Abstract
Phytophthora sojae Kaufmann & Gerdemann has been a yield limiting factor for more than 60 years on soybean (Glycine max L.) in Ohio and other regions where soils are poorly drained. Soybean is the primary host, although numerous Lupinus spp. have been shown to be infected based on artificial inoculations. More than 20 different major resistance (Rps) genes have been reported from sources from China, Japan and South Korea but few are deployed in cultivars. As in other host-pathogen systems with a gene-for-gene interaction, there are also numerous pathotypes (races) of P. sojae, with as few as 1 to more than 50 characterized within a single field. Due to high pathogen diversity and complexity in relation to pathotype, quantitative disease resistance (QDR) or partial resistance has become a priority and serves as the backbone for disease management in some production regions. However, QDR requires the use of seed treatments for early season protection in highly favourable environments. Current research efforts for the development of more durable management of this disease include the identification and characterization of novel Rps genes as well as markers and mechanisms that contribute to the expression of QDR. There are now two new seed treatment chemistries, ethaboxam and oxathiapiprolin, with efficacy towards P. sojae in addition to metalaxyl-based fungicides. Finally, pathogen population structure, both within and between production regions, as well as variability within Avr loci of P. sojae, are also elucidating potential new approaches to managing this disease.
Résumé
Depuis plus de 60 ans, Phytophthora sojae Kaufmann & Gerdemann est un facteur qui limite les rendements du soja (Glycine max L.) en Ohio et dans d’autres régions où les terres sont mal drainées. Le soja en est l’hôte primaire, mais, en se référant à des inoculations artificielles, il peut également infecter de nombreuses plantes du genre Lupinus. On rapporte qu’il existe plus de 20 gènes majeurs de résistance (Rps) en Chine, au Japon et en Corée du Sud, mais peu sont utilisés dans des cultivars. Comme dans le cas des autres systèmes hôte-agent pathogène dans lesquels il existe des interactions gène pour gène, il existe également plusieurs pathotypes (races) de P. sojae, parfois aussi peu que 1, et ce, jusqu’à plus de 50 caractérisés dans un même champ. Étant donné la grande diversité des agents pathogènes et l’extrême complexité relative au pathotype, le caractère quantitatif de résistance (CQR), ou résistance partielle, est devenu une priorité et sert de base à la gestion des maladies dans certaines régions productrices. Toutefois, le CQR requiert l’utilisation de traitements des semences dans les environnements à haut risque afin de les protéger tôt dans la saison. Les efforts de recherche actuels axés sur le développement de modes de gestion plus durables de cette maladie incluent l’identification et la caractérisation de nouveaux gènes Rps ainsi que de marqueurs et de mécanismes qui contribuent à l’expression du CQR. Il y a maintenant, en plus des fongicides à base de métalaxyl, deux nouveaux produits chimiques efficaces pour traiter les semences contre P. sojae: l’éthaboxam et l’oxathiapiproline. Finalement, la structure des populations d’agents pathogènes, dans et entre les régions productrices, ainsi que la variabilité au sein des locus Avr de P. sojae élucident les nouvelles approches possibles visant à gérer cette maladie.
Introduction
Phytophthora sojae Kauffmann and Gerdemann is one of the many soilborne oomycete (watermould) pathogens that can infect soybean from planting to harvest. There have been numerous surveys conducted over the past 60 or more years which have documented this pathogen’s distribution within and throughout the soybean production regions of the world (Kaufmann & Gerdemann, Citation1958; Miller et al., Citation1997; Ryley et al., Citation1998; Workneh et al., Citation1999; Grau et al., Citation2004; Sugimoto et al., Citation2006; Dorrance & Grünwald, Citation2009; Zhang et al., Citation2010; Dorrance, Citation2013). Yield losses can reach 100% if susceptible cultivars are planted in fields which have a history of this disease and are poorly drained. Annually, from 1996 to 2014, approximately 20.5 million metric ton losses were attributed to phytophthora root and stem rot with an average annual loss of over 1.1 million metric tons (Wrather et al., Citation2001; Wrather & Koenning, Citation2006, Citation2009; Koenning & Wrather, Citation2010; Allen et al., Citation2017). In addition to P. sojae, Phytophthora sansomeana E.M. Hansen & Reeser, is also recognized as contributing to overall root rot in soybean (Reeser et al., Citation1991; Malvick & Grunden, Citation2004; Rojas et al., Citation2017). This species, once classified with the Phytophthora megasperma complex, can also infect a wider range of hosts, including corn (Zelaya-Molina et al., Citation2010), Douglas fir, clover and wild carrot (Hansen et al., Citation2009). This species was isolated in previous surveys in the north-central region of the USA as a fast-growing Phytophthora sp. on potato dextrose agar, that was race non-classifiable (Reeser et al., Citation1991) and homothallic with large oospores (Malvick & Grunden, Citation2004).
This review will summarize the results from studies with P. sojae which focused on population genetic and pathotype diversity, potential forces driving this diversity, types of disease resistance, implications for resistance management, and an integrated systems approach to mitigating losses for soybean farmers.
Phytophthora sojae basics
Soybean is the primary host of P. sojae (reviewed in Erwin & Ribeiro, Citation1996), and early season symptoms include seed and seedling damping-off (, ). These symptoms, brown lesions and collapsing tissue, are indistinguishable from those caused by other plant pathogens belonging to the Oomycetes. Later season symptom development is directly related to the level of genetic resistance present in the cultivar. Cultivars with higher susceptibility will develop a greater amount of root rot as well as a chocolate brown stem canker from the base of the plant extending up the stem. There are instances where this canker will be limited to one side of the plant. The severely affected plants will appear wilted, the foliage will turn yellow and plants will die prematurely ().
Fig. 1 (Colour online) Phytophthora sojae infection of susceptible cultivars during the growing season. (a, b) Early season symptoms include seed rot or skips in the rows and seedling damping-off. (c) Symptoms of late season infections have the characteristic chocolate-brown stem canker combined with overall wilting and early plant death. (d) In both early and late season infections, oospores will develop in the tissue which allow long-term survival in the soil.
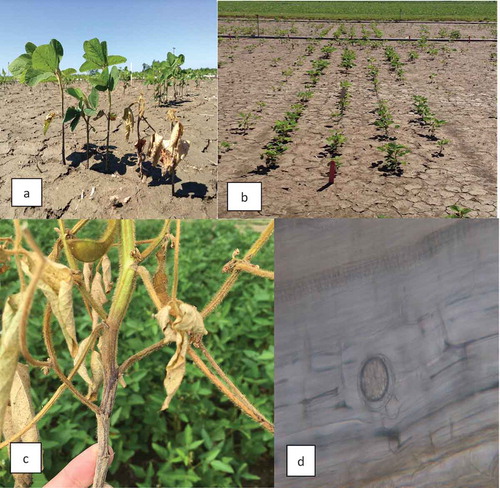
Phytophthora sojae is a homothallic or self-fertile oomycete species. It survives in the soil as oospores, which are thick double-walled structures (). When soils are flooded, non-dehiscent, lemon-shaped sporangia with long pedicels develop. The sporangia are non-papillate and numerous zoospores will form inside the structure. These are released into the soil water, and rely on chemotaxis to locate soybean roots (Morris & Ward, Citation1992). Differences in attraction or specificity towards compounds by different strains of P. sojae have been documented (Tyler et al., Citation1996). Once the zoospore reaches the root, it encysts, germinates and directly invades the root. Depending on the level and type of host resistance, the mycelium then colonizes the intercellular space, forming haustoria-like bodies in the neighbouring cells during the biotrophic phase of colonization. Oospores will form throughout the colonized tissue, often before the necrotrophic phase (). Oospores are believed to survive in soil for a very long period of time and do not germinate all at the same time (Schmitthenner, Citation1985).
This pathogen has been studied extensively, and considering the losses that were reported during the 1960s, has been managed effectively with a combination of host resistance, seed treatments and improved drainage. As in many host–pathogen systems with a limited host range, there are numerous R-gene mediated or Rps (Resistance Phytophthora sojae) genes in soybean for P. sojae as well as quantitative disease resistance (). Most of the Rps genes have an immune type response or a very strong resistance response that is expressed in the seed, root, hypocotyl and cotyledon of soybean. Few Rps genes, Rps1a, Rps1b, Rps1c and Rps1k, and to some extent Rps3a and Rps6 (Dorrance unpublished data; Robertson et al., Citation2009; Slaminko et al., Citation2010) have been deployed over Canadian and US production regions.
Diversity of pathotypes and avr genes
Initial surveys of pathotype diversity of P. sojae were conducted during the late 1960s to mid-1980s in Ontario, Canada and the USA. These surveys identified simple pathotypes, where the isolates recovered could only cause disease on plants with one or two Rps genes (, Grau et al., Citation2004). However, this began to change in the early 2000s as isolates with a greater degree of complexity began to emerge (Kaitany et al., Citation2001; Dorrance et al., Citation2003a; Jackson et al., Citation2004; Malvick & Grunden, Citation2004; Nelson et al., Citation2008). In addition, virulence to some Rps genes was maintained in the population, which indicates that recycling of Rps genes may not be an option (, Dorrance et al., Citation2016). For example, there are more populations across the north-central region in the USA that have virulence towards Rps1a, one of the first Rps-genes to be widely deployed in cultivars (Schmitthenner, Citation1985; Dorrance et al., Citation2016).
Table 1. Predominant virulencea towards resistance genes for Phytophthora sojae (Rps genes) in soybean over the decades.
In addition to the increasing complexity, there is also pathotype variability among different soybean productions regions in the USA (Jackson et al., Citation2004; Nelson et al., Citation2008; Dorrance et al., Citation2016) and Ontario (Xue et al., Citation2015). For example, Rps3a was deployed in only a few cultivars, but 40% of the isolates from Illinois had virulence towards this gene, while the same was true for less than 20% of the isolates recovered from Iowa, Indiana, Michigan, Minnesota, Nebraska, New York, Ohio and South Dakota during the same survey (Dorrance et al., Citation2016). In this same study, similar trends were reported for Rps1b, Rps1c and Rps6.
The effectors or Avr genes that trigger the R-gene defence response have been well studied in the P. sojae–soybean system, and to date all have a RXLR (arginine-x-leucine-arginine) protein motif (Jiang et al., Citation2008; Anderson et al., Citation2015). Numerous Avr genes in P. sojae have been cloned including: Avr1a (Qutob et al., Citation2009; Na et al., Citation2014); Avr1b-1 (Shan et al., Citation2004; Dou et al., Citation2008); Avr1c (Na et al., Citation2014); Avr1d (Na et al., Citation2013; Yin et al., Citation2013); Avr1k (Song et al., Citation2013); Avr3a/5 (Qutob et al., Citation2009; Dong et al., Citation2011b); Avr3b (Dong et al., Citation2011a); Avr3c (Dong et al., Citation2009); and Avr4/6 (Dou et al., Citation2010). Based on the isolates used to clone and sequence these genes, there have been several interesting findings. First, the same Avr gene may be detected by more than one R-gene as is the case for Avr1b-1, Avr3a/5 and Avr4/6 (Shan et al., Citation2004; Dou et al., Citation2010; Dong et al., Citation2011b). Some of the Avr genes are clustered in a locus such as Avr1b-1, Avr1k (Song et al., Citation2013) and Avr1a, Avr1c (Na et al., Citation2014). Several, Avr1a, Avr3a (Qutob et al., Citation2009), and Avr3c (Dong et al., Citation2009) have multiple copies within a locus. For most, deletions, and/or changes in transcription or in sequence as well as epigenetic silencing have resulted in virulence on the corresponding Rps gene (Dong et al., Citation2009; Qutob et al., Citation2009, Citation2013; Cui et al., Citation2012; Song et al., Citation2013; Na et al., Citation2014). Thus, several proven and hypothesized strategies were summarized by Na & Gijzen (Citation2016) for this pathogen to survive or reduce the chance of detection by Rps genes including: P. sojae Avr genes are positioned in regions with highly repetitive sequences as well as rich in transposons, which enhances the potential for genetically driven modifications; changes in expression through epigenetic switches; and potential interactions with small RNA. The functional analysis of these and other effectors that play a large role in establishing a successful invasion will continue to lead to a greater understanding of this host–pathogen system. Additionally, it will lead to potential novel strategies for identifying mechanisms of resistance and, more importantly, resistance deployment.
In addition to the characterization of the pathotype diversity that now exists and the number of different genetic mechanisms that contribute to that diversity, the genotypic diversity of P. sojae is much higher than expected for a soilborne organism (Stewart et al., Citation2016). In a comparison of single isolates collected from individual fields in Iowa, Ohio and South Dakota, there were 102 multi-locus genotypes (MLG) among the 107 isolates of P. sojae. Additionally, each isolate collected within the same field also had a unique MLG. Interestingly, isolates of P. sojae collected from two neighbouring states, Iowa and South Dakota, represented two very different populations. There is currently work in progress to define the size of these regional populations and to gain a deeper understanding of this diversity and how it may impact deployment of resistance. In the short term, this information will be used to better inform isolate selection for resistance characterization.
Management of Phytophthora sojae: resistance
Rps genes
Soybean is rich in sources of resistance towards P. sojae for Rps genes, which have an immune type of response. However, few have been fully characterized and deployed (Dorrance & Schmitthenner, Citation2000; Lohnes et al., Citation1996; Kyle et al., Citation1998; Jia, Citation2003; Huang et al., Citation2016; Matthiesen et al., Citation2016). Among the more than 20 Rps genes that have been identified, most map to Chromosome 3 including: Rps1a, 1b, 1c, 1d, 1k, 7, 9, UD25, Yu25, UN1, YD29, Q, HN and WY (Anderson & Buzzell, Citation1992b; Lohnes & Schmitthenner, Citation1997; Weng et al., Citation2001; Fan et al., Citation2009; Sugimoto et al., Citation2011; Sun et al., Citation2011; Wu et al., Citation2011; Lin et al., Citation2013; Zhang et al., Citation2013; Li et al., Citation2016, Citation2017; Cheng et al., Citation2017; Niu et al., Citation2017). Based on early analysis, many of the recently identified sources of R-genes, including potential novel Rps genes, were segregating for more than one resistance gene (Gordon et al., Citation2007a, Citation2007b; Lin et al., Citation2013; Li et al., Citation2016; Sahoo et al., Citation2017).
The primary questions for all of these Rps genes will be: is the Rps gene effective towards all of the populations or limited to specific regions? More importantly, how long will they last? Historically, Rps genes 1d, 2, 3b, 3c, 4, 5 and 7 were not commercially deployed as populations were already present that could successfully cause disease on these plants (Abney et al., Citation1997). Similar questions have arisen with Rps8 (Gordon et al., Citation2006) as this gene is not effective towards populations of P. sojae from Missouri, but is effective towards populations in more northern regions (Dorrance et al., Citation2016). A mechanism for evaluating the efficacy of putative novel Rps genes is needed prior to the expense of breeding them into adapted high-yielding cultivars. Simultaneously, a new differential set is needed to monitor development of virulence to these novel Rps genes in the future.
Quantitative resistance
The high level of genotype and pathotype diversity in regional populations of P. sojae, along with Rps gene-mediated resistance that is not effective towards all populations, raises the importance of quantitative disease resistance (QDR) for managing this soilborne pathogen. In the P. sojae–soybean system, QDR is expressed as an incomplete resistance with lower levels of root rot and the absence of stem rot in cultivars with high levels of resistance (Tooley & Grau, Citation1984; Schmitthenner, Citation1985; Mideros et al., Citation2007). Quantitative disease resistance, also called partial resistance, tolerance and field resistance in the soybean–P. sojae system, has been shown to provide protection when diverse pathotypes are present (Dorrance et al., Citation2003b) and does not limit yield (St. Martin et al., Citation1994). This resistance can be expressed and measured by decreased infection efficiency (fewer successful lesions develop for the same amount of zoospore inoculum), reduced lesion size and fewer oospores produced within the tissue (Mideros et al., Citation2007). Overall, this resistance can be measured via lesion length development in a tray test, growing through an inoculum layer in a greenhouse assay, as well as by field evaluations (Tooley & Grau, Citation1984; Burnham et al., Citation2003; Han et al., Citation2008; Li et al., Citation2010; Stewart & Robertson, Citation2012; Wang et al., Citation2012a). However, as Wang et al. (Citation2012a) demonstrated, different quantitative trait loci (QTL) in the same population may be identified based on the methodology.
Similar to the number of sources of resistance with Rps genes, large numbers of plant introductions and cultivars with high levels of partial resistance to P. sojae have also been identified (Dorrance & Schmitthenner, Citation2000; Schneider et al., Citation2016). Numerous populations have been mapped and QTL identified from ‘Conrad’ (Burnham et al., Citation2003; Han et al., Citation2008; Li et al., Citation2010; Wang et al., Citation2010; Cui et al., Citation2012; Stasko et al., Citation2016); ‘V71-370‘ (Tucker et al., Citation2010); PIs 398 841, 398 297, 407861A, 417 178, 427105B, 427 106 (Lee et al., Citation2013a, Citation2013b, Citation2014); PI 408105A (Nguyen et al., Citation2012); and PI 399 036 (Abeysekara et al., Citation2016). In these mapping studies, numerous loci were identified across the soybean genome (), the resistance was moderately to highly heritable, with some populations having several minor QTL, each contributing a small proportion to the overall resistance phenotype, while others with major QTL where one locus contributed more than 20% of the phenotypic variance (Tucker et al., Citation2010; Wang et al., Citation2010; Nguyen et al., Citation2012; Lee et al., Citation2013a, Citation2013b, Citation2014; Stasko et al., Citation2016). In addition, Schneider et al. (Citation2016) evaluated more than 790 plant introductions, and identified 16 SNP markers in a genome-wide association analysis that were associated with root rot score and final root weight. The majority of the plant introductions had very high levels of resistance. However, there were more than 100 removed from the final analysis as they had no visible root rot, indicative of an R-gene response.
Fig. 2 (Colour online) Soybean yield comparison of moderately susceptible cultivar ‘Sloan’, high levels of partial resistance ‘Conrad’, and combination of high partial plus 2 Rps gene at two locations in Ohio during 2011 with moderate and high disease pressure from Phytophthora sojae.
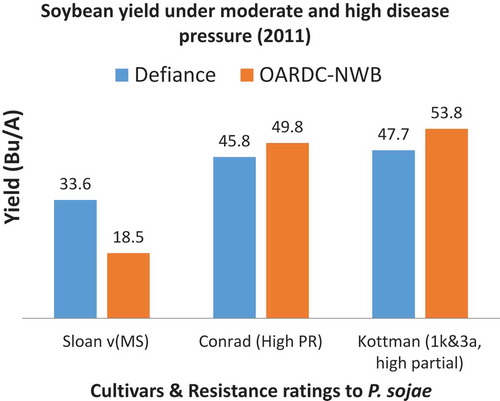
Fig. 3 (Colour online) Genomic regions on the soybean genome for Quantitative Disease Resistance Loci towards Phytophthora sojae from nine different recombinant inbred line populations (Tucker et al., Citation2010; Nguyen et al., Citation2012; Wang et al., Citation2012b; Lee et al., Citation2013a, Citation2013b, Citation2014). Closed or solid colours indicate the QDRL is conferred from the resistant parent, open is from the susceptible parent.
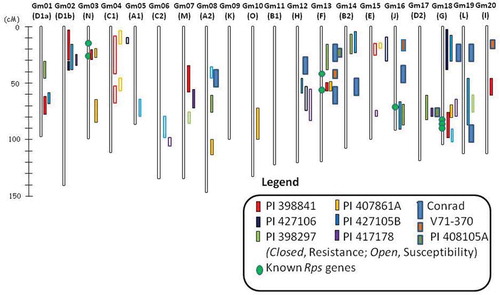
Fig. 4 (Colour online) Field evaluations of seed treatment fungicides and rates of mefenoxam and ethaboxam for efficacy towards Phytophthora sojae in 2012. These types of studies require saturated soil conditions, either naturally or through irrigation, shortly after planting. Efficacy can be measured as yield using cultivars that are moderately susceptible or with high levels of partial resistance. In this study, the moderately susceptible cultivar had a yield response with a seed treatment (P < 0.0001) and bars with letters that are the same indicate that those treatments were not significantly different based on Fisher’s protected least significant difference.
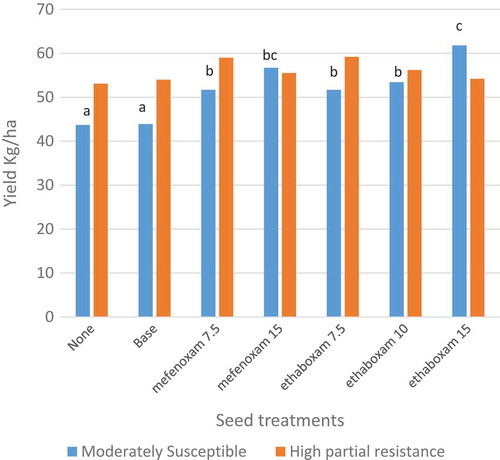
The current emphasis with these plant populations is to identify the mechanisms that contribute to the expression of this resistance. The soybean cultivar ‘Conrad’ has been the most studied to date, with several different mechanisms contributing to the overall resistance response, including: suberin (Thomas et al., Citation2007; Ranathunge et al., Citation2008), auxin-related susceptibility, potentially defeated Rps genes and signal transduction, among other mechanisms (Wang et al., Citation2012b). In a study that explored the overall gene expression response with the Affymetrix GeneChip, 89% of the genes had a significant change in expression five days after inoculation (Zhou et al., Citation2009). When these data were compared with the mapped QTL regions associated with resistance in ‘Conrad’, 76% of the genes within one of the QTL had a differential changes in expression following inoculation. Moreover, when sequences were compared of individual genes between ‘Conrad’ and susceptible parent, there was a higher proportion of SNPs in the upstream, intron and downstream regulatory regions, indicative of sites for regulatory control by the host or pathogen (Wang et al., Citation2012b).
To date, these data and those from current studies indicate that quantitative resistance is a complex network that potentially encompasses several mechanisms interacting independently or in a very tightly regulated fashion. Ultimately, breeding companies need to know not just the marker associated with the trait, but which allele for each of the suite of genes is most important to ensure the highest level of resistance is expressed once the pathogen is encountered in the field.
Management of Phytophthora sojae: fungicide seed treatments
The Rps genes in soybean for P. sojae are effective at the time of planting; however, quantitative resistance is not (Dorrance & McClure, Citation2001). Fungicide seed treatments have increased both plant stands and yield over numerous studies when environmental conditions are conducive for disease development (; Dorrance et al., 2004; Bradley, Citation2008; Dorrance et al., Citation2009). More importantly, there were significant differences between non-treated seed and treated seed for both moderately susceptible and moderately resistant cultivars evaluated in high disease environments (). This is important for disease management, which indicates all cultivars can benefit from the seed treatment if the risk of disease is high. In these regional comparisons, at locations where there was little to no disease pressure, the differences among the treatments were not significant (Dorrance et al., Citation2009).
Historically, metalaxyl and mefenoxam have been used as the primary seed treatments to protect soybean against watermoulds, including both Phytophthora sojae and Pythium spp. There are now two additional fungicide seed treatments available – ethaboxam (Dorrance et al., Citation2012; Radmer et al., Citation2017) and oxathiapiprolin (Miao et al., Citation2016; Vargas et al., unpublished data), that have efficacy towards P. sojae. Farmers who produce soybeans in these high disease-risk environments should ensure that at least one of these products is in their seed treatment mix.
Management of Phytophthora sojae: cultural practices
Phytophthora sojae and other watermoulds require free water and saturated soil conditions for zoospore development and movement towards the host. Avoiding planting prior to predicted heavy storms where flooding or saturated soils will occur, compaction of soil and improving drainage have all been recommended. Schmitthenner & Van Doren (Citation1985) demonstrated the effects of tiling and tillage on the severity of P. sojae on cultivars with an effective Rps gene, and low to moderate levels of partial resistance. The yield of the cultivar with an effective Rps gene did not change over any of the treatments. However, the yield of the moderately susceptible cultivar did improve with both tiling and tillage. These practices shorten the time that the soil is saturated. No-till or reduced tillage systems favour the build-up of inoculum in the seed bed (Workneh et al., Citation1998). This was based on soil cores that were collected and separated by depth into sections. Each soil core was then baited for the presence of P. sojae, and the highest densities came from those cores near the top of the soil profile. Interestingly, crop rotation has never been recommended as a management strategy as oospores survive for long periods of time (Schmitthenner, Citation1985).
Conclusions
Phytophthora sojae is one of the great success stories regarding the use of host resistance for disease management. If effective Rps genes are available, they will provide complete protection. However, as in many other host–pathogen systems, the response of the genes is ephemeral and additional tactics, such as combination with quantitative resistance and seed treatment fungicides, may be needed when there is a high risk for disease development. Prior to the deployment of cultivars with Rps1k, there was a greater interest in quantitative resistance (Tooley & Grau, Citation1984; Schmitthenner, Citation1985). The cultivars ‘Resnik’ and ‘Williams82’ have Rps1k combined with high to moderate levels of quantitative resistance (Bernard & Cremeens, Citation1988; McBlain et al., Citation1990). This most likely played a large role in the longer life of Rps1k (~20 years) compared with Rps1a which was not effective shortly after it was deployed (Schmitthenner, Citation1985). As phytophthora root and stem rot develops in the newly expanded regions of soybean production, hopefully the lessons from the past will minimize the losses for the future.
Acknowledgements
I would like to thank the organizers of this symposium for the honour to speak at the Canadian Phytopathological Society annual meeting in 2017. I am also deeply appreciative of the many students, post-docs and collaborators that I have worked with over the past 20 years who have contributed to much of the work cited in this review. Lastly, the soybean check-off, without whose support from Ohio Soybean Council, North Central Soybean Research Program and United Soybean Board, much of this work would not have been possible.
References
- Abeysekara NS, Matthiesen RL, Cianzio SR, Bhattacharyya MK, Robertson AE. 2016. Novel sources of partial resistance against Phytophthora sojae in soybean PI 399036. Crop Sci. 56:2322–2335.
- Abney TS, Melgar JC, Richards TL, Scott DH, Grogan J, Young J. 1997. New races of Phytophthora sojae with Rps1-d virulence. Plant Dis. 81:653–655.
- Allen TW, Bradley CA, Sisson AJ, Byamukama E, Chilvers MI, Coker CM, Collins AA, Damicone JP, Dorrance AE, Dufault NS, et al. 2017. Soybean yield loss estimates due to diseases in the United States and Ontario, Canada, from 2010 to 2014. Plant Health Progress. 18:19–27.
- Anderson RG, Devdutta D, Fedkenheuer K, McDowell JM. 2015. Recent progress in RXLR effector research. Mol Plant-Microbe Interact. 10:1063–1072.
- Anderson TR, Buzzell RI. 1992a. Diversity and frequency of races of Phytophthora megasperma f. sp. glycinea in soybean fields in Essex County, Ontario, 1980–1989. Plant Dis. 76:587–589.
- Anderson TR, Buzzell RI. 1992b. Inheritance and linkage of the Rps7 gene for resistance to Phytophthora rot of soybean. Plant Dis. 76:958–959.
- Bernard RL, Cremeens CR. 1988. Registration of ‘Williams 82ʹ soybean. Crop Sci. 28:1027.
- Bradley CA. 2008. Effect of fungicide seed treatments on stand establishment, seedling disease, and yield of soybean in North Dakota. Plant Dis. 92:120–125.
- Burnham KD, Dorrance AE, VanToai T, St. Martin SK. 2003. Quantitative trait loci for partial resistance to Phytophthora sojae in soybean. Crop Sci. 43:1610–1617.
- Cheng Y, Ma Q, Ren H, Xia Q, Song E, Tan Z, Li S, Zhang G, Nian H. 2017. Fine mapping of a Phytophthora resistance gene RpsWY in soybean (Glycine max L.) by high throughput genome-wide sequencing. Theor Appl Genet. 130:1041–1051.
- Cui L, Yin W, Dong S, Wang Y. 2012. Analysis of polymorphism and transcription of the effector gene Avr1b in Phytophthora sojae isolates from China virulent to Rps1b. Mol Plant Pathol. 13:114–122.
- Dong S, Qutob D, Tedman-Jones J, Kuflu K, Wang Y, Tyler BM, Gijzen M. 2009. The Phytophthora sojae avirulence locus Avr3c encodes a multi-copy RXLR effector with sequence polymorphisms among pathogen strains. PLoS ONE. 4:e5556.
- Dong S, Yin W, Kong G, Yang X, Qutob D, Chen Q, Kale SD, Sui Y, Zhang Z, Dou C, et al. 2011a. Phytophthora sojae avirulence effector Avr3b is a secreted NADH and ADP-ribose pyrophosphorylase that modulates plant immunity. PLoS Pathog. 7:e1002353.
- Dong S, Yu D, Cui L, Qutob D, Tedman-Jones J, Kale SD, Tyler BM, Wang Y, Gijzen M. 2011b. Sequence variants of the Phytophthora sojae RXLR effector Avr3a/5 are differentially recognized by Rps3a and Rps5 in soybean. PLoS ONE. 6:e20172.
- Dorrance AE. 2013. Phytophthora sojae on soybean. In: Lamour K, editor. Phytophthora: A global perspective. Boston (MA): CABI. CABI Plant Protection Series, 2; p. 79–86.
- Dorrance AE, Ellis ML, McDuffee D, Arthur K. 2012. Efficacy of ethaboxam toward species of Phytophthora and Pythium. Phytopathology. 102:S4–31.
- Dorrance AE, Grünwald NJ. 2009. Phytophthora sojae: Diversity among and within populations. In: Lamour K, Kamoun S, editors. Oomycete genetics and genomics: Biology interactions with plant and animals and toolbox. Hoboken (NJ): John Wiley & Sons, Inc. Chapter 10; p. 197–212.
- Dorrance AE, Kurle J, Robertson AE, Bradley CA, Giesler L, Wise K, Concibido VC. 2016. Pathotype diversity of Phytophthora sojae in eleven states in the United States. Plant Dis. 100:1429–1437.
- Dorrance AE, McClure SA. 2001. Beneficial effects of fungicide seed treatments for soybean cultivars with partial resistance to Phytophthora sojae. Plant Dis. 85:1063–1068.
- Dorrance AE, McClure SA, deSilva A. 2003a. Pathogenic diversity of Phytophthora sojae in Ohio soybean production fields. Plant Dis. 87:139–146.
- Dorrance AE, McClure SA, St. Martin SK. 2003b. Effect of partial resistance on Phytophthora stem rot incidence and yield of soybean in Ohio. Plant Dis. 87:308–312.
- Dorrance AE, Meharg C, Anderson TR, Berry SA. 2008. Isolation, storage, pathotype characterization, and evaluation of resistance for Phytophthora sojae in soybean. Plant Health Progress. doi:10.1094/PHP-2008-0118-01-DG
- Dorrance AE, Robertson AE, Cianzo S, Giesler LJ, Grau CR, Draper MA, Tenuta AU, Anderson TR. 2009. Integrated management strategies for Phytophthora sojae combining host resistance and seed treatments. Plant Dis. 93:875–882.
- Dorrance AE, Schmitthenner AF. 2000. New sources of resistance to Phytophthora sojae in the soybean plant introductions. Plant Dis. 84:1303–1308.
- Dou D, Kale SD, Liu T, Tang Q, Wang X, Arredondo FD, Basnayake S, Whisson S, Drenth A, MacLean D, et al. 2010. Different domains of Phytophthora sojae effector Avr4/6 are recognized by soybean resistance genes Rps4 and Rps6. Mol Plant-Microbe Interact. 23:425–435.
- Dou D, Kale SD, Wang X, Chen Y, Wang Q, Wang X, Jiang RH, Arredondo FD, Anderson RG, Thakur PB, et al. 2008. Conserved C-terminal motifs required for avirulence and suppression of cell death by Phytophthora sojae effector Avr1b. Plant Cell. 20:1118–1133.
- Erwin DC, Ribeiro OK. 1996. Phytophthora diseases worldwide. St. Paul (MN): APS Press; p. 436–440.
- Fan AY, Wang XM, Fang XP, Wu XF, Zhu ZD. 2009. Molecular identification of Phytophthora resistance gene in soybean cultivar Yudou 25. Acta Agron Sin. 35:1844–1850.
- Gordon SG, Berry SA, St. Martin SK, Dorrance AE. 2007a. Genetic analysis of soybean plant introductions with resistance to Phytophthora sojae. Phytopathology. 97:106–112.
- Gordon SG, Kowitwanich K, Pipatpongpinyo W, St. Martin SK, Dorrance AE. 2007b. Molecular marker analysis of soybean plant introductions with resistance to Phytophthora sojae. Phytopathology. 97:113–118.
- Gordon SG, St Martin SK, Dorrance AE. 2006. Rps8 maps to a resistance gene rich region on soybean molecular linkage group F. Crop Sci. 46:168–173.
- Grau CR, Dorrance AE, Bond J, Russin J. 2004. Fungal diseases. In: Boerma HR, Specht JE, editors. Soybeans: Improvement, production and uses. 3rd ed. Madison (WI): Agron. Monographs 16. ASA, CSSA, and SSSA; p. 679–763.
- Han Y, Teng W, Yu K, Poysa V, Anderson T, Qiu L, Lightfoot DA, Li W. 2008. Mapping QTL tolerance to Phytophthora root rot in soybean using microsattelite and RAPD/SCAR derived markers. Euphytica. 162:231–239.
- Hansen EM, Wilcox WF, Reeser PW, Sutton W. 2009. Phytophthora rosacearum and P. sansomeana, new species segregated from the P. megasperma “complex”. Mycologia. 101:129–135.
- Huang J, Guo N, Li Y, Sun J, Hu G, Zhang H, Li Y, Zhang X, Zhao J, Xing H, et al. 2016. Phenotypic evaluation and genetic dissection of resistance to Phytophthora sojae in the Chinese soybean mini core collection. BMC Genetics. 17:85.
- Jackson TA, Kirkpatrick TL, Rupe JC. 2004. Races of Phytophthora sojae in Arkansas soybean fields and their effects on commonly grown soybean cultivars. Plant Dis. 88:345–351.
- Jia H 2003. An evaluation of protocols for stabilizing pathotypes of Phytophthora sojae isolates and characterizing race specific resistant genes in soybean plant introductions [M.S. Thesis]. Columbus: The Ohio State University.
- Jiang RH, Tripathy S, Govers F, Tyler BM. 2008. RXLR effector reservoir in two Phytophthora species is dominated by a single rapidly evolving superfamily with more than 700 members. Proc Natl Acad Sci USA. 105:4874–4879.
- Kaitany RC, Hart LP, Safir GR. 2001. Virulence composition of Phytophthora sojae in Michigan. Plant Dis. 85:1103–1106.
- Kaufmann MJ, Gerdemann JW. 1958. Root and stem rot soybean caused by Phytophthora sojae n. sp. Phytopathology. 48:201–208.
- Koenning SR, Wrather JA. 2010. Suppression of soybean yield potential in the continental United States by plant diseases from 2006 to 2009. Plant Health Progress. doi:10.1094/PHP-2010-1122-01-RS
- Kyle DE, Nickell CD, Nelson RL, Pedersen WL. 1998. Response of soybean accessions from provinces in southern China to Phytophthora sojae. Plant Dis. 82:555–559.
- Lee S, Mian RMA, McHale L, Sneller CH, Dorrance AE. 2013a. Identification of quantitative trait loci conditioning partial resistance to Phytophthora sojae in soybean PI 407861A. Crop Sci. 53:1022–1031.
- Lee S, Mian RMA, McHale L, Wang H, Wijeratne A, Sneller C, Dorrance AE. 2013b. Novel quantitative trait loci for partial resistance to Phytophthora sojae in soybean PI 398841. Theor Appl Genet. 126:1121–1132.
- Lee S, Mian RMA, Sneller CH, Wang H, Dorrance AE, McHale L. 2014. Joint linkage QTL analysis for partial resistance to Phytophthora sojae using six nested inbred populations with heterogeneous conditions. Theor Appl Genet. 127:429–444.
- Li L, Lin F, Wang W, Ping J, Fitzgerald JC, Zhao M, Li S, Sun L, Cai C, Ma J. 2016. Fine mapping and candidate gene analysis of two loci conferring resistance to Phytophthora sojae in soybean. Theor Appl Genet. 129:2379–2386.
- Li X, Han Y, Teng W, Zhang S, Yu K, Poysa V, Anderson T, Ding J, Li W. 2010. Pyramided QTL underlying tolerance to Phytophthora root rot in mega-environments from soybean cultivars ‘Conrad’ and ‘Hefeng 25ʹ. Theor Appl Genet. 121:651–658.
- Li Y, Sun S, Zhong C, Wang X, Wu X, Zhendong Z. 2017. Genetic mapping and development of co-segregating markers of RpsQ, which provides resistance to Phytophthora sojae in soybean. Theor Appl Genet. 130:1223–1233.
- Lin F, Zhao M, Ping J, Johnson A, Zhang B, Abney TS, Hughes TJ, Ma J. 2013. Molecular mapping of two genes conferring resistance to Phytophthora sojae in a soybean landrace PI 567139B. Theor Appl Genet. 126:2177–2185.
- Lohnes D, Nickell D, Schmitthenner AF. 1996. Origin of soybean alleles for Phytophthora resistance in China. Crop Sci. 36:1689–1692.
- Lohnes DG, Schmitthenner AF. 1997. Position of the Phytophthora resistance gene Rps7 on the soybean molecular map. Crop Sci. 37:555–556.
- Malvick DK, Grunden E. 2004. Traits of soybean-infecting Phytophthora populations from Illinois agricultural fields. Plant Dis. 88:1139–1145.
- Matthiesen RL, Abeysekara NS, Ruiz-Rojas JJ, Biyashev RM, Maroof MAS, Robertson AE. 2016. A method of combining isolates of Phytophthora sojae to screen for novel sources of resistance to Phytophthora stem and root rot in soybean. Plant Dis. 100:1424–1428.
- McBlain BA, Fioritto RJ, St. Martin SK, Calip-DuBois A, Schmitthenner AF, Cooper RL, Martin RJ. 1990. Registration of ‘Resnik’ soybean. Crop Sci. 30:424–425.
- Miao J, Dong X, Lin D, Wang Q, Liu P, Chen F, Du Y, Liu X. 2016. Activity of the novel fungicide oxathiapiprolin against plant-pathogenic oomycetes. Pest Manage Sci. 72:1572–1577.
- Mideros S, Nita M, Dorrance AE. 2007. Characterization of components of partial resistance, Rps2, and root resistance to Phytophthora sojae in soybean. Phytopathology. 97:655–662.
- Miller SA, Madden LV, Schmitthenner AF. 1997. Distribution of Phytophthora spp. In Field Soils Determined by Immunoassay. Phytopathology. 87:101–107.
- Morris PF, Ward EWB. 1992. Chemoattraction of zoospores of the soybean pathogen, Phytophthora sojae, by isoflavones. Physiol Mol Plant Pathol. 40:17–22.
- Na R, Gijzen M. 2016. Escaping host immunity: New tricks for plant pathogens. PLoS Pathog. 12(7):e1005631.
- Na R, Yu D, Chapman BP, Zhang Y, Kuflu K, Austin R, Qutob D, Zhao J, Wang Y, Gijzen M. 2014. Genome re-sequencing and functional analysis places the Phytophthora sojae avirulence genes Avr1c and Avr1a in a tandem repeat at a single locus. PLoS One. 9:e89738.
- Na R, Yu D, Qutob D, Zhao J, Gijzen M. 2013. Deletion of the Phytophthora sojae avirulence gene Avr1d causes gain of virulence on Rps1d. Mol Plant-Microbe Interact. 26:969–976.
- Nelson BD, Mallik I, McEwen D, Christianson T. 2008. Pathotypes, distribution, and metalaxyl sensitivity of Phytophthora sojae from North Dakota. Plant Dis. 92:1062–1066.
- Nguyen VT, Vuong TD, Van Toai T, Lee JD, Wu X, Mian MAR, Dorrance AE, Shannon JG, Nguyen HT. 2012. Mapping of quantitative trait loci associated with resistance to Phytophthora sojae and flooding tolerance in soybean. Crop Sci. 52:2481–2493.
- Niu J, Guo N, Sun J, Li L, Cao Y, Li S, Huang J, Zhao J, Zhao T, Xing H. 2017. Fine mapping of a resistance gene RpsHN that controls Phytophthora sojae using recombinant inbred lines and secondary populations. Front Plant Sci. 8:538.
- Qutob D, Chapman BP, Gijzen M. 2013. Transgenerational gene silencing causes gain of virulence in a plant pathogen. Nat Comm. 4:1349.
- Qutob D, Tedman-Jones J, Dong S, Kuflu K, Pham H, Wang Y, Dou D, Kale SD, Arredondo FD, Tyler BM, et al. 2009. Copy number variation and transcriptional polymorphisms of Phytophthora sojae RXLR effector genes Avr1a and Avr3a. PLoS One. 4(4):e5066.
- Radmer L, Anderson G, Malvick DM, Kurle JE. 2017. Pythium, Phytophthora, and Phytopythium spp. associated with soybean in minnesota, their relative aggressiveness on soybean and corn, and their sensitivity to seed treatment fungicides. Plant Dis. 101:62–72.
- Ranathunge K, Thomas RH, Fang X, Peterson CA, Gijzen M, Bernards MA. 2008. Soybean root suberin and partial resistance to root rot caused by Phytophthora sojae. Phytopathology. 98:1179–1189.
- Reeser PQ, Scott DH, Ruhl DE. 1991. Recovery of race non-classifiable Phytophthora megasperma f. sp. glycinea from soybean roots in Indiana in 1990. Phytopathology. 81:1201.
- Robertson AE, Cianzio SR, Cerra SM, Pope RO. 2009. Within-field pathogenic diversity of Phytophthora sojae in commercial soybean fields in Iowa. Plant Health Progress. doi:10.1094/PHP-2009-0908-01-RS
- Rojas JA, Jacobs JL, Napieralski Karaj B, Bradley CA, Chase T, Esker PD, Giesler LJ, Jardine DJ, Malvick DK, Markell SG, et al. 2017. Oomycete species associated with soybean seedlings in North America—Part I: Identification and pathogenicity characterization. Phytopathology. 107:280–292.
- Ryley MJ, Obst NR, Irwin JAG, Drenth A. 1998. Changes in the racial composition of Phytophthora sojae in Australia between 1979 and 1996. Plant Dis. 82:1048–1054.
- Sahoo DK, Abeysekara NS, Cianzio SR, Robertson AE, Bhattacharyya MK. 2017. A novel Phytophthora sojae resistance Rps12 gene mapped to a genomic region that contains several Rps genes. PLoS ONE. 12:e0169950.
- Schmitthenner AF. 1985. Problems and progress in control of Phytophthora root rot of soybean. Plant Dis. 82:1048–1054.
- Schmitthenner AF, Van Doren DM. 1985. Integrated control of root rot of soybean caused by Phytophthora megasperma f. sp. glycinea. In: Parker CA, Rovira AD, Morre KJ, Wong PTW, editors. Ecology and Management of soilborne Plant Pathogens. St. Paul (MN): American Phytopathological Society; p. 263–266.
- Schneider R, Rolling W, Song Q, Cregan P, Dorrance AE, McHale L. 2016. Genome wide association mapping of partial resistance to Phytophthora sojae in soybean plant introductions from the Republic of Korea. BMC Genomics. 17:607.
- Shan W, Cao M, Leung D, Tyler BM. 2004. The Avr1b locus of Phytophthora sojae encodes an elicitor and a regulator required for avirulence on soybean plants carrying resistance gene Rps1b. Mol Plant-Microbe Interact. 17:394–403.
- Slaminko TL, Bowen CR, Hartman GL. 2010. Multi-year evaluation of commercial soybean cultivars for resistance to Phytophthora sojae. Plant Dis. 94:368–371.
- Song T, Kale SD, Arredondo FD, Shen D, Su L, Liu L, Wu Y, Wang Y, Dou D, Tyler BM. 2013. Two RxLR avirulence genes in Phytophthora sojae determine soybean Rps1k-mediated disease resistance. Mol Plant-Microbe Interact. 26:711–720.
- St Martin SK, Scott DR, Schmitthenner AF, McBlain BA. 1994. Relationship between tolerance to Phytophthora rot and soybean yield. Plant Breed. 113:331–334.
- Stasko AK, Wickramasinghe D, Nauth BJ, Acharya B, Ellis ML, Taylor CG, McHale L, Dorrance AE. 2016. High density mapping of resistance QTL towards Phytophthora sojae, Pythium irregulare, and Fusarium graminearum in the same soybean population. Crop Sci. 56:2476–2492.
- Stewart S, Robertson AE. 2012. A modified method to screen for partial resistance for Phytophthora sojae in soybean. Crop Sci. 52:1181–1186.
- Stewart S, Robertson AE, Wickramasinghe D, Draper MA, Michel A, Dorrance AE. 2016. Population structure among and within Iowa, Missouri, Ohio, and South Dakota populations of Phytophthora sojae. Plant Dis. 100:367–379.
- Sugimoto T, Yoshida S, Aino M, Watanabe K, Shiwaku K, Sugimoto M. 2006. Race distribution of Phytophthora on soybean in Hyogo, Japan. J Gen Plant Pathol. 72:92–97.
- Sugimoto T, Youshida S, Kaga A, Hajika M, Watanabe K, Aino M, Tatsuda K, Yamamoto R, Matoh T, Walker DR, et al. 2011. Genetic analysis and identification of DNA markers linked to a novel Phytophthora sojae resistance gene in the Japanese soybean cultivar Waseshiroge. Euphytica. 182:122–145.
- Sun S, Wu XL, Zhao JM, Wang YC, Tang QH, Yu DY, Gai JY, Xing H. 2011. Characterization and mapping of RpsYu25, a novel resistance gene to Phytophthora sojae. Plant Breeding. 130:139–143.
- Thomas R, Fang X, Ranathunge K, Anderson TR, Peterson CA, Bernards MA. 2007. Plant Physiol. 144:299–311.
- Tooley PW, Grau CR. 1984. Field characterization of rate-reducing resistance to Phytophthora megasperma f.sp. glycinea in soybean. Phytopathology. 74:1201–1208.
- Tucker DM, Maroof MAS, Mideros S, Skoneczka JA, Nabati DA, Buss GR, Hoeschele I, Tyler BM, St. Martin SK, Dorrance AE. 2010. Mapping quantitative trait loci for partial resistance to Phytophthora sojae in a soybean interspecific cross. Crop Sci. 50:628–635.
- Tyler BM, Wu M, Wang J, Cheung W, Morris PF. 1996. Chemotactic preferences and strain variation in the response of Phytophthora sojae zoospores to host isoflavones. Appl Environ Microbiol. 62:2811–2817.
- Wang H, St. Martin SK, Dorrance AE. 2012a. Comparison of phenotypic methods and yield contributions of quantitative trait loci for partial resistance to Phytophthora sojae in soybean. Crop Sci. 52:1–14.
- Wang H, Waller L, Tripathy S, St. Martin SK, Zhou L, Krampis K, Tucker DM, Mao Y, Hoeschele I, Saghai Maroof MA, et al. 2010. Analysis of genes underlying soybean quantitative trait loci conferring partial resistance to Phytophthora sojae. Plant Genome. 3:23–40.
- Wang H, Wijeratne A, Wijeratne S, Lee S, Taylor C, St. Martin SK, McHale L, Dorrance AE. 2012b. Dissection of two soybean QTL conferring partial resistance to Phytophthora sojae through sequence and gene expression analysis. BMC Genomics. 13:428.
- Weng C, Yu K, T R A, Poysa V. 2001. Mapping genes conferring resistance to Phytophthora root rot of soybean, Rps1a and Rps7. J Hered. 92:442–446.
- Workneh F, Tylka GL, Yang XB, Faghihi J, Ferris JM. 1999. Regional assessment of soybean brown stem rot, Phytophthora sojae, and Heterodera glycines using area-frame sampling: Prevalence and effects of tillage. Phytopathology. 89:204–211.
- Workneh F, Yang XB, Tylka GL. 1998. Effect of tillage practices on vertical distribution of Phytophthora sojae. Plant Dis. 82:1258–1263.
- Wrather JA, Koenning SR. 2006. Estimates of disease effects on soybean yields in the United States from 2003 to 2005. J Nematol. 38:173–180.
- Wrather JA, Koenning SR. 2009. Effects of diseases on soybean yields in the United States 1996 to 2007. Online. Plant Health Progress. doi:10.1094/PHP-2009-0401-01-RS
- Wrather JA, Stienstra WC, Koenning SR. 2001. Soybean disease loss estimates for the United States from 1996 to 1998. Can J Plant Pathol. 23:122–131.
- Wu XL, Zhang BQ, Sun S, Zhao JM, Yang F, Guo N, Gai JY, Xing H. 2011. Identification, genetic analysis and mapping of resistance to Phytophthora sojae of Pm28 in soybean. Agric Sci China. 10:1506–1511.
- Xue AG, Marchand G, Chen Y, Zhang S, Cober ER, Tenuta A. 2015. Races of Phytophthora sojae in Ontario, Canada, 2010–2012. Can J Plant Pathol. 37:376–383.
- Yin W, Dong S, Zhai L, Lin Y, Zheng X, Wang Y. 2013. The Phytophthora sojae Avr1d encodes an RxLR-dEER effector with presence and absence polymorphisms among pathogen strains. Mol Plant-Microbe Interact. 26:958–968.
- Zelaya-Molina LX, Ellis ML, Berry SA, Dorrance AE 2010. First report of Phytophthora sansomeana causing wilting and stunting of soybean in Ohio. Plant Dis. 94:125.
- Zhang J, Xia C, Duan C, Sun S, Wang X, Wu X, Zhu Z. 2013. Identification and candidate gene analysis of a novel Phytophthora resistance gene Rps10 in a Chinese soybean cultivar. PLoS ONE. 8(7):e69799.
- Zhang S, Xu P, Wu J, Xue AG, Zhang J, Li W, Chen C, Chen W, Lu H. 2010. Races of Phytophthora sojae and their virulences on soybean cultivars in Heilongjiang, China. Plant Dis. 94:87–91.
- Zhou L, Mideros SX, Bao L, Hanlon R, Arredondo FD, Tripathy S, Krampis K, Jerauld A, Evans C, St. Martin SK, et al. 2009. Infection and genotype remodel the entire soybean transcriptome. BMC Genomics. 10:49.