Abstract
A new disease on greenhouse pepper (Capsicum annuum L.) plants in Culiacan, Mexico with symptoms of chlorosis, wilting and vascular necrosis was observed in 2014. Sixty diseased pepper plants were collected from three different areas of the Culiacan Valley to determine the causal agent of the disease and analyse the potential risk presented by this disease in this region. Fusarium oxysporum was recovered consistently (88% of samples collected) and identified based on cultural, morphological and pathogenicity tests, as well as by PCR amplification and DNA sequencing of the TEF-1α gene. Two pathogenicity tests with 10 monoconidial isolates of F. oxysporum, which were selected randomly from diseased tissues, produced disease symptoms similar to those observed on pepper plants in the greenhouses. Symptoms were different from the typical root rot, wilting and necrosis caused by F. solani and F. oxysporum f. sp. radicis-capsici and instead matched those reported for F. oxysporum f. sp. capsici causing pepper wilt. The results suggest that the causal agent of pepper wilt in the samples analysed was F. oxysporum. This is the first report of pepper wilt caused by this fungus in Mexico, and this disease is a potential threat for pepper production in this region.
Résumé
En 2014, une nouvelle maladie s’attaquant aux plants de piments cultivés en serre (Capsicum annuum L.) à Culiacan, au Mexique, est apparue. Les symptômes se manifestaient par la chlorose, le flétrissement et la nécrose vasculaire. Soixante plants de piments infectés ont été collectés à trois différents endroits de la vallée de Culiacan afin de déterminer l’agent causal de la maladie et d’analyser le risque potentiel qu’elle représentait pour la région. Fusarium oxysporum a été invariablement récupéré (88% des échantillons collectés) et identifié en se basant sur les analyses culturales et morphologiques et les tests de pathogénicité, ainsi que par amplification par PCR et séquençage de l’ADN du gène du TEF-1α. Deux tests de pathogénicité menés avec10 isolats monoconidiaux de F. oxysporum, prélevés aléatoirement sur des tissus infectés, ont produit des symptômes semblables à ceux observés sur les plants cultivés en serre. Les symptômes différaient de ceux du pourridié, du flétrissement et de la nécrose typiques causés par F. solani et F. oxysporum f. sp. radicis-capsici et s’apparentaient davantage à ceux décrits pour Fusarium oxysporum f. sp. capsici causant le flétrissement du piment. Les résultats suggèrent que l’agent causal du flétrissement du piment trouvé dans les échantillons analysés était F. oxysporum. Il s’agit de la première mention du flétrissement du piment causé par ce champignon au Mexique, et cette maladie représente une menace potentielle à la production du piment dans cette région.
Introduction
Mexico is one of the main producers of peppers (Capsicum annuum L.) worldwide, with around 176,517 ha cultivated in 2014, representing about 65.4% of the total area cultivated to peppers in the Americas (FAO, 2017). Sinaloa State is one of the main producers of peppers within Mexico, where production reached 14,084 ha in 2016 (SAGARPA, 2017). Therefore, any new limitation to the production of this crop is of concern.
In 2014, greenhouse pepper growers at different sites in Culiacan, Sinaloa, reported a wilt disease that was affecting their plants, but the disease did not exhibit symptoms associated with other well-known pepper diseases (Black, Citation2003). Sweet pepper plants ‘Lorca’ in the production stage exhibited wilting, stunting, chlorosis of new leaves and vascular necrosis up to 30 cm from the base of the plants. At three 65 ha sites, there was 3–15% plant mortality at the beginning of the production stage, with an estimated loss of US $2,730,000.
Phytophthora capsici Leonian historically has been regarded as the causal agent of pepper wilt in this region of México (Velásquez-Valle et al., Citation2001). Based on reports from the growers and initial observations, P. capsici was believed to be the causal agent in this particular outbreak. The lack of root and crown rot symptoms, however, combined with the observation of vascular necrosis extending 30 cm above the base of the plants and the low level of control achieved with some specific chemical treatments for P. capsici, collectively suggested that an unknown pathogen was responsible for the observed symptoms.
Many isolates in the Fusarium species complex have been linked to pepper wilt. In Ontario, Canada and Almería, Spain, there were several recent reports of pepper wilt caused by Fusarium oxysporum Schlecht. causing root and basal stem rot on peppers (Lomas-Cano et al., Citation2014, Citation2016; Pérez-Hernández et al., Citation2014; Cerkauskas, Citation2017). On the other hand, some decades ago, several studies showed that different strains of F. solani Mart, were pathogenic on pepper (Govindaswamy, Citation1963; Joffe & Palti, Citation1972; Rahin & Sharif, Citation1985). Also, Fusarium oxysporum f. sp. vasinfectum Snyder & Hans. has been related to the Fusarium spp. that cause pepper wilts (Ortuño-Martínez et al., Citation1972; Miller et al., Citation1996). Rahin & Sharif (Citation1985) attributed pepper wilting to F. oxysporum var. redolens Wollenw, now classified as a separate species named F. redolens Wollenw, which can cause wilt in pepper plants at different phenological stages, but without host specificity. However, there are a few reports of vascular necrosis on pepper plants caused by Fusarium oxysporum f. sp. capsici Rivelli (Rivelli, Citation1989; Jones & Black, Citation1992). Black (Citation2003) described the symptoms of F. oxysporum f. sp. capsici as those of a vascular Fusarium wilt and suggested that many studies about Fusarium species that infect pepper did not provide sufficient evidence to distinguish it from a host-specific vascular wilt. Therefore, the literature regarding vascular necrosis caused by F. oxysporum in pepper plants remains unclear.
Fusarium oxysporum f. sp. capsici has been reported in pepper crops only in Louisiana, USA (Rivelli, Citation1989; Jones & Black, Citation1992). According to Black et al. (Citation1993), the geographic distribution of this pathogen remains unknown. Therefore, several epidemiological questions about this specific pathogen need to be evaluated further.
The warm and humid environment and high plant density associated with the production of greenhouse crops, along with the practice of monoculture, are conducive to the establishment and spread of fungal diseases. Fortunately, the majority of these diseases can be managed by manipulation of environmental conditions, the application of fungicides, or the use of resistant varieties (Marois & Mitchell, Citation1981; Jarvis, Citation1989; King et al., Citation2010). Nevertheless, when a new disease emerges, it can be very difficult to find a control solution if the pathogen agent is not properly identified. The implementation of appropriate disease management measures requires a reliable identification and detection of the pathogen (Moine et al., Citation2014).
In addition to classical morphological and colony growth techniques, molecular biology methods, especially those involving the polymerase chain reaction (PCR), have provided a rapid and sensitive approach for the detection and identification of plant pathogens and many soil-borne pathogenic fungi (O’Donnell, Citation2000; Vincenlli & Tisserat, Citation2008). Several DNA regions, such as the internal transcribed spacer (ITS) region of the ribosomal DNA (rDNA) and, particularly, the translation elongation factor 1-α (tef) gene, are useful for the identification of Fusarium spp. (O’Donnell et al., Citation1998; Geiser et al., Citation2004).
The objectives of the present study were to: (i) identify the pathogen infecting pepper plants using cultural, morphological and molecular methods; and (ii) analyse the risk posed to pepper by this disease in the Culiacan Valley, Sinaloa.
Materials and methods
Diseased plant samples
In November 2014, 60 pepper plants of the cultivar ʻLorcaʼ at the production stage (70 days old) showing wilt and other symptoms were sampled from commercial greenhouses located in three different areas of the Culiacan Valley (Costa Rica, Villa Juarez and La Palma). These areas are the most representative with respect to greenhouse pepper production systems in this region. These three sites form a 285.48 km2 triangle where the main production of peppers of the Culiacan Valley is concentrated. Symptoms on the plants sampled included wilting, stunting, chlorotic new leaves and vascular necrosis. Around 20 cm of tissue was sampled with a sterile scalpel from the regions on the margin of the xylem showing brown-dark necrosis. The samples were immediately surface-disinfected in 0.5% NaOCl (3 min) and 70% ethanol (3 min) and rinsed twice (5 min) in sterile distilled water. After drying on sterile absorbent paper, the collected tissues were cut in half. One half was frozen at −80°C, then lyophilized and stored at −15°C. The other part was sliced into small pieces and used for fungal isolation.
Fungal isolation and cultivation
Surface-sterilized tissue (see above) from diseased pepper plants was immediately plated in Petri dishes containing potato dextrose agar (PDA) and Komada’s medium (Komada, Citation1975). The dishes were incubated for 4–5 days at 28°C in the dark. The fungal colonies were examined following the morphological keys and methodology provided by Leslie & Summerell (Citation2006). To obtain monoconidial isolates, a small fragment of the mycelium from 6-day-old PDA cultures was transferred into 1.0 mL of sterile distilled water, serially diluted, and then plated on a new PDA dish. Two days later, the cultures were observed under a microscope, and individual germinating microspores were transferred to new PDA supplemented with pentachloronitrobenzene and chloramphenicol. Subsequently, 53 monoconidial isolates randomly selected from the 60 original isolates were sub-cultured on carnation leaf agar (CLA) to determine the species of the fungus isolated (Fisher et al., Citation1982).
DNA extraction and PCR assays
Twenty purified monoconidial isolates were selected and further characterized using PCR analysis. For DNA extraction, the method described by Velarde-Félix et al. (Citation2015) was used. A small fragment of fungal mycelium was collected from the culture medium with a bacteriological loop, macerated in a mortar with a pestle (previously sterilized and cooled to −70°C), to which 1 mL of extraction buffer (30 mM NaCl, 30 mM EDTA, pH 8.5) and 250 mM Tris Base (pH 8.5) was added. The homogenized mycelium was placed in a 1.5 mL sterile tube, then 100 µL of 10% cetyl trimethyl ammonium bromide (CTAB) and 250 µL 5M NaCl was added, and the mixture was incubated at 95°C for 15 min and then centrifuged. The aqueous solution was washed with one volume of chloroform and precipitated with cold absolute isopropanol. Finally, the DNA pellets obtained from different isolates were each re-suspended in 50 µL of nuclease-free water (Promega) and stored at 4°C.
The DNA extracted from the Fusarium isolates was analysed by PCR with the primers of O’Donnell et al. (Citation1998) and Geiser et al. (Citation2004), which amplified the translation elongation factor 1-alpha (TEF-1α) gene. The final reaction mixture (15 µL) contained 100 ng DNA template, an equimolar mixture of dATP, dCTP, dGTP and dTTP, 25 mM MgCl2, PCR buffer, 5U Taq DNA polymerase (Promega® PCR Master Mix, Madison, WI), and 40 pmoles of each oligonucleotide (Sigma®). The sequences of all the primers and PCR conditions used are provided in .
Table 1. Conditions of annealing temperatures and sequences of primers TEF-1α for PCR analysis of Fusarium spp.
The amplicon from one monoconidial isolate (strain number 8) of the 20 isolates analysed by PCR was excised from the agarose gel and purified through silica columns (EZ-10 Spin Column BS354 DNA Gel Extraction Kit, Bio Basic Inc., Ontario, Canada). After obtaining the purified PCR fragment, the sample was sent for sequencing at the National Laboratory of Genomics for Biodiversity (LANGEBIO) of the Center for Research and Advanced Studies (CINVESTAV-IPN), Irapuato, Guanajuato, Mexico. A similarity search with the DNA sequence was performed using the BLAST program, whereby the nucleotide sequences in the study were compared with those in the GenBank databases (National Center for Biotechnology Information (NCBI); http://www.ncbi.nlm. nih.gov/BLAST/).
Phylogenetic analyses
Phylogenetic analyses were performed using the EF-1α sequences. The sequences from all of the isolates were aligned using ClustalW (Thompson et al., Citation1994). A neighbour-joining tree was generated based on the alignment obtained with MEGA 7.0 (Tamura et al., Citation2011). The confidence of the nodes was tested with 1000 bootstrap replicates.
Pathogenicity tests
Two pathogenicity tests were carried out to evaluate the pathogenicity of 10 isolates randomly selected from the collection of 20 F. oxysporum isolates that were characterized as described above. The first and second trials were carried out during the winter and spring, respectively. Seedlings were grown in a greenhouse for 21 days with temperatures varying between 22–34°C and 12 h of light per day until inoculation. The pathogenicity was tested by inoculation of pepper plants ‘Lorca’ at the 2–3 true-leaf stage. This cultivar was selected because it was the cultivar grown at the sampled sites. Briefly, the roots of 20 plants grown in autoclaved peat Kekkila® (Vantaa, Finland), were washed, soaked in a conidial suspension (1 × 105 CFU mL−1) of each isolate for 20 min, and transplanted into a sandy loam soil mix in trays of 200 cells (3.1 × 3.1 cm size of each cell). Twenty plants were washed and soaked in water prior to transplanting as negative controls. After inoculation, the plants were maintained for 60 days in a growth chamber with a 12-h photoperiod of 400 lux and temperatures of 23–26°C. In both assays, a completely randomized design was used with two replicates of 10 plants each.
The pathogenicity of the isolates was assessed based on the level of symptom severity on the inoculated plants. Disease severity was rated on a scale of 1–9, where: 1 = plant without symptoms; 3 = plant without foliar symptoms and light necrosis in the xylem; 5 = light yellowing of the leaves and dark necrosis of the xylem but not above the cotyledons; 7 = stunted plant (plant that stops growing) with strong yellowing of the leaves with dark necrosis of the xylem above the cotyledons; and 9 = dead plant. Disease ratings were carried out at 60 days post-inoculation (dpi) in both assays, since about 90% of the inoculated plants showed symptoms of wilt by this time. The assay was repeated. The first trial was conducted in January 2015 and the second one in March 2015. Both trials were carried out under the same conditions described above.
Data analysis
Data obtained from the two pathogenicity assessments of F. oxysporum in the two assays were subjected to non-parametric variance analysis with the Kruskal–Wallis and Dunn median test, in order to determine the significant difference of virulence among strains (P ≤ 0.05). All statistical analyses were performed with the SAS software (SAS, Citation1999).
Results
Disease symptoms
Pepper plants dying from unspecified causes were reported from commercial greenhouse operations in Culiacan. Symptomatic plants had chlorotic new leaves, wilting, stunting, flower abortion and vascular necrosis (). Most of the plants sampled exhibited vascular necrosis 30 cm above the base of the plants (). Diseased pepper plants died within 60 days of the first appearance of chlorosis and wilting symptoms.
Fig. 1 (Colour online) Symptoms on diseased pepper plants. (a) Chlorotic leaves. (b) Wilted plants. (c) Dark-brown discolouration of xylem vessels 30 cm above the base of the plants. (d) Dead plants. (e-g) Fungal isolates from diseased pepper plants. (e) Top view of a typical colony growing on potato dextrose agar. (f) Microconidia and macroconidia, scale bar = 50 µm, (g) Phialides = 5 µm.
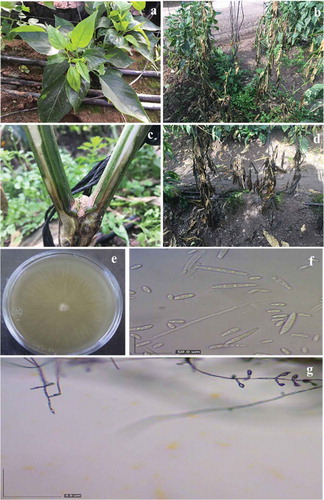
Sample collection and fungal description
Of the 60 pepper samples collected from three different areas of the Culiacan Valley, 53 fungal isolates were recovered from the plant tissues showing vascular necrosis symptoms. Surface disinfection and dissection of the diseased tissues into small pieces before plating onto growth medium allowed the consistent isolation of one fungus with uniform morphology in culture, producing cottony, white to yellowish colonies on PDA and Komada media (). Microscopic observations revealed that all isolates formed conidiophores and microconidia typical of the genus Fusarium (). No Phytophthora spp. or other microorganisms were observed on the two media used (PDA and Komada).
All 53 Fusarium isolates were identified on CLA medium as F. oxysporum on the basis of morphological characters, macroconidia (21.9 to 30.2 × 4.0 to 6.1 µm) with three to four septa, with foot-shaped basal cells and microconidia (6.0 to 14.5 × 2.8 to 4.2 µm) with 0 to 1 septa borne in false heads of short monophialides (-g). Chlamydospores were not evident.
Fungal identification
PCR analysis of all F. oxysporum isolates generated the expected 654 bp amplicon of the TEF-1α gene (). Subsequent database searches with BLASTn software indicated that the resulting sequence shared 100% similarity with the corresponding gene sequences of F. oxysporum in GenBank deposited by Lomas-Cano et al. (Citation2014) and Pérez-Hernández et al. (Citation2014), respectively (accession nos HG916993 and KF928931). One representative sequence from the 10 obtained was deposited in GenBank under accession no. KT780441.
Phylogenetic analysis of this sequence grouped a representative strain used in this study within the F. oxysporum species, especially with other strains isolated from pepper plants. It was clearly differentiated from the other F. oxysporum originating from different hosts and from the other species of Fusarium ().
Pathogenicity tests
There were no significant differences between the two trials in terms of symptoms severity on pepper at 60 dpi (H = 3.35; df = 1; P = 0.0672), with an average disease severity of 7.6 in the first trial and 7.5 in the second trial. The 10 monoconidial F. oxysporum isolates were pathogenic on pepper ʻLorcaʼ, although aggressiveness varied significantly (H = 89.31; df = 9; P ≤ 0.0001) (). While plants developed vascular necrosis following inoculation with each of the 10 isolates, the symptom severity varied. Isolates 9, 7, 3, 8, 10 and 4 were the most aggressive in both trials, causing disease severities of 8.9, 8.1, 8.0, 8.0, 7.9 and 7.7, respectively. The next most aggressive were isolates 2, 1, 5 and 6, which caused average disease severities of 7.2, 7.1, 6.4 and 6.2, respectively (). The first wilting symptoms were observed at 29 dpi on the inoculated plants in both assays. A longitudinal section of the stems of the diseased plants revealed dark-brown colouration of the vascular tissues (Fig. 4), matching the symptoms originally observed in pepper plants grown in commercial greenhouses (). The fungus was successfully re-isolated from the inoculated plants, completing Koch’s postulates in both assays. No symptoms were observed on the non-inoculated plants after 60 days.
Table 2. Pooled results of the first and second pathogenicity assay with Capsicum annuum ‘Lorca’ inoculated with 10 monoconidial Fusarium oxysporum strains isolated from pepper plants.
Discussion
This study identified and confirmed F. oxysporum as the causal agent of vascular necrosis and pepper wilt of greenhouse pepper plants. This is the first report of F. oxysporum causing vascular necrosis in pepper plants in Mexico, and represents a new geographic location for this disease, which had been reported previously only in Louisiana, USA (Rivelli, Citation1989; Jones & Black, Citation1992). To our knowledge, these results represent the second report of these symptoms caused by F. oxysporum worldwide, but this fungus could be present in other regions of the USA and Mexico. Therefore, further studies on its epidemiology on pepper and breeding for resistance need to be conducted. These results also provide basic information for the implementation of appropriate disease management strategies for this pathogen in Sinaloa (Retes-Manjarrez et al., Citation2016).
Different Fusarium species have been suggested as pepper pathogens in the literature over the years, including different species, varieties and formae speciales. Rivelli (Citation1989) evaluated F. oxysporum f. sp. vasinfectum, F. oxysporum f. sp. lycopersici, F. oxysporum f. sp. melonis and F. oxysporum f. sp. conglutinans and none of these formae speciales caused symptoms in Tabasco chili (Capsicum frutescens). Another group of authors suggested that F. solani was the causal agent of pepper wilt, but a causal relationship was not tested and, therefore, this fungus was not shown to be pathogenic on the inoculated pepper cultivar (Goidanich, Citation1964; Joffe & Palti, Citation1972). Rabinowitch & Cohen (Citation2012) attributed pepper wilt to F. oxysporum f. sp. radicis-cucumerinum. In the current study, all of the F. oxysporum strains isolated from pepper plants were pathogenic on pepper plants.
Although Fusarium spp. are significant plant pathogens affecting a large variety of hosts, their identification at the species level remains complicated (Geiser et al., Citation2004; Leslie & Summerell, Citation2006). The accurate identification of this new pepper pathogen represented a unique challenge because of the prevalence of Fusarium spp. attacking pepper plants and the taxonomic complexity related to Fusarium species in this crop (Black et al., Citation1993). By morphological observations and molecular analysis through TEF-1α sequencing of the amplicons, the causal agent was confirmed to be F. oxysporum. The TEF-1α sequence of strain number 8 from diseased tissue showed 100% identity with accessions from F. oxysporum f. sp. radicis-capsici f. sp. nov. isolated from pepper plants in Spain (Lomas-Cano et al., Citation2014; Pérez-Hernández et al., Citation2014). The symptomatology of vascular necrosis in this study, however, differed from that reported by Lomas-Cano et al. (Citation2014), Pérez-Hernández et al. (Citation2014) and Cerkauskas (Citation2017); these researchers described symptoms consisting of root and basal stem rot in pepper plants in Canada and Almería, Spain. These differences in symptomatology could be attributed to the use of different pepper cultivars, high genetic variation among the pathogen strains, different environmental conditions, and/or to the interaction of all these factors. In contrast, the vascular necrosis observed in this study matched those associated with pepper wilt caused by F. oxysporum f. sp. capsici causing pepper wilt (Black, Citation2003). Unfortunately, no corresponding TEF-1α sequence for F. oxysporum f. sp. capsici was found in GenBank, preventing a direct comparison.
The phylogenetic analysis of the sequence KT780441 showed that our sequence was grouped mainly within F. oxysporum that attacked peppers and clearly differentiated from the other F. oxysporum and Fusarium species originating from other hosts, like tomato (Solanum lycopersicum L.), potato (Solanum tuberosum L.), carnation (Dianthus caryophyllus L.), banana (Musa spp. L.), tulipa (Tulipa spp. L.), chickpea (Cicer arietinum L.) and mango (Mangifera indica L.) (). These results indicated that our strain was grouped specifically within F. oxysporum and is closer to Fusarium strains that attacked pepper in Spain versus other hosts. Although the origin of these strains remains unknown, Gordon & Martyn (Citation1997) suggested that most of the new occurrences of Fusarium wilt appear to be the result of a recent introduction rather than an independent local origin of the pathotype.
Fig. 2 PCR products obtained from genomic DNA of isolates of Fusarium oxysporum with the primer pair EF-1 and EF-2, viewed on a 1.0% agarose gel. Lane-1 ‘HyperLadder 1kb Plus’; lanes 2–4: isolates from diseased pepper plants; lane 5: purified mycelium of F. oxysporum isolate (positive control); lane 6: negative control.
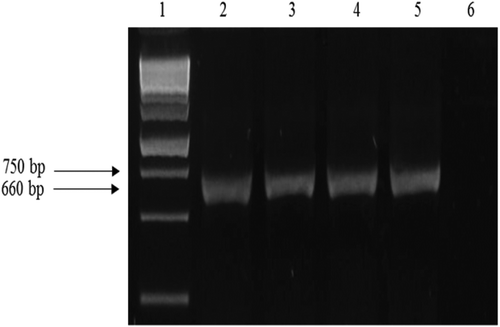
Fig. 3 Phylogenetic dendrogram using Neighbour-joining method based on the alignment of partial nucleotide sequences of the TEF-1α gene of Fusarium oxysporum with that of a selected F. oxysporum isolate (number 8 *) included in both pathogenicity trials. Values at the nodes represent the percentage bootstrap scores (1000 replicates), with only those values above 50 shown.
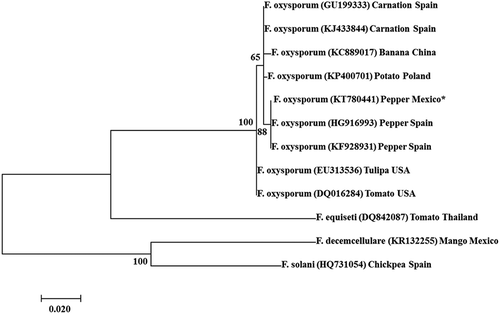
Fig. 4 (Colour online) Inoculation studies. (a) Control plant inoculated with agar medium (left) and plants (right) inoculated with Fusarium oxysporum isolated from pepper plants. (b) Dark necrosis of vascular tissues from one pepper plant inoculated with the same strain of F. oxysporum. Photographs a and b were taken 50 days after inoculation. (c) View of a tray of pepper plants inoculated with four different monoconidial strains of F. oxysporum. Photo was taken 29 days after inoculation.
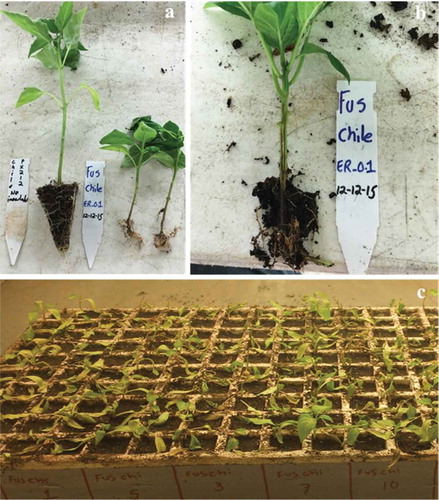
Several formae speciales of F. oxysporum are known, reflecting host range specialization (Snyder & Hansen, Citation1940). The formae speciales designations were intended to describe the physiological capabilities of the fungi and are not part of the formal taxonomic hierarchy (Kistler, Citation1997). Isolates with the same or similar host ranges are assigned to formae speciales and currently there are more than 120 f. sp. described in F. oxysporum (Michielse & Rep, Citation2009). Genetic identification by comparison of the TEF-1α gene sequences is not sufficient to determine the specific formae speciales and further studies of the host range of the F. oxysporum isolates from this study are needed to determine the correct forma speciales. Nonetheless, based on the current results, we confirm that the causal agent of pepper wilt in the samples collected in Culiacan, Mexico, is F. oxysporum, regardless of its specific formae speciales. To our knowledge, this is the first report of this species causing vascular necrosis and pepper wilt in Mexico.
The causal agent of the pepper wilt and vascular necrosis was identified as F. oxysporum in 88% of the 60 samples analysed from three sites in the Culiacan valley. These results suggest that F. oxysporum is present at all three sites. Since the three sites (Costa Rica, Villa Juarez and La Palma towns) form a triangle of approximately 285 km2 encompassing the region where most of the pepper production occurs in the Culiacan Valley, F. oxysporum represents a potentially serious risk for pepper production in this region. These results are consistent with those of Velásquez-Valle et al. (Citation2001), who reported that Fusarium spp. are distributed more widely in the centre and north of Mexico and are one of the main limitations for pepper production in this part of Mexico.
In the pathogenicity tests, all 10 monoconidial isolates of F. oxysporum were pathogenic on pepper ʻLorcaʼ, although their aggressiveness varied significantly. It is known that F. oxysporum shows variability in many characteristics, including morphology, pathogenicity and host range (Gordon & Martyn, Citation1997; Baayen et al., Citation2000). Boix-Ruíz et al. (Citation2014) also found different levels of variation for the pathogenicity trait in F. oxysporum f. sp. lycopersici races 2 and 3 and in isolates of F. oxysporum f. sp. radicis-lycopersici under different temperature conditions. However, temperature is not a unique factor influencing these results. Based on the current results, we suggest that chemical control is not suitable for these F. oxysporum isolates because it could result in the rapid generation of fungicide-resistant strains based on the high level of genetic variation.
Fusarium oxysporum is one of the most challenging fungal species to manage in protected crops (Dean et al., Citation2012). Unfortunately for the growers, the use of chemical fungicides has been gradually restricted in Mexico for environmental reasons and due to undesirable effects on health (Zasada et al., Citation2010). Several agroecological techniques and biological organisms are known to affect Fusarium populations (Jarvis, Citation1989), including sanitation, soil tillage, organic amendments, green manure, biological control, heat-based methods, among others. Each of these techniques is only partly effective, necessitating their combination, which further complicates their adoption in farms where space available under shelters for non-commercial practices is very limited (Collange et al., Citation2014). An effective alternative, without bio-risk, and accepted for the integrated management of Fusarium is the development of resistant genotypes (King et al., Citation2010). To help reduce F. oxysporum wilt in pepper in this region of Mexico, the implementation of breeding programmes needs to be considered. Seed companies and growers should also take into account the susceptibility of the currently grown pepper cultivars in this region to determine which one is the best option to plant in greenhouses where F. oxysporum was detected. Moreover, based on these results, growers need to focus on specific methods for the management of F. oxysporum when dealing with vascular necrosis/wilting of pepper in this region.
Additional information
Funding
References
- Baayen RP, O’Donnell K, Bonants PJM, Cigelnik E, Kroon LPN, Roebroeck RJA, Waalwijk C. 2000. Gene genealogies and AFLP analyses in the Fusarium oxysporum complex identify monophyletic and nonmonophyletic formae speciales causing wilt and rot diseases. Phytopathology. 90:891–900.
- Black LL. 2003. Fusarium wilt. In: Perezny K, Roberts PD, Murphy JF, Goldberg NP, editors. Compendium of pepper diseases. St. Paul (MN): APS Press; p. 14–15.
- Black LL, Green SK, Hartman GL, Poulos JM. 1993. [Pepper diseases: A field guide]. Taipei: Centro asiático de investigación y desarrollo vegetal (AVRDC); p. 24–25. Spanish.
- Boix-Ruíz A, Gálvez-Patón L, De Cara-García M, Palmero-Llamas D, Camacho-Ferre F, Tello-Marquina JC. 2014. Comparison of analytical techniques used to identify tomato-pathogenic strains of Fusarium oxysporum. Phytoparasitica. 43:471–483.
- Cerkauskas RF. 2017. Etiology and management of Fusarium crown and root rot (Fusarium oxysporum) on greenhouses pepper in Ontario, Canada. Can J Plant Pathol. 39:121–132.
- Collange B, Navarrete M, Montfort F, Mateille T, Tavoillot J, Martiny B, Tchamitchian M. 2014. Alternative cropping systems can have contrasting effects on various soil-borne diseases: Relevance of a systemic analysis in vegetable cropping systems. Crop Prot. 55:7–15.
- Dean R, Van Kan JA, Pretorius Z, Hammond-Kosack KE, Di Pietro A, Spanu PD, Rudd JJ, Dickman M, Kahmann R, Ellis J, et al. 2012. The top 10 fungal pathogens in molecular plant pathology. Mol Plant Pathol. 13:414–430.
- Fisher NL, Burgess LW, Toussoun TA, Nelson PE. 1982. Carnation leaves as a substrate and for preserving cultures of Fusarium species. Phytopathology. 72:151–153.
- Food and Agriculture Organization of the United Nations. Statistics Division. [Internet]. [revised 2017 Mar 18; accessed 2017 Mar 18]. Available from: http://faostat3.fao.org/
- Geiser DM, Jimenez-Gasco MM, Kang S, Makalowska I, Veeraraghavan N, Ward TJ, Zhang N, Kuldau GA, O´Donnell K. 2004. FUSARIUM-ID v. 1.0: A DNA sequence database for identifying Fusarium. Eur J Plant Pathol. 110:473–479.
- Goidanich G. 1964. Plant pathology manual. Ed. Edizioni Agricole Bologna. Rome, Italy Italian.
- Gordon TR, Martyn RD. 1997. The evolutionary biology of Fusarium oxysporum. Annu Rev Phytopathol. 35:111–128.
- Govindaswamy CV. 1963. Studies on root-rot diseases of eggplant, chilli and tomato in the Madras State. J Madras Univ. 23:203–230.
- Jarvis WR. 1989. Managing diseases in greenhouse crops. Plant Dis. 73:190–194.
- Joffe AZ, Palti J. 1972. Fusarium species of the Martiella section in Israel. Phytopathol Zeitsch. 73:123–148.
- Jones MM, Black LL. 1992. Response of Capsicum spp. to Fusarium oxysporum f. sp. capsici and VCG characterization of pathogenic isolates. Phytopathology (Oregon), USA. APS Press; p. 1092.
- King SR, Davis AR, Zhang X, Crosby K. 2010. Genetics, breeding and selection of rootstocks for Solanaceae and Cucurbitaceae. Sci Hortic. 127:106–111.
- Kistler HC. 1997. Genetic diversity in the plant-pathogenic fungus Fusarium oxysporum. Phytopathology. 87:474–479.
- Komada H. 1975. Development of a selective medium for quantitative isolation of Fusarium oxysporum from natural soil. Rev Plant Prot Res. 8:114–125.
- Leslie JF, Summerell BA. 2006. The fusarium laboratory manual. Ames (Iowa) Blackwell Publishing; p. 388.
- Lomas-Cano T, Boix-Ruiz A, Palmero-Llamas D, de Cara-García M, Marín-Guirao JI, Palmero-Llamas D, Camacho-Ferre F, Tello-Marquina JC. 2016. Etiological and epidemiological concerns about pepper root and lower stem rot caused by Fusarium oxysporum f. sp. radicis-capsici f. sp. novo. Phytoparasitica. 44:283–293.
- Lomas-Cano T, Palmero-Llamas D, de Cara-García MC, Boix-Ruiz A, Camacho-Ferre F, Tello-Marquina JC. 2014. First report of Fusarium oxysporum of sweet pepper seedlings in Almeria, Spain. Plant Dis. 98:1435.
- Marois JJ, Mitchell DJ. 1981. Effects of fumigation and fungal antagonists on the relationships of inoculum density to infection incidence and disease severity in Fusarium crown rot of tomato. Phytopathology. 71:167–170.
- Michielse CB, Rep M. 2009. Pathogen profile update: Fusarium oxysporum. Mol Plant Pathol. 10:311–324.
- Miller SA, Rowe RC, Riedel RM. 1996. Fusarium and Verticillium wilts of tomato, potato, pepper and eggplant. Columbus (Ohio) The Ohio State Univ. Exten. Fact. HYG-3122-96.
- Moine LM, Labbe C, Louis-Seize G, Seifert KA, Belanger RR. 2014. Identification and detection of Fusarium striatum as a new record of pathogen to greenhouse tomato in northeastern America. Plant Dis. 98:292–298.
- O’Donnell K. 2000. Molecular phylogeny of the Nectria haematococca-Fusarium solani species complex. Mycologia. 92:919–938.
- O’Donnell K, Kistler HC, Cigelnik E, Ploetz RC. 1998. Multiple evolutionary origins of the fungus causing Panama disease of banana: Concordant evidence from nuclear and mitochondrial gene genealogies. Proc Nat Acad Sci USA. 95:2044–2049.
- Ortuño-Martínez A, Parra-Gilabert M, Cánovas-Candel FL, Hernández-Rabay A. 1972. La Fusariosis del pimiento para pimentón e influencia del maneb en el cultivo y producción de esta solanácea. Instituto de Orientación y Asistencia Técnica del Sureste. Murcia (España): Centro de Edafología y Biología Aplicada del Segura.
- Pérez-Hernández A, Serrano-Alonso Y, Aguilar-Pérez ML, Gómez-Uroz R, Gómez-Vázquez J. 2014. Damping-off and root rot of pepper caused by Fusarium oxysporum in Almeria province, Spain. Plant Dis. 98:1159.
- Rabinowitch HD, Cohen R. 2012. Genetics and breeding for resistance and grafting for protection against Fusarium oxysporum wilts. In: Gullino L, Katan J, Garibaldi A, editors. Fusarium wilts of greenhouse vegetable and ornamental crops. Part II. Saint Paul (MN): APS Press.
- Rahin AA, Sharif FM. 1985. A study of pepper wilt in northern Iraq. In: Parker CA, Rovira AD, Moore KJ, Wong PTW, Kollmorgen JF, editors. Ecology and management of soilborne plant pathogens. Saint Paul (MN): APS Press; p. 59–62.
- Retes-Manjarrez JE, Hernández-Verdugo S, Pariaud B, Melgoza-Villagómez CM, Pacheco-Olvera A, Parra-Terraza S, Garzón-Tiznado JA. 2016. [Detection of pepper huasteco yellow vein virus resistance and heritability in wild genotypes of Capsicum annuum L.]. Interciencia. 41:541–547. Spanish.
- Rivelli VC. 1989. A wilt of pepper incited by Fusarium oxysporum f. sp. capsici formae specialis nova. [ M. Sc. dissertation]. Baton Rouge (Louisiana) Louisiana State University.
- SAS. 1999. SAS/STAT. User’s guide. Rel. 6.03. Cary (NC EEUU): SAS Institute Inc; p. 1028.
- Secretaría de Agricultura, Ganadería, Desarrollo Rural, Pesca y Alimentación. Servicio de Información Agroalimentaria y Pesquera, México.[Internet.]. [revised 2017 Mar 4; accessed 2017 Mar 4]. Available from: www.siap.gob.mx
- Snyder WC, Hansen HN. 1940. The species concept in Fusarium. Am J Bot. 27:64–67.
- Tamura K, Peterson D, Peterson N, Stecher G, Nei M, Kumar S. 2011. MEGA 5: Molecular evolutionary genetics analysis using maximum likelihood, evolutionary distance, and maximum parsimony methods. Mol Biol Evol. 28:2731–2739.
- Thompson JD, Higgins DG, Gibson TJ. 1994. CLUSTAL W: Improving the sensitivity of progressive multiple sequence alignment through sequence weighting, position-specific gap penalties and weight matrix choice. Nucl Acids Res. 22:4673–4680.
- Velarde-Félix S, Ortega-Murrieta PF, Fierros-Leyva GA, Padilla-Valenzuela I, Gutiérres-Pérez E, Rodríguez-Cota FG, López-Valenzuela JA, Acosta-Gallegos JA, Garzón-Tiznado JA. 2015. [Molecular and biological identification of races 0 and 5 of Fusarium oxysporum Schlechtend: Fr f. sp. ciceris (Padwick) Matuo and K. Sato of chickpea in the Northwest of Mexico]. REMEXCA. 6:735–748. Spanish.
- Velásquez-Valle R, Medina-Aguilar MM, Luna-Ruiz JJ. 2001. [Symptomatology and genera of pathogens associated with root rot of chili (Capsicum annuum L.) in North-Central Mexico]. Rev Mex Fitopatol. 19:175–181. Spanish.
- Vincenlli O, Tisserat N. 2008. Nucleic acid-based pathogen detection in applied plant pathology. Plant Dis. 92:660–669.
- Zasada IA, Halbrendt JM, Kokalis-Burelle N, LaMondia J, McKenry MV, Noling JW. 2010. Managing nematodes without methyl bromide. Annu Rev Phytopathol. 48:311–328.