnagel BG. 1991. Epidemiology of spot-type net blotch on spring barley in Saskatchewan. Phytopathology. 81:1446–1452.
- Villani SM, Cox KD. 2011. Characterizing fenbuconazole and propiconazole sensitivity and prevalence of ‘Mona’ in isolates of Monilinia fructicola from New York. Plant Dis. 95:828–834.
- Wise KA, Bradley CA, Pasche JS, Gudmestad NC. 2009. Resistance to QoI fungicides in Ascochyta rabiei from chickpea in the northern great plains. Plant Dis. 93:528–536.
- Wise KA, Bradley CA, Pasche JS, Gudmestad NC, Dugan FM, Chen W. 2008. Baseline sensitivity of Ascochyta rabiei to azoxystrobin, pyraclostrobin and boscalid. Plant Dis. 92:295–300.
- Wood PM, Hollomon DW. 2003. A critical evaluation of the role of alternative oxidase in the performance of strobilurin and related fungicides acting at the Qo site of complex III. Pest Manage Sci. 59:499–511.
- Xue G, Burnett PA, Helm J. 1994. Severity of, and resistance of barley varieties to, scald and net blotch in central Alberta. Can Plant Dis Survey. 74:13–17.