Abstract
Zucchini yellow mosaic virus (ZYMV) causes one of the most destructive diseases of cucurbits worldwide. Although the virus has been present in China for more than 25 years, there is little information about biological and molecular traits of ZYMV isolates from China. This study aimed to characterize 26 ZYMV isolates from Xinjiang, China based on pathotypes, molecular variability and host range. Phylogenetic analysis of the complete sequences of the coat protein gene revealed three distinct groups (A, B and C), with four subgroups in A (I–IV). All isolates from Xinjiang were placed in group C and subgroups A–I and A–II. To test for differences in host range among different phylogenetic groups from Xinjiang, 13 isolates were mechanically inoculated onto 14 plant species in five families and their pathotypes were also identified based on the reaction of a resistant melon accession PI414723. Overall, the results showed that there were no correlations of host responses to inoculation with ZYMV isolates from different phylogenetic groups. However, on Cucurbita pepo, differences in host responses to inoculation with the ZYMV isolates from different phylogenetic groups were observed. Although the host range of the 13 isolates tested was similar, their biological properties on different hosts were slightly different from the ZYMV isolates characterized in other countries. The results of the pathotype test showed that the 13 ZYMV isolates were classified as pathotype 0. The information obtained is important in breeding for resistant varieties.
Résumé
Le virus de la mosaïque jaune de la courgette (VMJC) cause une des maladies les plus destructrices chez les cucurbitacées, et ce, à l’échelle de la planète. Bien que le VMJC soit apparu en Chine il y a plus de 25 ans, nous possédons peud’information sur les caractères biologiques et moléculaires de sesisolats. Cette étude visait à caractériser 26 isolats de VMJC provenant de la province du Xinjiang, en Chine, en se basantsur les pathotypes, la variabilité moléculaire et la gamme de plantes hôtes. L’analyse phylogénétique des séquences complètes du gène de la protéine capsidiaire a révélé trois groupes (A, B et C), dont quatre sous-groupes chez A (I–IV). Tous les isolats du Xinjiang ont été placés dans le groupe C et les sous-groupes A–I et A–II. Afin de tester les différences quant à la gamme de plantes hôtes parmi divers groupes phylogénétiques provenant du Xinjiang, 14 espèces de plantes de 5 familles ont été inoculées mécaniquement avec 13 isolats, et leurs pathotypes ont également été identifiés en se basant sur la réaction de l’obtention PI414723 d’un melon résistant. En général, les résultats ont montré qu’il n’y a pas de corrélation entre les réactions de l’hôte et l’inoculation avec les isolats de VMJC provenant de différents groupes phylogénétiques. Toutefois, nous avons observé, sur Cucurbitapepo, des différences quant à la réaction de l’hôte à l’inoculation avec des isolats de VMJC provenant de différents groupes phylogénétiques. Bien que la gamme de plantes hôtes des 13 isolats testés fût similaire, leurs propriétés biologiques quant à divers hôtes différaient légèrement des isolats de VMJC caractérisés dans d’autres pays. Les résultats des tests effectués sur les pathotypes ont montré que les 13 isolats de VMJC étaient classifiés en tant que pathotype 0. L’information obtenue est importante pour la sélection de variétés résistantes.
Introduction
Zucchini yellow mosaic virus (ZYMV) belongs to the genus Potyvirus in the family Potyviridae (Coutts et al. Citation2011) and was first described in Italy in 1973 (Lisa et al. Citation1981). Within a decade, the virus had spread worldwide, causing significant economic and yield losses of cucurbit crops (Desbiez & Lecoq Citation1997). ZYMV is transmitted non-persistently by a number of aphid species (Katis et al. Citation2006; Simmons et al. Citation2008). Seed transmission occurs occasionally at very low rates in some cucurbit crops (Bananej et al. Citation2008; Coutts et al. Citation2011).
ZYMV has a genome of approximately 9.6 kb in size that encodes a polyprotein precursor that is subsequently processed into 10 putative proteins (Gal-On Citation2007). Of these, the coat protein (CP) is encoded at the C-terminus of the polyprotein precursor and the CP gene is extensively used to differentiate among isolates and infer phylogenetic relationships among strains (Shukla et al. Citation1991; Rybicki & Shukla Citation1992; Urcuqui-Inchima et al. Citation2001). Strains of ZYMV differing in biological properties have been described and serological variability of the CP was established (Desbiez et al. Citation2002; Zhao et al. Citation2003; Glasa & Pittnerova Citation2006; Bananej et al. Citation2008; Coutts et al. Citation2011). In addition, previous studies used nucleotide sequences of the CP gene to distinguish phylogenetic groups (Desbiez et al. Citation2002; Zhao et al. Citation2003; Ha et al. Citation2008; Vučurović et al. Citation2012; Romay et al. Citation2014). These phylogenetic analyses revealed two or three major groups of ZYMV isolates with different geographic distributions.
Melon (Cucumis melo L.) is one of the most important horticultural crops worldwide and belongs to the Cucurbitaceae family (Mascarell-Creus et al. Citation2009). China is the largest melon-producing country in the world (FAOSTAT Citation2014). The Xinjiang Uygur autonomous region which covers an area of 1.66-million km2 is located in the north-west region of China (Rui et al. Citation2002). Melon is one of the most economically important crops in Xinjiang and the region is currently the largest area for melon production in China (Anonymous Citation2015). ZYMV was first reported in Xinjiang in 1989 and this was also the first report of ZYMV in China (Zheng & Dong Citation1989). Although the virus has been present in China for over 25 years and is associated with significant economic and yield losses of cucurbit crops, little is known about its biological and molecular traits, especially in the north-west region of China. Such information is critical for the development of virus-resistant melon cultivars and the understanding of the complexity and epidemiology of the pathogen. Hence, this study aimed to characterize biological and molecular variability of 26 ZYMV isolates infecting melon from multiple locations in Xinjiang of China based on host range, pathotype and sequence variability of the CP region.
Materials and methods
Field sampling
During the 2013 and 2014 growing seasons, a survey was conducted of melon plants growing in 13 major melon-growing locations in Xinjiang (see ). In each location, three fields were randomly selected and inspected for each of the major melon cultivars. Young leaves showing virus-like symptoms were randomly collected throughout each field. Most samples were taken from commonly grown cultivars including ‘Xizhoumi’, ‘Queen’, ‘Jiashi’, ‘Jinlong’, ‘Huangdanzi’, ‘Bailan’ and ‘Laohan’. A total of 492 samples were collected and placed in a cooler box with ice for transportation to the laboratory within 24 h and then stored at −70°C until extraction of nucleic acids was conducted.
Fig. 1 Locations in Xinjiang where Zucchini yellow mosaic virus isolates were collected. The 13 locations are Changji city (CJ), Shihezi city (SHZ), Wujiaqu 102-regiment (WQ-102), Lukeqin township in Shanshan county (LKQ), Tuyugou township in Shanshan county (TYG), Pizhan township in Shanshan county (PZ), Qiketai township in Shanshan county (QKT), Toksun county (TKS), Turpan city (TP), Jiashi county (JS), Fukang city (FK), Nanhu township in Hami city (NH), and Huayuan township in Hami city (HY).
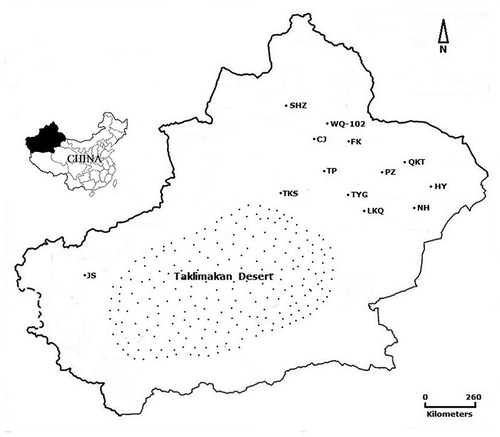
RT-PCR detection
Total RNA was extracted from each leaf sample using GeneJETTM Plant RNA Purification Mini Kit (Fermentas, Vilnius, Lithuania) according to the manufacturer’s instructions. A two-step reverse transcription-polymerase chain reaction (RT-PCR) protocol was used to detect ZYMV in the melon samples. Specific PCR primer pairs CPfwd (5′GCTCCATACATAGCTGAGAC3′) and CPrev (5′AACGGAGTCTAATCTCGAGC3′) were adopted from a previous publication by Pfosser & Baumann (Citation2002) for amplification of the ZYMV CP gene. The reverse primer CPrev was also used as the primer for synthesis of the first-strand complementary DNA (cDNA).
First-strand cDNA was synthesized from total RNA using Moloney murine leukemia virus (M-MLV) reverse transcriptase RNaseH Minus (TaKaRa, Dalian, China) according to the manufacturer’s instructions. The 10 μL reaction volume contained 5 μL of total RNA, 0.5 μL of 200 U μL−1 M-MLV reverse transcriptase RNaseH Minus, 2 μL of 5×RT-buffer, 0.25 μL of 40 U μL−1 RNase inhibitor (TaKaRa), 1 μL of 10 μM reverse primer CPrev, 0.5 μL of 10 mM dNTP mix, and 0.75 μL of RNase free ddH2O (diethylpyrocarbonate-treated water). The reverse transcription was undertaken for 60 min at 42°C in a TProfessional Thermocycler (Biometra, Analytik Jena, Germany). The resulting cDNA was used as templates for PCR using the proofreading Ex Taq DNA Polymerase (TaKaRa) and primer pairs CPrev and CPfwd. The PCR reaction (50 μL) consisted of 5 μL of 10×Ex Taq buffer containing Mg2+, 0.5 μL of 5 U μL−1 of the proofreading Ex Taq DNA Polymerase (TaKaRa), 2 μL of 2.5 mM dNTP mix, 1 μL each of 10 μM forward (CPfwd) and reverse (CPrev) primers, 3 μL of cDNA template, and 37.5 μL of RNase free ddH2O. The PCR was followed by an initial denaturing step of 3 min at 94°C and 35 cycles of 25 s at 94°C, 25 s at 55°C and 70 s at 72°C, and a final extension step at 72°C for 10 min. PCR was carried out in a TProfessional Thermocycler. PCR amplification products were visualized under a UV transilluminator (Bio-Rad, USA) after electrophoresis on 1% (w/v) agarose gel containing ethidium bromide.
Molecular cloning and sequencing
From melon samples found infected with ZYMV by RT-PCR, 26 were chosen to represent different geographic origins and melon cultivars, and the complete CP nucleotide sequences of all 26 isolates were amplified by RT-PCR as described above (). Amplified products of a predicted size were gel purified using the DNA Gel Extraction Kit (Sangon Biotech, Shanghai, China) and then cloned in a pMD18-T vector (TaKaRa) according to the manufacturer’s instructions, followed by the transformation of competent Escherichia coli strain DH5α. Potential recombinant clones were identified using PCR. The sequencing was performed by BGI (Beijing, China). In order to exclude in vitro RT-PCR errors, at least three independent clones of each isolate were sequenced in both directions, and a consensus sequence was derived when the three independent clones showed ≥RT-PCR errors, aThis consensus was termed ‘unique sequence’. Moreover, additional clones were sequenced to investigate the possible occurrence of mixtures of distinct sequence variants within individual samples if the isolate showed <99.5% nucleotide sequence identity between the three initially sequenced clones.
Table 1. Zucchini yellow mosaic virus isolates used in this study.
Sequence analysis
Database homology searches were performed using BLAST (http://www.ncbi.nlm.nih.gov/blast). Multiple sequence alignment was carried out using ClustalX 2.0 (Larkin et al. Citation2007) and sequence identity was calculated using MegAlign program by the DNASTAR Lasergene package (Madison, WI, USA). The MODELTEST implemented in MEGA5 (Tamura et al. Citation2011) was used for determining the best-fitting model of nucleotide substitution. The Tamura 3-parameter model (T92 + G) was chosen and this was used to infer their phylogenetic relationships and assess average genetic distances among ZYMV isolates. Phylogenetic trees were constructed by neighbour-joining (NJ) method using MEGA 5 (bootstrap analysis with 1000 replicates). The ratio (ω) of non-synonymous (dN) to synonymous (dS) mutation rates was calculated using the Pamilo-Bianchi-Li method (Li Citation1993; Pamilo & Bianchi Citation1993) implemented in MEGA 5.
Virus isolates and biological cloning
From the 26 melon samples (), 13 isolates were chosen according to geographic origin, and these isolates were transmitted by three local lesion passages in Chenopodium quinoa. Isolates were then maintained in courgette (Cucurbita pepo) by mechanical transmission. After multiplication in courgette plants, the isolates were confirmed by RT-PCR as described above. Such biologically cloned isolates were used for biological and molecular characterization. The isolates were named according to their geographic origins ().
Table 2. Reaction of host plants to Zucchini yellow mosaic virus isolates from Xinjiang and confirmation of infection by RT-PCR.
Bioassay and host range test
The 13 ZYMV isolates () were characterized biologically by reaction to inoculation of a variety of hosts. Fourteen different plant species including melon (Cucumis melo L.) (cultivars PI414723 and ‘Jiashi’), cucumber (Cucumis sativus L.), pumpkin (Cucurbita moschata Duch.), courgette (Cucurbita pepo L.), towel gourd (Luffa cylindrica (L.) Roem.), thorn apple (Datura stramonium L.), goosefoot (Chenopodium amaranticolor Coste & Reyn.), quinoa (Chenopodium quinoa Willd.), globe amaranth (Gomphrena globosa L.), cowpea (Vigna unguiculata (Linn.)Walp), kidney bean (Phaseolus vulgaris L.), broad bean (Vicia faba L.), pea (Pisum sativum L.), and tobacco (Nicotiana tabacum L.) were selected for host range tests by mechanical inoculation. All plants were at the four leaf stage of development. Infected courgette leaves were ground in 10 mM sodium phosphate buffer (pH 7.0) containing 10 mM sodium sulphite at a ratio of 1:10 (w/v), and the saps were rubbed on the tested plant leaf surfaces that were dusted with carborundum. Healthy control plants were inoculated with buffer only. Inoculated plants were maintained in an insect-proof, air-conditioned glasshouse at 25–28°C and observed daily for 21 days. Infections were confirmed at 3 weeks post-inoculation (wpi) by RT-PCR as described above. For pathotype test, Cucumis melo accession PI414723 was mechanically inoculated with selected ZYMV isolates (Pitrat & Lecoq Citation1984). Viral infections were assessed through symptom evaluation and by RT-PCR. Bioassay and host range tests were repeated twice with at least three plants for each inoculation.
Results
Field sampling
The survey results showed that ZYMV was detected in nine of 13 locations. The following locations – FK, HY, NH and PZ – were PCR-negative based on sample numbers of 5, 69, 36 and 37, respectively. Among the melon cultivars sampled, no PCR positive reaction was detected from cultivars ‘Jinlong’ and ‘Queen’. The total number of samples tested by RT-PCR was 91 and 51 for cultivars ‘Queen’ and ‘Jinlong’, respectively. The cultivar ‘Queen’ was planted in locations HY, NH and PZ. Moreover, the cultivar ‘Jinlong’ was planted in locations HY and NH.
Molecular variability
The results of coat protein gene sequences of 26 ZYMV isolates indicated that the initial three clones for each isolate were ≥99.5% identical and, thus, were considered to represent a single sequence. The sequences of these isolates were deposited in GenBank (). The identity of the complete CP nucleotide sequences of the 26 ZYMV isolates ranged from 81.6 to 100% (87.8 to 100% amino acid identities) (Supplemental table S1). Multiple alignments revealed that a highly conserved amino acid triplet Asp-Ala-Gly (DAG) which was essential for potyvirus transmission by aphids (Atreya et al. Citation1990) was found at the N-terminus of the CP amino acid sequences of the 26 ZYMV isolates. The mean genetic distance within the isolates was 0.2306 ± 0.0387. The direction and strength of the selection pressure acting on coding regions within ZYMV populations were also assayed. We estimated the relative rates of change at non-synonymous (dN) and synonymous positions (dS) by ω = dN/dS. The dN/dS ratio for the 26 ZYMV isolates was 0.0857. The dN/dS ratios<1 showed that purifying selection was restricting variability in the population.
A neighbour-joining tree was constructed using the complete CP nucleotide sequences of the 26 ZYMV isolates with representative sequences present in GenBank (). The tree comprised three groups (A–C) and group A consisted of four subgroups (I–IV). Isolates from locations TP, TYG, WJQ-102, TKS, SHZ and CJ were all in group A which is apparently the most widespread worldwide. All isolates from location JS were in group C, and this group contained no other ZYMV isolates from Xinjiang. Moreover, all isolates from location TP were in subgroup A-I and isolates from locations TYG, WJQ-102, TKS, SHZ, and CJ were in subgroup A-II. Sequence analysis indicated that the nine ZYMV isolates from location JS shared 99.8–100% nucleotide homology with each other, but they showed 81.6–82.3% identity with other isolates from Xinjiang (Supplemental table S1).
Fig. 2 Neighbour-joining tree depicting the phylogenetic relationships between 26 ZYMV isolates from Xinjiang and 70 published complete coat protein (CP) nucleotide sequences. The name of isolates obtained in this study is shown in bold letters. Bootstrap values (1000 replicates) above 50% are shown.
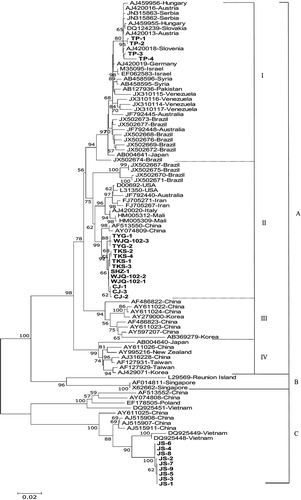
Biological variability
The 13 ZYMV isolates from different phylogenetic groups () were mechanically inoculated onto a range of 14 plant species belonging to Cucurbitaceae, Solanaceae, Chenopodiaceae, Amaranthaceae and Fabaceae. Reaction of host plants to ZYMV isolates tested in this study is summarized in . The majority of cucurbit species were systemically infected and showed typical mosaic symptoms. The symptoms also included stunting, leaf deformation and chlorotic local lesions. Overall, there were no obvious correlations of host responses to inoculation with ZYMV isolates from different phylogenetic groups. However, in Cucurbita pepo, all three isolates from subgroup A-I induced mosaic and leaf deformation (), all isolates from subgroup A-II induced mosaic (), whereas all three isolates from group C induced chlorotic local lesions (). The isolate SHZ-1 from subgroup A-II was asymptomatic on Cucumis melo (cultivar ‘Jiashi’), but the virus could be detected using RT-PCR. Moreover, the isolates WJQ-102-1, TKS-1 and TKS-2 from subgroup A-II did not induce any symptoms on Cucumis sativus and the inoculated plants were negative in RT-PCR. Most isolates tested induced necrotic local lesions on the inoculated leaves of Chenopodium amaranticolor and C. quinoa (–h), whereas the isolates CJ-1 and CJ-2 from subgroup A-II did not induce any symptoms. The isolates WJQ-102–1, TKS-1 and TKS-2 from subgroup A-II induced necrotic local lesions on the inoculated leaves of Gomphrena globosa and the virus could be detected using RT-PCR. The isolate TYG-1 from subgroup A-II was asymptomatic on G. globosa, but the virus could be detected. Other isolates did not induce any symptoms on G. globosa and the virus was not detected in the inoculated leaves using RT-PCR. The attempts to transmit the isolates to Nicotiana tabacum, Datura stramonium, Vicia faba, Phaseolus vulgaris, Vigna unguiculata or Pisum sativum failed as confirmed by negative RT-PCR. In addition, the results of pathotype test showed that the 13 ZYMV isolates tested in this study did not induce any symptoms on melon accession PI414723 and the inoculated plants were negative in RT-PCR (). Hence, these ZYMV isolates were classified as pathotype 0.
Fig. 3 (Colour online) Symptoms of different hosts inoculated with Zucchini yellow mosaic virus different phylogenetic group isolates from Xinjiang: a, Mosaic in Cucumis melo (cultivar ‘Jiashi’) infected with isolate TKS-2 from subgroup A-II; b, Mosaic induced by isolate WJQ-102–1 from subgroup A-II in Cucumis melo (cultivar ‘Jiashi’); c, Mosaic caused by isolate TP-1 from subgroup A-I in Cucumis melo (cultivar ‘Jiashi’); d, Mosaic in Cucumis melo (cultivar ‘Jiashi’) infected with isolate JS-3 from group C; e, Mosaic in Luffa cylindrica infected with isolate SHZ-1 from subgroup A-II; f, Chlorotic local lesions induced by isolate TKS-2 from subgroup A-II in Chenopodium quinoa. g, Necrotic local lesions in Chenopodium quinoa infected with isolate JS-1 from group C; h, Necrotic local lesions in Chenopodium quinoa infected with isolate TP-3 from subgroup A-I; i, Mosaic in Cucurbita pepo infected with isolate TYG-1 from subgroup A-II; j, Chlorotic local lesions caused by isolate JS-1 from group C in Cucurbita pepo; k, Mosaic induced by isolate CJ-1 from subgroup A-II in Cucurbita moschata; l, Mosaic and leaf deformation in Cucurbita pepo infected with isolate TP-1 from subgroup A-I; m, Mosaic caused by isolate TP-2 from subgroup A-I in Cucumis sativus; n, Mosaic, leaf deformation and stunting in Cucumis sativus infected with isolate TYG-1 from subgroup A-II. The letters NC show negative control plants which were inoculated with buffer only.
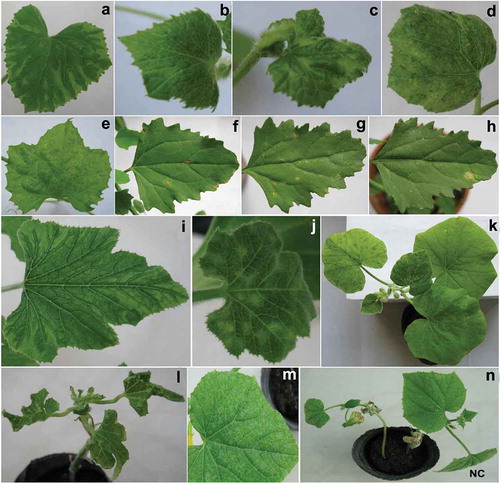
Discussion
Zucchini yellow mosaic virus is one of the most economically important viruses of cucurbit crops worldwide. Its origins and mechanisms for evolution, geographic distribution and worldwide spread represent important questions to understand plant virus emergence. Our results showed that ZYMV was detected in nine of 13 locations in Xinjiang. It is interesting to see that four locations and two cultivars were totally PCR negative. This may be related to agronomic and ecological factors such as cropping system and the abundance of aphid vector populations. These factors which determine the prevalence of viral diseases are largely correlated with climatic conditions that differ greatly among geographic locations. The cultivar ‘Queen’ was planted in locations HY, NH and PZ. Moreover, the cultivar ‘Jinlong’ was planted in locations HY and NH. Locations HY and NH which belong to Hami city are located in the eastern region of Xinjiang (). It is noteworthy that the sunshine duration of Hami city was more than 1772 hours during the 2014 growing season (May–September), but it was less than 1660 hours in other locations (Anonymous Citation2015). In addition, the two cultivars free of ZYMV were separated by cotton in some melon fields in locations HY and NH, potentially disrupting aphid spread. Additionally, the relative virus incidence on melon cultivars may be related to genetic differences between the cultivars. However, information on these genetic differences is still lacking. Further research is needed to reveal the basis of genetic differences between melon cultivars planted in Xinjiang.
Sequence analysis showed phylogenetic differences between ZYMV isolates from different geographic locations. Previous phylogenetic analyses of ZYMV isolates from different countries revealed three main groups as proposed by Coutts et al. (Citation2011) and Romay et al. (Citation2014). Our results support division of ZYMV isolates from China into three major phylogenetic groups (A, B and C). These molecular groupings suggest that at least three separate introduction events of ZYMV occurred in Xinjiang, as has been suggested for Australia (Coutts et al. Citation2011). While it is difficult to determine the pathway by which ZYMV first entered Xinjiang, we can reasonably infer that infected cucurbit material imported from another region may have provided the initial virus source prior to adoption of stringent quarantine procedures (Lecoq et al. Citation2003; Simmons et al. Citation2011).
Changes in genetic composition of a virus population can compromise durability and effectiveness of disease control strategies (Acosta-Leal et al. Citation2011). An improved understanding of selective forces driving ZYMV evolution and genetic diversity, therefore, can help to identify more effective and durable disease management strategies. A high-level genetic diversity among ZYMV isolates was previously reported in Asia (particularly China) (Simmons et al. Citation2008). In support of this we found that the mean genetic distance within 26 ZYMV isolates obtained in this study (0.2306 ± 0.0387) was higher than those within most other geographic regions (Bananej et al. Citation2008). Our study indicated an overall dN/dS ratio of <1 for the complete CP sequences of the 26 ZYMV isolates from Xinjiang and this was in agreement with the previous study by Coutts et al. (Citation2011) who reported an overall dN/dS ratio of <1 for 143 ZYMV complete CP sequences. A value of ω<1 indicates negative selection; ω=1 indicates neutral evolution; ω>1 corresponds to positive selection (Coutts et al. Citation2011). In addition, Simmons et al. (Citation2008) found no evidence for positive selection acting on ZYMV CP sequences and suggested the in situ evolution of ZYMV within several countries; they also suggested that human activities have played a central role in ZYMV dispersal. Thus, the lack of positive selection acting on the CP gene of ZYMV within the population indicates there is little change once a ZYMV isolate becomes established in a location, unless a new virus variant is introduced, as observed in France (Lecoq et al. Citation2009). For example, nine isolates from location JS showed little sequence variation with each other, but they showed high-level variation with other isolates from Xinjiang. However, selection pressures on the ZYMV CP might be different from other regions of the ZYMV genome, so further analysis is needed to confirm this. In addition, the 26 ZYMV isolates obtained in this study had a conserved amino acid triplet DAG and this was in agreement with the previous study by Bananej et al. (Citation2008), who reported that 12 Iranian ZYMV isolates had the DAG at the N-terminal part of the CP. Nevertheless, their transmission by aphids has yet to be confirmed.
Previous studies revealed that ZYMV isolates from different phylogenetic groups differed in biological characteristics (Coutts et al. Citation2011; Spadotti et al. Citation2015). In this study, we attempted to determine if there were differences in host responses to inoculation with ZYMV isolates from different phylogenetic groups from Xinjiang. Sap inoculation of the 13 ZYMV isolates onto a range of 14 plant species revealed differences in symptoms and host range (). Overall, there were no obvious correlations of host responses to inoculation with these isolates except for Cucurbita pepo. Therefore, it may be possible to differentiate ZYMV isolates from group C and subgroups A-I and A-II by their reactions on this host, but more isolates would need to be tested to establish this. In addition, Coutts et al. (Citation2011) cautiously suggested that symptomless infections in Chenopodium quinoa might be a way to differentiate A-II isolates. In the present study, most isolates tested induced necrotic local lesions on inoculated leaves of C. quinoa, whereas the isolates CJ-1 and CJ-2 from subgroup A-II did not induce any symptoms. However, other isolates from subgroup A-II induced necrotic local lesions on inoculated leaves of C. quinoa. Thus, our results did not concur with the observations by Coutts et al. (Citation2011). Our results suggested that the symptomless infections in C. quinoa may not be used to differentiate A-II isolates.
The complete CP sequences of isolates TYG-1, TKS-1, TKS-2, WJQ-102-1, SHZ-1, CJ-1 and CJ-2 have 99.4–100% nucleotide identity, and 99.3–100% amino acid identity (Supplemental table S1), but showed differences in bioassays (). Previous studies have shown that direct connections were demonstrated between the CP coding region and the pathotype of potyviruses, including ZYMV (Andrejeva et al. Citation1999; Ullah & Grumet Citation2002; Ullah et al. Citation2003; Bukovinszki et al. Citation2007). Moreover, the CP coding region is a valuable determinant in the potyvirus classification (Shukla et al. Citation1994). Although the isolates TYG-1, TKS-1, TKS-2, WJQ-102-1, SHZ-1, CJ-1 and CJ-2 were closely related in CP gene identity, they showed different phenotypes in some host plants tested. For example, the CP of TKS-1 and SHZ-1 had identical amino acid sequences (Supplemental table S1). However, isolate SHZ-1 was asymptomatic on Cucumis melo (cultivar ‘Jiashi’), but isolate TKS-1 induced mosaic on this plant species. Isolate TKS-1 did not induce any symptoms on Cucumis sativus, but isolate SHZ-1 induced mosaic on this species. Furthermore, isolate TKS-1 induced necrotic local lesions on inoculated leaves of Gomphrena globosa, but isolate SHZ-1 did not induce any symptoms on this species (). It is unlikely that the CP coding region could be responsible for the significant differences existing between the phenotype responses of these isolates. Previous studies have shown that the ZYMV HC-Pro is a multifunctional protein that plays important roles in biological properties, such as synergism, gene silencing suppression and symptom expression (Gal-On Citation2007). In the ZYMV HC-Pro, a single amino acid substitution from R to I (position 180) critically alters the virus phenotype responses, which changes from aggressive to causing mild mosaic symptoms on squash, and from aggressive to symptomless on watermelon, melon and cucumber (Gal-On Citation2007). The same might hold true in the case of ZYMV isolates obtained in this study. In conclusion, although some ZYMV isolates from Xinjiang presented a low molecular diversity, they were biologically variable. This suggests that they share a common origin but that they have subsequently adapted to their different hosts. In agreement with our results, Chikh Ali et al. (Citation2009) reported that although two Syrian ZYMV isolates (SYZY-1 and SYZY-3) were closely related regarding the CP gene identity, they showed different phenotypes in host plants tested. The SYZY-1 CP nucleotide and amino acid sequences had a similarity of 99.5 and 100% with that of SYZY-3, respectively. However, the two isolates showed biological diversity with regard to their pathogenicity and symptoms. The authors cautiously suggested that adaptation to different hosts might have caused the variable biological properties of the two Syrian ZYMV isolates (Chikh Ali et al. Citation2009).
Although the host range of the 13 ZYMV isolates tested in this study was similar, biological properties on different hosts revealed some differences compared with the ZYMV isolates collected from other countries such as Iran (Bananej et al. Citation2008), Chile (Prieto et al. Citation2001), Syria (Chikh Ali et al. Citation2009) and Brazil (Spadotti et al. Citation2015). For example, all ZYMV isolates from Iran can induce symptoms (mosaic and yellowing) on Cucumis sativus (Bananej et al. Citation2008). In contrast, isolates WJQ-1, TKS-1 and TKS-2 from Xinjiang did not infect this host (). Some strains of ZYMV from Lebanon and Israel have been reported to produce systemic infection without visible symptoms in Pisum sativum (Lesemann et al. Citation1983; Antignus et al. Citation1989), but all ZYMV isolates from Xinjiang did not infect this species. There was no infection in Pisum sativum also reported for a Chilean isolate (Prieto et al. Citation2001). These results suggested that under field conditions, cucurbit plants may be an important virus source. Furthermore, differences in biological properties between ZYMV isolates reflect changes in the nucleotide sequences in its CP, HC-Pro or P3 protein genes (Gal-On Citation2007; Glasa et al. Citation2007). For example, isolate SHZ-1 obtained in this study induced mosaic on Cucurbita pepo (), but the Syrian ZYMV isolate SYZY-1 induced stunting, yellowing, leaf deformation, blister and necrosis on this plant species (Chikh Ali et al. Citation2009). Interestingly, two amino acid substitutions were found at the N-terminal part of the CP of these two isolates. The isolate SHZ-1 had two amino acid residues T and R at positions 5 and 23 in the CP, respectively, while the isolate SYZY-1 displayed P and K at the same positions in the CP.
The melon accession PI414723 is the only known source for ZYMV resistance in melon (Danin-Poleg et al. Citation2002). ZYMV resistance was reported to be controlled by the Zym-1 gene and possibly by two additional genes with minor genetic effects (Danin-Poleg et al. Citation1997; Périn et al. Citation2002). However, the reaction of PI414723 to ZYMV is not uniform. Based on three possible reactions of this accession, Lecoq & Pitrat (Citation1984) classified isolates of the virus into three pathotypes: no symptoms (pathotype 0), systemic necrotic or chlorotic spots (pathotype 1), and systemic mosaic and wilting (pathotype 2). Our results showed that the 13 ZYMV isolates from Xinjiang were classified as pathotype 0. The pathotype of 173 ZYMV isolates from Martinique was determined based on the reaction of melon accession PI414723 that was mechanically inoculated. The results showed that 88, 64 and 21 isolates belonged to pathotypes 0, 1 and 2, respectively (Desbiez et al. Citation2002). The previous study by Romay et al. (Citation2014) reported that plants of melon accession PI414723 developed systemic chloro-necrotic spots with 33 of 34 ZYMV isolates from Venezuela indicating that most Venezuelan isolates of ZYMV belong to pathotype 1. Only one isolate was classified as pathotype 2 (Romay et al. Citation2014). Spadotti et al. (Citation2015) reported that 10 severe Brazilian ZYMV isolates from subgroups A-I and A-II were classified as belonging to pathotype 0. Overall, the molecular clustering of ZYMV isolates was not well correlated with their pathotype. For example, the 10 Brazilian ZYMV isolates which were assigned to pathotype 0 clustered into subgroups A-I and A-II (Spadotti et al. Citation2015). This is in agreement with our results (). Moreover, the Venezuelan ZYMV isolates which were assigned to pathotype 1 clustered into subgroup A-I (Romay et al. Citation2014). Our results showed that the 13 ZYMV isolates which were assigned to pathotype 0 clustered into group C and subgroups A-I and A-II (). The pathotype 1 and 2 isolates were not found in our study. In previous studies of ZYMV biological variability, a low proportion of pathotype 2 isolates has also been observed (Lecoq & Pitrat Citation1984; Desbiez et al. Citation2002; Yakoubi et al. Citation2008; Romay et al. Citation2014; Spadotti et al. Citation2015). Only in Sudan was the frequency of pathotype 2 found to be high in natural ZYMV populations; this was related to the frequent occurrence of wild melons possessing the Zym gene (Mahgoub et al. Citation1998). As the 13 ZYMV isolates from Xinjiang were assigned to pathotype 0, melon accession PI414723 is of considerable significance when virus-resistant melon cultivars are to be developed. It is concluded that melon cultivars carrying the Zym-1 resistance gene may be effective in controlling ZYMV in different melon-growing locations in Xinjiang. To our knowledge, this is the first report of ZYMV pathotypes in China. This information is valuable for the breeding programmes of virus-resistant cultivars.
Supplemental Table 1
Download MS Word (19.8 KB)Acknowledgements
This work was supported by the National Natural Science Funding of China (under grant numbers 31060148 and 31260258); and the Innovative Project of Xinjiang University (under grant number XJUBSCX-2012025). We thank Zi-Qiang Zhang and Hao Lu for help with the sample collection.
Supplemental material
Supplemental data for this article can be accessed online here: http://dx.doi.org/10.1080/07060661.2017.1285356.
Additional information
Funding
References
- Acosta-Leal R, Duffy S, Xiong Z, Hammond RW, Elena SF. 2011. Advances in plant virus evolution: translating evolutionary insights into better disease management. Phytopathology. 101:1136–1148.
- Andrejeva J, Puurand U, Merits A, Rubenstein F, Jarvekulg L, Valkonen JPT. 1999. Potyvirus helper component-proteinase and coat protein (CP) have coordinated functions in virus host interactions and the same CP motif affects virus transmission and accumulation. J Gen Virol. 80:1133–1139.
- Anonymous. 2015. Xinjiang statistical yearbook: statistic bureau of Xinjiang Uygur autonomous region. [ cited 2017 Jan 4]. Available from: http://www.xjtj.gov.cn/sjcx/tjnj_3415/
- Antignus Y, Raccah B, Gal-On A, Cohen S. 1989. Biological and serological characterization of Zucchini yellow mosaic and Watermelon mosaic virus-2 isolates in Israel. Phytoparasitica. 17:289–298.
- Atreya CD, Raccah B, Pirone TP. 1990. A point mutation in the coat protein abolishes aphid transmissibility of a potyvirus. Virology. 178:161–165.
- Bananej K, Keshavarz T, Vahdat A, Salekdeh GH, Glasa M. 2008. Biological and molecular variability of Zucchini yellow mosaic virus in Iran. J Phytopathol. 156:654–659.
- Bukovinszki A, Gotz R, Johansen E, Maiss E, Balazs E. 2007. The role of the coat protein region in symptom formation on Physalis floridana varies between PVY strains. Virus Res. 127:122–125.
- Chikh Ali M, Maoka T, Natsuaki KT. 2009. Biological and molecular characterization of two Zucchini yellow mosaic virus isolates from Syria. EPPO Bulletin. 39:99–104.
- Coutts BA, Kehoe MA, Webster CG, Wylie SJ, Jones RAC. 2011. Zucchini yellow mosaic virus: biological properties, detection procedures and comparison of coat protein gene sequences. Arch Virol. 156:2119–2131.
- Danin-Poleg Y, Paris HS, Cohen S, Rabinowitch HD, Karchi Z. 1997. Oligogenic inheritance of resistance to Zucchini yellow mosaic virus in melons. Euphytica. 93:331–337.
- Danin-Poleg Y, Tadmor Y, Tzuri G, Reis N, Hirschberg J, Katzir N. 2002. Construction of a genetic map of melon with molecular markers and horticultural traits, and localization of genes associated with ZYMV resistance. Euphytica. 125:373–384.
- Desbiez C, Lecoq H. 1997. Zucchini yellow mosaic virus. Plant Pathol. 46:809–829.
- Desbiez C, Wipf-Scheibel C, Lecoq H. 2002. Biological and serological variability, evolution and molecular epidemiology of Zucchini yellow mosaic virus (ZYMV, Potyvirus) with special reference to Caribbean islands. Virus Res. 85:5–16.
- FAOSTAT. 2014. The FAO statistical database (FAOSTAT): food and agriculture organization of the United Nations. [ cited 2016 Jul 15]. Available from: http://faostat3.fao.org
- Gal-On A. 2007. Zucchini yellow mosaic virus: insect transmission and pathogenicity – the tails of two proteins. Mol Plant Pathol. 8:139–150.
- Glasa M, Pittnerova S. 2006. Complete genome sequence of a Slovak isolate of Zucchini yellow mosaic virus (ZYMV) provides further evidence of a close molecular relationship among Central European ZYMV isolates. J Phytopathol. 154:436–440.
- Glasa M, Svoboda J, Novakova S. 2007. Analysis of the molecular and biological variability of Zucchini yellow mosaic virus isolates from Slovakia and Czech Republic. Virus Genes. 35:415–421.
- Ha C, Revill P, Harding RM, Vu M, Dale JL. 2008. Identification and sequence analysis of potyviruses infecting crops in Vietnam. Arch Virol. 153:45–60.
- Katis NI, Tsitsipis JA, Lykouressis DP, Papapanayotou A, Margaritopoulos JT, Kokinis GM, Perdikis DC, Manoussopoulos IN. 2006. Transmission of Zucchini yellow mosaic virus by colonizing and non-colonizing aphids in Greece and new aphid species vectors of the virus. J Phytopathol. 154:293–302.
- Larkin MA, Blackshields G, Brown NP, Chenna R, McGettigan PA, McWilliam H. 2007. Clustal W and clustal X version 2.0. Bioinformatics. 23:2947–2948.
- Lecoq H, Desbiez C, Wipf-Scheibel C, Girard M. 2003. Potential involvement of melon fruit in the long distance dissemination of cucurbit potyviruses. Plant Dis. 87:955–959.
- Lecoq H, Pitrat M. 1984. Strains of Zucchini yellow mosaic virus in muskmelon (Cucumis melo L.). J Phytopathol. 111:165–173.
- Lecoq H, Wipf-Scheibel C, Chandeysson C, Leˆ Van A, Fabre F, Desbiez C. 2009. Molecular epidemiology of Zucchini yellow mosaic virus in France: an historical overview. Virus Res. 141:190–200.
- Lesemann DE, Makkouk KM, Koenig R, Natafji Samman E. 1983. Natural infection of cucumbers by Zucchini yellow mosaic virus in Lebanon. Phytopathol Z. 108:304–313.
- Li WH. 1993. Unbiased estimation of the rates of synonymous and nonsynonymous substitution. J Mol Evol. 36:96–99.
- Lisa V, Boccardo G, D’Agostino G, Dellavalle G, D’Aquilio M. 1981. Characterization of a potyvirus that causes zucchini yellow mosaic. Phytopathology. 71:667–672.
- Mahgoub HA, Desbiez C, Wipf-Scheibel C, Dafalla G, Lecoq H. 1998. Biological and serological variability of Zucchini yellow mosaic virus in Sudan. J Phytopathol. 146:333–337.
- Mascarell-Creus A, Cañizares J, Vilarrasa-Blasi J, Mora-García S, Blanca J, Gonzalez-Ibeas D, Saladié M, Roig C, Deleu W, Picó-Silvent B, et al. 2009. An oligo-based microarray offers novel transcriptomic approaches for the analysis of pathogen resistance and fruit quality traits in melon (Cucumis melo L.). BMC Genomics. 10:467–481.
- Pamilo P, Bianchi NO. 1993. Evolution of the Zfx and Zfy genes: rates and interdependence between the genes. Mol Biol Evol. 10:271–281.
- Périn C, Hagen LS, De Conto V, Katzir N, Danin-Poleg Y, Portnoy V, Baudracco-Arnas S, Chadoeuf J, Dogimont C, Pitrat M. 2002. A reference map of Cucumis melo based on two recombinant inbred lines populations. Theor Appl Genet. 104:1017–1034.
- Pfosser MF, Baumann H. 2002. Phylogeny and geographical differentiation of Zucchini yellow mosaic virus isolates (Potyviridae) based on molecular analysis of the coat protein and part of the cytoplasmic inclusion protein genes. Arch Virol. 147:1599–1609.
- Pitrat M, Lecoq H. 1984. Inheritance of zucchini yellow mosaic virus resistance in Cucumis melo L. Euphytica. 33:57–61.
- Prieto H, Bruna A, Hinrichsen P, Muñoz C. 2001. Isolation and molecular characterization of a Chilean isolate of Zucchini yellow mosaic virus. Plant Dis. 85:644–648.
- Romay G, Lecoq H, Geraud-Pouey F, Chirinos DT, Desbiez C. 2014. Current status of cucurbit viruses in Venezuela and characterization of Venezuelan isolates of Zucchini yellow mosaic virus. Plant Pathol. 63:78–87.
- Rui ZY, Goldfarb RJ, Qiu YM, Zhou TH, Chen RY, Pirajno F, Yun G. 2002. Paleozoic–early Mesozoic gold deposits of the Xinjiang autonomous region, northwestern China. Mineralium Deposita. 37:393–418.
- Rybicki EP, Shukla DD. 1992. Coat protein phylogeny and systematics of potyviruses. Arch Virol. 5:139–170.
- Shukla DD, Frenkel MJ, Ward CW. 1991. Structure and function of the potyvirus genome with special reference to the coat protein coding region. Can J Plant Pathol. 13:178–191.
- Shukla DD, Ward CW, Brunt AA. 1994. The Potyviridae. Wallingford (GB): CAB International; p. 187–208.
- Simmons HE, Holmes EC, Gildow FE, Bothe-Goralczyk MA, Stephenson AG. 2011. Experimental verification of seed transmission of Zucchini yellow mosaic virus. Plant Dis. 95:751–754.
- Simmons HE, Holmes EC, Stephenson AG. 2008. Rapid evolutionary dynamics of Zucchini yellow mosaic virus. J Gen Virol. 89:1081–1085.
- Spadotti DMDA, Wassano DT, Rezende JAM, Camargo LEA, Inoue-Nagata AK. 2015. Biological and molecular characterization of Brazilian isolates of Zucchini yellow mosaic virus. Sci Agric. 72:187–191.
- Tamura K, Peterson D, Peterson N, Stecher G, Nei M, Kumar S. 2011. MEGA5: molecular evolutionary genetics analysis using maximum likelihood, evolutionary distance, and maximum parsimony methods. Mol Biol Evol. 28:2731–2739.
- Ullah Z, Chai B, Hammar S, Raccah B, Gal-On A, Grumet R. 2003. Effect of substitution of the amino termini of coat proteins of distinct potyvirus species on viral infectivity and host specificity. Physiol Mol Plant Pathol. 63:129–139.
- Ullah Z, Grumet R. 2002. Localization of Zucchini yellow mosaic virus to the veinal regions and role of viral coat protein in veinal chlorosis conditioned by the Zym potyvirus resistance locus in cucumber. Physiol Mol Plant Pathol. 60:79–89.
- Urcuqui-Inchima S, Haenni A, Bernardi F. 2001. Potyvirus proteins: a wealth of functions. Virus Res. 74:157–175.
- Vučurović A, Bulajić A, Stanković I, Ristić D, Berenji J, Jović J, Krstić B. 2012. Non-persistently aphid-borne viruses infecting pumpkin and squash in Serbia and partial characterization of Zucchini yellow mosaic virus isolates. Eur J Plant Pathol. 133:935–947.
- Yakoubi S, Desbiez C, Fakhfakh H, Wipf-Scheibel C, Fabre F, Pitrat M, Marrakchi M, Lecoq H. 2008. Molecular, biological and serological variability of Zucchini yellow mosaic virus in Tunisia. Plant Pathol. 57:1146–1154.
- Zhao MF, Chen J, Zheng HY, Adams MJ, Chen JP. 2003. Molecular analysis of Zucchini yellow mosaic virus isolates from Hangzhou, China. J Phytopathol. 151:307–311.
- Zheng GY, Dong T. 1989. Occurrence of Zucchini yellow mosaic virus in Xinjiang. Acta Phytopathologica Sinica. 21:72. Chinese.